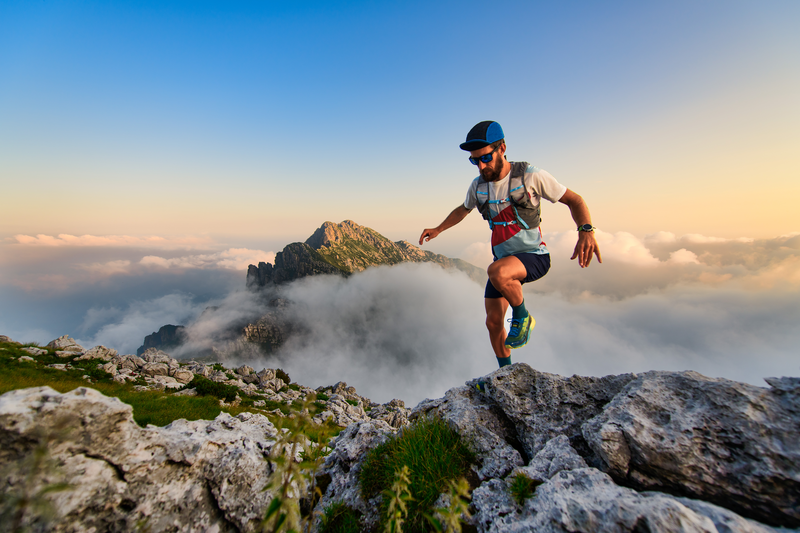
95% of researchers rate our articles as excellent or good
Learn more about the work of our research integrity team to safeguard the quality of each article we publish.
Find out more
SYSTEMATIC REVIEW article
Front. Vet. Sci. , 20 November 2024
Sec. Animal Nutrition and Metabolism
Volume 11 - 2024 | https://doi.org/10.3389/fvets.2024.1484870
This article is part of the Research Topic Using eco-friendly feedstuffs in ruminants to achieve a cleaner environment and reduced carbon footprint View all 8 articles
There has been a growing interest in using insects as sustainable protein sources for ruminant feed, such as the adults of the two-spotted cricket (Gryllus bimaculatus), larvae of the mealworm beetle (Tenebrio molitor), black soldier fly (Hermetia illucens), and pupae of the silkworm (Bombyx mori). The advantages of these insects over other plant materials lie in their elevated levels of crude protein and fat. However, this interest lacks a comprehensive understanding of the impact of insects on the ruminal fermentation processes, including digestibility and gas production, as well as the impact on animal performance and related health aspects. This review offers a comprehensive analysis of ruminal fermentation indices across diverse insect species. Employing descriptive and meta-analysis methodologies, we examined the impact of incorporating insect-derived meals in ruminants’ diets. Moreover, we evaluated the growth performance and biochemical parameters of blood in ruminants when species such as Tenebrio molitor, Hermetia illucens, Oriental Hornet (Vespa Orientalis), and Bombyx mori were incorporated into ruminants’ diets. The meta-analysis was performed on a limited dataset of 14 in vitro and eight in vivo trials, investigating insect meal as a potential feed source. A comparison is drawn between these insect-based feeds and conventional dietary sources such as soybean meal, alfalfa hay, and commercial concentrate diets. Our meta-analysis revealed that incorporating Gryllus bimaculatus and Hermetia illucens to partially replace protein sources in ruminants’ diet did not adversely affect digestibility, ruminal fermentation, and ruminant production, supporting the feasibility as a feed ingredient for ruminant animals. In addition, the oriental hornet showed an overall higher outcome on the final BW, ADG, digestibility, and volatile fatty acid (VFA) production, suggesting the promising effect of this insect for future use in ruminants. The data also indicates that dietary insect inclusion levels should not exceed 30% (DM basis) to achieve an optimal ruminal fermentation profile. Furthermore, it offers comparative insights into the nutritional value of these insects, which warrant further investigation at the in vivo level. Ultimately, the existing understanding of the nutritional utilization potential of these insects by ruminants, particularly concerning macro- and micronutrients, is evaluated and revealed to be significantly constrained.
By the year 2050, the global human population is projected to reach approximately 9.5 billion, necessitating a corresponding 70% increase in demand for animal-based food production, such as milk and meat (1). The primary livestock categories include pigs, with a production of 112.33 million metric tons (MT); poultry, with 109.02 million MT; and cattle (including beef and buffalo meat), with 67.99 million MT, collectively representing 91.80% of global meat production (2). As the most populous country globally, China has a significant demand for livestock products, particularly those derived from ruminant animals. Consequently, this surge in demand for animal-derived products may escalate the need for livestock feed (3). Providing sufficient feed for livestock is anticipated to encounter challenges as available land for cultivating feed resources diminishes. Intensive livestock production systems heavily depend on soybean meal (SBM) as a primary source of protein and essential amino acids (4). Nevertheless, its extensive use raises concerns regarding environmental sustainability and its competition with human nutrition (5).
Insects represent promising and innovative feed ingredients due to their valuable chemical composition. They are notably rich in proteins and contain significant amounts of lipids, making them suitable as protein and energy sources in animal dietary formulations (6). Insects have been incorporated as a feed ingredient in various animal species, including broiler chickens (7), laying hens (8), turkey (9), ducks (10), quail (11), rabbit (12), swine (13), companion animals (14), and aquatic species (15, 16). Despite their widespread use across these species, the utilization of insects in ruminant diets has been relatively limited. The limited adoption of insects in ruminant diets may be attributed to concerns regarding the potential risk of transmitting bovine spongiform encephalopathy (mad cow disease) despite the absence of evidence supporting such a linkage to date (17). While ruminants primarily consume grasses, legumes, and agricultural by-products, they frequently require protein supplements to enhance their production efficiency (18).
Moreover, feed expenses represent a significant constraint on advancing the livestock production industry. Feed costs typically constitute around 50–70% of the total budget, with protein requirements alone accounting for more than 15% of the overall feed expenditure (19). Hence, to address the rising demand for animal products in the coming years, there is an urgent need for innovative solutions and sustainable alternatives to traditional protein sources in animal diets, aiming to minimize environmental impact. The utilization of insects as animal feed offers substantial environmental benefits compared to conventional sources. Insects play a key role in bioconverting waste materials, require less water and land for cultivation, and contribute to lower greenhouse gas (GHG) emissions (5). Therefore, this development has prompted policymakers in the European Union to approve the use of insects as feed for pigs and poultry in April 2021, in addition to their existing approval for aquaculture since July 2017 (20). Currently, there is limited available data on the utilization and effects of insects as alternative feed for ruminants. Therefore, further scientific research is needed to raise awareness of this topic among policymakers in Europe, China, and globally and to assist in establishing a regulatory framework for licensing insects as ruminant feed (21).
The utilization of insects as feed for monogastric animals has been extensively reviewed (22). However, there remains a gap in applying insects as feed for ruminants. In Asia, Africa, Oceania, and South America, insects have historically served as traditional food sources within these regions. They have recently garnered interest as alternative protein sources in additional regions, including Europe and North America (23). In the United States, the black soldier fly (Hermetia illucens) is utilized exclusively in aquaculture. In Canada, the use of Hermetia illucens larvae is approved for both aquaculture and poultry production. Brazil currently lacks specific legislation addressing this matter, with insects permitted only for feeding non-ruminant animals (24). In countries like China and South Korea, this matter has no restrictions or limitations (25).
Presently, there is a lack of statistical data regarding the commercial rearing of insects. However, numerous countries have begun farming insects like crickets for the feed market. Annual insect meal production is anticipated to increase to 1.2 million tons by 2025 (26). Cricket presents promising potential as an alternative feed resource for animals. Figure 1 illustrates the detailed life cycle of selected insect species in this context. Typically, crickets are reared for approximately 5–6 reproductive cycles before being discarded due to diminished productivity. These discarded crickets can subsequently be utilized as animal feed (27). The black soldier fly (Hermetia illucens) exhibits a life cycle lasting approximately 40–43 days (28). Research interest in using edible insects as alternative feed has surged recently. As Hanönü et al. (29) reported, insect farming emerges as a more cost-effective option when evaluating land allocation for forage crop cultivation and their associated water requirements. Insects exhibit efficient feed conversion rates and rapid growth rates. It is estimated that approximately 2 kg of organic waste and 1 m2 of space could yield 1 kg of insect protein. In particular, Hermetia illucens has gained increasing commercial utilization in animal feed due to its ease of rearing, high productivity, rich nutritional content, and efficient organic waste utilization. Hermetia illucens larvae have demonstrated the capacity to consume substrate ranging from 25 mg to 500 mg of fresh matter per larva per day, achieving a body length of approximately 27 mm, width of 6 mm, and weight of 220 mg by 14 days of age (30).
Figure 1. Schematic processes indicating a complete life cycle throughout major developmental Gryllus bimaculatus, Tenebrio molitor, and Bombyx mori; wk., week; *up to value, for example, in Gryllus bimaculatus, the egg-laying stage lasts from 0 to 9.55 h, etc.
Moreover, Indonesia, characterized by its archipelagic geography and tropical climate, offers favorable conditions for Hermetia illucens production. Data from the Indonesian Ministry of Fisheries in 2021 indicate the presence of over 175 Hermetia illucens farmers spanning from the Sumatra Island (western) to the Papua Island (eastern) regions, with an average production rate of 100 kg per day (2). One challenge associated with small-scale production is the cost factor. Implementing good manufacturing practices could help mitigate the production costs of insects. Numerous insect species have undergone evaluation as potential components of ruminant diets, with notable candidates including the larvae of the mealworm beetle (Tenebrio molitor), pupae of the silkworm (Bombyx mori), larvae of the black soldier fly (Hermetia illucens), and adult two-spotted cricket (Gryllus bimaculatus) (Figure 2). However, research into consumer and stakeholder perspectives regarding the use of insects in farm animal diets remains limited. The chemical composition and average nutritional values of commonly studied insects used in ruminant nutrition research, such as Gryllus bimaculatus adults, Tenebrio molitor larvae, Hermetia illucens larvae, and Bombyx mori pupae are fully described in Table 1.
Figure 2. The number of original scientific research articles (excluding review articles) specifically investigating the relationship between insects and ruminant nutrition was determined using Google Scholar, Science Direct, PubMed, Web of Science, and Scopus databases. The search utilized the following keywords: (A) “insect, rumen” or (B) the names of insect species previously studied concerning ruminants by researchers. The highlighted insect species (bolded lines—Gryllus bimaculatus, Tenebrio molitor, Hermetia illucens, and Bombyx mori) were emphasized due to their frequent study in this context.
Table 1. The nutritive value variability of the selected insect species1.
Consequently, this article is structured as follows: following the introduction, the second section discusses insects’ effects on in vitro ruminal fermentation characteristics, mainly focusing on ruminal digestibility and gas production in vitro. The third section is centered on the evidence of in vivo studies investigating insects on ruminants, including the impacts on ruminal fermentation, productive performance, and health. The fourth section presents an economic evaluation of insect protein compared to alternative protein sources. The fifth section then thoroughly examines the legislative framework necessary for introducing a novel protein source into the specific sector of ruminant nutrition, specifically scrutinizing the governmental regulations governing insect utilization in the European Union. The sixth section delves into ethical considerations surrounding the use of insects. The seventh section summarizes the current research gaps and outlines future directions for applying insects in ruminant nutrition. Subsequently, the eighth section (Supplementary material 1) offers insights into statistical modeling and prediction of the optimal inclusion level of insects in the ruminants’ diet based on in vitro and in vivo studies, focusing on crucial aspects of ruminal fermentation driven by data availability. Finally, the conclusion will recapitulate the existing challenges and propose avenues for future research in this domain.
Supplementary Tables S1, S2 (Supplementary material 2) presents the in vitro experiments examined in this review regarding the impact of different insects on ruminal fermentations. The detailed ruminal in vitro fermentation profiles of specific insects, Gryllus bimaculatus and Bombyx mori, have been outlined in Supplementary Table S6 (Supplementary material 2). Figure 3 illustrates the ruminal fermentation metrics based on in vitro and in vivo studies examining the effects of various insect species and morphological stages. In a study conducted by Renna et al. (5), the in vitro ruminal fermentation characteristics were examined after 24 h of incubation using Gryllus bimaculatus adult meal as the incubation substrate, compared with control meals. The findings revealed significant reductions in total gas production with Gryllus bimaculatus adult meal treatments, showing 72.6, 70.6, and 57.3% for soybean, rapeseed, and sunflower meals, respectively. Similarly, methane (CH4) production was notably lower with Gryllus bimaculatus adult meal treatments, with reductions of 79, 73.9, and 62.4% for meals of soybean, rapeseed, and sunflower, respectively. However, due to high fat and chitin content, the in vitro organic matter digestibility (IVOMD) in Gryllus bimaculatus adult meal treatments also decreased significantly by 45, 36, and 21% compared to soybean meal, rapeseed meal, and sunflower meal, respectively. Furthermore, the total saturated fatty acid (SFA) content was significantly lower by 6.79% compared to the soybean meal group. Nonetheless, the digestibility of Gryllus bimaculatus adult meal was relatively low, potentially limiting their utilization as feed ingredients.
Figure 3. The comprehensive assessment of the impact of various insect species on ruminant nutrition, as examined through both in vitro and in vivo studies; NH3, Ammonia; TVFA, Total volatile fatty acids; C2, Acetate; C3, Propionate; C4, Butyrate; DMD, Dry matter digestibility; OMD, Organic matter digestibility; NDFD, Neutral detergent fiber digestibility; ADFD, Acid detergent fiber digestibility; TGP, Total gas production; DM, Dry matter; DDM, Degraded dry matter; CH4, Methane; CO2, Carbon dioxide. The value means of the corresponding measurements obtained from the original data gathered across all investigations encompassing insects on ruminants, as examined in this paper (both in vitro and in vivo), were depicted graphically.
To enhance the feeding value of Gryllus bimaculatus adult meals, specific treatments or processing methods targeting the removal of the exoskeleton fraction or chitin may be necessary. Comparative analysis of these findings with other studies demonstrates the impact of cricket exoskeleton removal through manual methods (removing the head, legs, and wings of oven-dried crickets) or chemical extraction (delipidation), resulting in a chitin reduction of 54.5 and 100%, respectively. When used as the sole substrate for in vitro ruminal fermentation, exoskeleton removal and chemical extraction of crickets were found to increase in vitro dry matter digestibility—IVDMD (and IVOMD) by 1.9% (2%) and 2% (1.7%) compared to whole cricket meal, respectively. Furthermore, in the same study, researchers reported that using crickets after exoskeleton removal and whole cricket meal to fully replace SBM at an inclusion level of 30% in the diet increased IVDMD by 1.9% and IVOMD by 2.7%, respectively. However, these increases were not statistically significant (27). In a study conducted by Ahmed et al. (19), it was observed that supplementing the diet with 10% Gryllus bimaculatus adult meal resulted in the replacement of 25% of SBM in the control group. This substitution significantly reduced total gas production by 16.5 and 12.1% per gram of DM and digestible dry matter (DDM), respectively. CH4 production also decreased significantly by 26.7 and 22.5%, respectively. Interestingly, the digestibility of DM, OM, NDF, and ADF did not exhibit significant differences and yielded similar results between the experimental and control groups. These findings suggest that the investigated insects could be a sustainable alternative to replace 25% of the high-quality and expensive protein source, soybean meal, at a 10% inclusion level without inducing any adverse effects.
These findings highlight the potential of using Gryllus bimaculatus adult meal as a viable and environmentally friendly protein source in livestock feed formulations. Further research is warranted to explore long-term effects on animal performance and health. The optimal inclusion levels of Gryllus bimaculatus adult’s meal in ruminant diets have been demonstrated to play a pivotal role. In a recent study by Khonkhaeng et al. (31), the inclusion of Gryllus bimaculatus adult meal in ruminant diets was examined across a range from 65.1% to 70% by eight treatments, with a gradient increase of 0.7% for each treatment. The authors observed that when Gryllus bimaculatus adult meals were included at levels up to 67.9% in the diet, there was a significant linear decrease in IVOMD without affecting total gas production. However, concerning IVDMD, it was suggested that Gryllus bimaculatus adult meal in ruminant diets should be maintained at levels below 65.1% to avoid compromising IVDMD. This outcome is contrary to that of Ahmed and Nishida (1), who observed a linear decrease in IVDMD with the inclusion of Gryllus bimaculatus adults at 30% of the diet compared to the control group; this study aligns with existing literature. Specifically, it observed reductions of 16.6% in total gas production and 12.5% in CH4 production, consistent with prior research. Hence, the authors concluded that including Gryllus bimaculatus adult meal at up to 20% of the diet did not adversely affect nutrient digestibility.
To better understand the ideal inclusion levels, future trials could explore varying forage-to-concentrate (F:C) ratios, as investigated in studies such as Khonkhaeng et al. (31) (F:C 70:30) and Ahmed and Nishida (1) (F:C 60:40). These additional investigations would contribute valuable insights into optimizing Gryllus bimaculatus adult meal inclusion in ruminant diets. In a previous study emphasizing the significance of F:C ratios, researchers investigated the substitution of Gryllus bimaculatus adult meal for SBM at varying levels (25, 50, 75, and 100% replacement) that corresponded to inclusion levels of Gryllus bimaculatus adult meal in the diet at 4, 8, 12, and 16%, respectively. The F:C ratios were expressly set at 60:40 and 40:60. The study revealed that IVDMD was significantly higher with a F:C ratio of 40:60 compared to the corresponding ratio of 60:40. Similarly, a reduction in CH4 production was observed when the F:C ratio was decreased while maintaining the same level of Gryllus bimaculatus adult meal in the diet (23). These findings underscore the importance of F:C ratios in optimizing nutrient utilization and CH4 emissions in ruminant diets supplemented with Gryllus bimaculatus adult meal. In summary, these findings underscore the need for further investigation, including feeding trials in vivo, to better understand and optimize the utilization of Gryllus bimaculatus adult meal as a potential feed resource.
Regarding the impact of Tenebrio molitor larvae meal on ruminal fermentation characteristics, this study revealed notable effects when used as the sole substrate. Tenebrio molitor larvae meal significantly reduced total gas production by 68.6, 66.2, and 51% compared to soybean, rapeseed, and sunflower meals, respectively. However, IVOMD decreased by 41, 32, and 17% compared to soybean, rapeseed, and sunflower meals, respectively. Additionally, the SFA content in the ruminal fluid was reduced by 53.2, 44, and 41.1% when compared to soybean (SBM), rapeseed, and sunflower meals, respectively. These outcomes are attributed to the higher fat content in Tenebrio molitor larvae meal (39.2%) compared to SBM (0.6%), rapeseed meal (2.8%), and sunflower meal (1.7%) (5). This discovery aligns with the findings of Jayanegara et al. (3), who reported that the higher fat content in Tenebrio molitor meal (20.3%) compared to SBM (2.7%) led to a significant reduction in IVDMD and IVOMD by 29.2 and 26.1%, respectively. Moreover, total gas and CH4 production were significantly decreased by 46.7 and 55.1%, respectively, when Tenebrio molitor meal was used as the sole substrate during 24-h anaerobic in vitro fermentation. A noteworthy discovery emerged when comparing Tenebrio molitor with two other non-plant protein sources: grasshopper meal (Melanoplus sanguinipes) and ant egg meal (Solenopsis invicta). This study observed that in vitro ruminal fermentation decreased total gas and CH4 production with these alternative protein sources while maintaining IVDMD (32). The research conducted by Hanönü et al. (29) demonstrated that supplementing alfalfa hay with Tenebrio molitor larvae meal at levels of 0.5, 1, and 1.5% led to a significant increase in IVOMD, both linearly and quadratically. A possible explanation for this effect could be attributed to the in situ ruminal dry matter (DM) digestibility of Tenebrio molitor larvae meal, which was determined to be 85.7% after 24 h, surpassing alfalfa. The in vitro degradable protein content was similar (around 60%) between SBM and Tenebrio molitor larvae meal (33).
The detailed ruminal in vitro fermentation profiles of specific insects, Hermetia illucens and Acheta domesticus, have been compiled in Supplementary Table S6 (Supplementary material 2). Regarding the impact of Hermetia illucens larvae meal on ruminant nutrition, studies have reported a significant decrease in both IVDMD and IVOMD by 35 and 34%, respectively, when used as the sole substrate compared to SBM after a 48-h incubation period. Additionally, total gas and CH4 production were markedly reduced by 56.3 and 67.5%, respectively, in the Hermetia illucens larvae meal group compared to SBM (3). This finding broadly supports the work of other studies in this area linking Hermetia illucens larvae meal with IVOMD. According to Renna et al. (5), IVOMD was notably lower by 46, 37, and 22% in Hermetia illucens larvae meal compared to SBM, rapeseed meal, and sunflower meal, respectively. Consequently, there was a reduction in total gas production by 71.3, 69.2, and 55.3%, and CH4 production by 77.5, 72.1, and 59.8% by Hermetia illucens larvae compared to SBM, rapeseed meal, and sunflower meal, respectively. This effect is attributed to the high fat (26.9%) and chitin content (5.2%) in Hermetia illucens larvae. Consistent with these findings, a prior study has shown that chemically defatted (using a hexane solution) and mechanically defatted (using an expeller) Hermetia illucens larvae led to significant increases in IVDMD and IVOMD by 26.7% (27.1%) and 14.9% (26.5%), respectively, compared to the intact Hermetia illucens larvae meal group. These effects were observed when these different insect inclusion levels were at 20% in the diet without influencing CH4 production (34). Hence, extracting fat from Hermetia illucens larvae is essential for optimizing the insect’s suitability as a feed ingredient for ruminant livestock. This finding aligns with recent research demonstrating that supplementing the diet with defatted Hermetia illucens larvae meal at 3.2%, representing a 20% substitution for SBM, led to notable increases in in vitro neutral detergent fiber digestibility (IVNDFD) over a 24-h incubation period, following a linear and quadratic trend compared to the control group. Moreover, IVDMD and IVNDFD exhibited enhancements of 6.31 and 4.64%, respectively, with defatted Hermetia illucens larvae meal at 3.2% in the diet during a 48-h incubation period. These outcomes may be attributed to the lower inclusion rate of Hermetia illucens larvae meal (3.2%) without significantly impacting the fat content (3.74%) when compared to the control group’s fat content (3.19%) (24).
In summary, substituting SBM with Hermetia illucens larvae meal in ruminant diets often reduces nutritional quality in vitro. The main challenges associated with incorporating Hermetia illucens larvae meal include their significant chitin content, indicated by elevated levels of neutral detergent insoluble crude protein and acid detergent insoluble crude protein, as well as their high-fat content, which can adversely affect ruminal digestibility. Despite these challenges, a distinct advantage of using Hermetia illucens larvae meal over SBM is their lower CH4 emissions. Enhancing the nutritional value of Hermetia illucens larvae meal requires the application of specific treatments or processing methods.
Ahmed et al. (19) reported that including 10% Bombyx mori pupae meal in the diet, replacing 25% of SBM, did not affect IVDMD, IVOMD, IVNDFD, or in vitro acid detergent fiber digestibility (IVADFD) compared to the control group, which included 40% SBM. However, the production of carbon dioxide (CO2) and CH4 per gram of DDM was significantly reduced by 13.7 and 19.4%, respectively, in the Bombyx mori pupae meal group compared to the control group. Notably, chitin is a component known to be poorly digested by animals and can contribute to lower IVDMD and IVOMD. The chitin content in the Bombyx mori pupae meal was measured at 9.83%. The inclusion of insects at a substitution level of 25% for SBM in this study did not negatively affect nutrient digestibility, likely due to the relatively low inclusion rate employed. Additional research is needed to explore the effects of higher inclusion levels of this insect, mainly when replacing soybean meal entirely. This investigation could assess their potential as effective options for reducing CH4 production in ruminants.
Therefore, Ahmed and Nishida (1) conducted a study examining the inclusion of different levels (10, 20, 30, and 40%) of Bombyx mori pupae meal in the diet. The authors observed that including Bombyx mori pupae meal up to 30% in the diet resulted in a linear and quadratic decrease in IVDMD compared to the control group, which consisted of 300 mg of grass hay and 200 mg of concentrate mixture during a 24-h fermentation period. Furthermore, including 20% Bombyx mori pupae meal in the diet was deemed a safe threshold as it did not significantly impact IVDMD but led to a notable reduction in total gas and CH4 production by 9.2 and 9.9%, respectively. It suggests that a 20% inclusion level of Bombyx mori pupae meal could be a suitable option for minimizing CH4 emissions without affecting DM digestibility. Further trials were conducted using different F:C ratios to better understand the ideal inclusion levels. Based on the findings, it can be concluded that supplementing Bombyx mori pupae oil at a 2% level reduces CH4 production by 12%–15% without negatively impacting feed fermentation. The reduction in CH4 may be more notable when the oil supplement is added to a high-concentrate diet (F:C; 70:30) compared to a diet with a lower concentrate ratio (F:C; 40:60), resulting in reductions of 5.28 and 4.52%, respectively, compared to the control group (no oil supplement). Thirumalaisamy et al. (35) (Supplementary Table S7 in Supplementary material 2) presents the variations in ruminal fermentation parameters observed in response to different insects during the in vitro experiments. Hermetia illucens supplementation led to a notable decrease in acetate production by 34.5% compared to the control group (p = 0.03). As a result, there was a pronounced reduction in the acetate:propionate (C2:C3) ratio (p = 0.03; Supplementary Table S7 in Supplementary material 2).
Supplementary Table S8 (Supplementary material 2) presents descriptive statistics for the variables used in assessing the impact of Hermetia illucens, Tenebrio molitor, Bombyx mori, and Vespa orientalis on blood biochemical parameters. Astuti et al. (36) documented that incorporating cricket meal at a concentration of 30% within the concentrate for post-weaning Etawah crossbred goats resulted in physiological responses (rectal temperature, heart rates, and respiration rate) that fell within normal ranges. However, the experimental group exhibited 182% significantly higher crude fat intake compared to the control group. Importantly, no adverse effects on ruminal fermentation profiles were observed, and the goats in the experimental group performed comparably to those on the control ration. These findings are consistent with earlier observations indicating that incorporating 15% cricket meal (replacing 100% soybean meal) as a protein source in lamb rations does not adversely affect palatability, performance, digestibility of DM and crude protein, feed efficiency, or blood metabolite profiles (including glucose, triglycerides, and total protein).
Furthermore, utilizing 7.5% cricket meal in lamb rations has been shown to reduce CH4 production, as reported by the authors significantly. Therefore, replacing soybean meal with 7.5% cricket meal may be more advantageous, considering the positive impact on CH4 reduction (37). Another example of this is the study carried out by Phesatcha et al. (4), which demonstrated that incorporating adult cricket meal (Gryllus bimaculatus) at 8% of the ration resulted in a significant linear increase of 25.6% in average daily gain and 7.46% in apparent digestibility of crude protein in Thai native male beef cattle. This increase was accompanied by linearly significant rises in rumen ammonia-nitrogen (26.5%) and blood urea nitrogen (6.4%). Furthermore, total volatile fatty acids (TVFA) were linearly increased by 26.5%, predominantly due to a 4.2% higher propionic acid level compared to the control group when cricket meal was included at 12% of the ration. Their study highlighted that cricket meal had a CP content of 62.4%, higher than soybean meal (SBM), influencing the alteration in TVFA.
Consequently, the C2:C3 ratio was significantly reduced. Moreover, estimated CH4 emissions decreased by 20.9%, partially explained by a 35.9% decrease in protozoa when cricket meal completely replaced SBM in the ration. These findings suggest the potential benefits of cricket meal in improving cattle performance and reducing CH4 emissions in feed formulations. Moreover, several research studies have recently investigated the use of defatted silkworm pupae meal in ruminant nutrition. Rashmi et al. (38) conducted a study that concluded that defatted silkworm pupae meal could be safely included at a level of 4.1% in cattle concentrate mixtures (substituting soybean meal up to 30%) without adverse effects on health or performance. This finding suggests that defatted silkworm pupae meal is a promising alternative to traditional protein sources for cattle, offering both nutritional benefits and cost advantages. A notable aspect of their study is the cost-effectiveness of defatted silkworm pupae meal compared to soybean meal. The price of defatted silkworm pupae meal was found to be 51.2% lower than soybean meal when calculated on per kilogram of crude protein basis. This cost advantage further enhances the appeal of defatted silkworm pupae meals as a viable protein source for cattle feed formulations. A notable finding from the earlier-reported results highlights the effective use of silkworm pupae oil to enhance ether extract digestibility by approximately 10% and reduce enteric CH4 emissions by 17.5%–20.5%. These improvements were achieved without compromising nutrient intake or digestibility when oil supplementation was administered continuously (daily) or intermittently (alternate week) at a consistent level of 2% of the diet.
Furthermore, the observed reduction in CH4 emissions is attributed to a decrease in protozoa population. Expressly, significant decreases were noted in total protozoa (39.8%–42%) and Isotrichidae (40.3%–41.8%) (39). These findings align with a meta-analysis by Dai et al. (40), which demonstrated that CH4 emissions correlate positively with total rumen protozoa and Isotrichidae but not with Ophyroscolecidae. In summary, using silkworm pupae oil as a supplement in livestock diets shows promise for improving nutrient digestibility and reducing CH4 emissions through targeted modulation of rumen microbial populations. Further research could contribute valuable insights into sustainable livestock production practices.
Only one study has explored the effects of Oriental Hornet meal on lamb nutrition (41). The findings from this study revealed significant improvements in the digestibility of DM, organic matter (OM), crude protein, and ether extract when Oriental Hornet meal was included at a level of 3.42% of the ration. Specifically, digestibility increased by 2.32, 2.99, 9.74, and 1.93%, respectively. Moreover, including Oriental Hornet meal at this level led to notable enhancements in average body weight gain (30.9%) and growth rate (30.7%) compared to the control group. This improvement can be attributed to the higher total digestible nutrients and digestible crude protein content in the experimental ration, which were 1.56 and 1.43% higher than the control group, respectively, due to the substitution of Oriental Hornet meal for SBM. An intriguing finding was the significantly increased economic efficiency of 19.1% observed in the experimental group compared to the control group. This higher economic efficiency suggests a higher net return from using Oriental Hornet meal, making it potentially well-suited for the Egyptian market. In summary, the limited study on Oriental Hornet meal in lamb nutrition demonstrated promising effects on digestibility, growth performance, and economic efficiency. Further research could provide valuable insights into the potential utilization of Oriental Hornet meals as a cost-effective and beneficial protein source for ruminants, particularly in specific regional markets like Egypt (41).
In addition, recent research comparing the supplementation of 4% Hermetia illucens oil to sheep ration vs. no supplementation has shown significant increases in both TVFA and total bacteria in the ruminal fluid, with increments of up to 44.8 and 77.1%, respectively (28). The variation in total bacterial population can be attributed to several factors, including differences in rations, types of feed, timing and methods of rumen fluid collection, and feeding frequency. Rations containing easily digestible protein and carbohydrates promote bacterial growth in the rumen. In the study by Ningsih et al. (28), the experimental diets exhibited a total digestible nutrients (TDN) content up to 5% higher than the control meal. This increase likely contributed to the observed rise in the TVFA in the rumen, presumably due to the higher bacterial population resulting from the addition of black soldier fly oil supplementation. Consistent with the findings of this study, previous research has shown that the addition of Hermetia illucens fat at a level of 0.2% in the ration of multiple-breeding black-motley cows resulted in a significant increase in TVFA production in the rumen (42).
Recent investigations have explored the impact of incorporating Hermetia illucens meal into sheep nutrition. Researchers observed that replacing soybean meal with black soldier fly larvae did not negatively affect the performance or hematological profile of the sheep. Notably, body weight gain tended to increase (p = 0.082), and feed conversion ratio tended to decrease (p = 0.089) when Hermetia illucens larvae meal was included at 2.5 and 5% of the ration, respectively. Furthermore, analysis of blood leukocyte differentiation, including lymphocytes, monocytes, neutrophils, eosinophils, and basophils, showed no significant differences, indicating that all animals maintained a healthy status (43). Because lymphocytes play a central role in adaptive immunity, recognizing and targeting specific pathogens. Monocytes can differentiate into macrophages upon entering tissues, where they play a vital role in engulfing and digesting pathogens. Neutrophils, the most abundant white blood cells, act as the body’s primary defense against infections by engulfing and destroying bacteria through phagocytosis. Eosinophils combat parasitic infections and regulate allergic responses by releasing toxic proteins. Basophils release histamine and other chemicals involved in allergic reactions, contributing to the inflammatory response and defense against certain parasites (44).
Moreover, Supplementary Tables S1–S5 (Supplementary material 2) contains a comprehensive list of both in vitro and in vivo experiments discussed in the review, detailing experimental methodologies such as methods used, incubation times or experimental periods, information about animal donors including their status and feeding regimens, specifics of treatments applied, insect species studied, and ethical approvals obtained. Besides, depending on the species, form, and inclusion level of insects, substituting soybean meal can have varying degrees of impact on ruminal fermentation indices and performance, as detailed in Table 2. Moreover, descriptive statistics of the variables in the database used to evaluate the effect of Gryllus bimaculatus, Hermetia illucens, and Bombyx mori on ruminal fermentation parameters in ruminants (in vivo) have been shown in Supplementary Table S9 (Supplementary material 2). Supplementary Table S10 (Supplementary material 2) displays the impact of various insects on ruminal fermentation parameters. The Gryllus bimaculatus treatment yielded a significant increase (p < 0.01) in ruminal pH, rising by 3.76% compared to the control. Vespa Orientalis treatments enhanced the apparent digestibility of DM by 7% (p = 0.003) compared to the control.
Table 2. Effect of various invertebrate insects used as either protein or fat (energy) source carrier on the ruminant species response.
Furthermore, the Bombyx mori treatment notably increased the apparent digestibility of acid detergent fiber (ADF) compared to the control treatment (p = 0.007; Supplementary Table S10 in Supplementary material 2). Supplementary Table S11 (Supplementary material 2) illustrates their influence on biochemical parameters in the context of in vivo experiments. None of the dietary insect interventions elicited discernible alterations in the blood biochemical profiles of ruminants compared to the control.
Table 3 demonstrates the economic feasibility of selected insects relative to plant-based protein sources. The current prices of soybean meal, rapeseed meal, and sunflower meal feeds are approximately €0.486, €0.3, and €0.237 per kg, respectively. Meanwhile, the current prices of Hermetia illucens and Tenebrio molitor are approximately €7.25 and €14.5 per kg, respectively. Therefore, for a comprehensive assessment between insects and traditional plant protein sources, it is essential to adjust the nutritional value based on parameters such as crude protein content or essential amino acids profile. This adjustment allows a more accurate comparison of their economic and nutritional merits. The findings indicate that replacing each euro of SBM with Hermetia illucens would cost 16.2 €/kg for protein, 11.9 €/kg for lysine (Lys), and 20 €/kg for methionine (Met) for farmers.
Similarly, replacing each euro of SBM with Tenebrio molitor would lead to costs of 35.2 €/kg for protein, 55.3 €/kg for Lys, and 36.1 €/kg for Met for farmers. The elevated cost of insect meal currently limits its application in ruminant diets. Nevertheless, to be competitive, expanding the scale of insect breeding operations within companies is expected to enhance efficiency and decrease the overall cost of insect protein production over time (45). Achieving mass production remains a distant prospect. While definitive conclusions on cost reduction or profit increase in insect production were not drawn, it has been proposed that greater mechanization could lead to reduced labor costs, and utilizing low-value feed substrates may decrease operational expenses. In terms of farm output sales, commercializing insect frass as fertilizer could offer an additional income stream for insect farmers (46). The potential of insects as a viable alternative feed component is attributed to their short life cycle.
Furthermore, projections from the International Platform of Insects for Food and Feed suggest a significant rise in the utilization of insects for food and feed within the European Union. The insect volume is expected to escalate from 500 tons in 2020 to surpass 1 million tons by 2025, reaching an estimated 3 million tons by 2030, encompassing both larvae and adult forms. This upward trajectory in market demand likely mirrors the lucrative opportunities available to stakeholders engaged in insect production. This growth is anticipated to contribute to heightened consumer awareness regarding the detrimental impacts of conventional animal feed production (45).
Insect meals are categorized as processed animal proteins and are subject to prohibitions on their utilization in numerous high-income nations (e.g., European countries). On the contrary, developing and emerging regions often lack specific legislation. For instance, in Asia, Thailand, a leading producer of crickets, is actively developing the first set of guidelines for insect breeding. In China, insects are widely used as feed and food components in various regions, yet they have not yet been officially recognized under food law (45). In the Americas, there is no specific prohibition or approval concerning the use of insect proteins in the processing, marketing, or incorporation into animal feed within this region. In the recent past, within the European Union, the approval for incorporating insects into farm animal feed was restricted to seven specific insect species, as outlined in Commission Regulation (EU) 2017/893 Commission Regulation-EU (47). These approved species encompassed two mealworm species (Tenebrio molitor, Alphitobius diaperinus), two fly species (Hermetia illucens, Musca domestica), and three cricket species (Acheta domesticus, Gryllodes sigillatus, Gryllus assimilis). Over time, there has been a growing expansion in the utilization of insect species for animal feed. Domestic silkworms, which exclusively consume mulberry leaves, pose no risk of contamination from animal-origin food sources that are not permitted for insect feed. Silkworms (Bombyx mori) have recently been added to the roster of authorized insect species for manufacturing processed animal protein utilized in animal feed, as delineated in Commission Regulation (EU) 2021/1925 (Commission Regulation-EU) (48).
Although legal regulations regarding the use of insects as feed vary regionally, researchers and feed manufacturers have a notable global interest in promoting innovation and research in this field. In the coming years, this interest may lead to legislative changes similar to those observed for monogastric animals, facilitating broader acceptance and utilization of insects in ruminant feeding practices worldwide. In summary, regarding the current global legislative framework concerning the use of insects as feed for ruminants, both insect oil and meal are explicitly authorized in countries including Mexico, Colombia, Brazil, Morocco, Algeria, Niger, Nigeria, Sudan, South Africa, Namibia, Ethiopia, India, Australia, and New Zealand. Insect oils are authorized but not insect meals in countries such as Russia, Finland, Sweden, Norway, Iceland, the United Kingdom, Denmark, Belarus, Estonia, Ireland, France, Spain, Italy, Romania, Ukraine, and Poland. Some countries like Egypt, Ecuador, Chile, Canada, and Alaska (United States) lack specific insect regulatory frameworks. However, countries such as Argentina, Iran, Japan, North Korea, and Tunisia do not authorize insect oils or meals to be used as feed for ruminants (20). Moreover, a structured compilation of legislative documents from the European Parliament and the Council (EC) concerning insect production for food and feed is presented in Supplementary Table S12 (Supplementary material 2), arranged chronologically.
Insects possess the potential to be incorporated into livestock production systems as a source of feed. However, insects must be cultivated on a large scale within a “mini-livestock” framework to be effective as feed. Because these large-scale rearing systems are relatively novel, formal industry standards and welfare regulations have not been fully established, resulting in unresolved questions related to insect welfare. Considering the significance of consumer attitudes in shaping the social acceptance of insect production, it is essential to analyze consumers’ ethical perspectives on using insects as livestock feed. As per Fukuda et al. (49), sampling involved convenience sampling of 361 adult consumers in the United States. When queried about using insects as livestock feed, 34% of respondents expressed support, 52% remained neutral, and 15% voiced opposition. Among those opposed, 58% cited ethical concerns as their rationale for opposition. Among respondents who expressed support or neutrality regarding using insects as livestock feed, 29% identified concerns related to livestock welfare, while 26% identified concerns related to insect welfare as perceived risks. These observations suggest that insect producers have an incentive to implement best practices that are perceived as fostering high-welfare conditions for their “mini-livestock” when used for livestock feed. Moreover, the findings indicate that, although the existing research on consumer acceptance is limited, it is unlikely to impede the development of the insect protein industry for feed. Nonetheless, additional research is needed to investigate consumer willingness to pay for animal products derived from animals fed with insects and assess whether insects contribute to improved acceptability, both in terms of general perception and sensory appeal, compared to conventional products (50).
The following issues warrant attention: (1) Nutrient requirements and digestibility—research gap: limited comprehensive studies on the specific nutrient requirements of ruminants when fed insect-based diets, especially the insects’ CP conventional factor for proximate analysis not unified yet. Because a portion of the nitrogen is contained within chitin, it is also extracted during protein analysis using the traditional Kjeldahl method, resulting in overestimating the actual CP content. Future direction: to standardize the conventional factor for CP content in potential insect feeds for ruminants across various species and morphological stages of the insects. (2) Feed formulation optimization—research gap: insufficient knowledge about optimal feed formulations incorporating insect meals for different classes of ruminants (e.g., lactating cows, growing calves). Future direction: explore novel feed formulation strategies that maximize the nutritional value of insect-based feeds while ensuring balanced diets for ruminant health and performance. Investigate the synergistic effects of combining insects with other feed ingredients. (3) Long-term effects on animal health and performance—research gap: limited understanding of the long-term impact of insect-based diets on ruminant health, productivity, and reproductive performance. Future direction: conduct longitudinal studies to assess the effects of sustained insect feeding on rumen health, metabolic function, immunity, and overall animal performance over extended periods. Investigate potential benefits or challenges associated with prolonged insect-based feeding. More studies are required to understand the impact of insect-based diets on ruminal fermentation dynamics, microbial populations, and metabolite production. Investigating potential health risks or safety concerns associated with feeding insects to ruminants is essential. Studies should focus on assessing antinutritional factors, toxins, or allergens in insect-based feeds. (4) Environmental impact and sustainability—research gap: incomplete evaluation of the environmental sustainability aspects of using insects as feed in dairy production systems. Future direction: quantify greenhouse gas emissions, resource utilization, and ecological footprints associated with insect farming and incorporation into ruminant diets. Explore integrated systems that leverage insect farming for waste management and circular economy principles. (5) Consumer acceptance and market dynamics—research gap: limited understanding of consumer perceptions and acceptance of dairy products derived from ruminants-fed insect-based diets. Future direction: investigate consumer attitudes toward insect-fed dairy products, addressing concerns related to food safety, quality, and ethical considerations. Develop strategies to enhance market acceptance and promote the adoption of insect-derived feed in dairy production systems. (6) Regulatory framework and policy development—research gap: inadequate regulatory guidelines and policy frameworks governing the use of insects in ruminant nutrition. Future direction: collaborate with regulatory bodies to establish evidence-based standards for insect-derived feed safety and quality assurance. Advocate for policy changes that support the sustainable integration of insects into ruminant diets. (7) Innovative approaches and technology—research gap: limited exploration of innovative technologies and processing methods for optimizing insect-derived feed production and utilization in dairy systems. Future direction: explore novel approaches such as precision feeding, genetic selection for enhanced utilization of insect proteins, and advanced processing techniques to improve the efficiency and efficacy of insect-based ruminant nutrition. Research should explore different insect species and their processing methods to optimize nutrient bioavailability and ensure feed safety. Comparative studies between fresh, dried, and processed insects can provide valuable insights. Addressing these research gaps and advancing future directions will facilitate the broader adoption of insect-derived feed in ruminant nutrition, promoting sustainability, efficiency, and resilience in dairy production systems; especially for neonatal calves, particularly those with underdeveloped rumens, the abomasum assumes paramount importance. The abomasum comprises 60–70% of the calf’s stomach capacity and secretes gastric juices rich in hydrochloric acid and digestive enzymes. These enzymes facilitate the breakdown of proteins, fats, and carbohydrates in the ingested feed, whether insects or other nutrients, into simpler forms readily absorbed by the calf’s body.
Recent data confirm the feasibility of integrating insects into ruminant diets, showing predominantly positive effects on growth performance, ruminal fermentation indices, and methane mitigation. However, the absence of global uniformity in insect products highlights the need for attention and standardization. To optimize the efficiency of insect and ruminant production, comprehensive assessments of economically viable insect species should be prioritized in future studies. Moreover, (1) environmental sustainability: using insects as feed aligns with sustainability goals by reducing reliance on conventional protein sources like soybean meal, which are resource-intensive and contribute to environmental degradation. Insects have a lower ecological footprint and can be produced using organic waste streams. (2) Improved feed efficiency: Insect-derived feeds offer opportunities to optimize feed efficiency in ruminants, potentially enhancing animal performance and productivity; nevertheless, the expenses associated with feed must be tackled. (3) Consumer acceptance and market trends: despite initial consumer reservations, there is growing interest in insect-fed dairy products due to their sustainability credentials and nutritional benefits. Dairy producers can leverage this trend to diversify product offerings and capture niche markets. (4) Research and development: continued research is needed to address knowledge gaps related to nutrient requirements, feed formulation, long-term health effects, and market dynamics surrounding insect-based ruminant nutrition. (5) Policy and regulatory considerations: policymakers and industry stakeholders should collaborate to establish clear guidelines and regulations governing the use of insects in ruminant diets, ensuring food safety and quality standards are met. Adopting insect-based feed strategies holds significant promise for enhancing ruminant nutrition and advancing environmental sustainability in dairy production. Dairy producers can benefit from diversifying feed sources, reducing reliance on traditional protein sources, and improving overall feed efficiency. Researchers should prioritize studies to optimize insect-derived feed formulations and assess their long-term impacts on ruminant health and performance. Policymakers and industry stakeholders play a crucial role in facilitating the adoption of insect-based feed by establishing supportive regulatory frameworks and promoting consumer acceptance. By embracing insect-based nutrition, the dairy industry can contribute to a more sustainable and resilient agricultural future.
The original contributions presented in the study are included in the article/Supplementary material, further inquiries can be directed to the corresponding authors.
MG: Conceptualization, Methodology, Project administration, Resources, Software, Visualization, Writing – original draft. ME: Data curation, Supervision, Writing – review & editing. MS-S: Data curation, Writing – review & editing. AC: Data curation, Writing – review & editing. YY: Methodology, Writing – review & editing. AI: Data curation, Methodology, Validation, Writing – review & editing. BX: Data curation, Validation, Writing – review & editing. Z-jC: Writing – review & editing. IF: Writing – review & editing. HJ: Writing – review & editing. AA: Data curation, Writing – review & editing. Y-bL: Data curation, Funding acquisition, Supervision, Writing – review & editing.
The author(s) declare that financial support was received for the research, authorship, and/or publication of this article. This research was funded by the National Ministry-Region Joint Project, China (grant no. 21132), specifically under subproject (grant no. 10000-23112101/177). The publication was also co-financed with the Inner Mongolia Autonomous Region Science and Technology Plan (grant no. 2023YFHH0114), the Inner Mongolia Autonomous Region Open Competition Projects (grant no. 2022JBGS0024), Agriculture Research System of China (Cars38), Inner Mongolia Autonomous Region Department of Education first-class scientific research project (grant no. YLXKZX-ND-026, YLXKZX-ND-029), and Bayannur Science and Technology Key Breakthrough Project (grant no. NMKJXM202404-1).
We greatly appreciate the support of the Chinese Academy of Sciences President’s International Fellowship Initiative (PIFI, Project No. 2025PVA0045).
The authors declare that the research was conducted in the absence of any commercial or financial relationships that could be construed as a potential conflict of interest.
All claims expressed in this article are solely those of the authors and do not necessarily represent those of their affiliated organizations, or those of the publisher, the editors and the reviewers. Any product that may be evaluated in this article, or claim that may be made by its manufacturer, is not guaranteed or endorsed by the publisher.
The Supplementary material for this article can be found online at: https://www.frontiersin.org/articles/10.3389/fvets.2024.1484870/full#supplementary-material
SUPPLEMENTARY MATERIAL 1 | Data processing and meta-analysis.
SUPPLEMENTARY MATERIAL 2 | Datasheet.
SUPPLEMENTARY MATERIAL 3 | References.
ADF, Acid detergent fibre; ADFD, Acid detergent fibre digestibility; ALT, Alanine transaminase; ALP, Alkaline phosphatase; AST, Aspartate transaminase; BUN, Blood urea nitrogen; BW, Body weight; CH4, Methane; CO2, Carbon dioxide; CP, Crude protein; C2, Acetate; C3, Propionate; C4, Butyrate; DDM, Digestible dry matter; DM, Dry matter; DMD, Dry matter digestibility; EAA, Essential amino acid; HCT, Hematocrit test; HGB, Hemoglobin; IVDMD, In vitro dry matter digestibility; IVOMD, In vitro organic matter digestibility; IVNDFD, In vitro neutral detergent fibre digestibility; IVADFD, In vitro acid detergent fibre digestibility; Max, Maximum; Min, Minimum; NA, Not applied; NDF, Neutral detergent fibre; NDFD, Neutral detergent fibre digestibility; ND, Not detected; NEAA, Non-essential amino acid; NH3, Ammonia; OM, Organic matter; OMD, Organic matter digestibility; RBC, Red blood cell; RMSE, Root mean square error; SD, Standard deviation; TGP, Total gas production; TVFA, Total volatile fatty acids; WBC, White blood cells.
1. Ahmed, E, and Nishida, T. Optimal inclusion levels of cricket and silkworm as alternative ruminant feed: A study on their impacts on rumen fermentation and gas production. Sustain For. (2023) 15:1415. doi: 10.3390/su15021415
2. Astuti, DA, and Wiryawan, KG. Black soldier fly as feed ingredient for ruminants. Anim Biosci. (2022) 35:356–63. doi: 10.5713/ab.21.0460
3. Jayanegara, A, Yantina, N, Novandri, B, Laconi, EB, and Nahrowi, RM. Evaluation of some insects as potential feed ingredients for ruminants: chemical composition, in vitro rumen fermentation and methane emissions. J Indones Trop Anim Agric. (2017) 42:247. doi: 10.14710/jitaa.42.4.247-254
4. Phesatcha, B, Phesatcha, K, Matra, M, and Wanapat, M. Cricket (Gryllus bimaculatus) meal pellets as a protein supplement to improve feed efficiency, ruminal fermentation and microbial protein synthesis in Thai native beef cattle. Anim Biosci. (2023) 36:1384–92. doi: 10.5713/ab.23.0107
5. Renna, M, Coppa, M, Lussiana, C, Le Morvan, A, Gasco, L, and Maxin, G. Full-fat insect meals in ruminant nutrition: in vitro rumen fermentation characteristics and lipid biohydrogenation. J Anim Sci Biotechnol. (2022) 13:138. doi: 10.1186/s40104-022-00792-2
6. Hawkey, KJ, Lopez-Viso, C, Brameld, JM, Parr, T, and Salter, AM. Insects: a potential source of protein and other nutrients for feed and food. Annu Rev Anim Biosci. (2021) 9:333–54. doi: 10.1146/annurev-animal-021419-083930
7. Kierończyk, B, Sypniewski, J, Rawski, M, Czekała, W, Świątkiewicz, S, and Józefiak, D. From waste to sustainable feed material: the effect of oil on the growth performance, nutrient digestibility, and gastrointestinal tract morphometry of broiler chickens. Ann Anim Sci. (2020) 20:157–77. doi: 10.2478/aoas-2019-0066
8. Heuel, M, Sandrock, C, Leiber, F, Mathys, A, Gold, M, Zurbrügg, C, et al. Black soldier fly larvae meal and fat can completely replace soybean cake and oil in diets for laying hens. Poult Sci. (2021) 100:101034. doi: 10.1016/j.psj.2021.101034
9. Sypniewski, J, Kierończyk, B, Benzertiha, A, Mikołajczak, Z, Pruszyńska-Oszmałek, E, Kołodziejski, P, et al. Replacement of soybean oil by Hermetia illucens fat in Turkey nutrition: effect on performance, digestibility, microbial community, immune and physiological status and final product quality. Br Poult Sci. (2020) 61:294–302. doi: 10.1080/00071668.2020.1716302
10. Gariglio, M, Dabbou, S, Biasato, I, Capucchio, MT, Colombino, E, Hernández, F, et al. Nutritional effects of the dietary inclusion of partially defatted Hermetia illucens larva meal in Muscovy duck. J Anim Sci Biotechnol. (2019) 10:37. doi: 10.1186/s40104-019-0344-7
11. Cullere, M, Woods, MJ, van Emmenes, L, Pieterse, E, Hoffman, LC, and Dalle, ZA. Hermetia illucens larvae reared on different substrates in broiler quail diets: effect on physicochemical and sensory quality of the quail meat. Animals. (2019) 9:525. doi: 10.3390/ani9080525
12. Gasco, L, Dabbou, S, Trocino, A, Xiccato, G, Capucchio, MT, Biasato, I, et al. Effect of dietary supplementation with insect fats on growth performance, digestive efficiency and health of rabbits. J Anim Sci Biotechnol. (2019) 10:4. doi: 10.1186/s40104-018-0309-2
13. Altmann, BA, Neumann, C, Rothstein, S, Liebert, F, and Mörlein, D. Do dietary soy alternatives lead to pork quality improvements or drawbacks? A look into micro-alga and insect protein in swine diets. Meat Sci. (2019) 153:26–34. doi: 10.1016/j.meatsci.2019.03.001
14. Freel, TA, McComb, A, and Koutsos, EA. Digestibility and safety of dry black soldier fly larvae meal and black soldier fly larvae oil in dogs. J Anim Sci. (2021) 99:skab047. doi: 10.1093/jas/skab047
15. Weththasinghe, P, Hansen, JØ, Nøkland, D, Lagos, L, Rawski, M, and Øverland, M. Full-fat black soldier fly larvae (Hermetia illucens) meal and paste in extruded diets for Atlantic salmon (Salmo salar): effect on physical pellet quality, nutrient digestibility, nutrient utilization and growth performances. Aquaculture. (2021) 530:735785. doi: 10.1016/j.aquaculture.2020.735785
16. Prakoso, VA, Irawan, A, Iswantari, A, Maulana, F, Samsudin, R, and Jayanegara, A. Evaluation of dietary inclusion of black soldier fly (Hermetia illucens) larvae on fish production performance: a meta-analysis. J Insects Food Feed. (2022) 8:1373–84. doi: 10.3920/JIFF2021.0159
17. Jayanegara, A, Gustanti, R, Ridwan, R, and Widyastuti, Y. Fatty acid profiles of some insect oils and their effects on in vitro bovine rumen fermentation and methanogenesis. Ital J Anim Sci. (2020) 19:1310–7. doi: 10.1080/1828051X.2020.1841571
18. Jayanegara, A, Novandri, B, Yantina, N, and Ridla, M. Use of black soldier fly larvae (Hermetia illucens) to substitute soybean meal in ruminant diet: an in vitro rumen fermentation study. Vet World. (2017) 10:1436–46. doi: 10.14202/vetworld.2017.1439-1446
19. Ahmed, E, Fukuma, N, Hanada, M, and Nishida, T. Insects as novel ruminant feed and a potential mitigation strategy for methane emissions. Animals. (2021) 11:2648. doi: 10.3390/ani11092648
20. Renna, M, Rastello, L, Veldkamp, T, Toral, PG, Gonzalez-Ronquillo, M, Jimenez, LER, et al. Are insects a solution for feeding ruminants? Legislation, scientific evidence, and future challenges. Anim Front. (2023) 13:102–11. doi: 10.1093/af/vfad026
21. Renna, M, Rastello, L, and Gasco, L. Can insects be used in the nutrition of ruminants? J. Insects Food Feed. (2022) 8:1041–1045.
22. Kierończyk, B, Rawski, M, Mikołajczak, Z, Homska, N, Jankowski, J, Ognik, K, et al. Available for millions of years but discovered through the last decade: insects as a source of nutrients and energy in animal diets. Anim Nutr. (2022) 11:60–79. doi: 10.1016/j.aninu.2022.06.015
23. Phesatcha, B, Phesatcha, K, Viennaxay, B, Matra, M, Totakul, P, and Wanapat, M. Cricket meal (Gryllus bimaculatus) as a protein supplement on in vitro fermentation characteristics and methane mitigation. Insects. (2022) 13:129. doi: 10.3390/insects13020129
24. Kahraman, O, Gülşen, N, İnal, F, Alataş, MS, İnanç, ZS, Ahmed, İ, et al. Comparative analysis of in vitro fermentation parameters in Total mixed rations of dairy cows with varied levels of defatted black soldier Fly larvae (Hermetia illucens) as a substitute for soybean meal. Fermentation. (2023) 9:652. doi: 10.3390/fermentation9070652
25. Gasco, L, Biancarosa, I, and Liland, NS. From waste to feed: A review of recent knowledge on insects as producers of protein and fat for animal feeds. Curr Opin Green Sustain Chem. (2020) 23:67–79. doi: 10.1016/j.cogsc.2020.03.003
26. DiGiacomo, K, and Leury, BJ. Review: insect meal: a future source of protein feed for pigs? Animal. (2019) 13:3022–30. doi: 10.1017/S1751731119001873
27. Jayanegara, A, Sholikin, MM, Sabila, DAN, Suharti, S, and Astuti, DA. Lowering chitin content of cricket (Gryllus assimilis) through exoskeleton removal and chemical extraction and its utilization as a ruminant feed in vitro. Pak J Biol Sci. (2017) 20:523–9. doi: 10.3923/pjbs.2017.523.529
28. Ningsih, RHC, Ramadani, AD, Raynissa, DJ, Diapari, D, Fassah, DM, Astuti, DA, et al. Effects of black soldier Fly oil and calcium soap supplementation on rumen fermentability of Garut sheep. IOP Conf Ser Earth Environ Sci. (2023) 1208:012059. doi: 10.1088/1755-1315/1208/1/012059
29. Hanönü, M, Özdemir, M, and Beyzi, SB. Effects of mealworm (Tenebrio Molitor L.) larvae supplementation to Alfalfa hay at different levels on in vitro gas and methane production. ethabd (2024) 7:8–11. doi: 10.55257/ethabd.1393914
30. Park, HH (2016). Black soldier Fly larvae manual. Available online at: https://hdl.handle.net/20.500.14394/44241 (Accessed August 19, 2024).
31. Khonkhaeng, B, Wanapat, M, Wongtangtintharn, S, Phesatcha, K, Supapong, C, Suntara, C, et al. Tropical plant phytonutrient improves the use of insect protein for ruminant feed. Agriculture. (2022) 12:1628. doi: 10.3390/agriculture12101628
32. Samadi,, Wajizah, S, Pratama, SM, and Jayanegara, A. Evaluation of nutritive values of various non-conventional protein sources as potential feed ingredients for ruminants. Biodivers J Biol Divers. (2023) 24:4069. doi: 10.13057/biodiv/d240745
33. Robles-Jimenez, LE, Cardoso-Gutierrez, BSE, Vargas-Bello-Perez, E, Castelan-Ortega, OA, and Gonzalez-Ronquillo, M. 112. Insects as sustainable feed alternative for ruminants: chemical composition and in vitro gas production. Anim Sci Proc. (2022) 13:83–4. doi: 10.1016/j.anscip.2022.03.113
34. Mulianda, R, Harahap, R, Laconi, E, Ridla, M, and Jayanegara, A. Nutritional evaluation of Total mixed ration silages containing maggot (Hermetia illucens) as ruminant feeds. J Anim Health Product. (2020) 8:138–44. doi: 10.17582/journal.jahp/2020/8.3.138.144
35. Thirumalaisamy, G, Malik, PK, Kolte, AP, and Bhatta, R. In vitro evaluation of graded level of silkworm pupae (Bombyx mori) oil on methane production, fermentation characteristics, and protozoal populations. Vet World. (2020) 13:586–92. doi: 10.14202/vetworld.2020.586-592
36. Astuti, DA, Anggraeny, A, Khotijah, L, Suharti, S, and Jayanegara, A. Performance, physiological status, and rumen fermentation profiles of pre-and post-weaning goat kids fed cricket meal as a protein source. Trop Anim Sci J. (2019) 42:145–51. doi: 10.5398/tasj.2019.42.2.145
37. Astuti, DA, Khotijah, L, and Rismarianty, A. Utilization and evaluation of cricket meal as protein source in lamb ration. Ind J Vet Sci Biotechnol. (2016) 11:53–7.
38. Rashmi, KM, Chandrasekharaiah, M, Soren, NM, Prasad, KS, David, CG, Thirupathaiah, Y, et al. Defatted silkworm pupae meal as an alternative protein source for cattle. Trop Anim Health Prod. (2022) 54:327. doi: 10.1007/s11250-022-03323-3
39. Thirumalaisamy, G, Malik, PK, Kolte, AP, Trivedi, S, Dhali, A, and Bhatta, R. Effect of silkworm (Bombyx mori) pupae oil supplementation on enteric methane emission and methanogens diversity in sheep. Anim Biotechnol. (2022) 33:128–40. doi: 10.1080/10495398.2020.1781147
40. Dai, X, Kalscheur, KF, Huhtanen, P, and Faciola, AP. Effects of ruminal protozoa on methane emissions in ruminants—A meta-analysis. J Dairy Sci. (2022) 105:7482–91. doi: 10.3168/jds.2021-21139
41. AbdelHakeam, MA, Mohamed, MY, AbdEl-Salam, OM, and Ali, SM. Effect of insect meal as substitute for soybean meal on performance of Ossimi lambs. J Livest Sci. (2024) 15:27–41. doi: 10.33259/JLivestSci.2024.27-41
42. Nekrasov, RV, Ivanov, GA, Chabaev, MG, Zelenchenkova, AA, Bogolyubova, NV, Nikanova, DA, et al. Effect of black soldier Fly (Hermetia illucens L.) fat on health and productivity performance of dairy cows. Animals. (2022) 12:2118. doi: 10.3390/ani12162118
43. Rahman, R, Laconi, EB, Jayanegara, A, and Astuti, DA. Effect of dietary black soldier fly larvae (Hermetia illucens) and bioconversion product of cocoa pod husk on performance and hematological profile of sheep. IOP Conf Ser Mater Sci Eng. (2021) 1098:062058. doi: 10.1088/1757-899X/1098/6/062058
44. Aydin, M, and Kisadere, İ. Some hematological parameters and enzyme histochemistry of peripheral bloodleukocytes in Kivircik sheep. Turk J Vet Anim Sci. (2022) 46:275–84. doi: 10.55730/1300-0128.4175
45. Shah, AA, Totakul, P, Matra, M, Cherdthong, A, Hanboonsong, Y, and Wanapat, M. Nutritional composition of various insects and potential uses as alternative protein sources in animal diets. Anim Biosci. (2022) 35:317–31. doi: 10.5713/ab.21.0447
46. Niyonsaba, HH, Höhler, J, Kooistra, J, Fels-Klerx, HD, and Meuwissen, MPM. Profitability of insect farms. J Insects Food Feed. (2021) 7:923–34. doi: 10.3920/JIFF2020.0087
47. European Commission. Commission regulation (EU) 2017/893 of 24 may 2017 amending annexes I and IV to regulation (EC) no 999/2001 of the European Parliament and of the council and annexes X, XIV and XV to commission regulation (EU) no 142/2011 as regards the provisions on processed animal protein. Off J Eur Union. (2017) 138:92–116.
48. European Commission. Commission Regulation (EU) 2021/1925 of 5 November 2021 amending certain Annexes to Regulation (EU) No 142/2011 as regards the requirements for placing on the market of certain insect products and the adaptation of a containment method. Off J Eur Union. (2021) 393:4–8.
49. Fukuda, EP, Carrasco, MN, Perez, AJ, Fischer, B, and Drewery, ML. US consumer perceptions of insects as livestock feed: Ethical considerations for insects. J Insects Food Feed. (2023) 10:125–39. doi: 10.1163/23524588-20230028
50. Sogari, G, Amato, M, Biasato, I, Chiesa, S, and Gasco, L. The potential role of insects as feed: A multi-perspective review. Animals. (2019) 9:119. doi: 10.3390/ani9040119
51. Lagos, LV, and Stein, HH. Chemical composition and amino acid digestibility of soybean meal produced in the United States, China, Argentina, Brazil, or India1. J Anim Sci. (2017) 95:1626–36. doi: 10.2527/jas.2017.1440
52. Cheng, H, Liu, X, Xiao, Q, Zhang, F, Liu, N, Tang, L, et al. Rapeseed meal and its application in pig diet: A review. Agriculture. (2022) 12:849. doi: 10.3390/agriculture12060849
53. Liu, JD, Li, QY, Zeng, ZK, Li, P, Xu, X, Wang, HL, et al. Determination and prediction of the amino acid digestibility of sunflower seed meals in growing pigs. Asian Australas J Anim Sci. (2015) 28:86–94. doi: 10.5713/ajas.14.0109
54. CLAL (2024). Il mercato del latte. Available online at: https://www.clal.it/index.php?section=home&redirectfrom=oilseeds-price-eu (Accessed August 20, 2024).
Keywords: insects as feed, in vitro digestibility, in vivo , methane, total gas production
Citation: Gao M, El-Sherbiny M, Szumacher-Strabel M, Cieślak A, Yanza YR, Irawan A, Xie B, Cao Z-j, Fusaro I, Jalal H, Abd El Tawab AM and Liu Y-b (2024) Cutting-edge exploration of insect utilization in ruminant nutrition—feature and future: a systematic review and meta-analysis. Front. Vet. Sci. 11:1484870. doi: 10.3389/fvets.2024.1484870
Received: 22 August 2024; Accepted: 05 November 2024;
Published: 20 November 2024.
Edited by:
Sadarman Sadarman, State Islamic University of Sultan Syarif Kasim Riau, IndonesiaReviewed by:
Hani M. El-Zaiat, Sultan Qaboos University, OmanCopyright © 2024 Gao, El-Sherbiny, Szumacher-Strabel, Cieślak, Yanza, Irawan, Xie, Cao, Fusaro, Jalal, Abd El Tawab and Liu. This is an open-access article distributed under the terms of the Creative Commons Attribution License (CC BY). The use, distribution or reproduction in other forums is permitted, provided the original author(s) and the copyright owner(s) are credited and that the original publication in this journal is cited, in accordance with accepted academic practice. No use, distribution or reproduction is permitted which does not comply with these terms.
*Correspondence: Mohamed El-Sherbiny, ZWxzaGVyYmlueS5ucmMuZWdAZ21haWwuY29t; Yong-bin Liu, eWJsaXVAaW11LmVkdS5jbg==
Disclaimer: All claims expressed in this article are solely those of the authors and do not necessarily represent those of their affiliated organizations, or those of the publisher, the editors and the reviewers. Any product that may be evaluated in this article or claim that may be made by its manufacturer is not guaranteed or endorsed by the publisher.
Research integrity at Frontiers
Learn more about the work of our research integrity team to safeguard the quality of each article we publish.