- 1Animal Production Department, College of Food and Agriculture Sciences, King Saud University, Riyadh, Saudi Arabia
- 2Animal Science College, Zhejiang University, Zijingang Campus, Hangzhou, China
- 3Hankkija Oy, Hyvinkää, Finland
- 4Department of Animal Wealth Development, Faculty of Veterinary Medicine, Mansoura University, Mansoura, Egypt
The objective of this study was to enhance the knowledge about the effects of hydrolyzed yeast supplementation on growth performance, woody breast myopathy, and its mechanism on intestinal homeostasis using antioxidant and immunomodulatory-related gene expressions in heat-stressed broiler chickens. In a 35-d feeding experiment, 160-day-old male Ross 308 broiler chickens were assigned to four dietary groups, consisting of eight replicates and five birds per replicate. Experimental diets contained four levels of hydrolyzed yeast (HY) (0, 400, 800, or 1,200 mg.kg−1) derived from Saccharomyces cerevisiae. On d 25, birds were exposed to cyclic heat stress (HS) (35°C for 8 h/d from 8 a.m. to 4.00 p.m.) for 10 days. Adding HY at 800 mg.kg−1 numerically decreased the feed conversion ratio (FCR) on days 25–35 (heat stress period) by 2.50%. Furthermore, the addition of HY reduced (P = 0.005) mortality rate compared with those of birds fed the control diet. Supplementation of HY exhibited efficacy (P = 0.09) in diminishing woody breast (WB) in terms of incidence and degree of severity. Furthermore, the added HY decreased (P < 0.001) drip loss values of the Pectoralis major compared with the control diet group; the addition of HY at 400 and 1,200 mg.kg−1 decreased (P < 0.001) cooking loss values in the Pectoralis major. In addition, HY supplementation at 800 mg.kg−1 decreased (P = 0.04) the duodenal mRNA expression of the avian β-defensin 10 (AvBD10) and increased (P < 0.05) the mRNA expression of nuclear factor erythroid 2–related factor 2 (Nrf2), nuclear factor kappa-light-chain-enhancer of activated B cells (NF-κB), and secreted immunoglobulin A (sIgA). The addition of HY at 400 and 800 mg.kg−1 decreased (P = 0.001) the duodenal mRNA expression of copper and zinc superoxide dismutase (Cu-ZnSOD1). HY supplementation tended to decrease (P =0.07) the duodenal mRNA expression of heat shock protein 70 (HSP70). The results suggest that hydrolyzed yeast supplementation to broiler chickens exposed to heat stress might improve intestinal redox homeostasis and decrease the mortality rate. The inclusion of 800 mg.kg−1 HY in the diet enhanced duodenal redox homeostasis, while 400–1,200 mg.kg−1 HY reduced mortality rate and exhibited lower drip loss values and reduced woody breast of Pectoralis major in terms of incidence and degree of severity.
1 Introduction
Postbiotics are a promising alternative to antimicrobial growth promoters (AGPs) owing to increasing concerns regarding the spread of antimicrobial resistance, and they have physiological benefits to the host either directly or indirectly (1–5). The term postbiotics has been employed to define inanimate microorganisms or soluble factors (metabolic products or byproducts) secreted by live microorganisms or released after their lysis (4). Hydrolyzed yeast, which originates from Saccharomyces cerevisiae, has attracted much attention as a feed supplement that consists of the total content of the yeast residue from the lysis process. Thus, it contains nucleotides, ß-glucan, B vitamins, mannan oligosaccharides, and amino acids (6, 7). Hydrolyzed yeast has a cost-effective advantage over other yeast byproducts and its feature to support beneficial interactions within the digestive and immune systems of animal due to its low molecular weight contributing to higher solubility in aqueous media makes it a promising feed additive (7, 8). Recently, it has been found that hydrolyzed yeast supplementation improved performance, meat quality, and antioxidant status and decreased Escherichia coli in broiler chickens (8–11).
There is a growing interest in expanding studies to mitigate the adverse effects of thermal stress on fast-growing broiler chickens as the global annual environmental temperature continues to rise. Heat stress affects meat quality, particularly through increased oxidation of proteins that reduced protein accumulation and increased fat accumulation in breast meat (12, 13), which makes proteins in the breast filets susceptible to oxidative stress and lowers their ability to bind water, resulting in increased cooking losses (10). Heat stress negatively affects cooking loss (CL) and drip loss (DL) in meat of broilers (12–14). In addition, the relationships between woody breast muscle myopathy and intestinal homeostasis have been reported (15–17). Recently, it has been found that heat stress independent of feed depression can induce significant differences in the duodenal metabolome of broiler chickens (18). It is well-acknowledged that heat stress reduces the immunological robustness, in terms of decreased secretory IgA (sIgA) and increased loads of intestinal Escherichia coli, Salmonella, Clostridium perfringens, and coliforms, and decreased numbers of Lactobacillus and Bifidobacterium; and such imbalance leads to the higher mortality rate (12, 19). Furthermore, heat stress affects the avian beta-defensins (AvBDs) (20) and induces oxidative stress resulting in cellular damage and inflammatory reactions, ultimately compromising growth performance and increasing energy expenditure due to the need of bird for oxidative stress mitigation and thermoregulation (20–24).
Our hypothesis was that the supplementation of dietary hydrolyzed yeast could promote intestinal health in heat-stressed broiler chickens. To our knowledge, no other reports exist regarding the impacts of dietary hydrolyzed yeast on growth performance, intestinal redox homeostasis, and woody breast myopathy in heat-stressed broiler chickens. The objective of the present study was to enhance the knowledge about the effects of hydrolyzed yeast supplementation on woody breast myopathy and its mechanism on intestinal homeostasis using antioxidant and immunomodulatory-related gene expressions in heat-stressed broiler chickens.
2 Materials and methods
2.1 Birds, housing, and experimental diets
In total, 160 1–day–old male Ross 308 broiler chickens were obtained from a commercial hatchery after they were vaccinated for infectious bronchitis and infectious bursal disease and were housed in an environmentally controlled room at the research unit of the Animal Production Department, King Saud University, Riyadh, Saudi Arabia, in wire battery cages, and each cage (58 × 50 × 35) was furnished with a radiant heater, a linear feeder, and a nipple drinker. Mash diets and water were administered ad libitum for the duration of the study (35 d). Mortality was recorded daily, and the percentage mortality rate was calculated for the starter phase (0–10 d), grower phase (11–24 d), and finisher phase (25–35 d). Broilers were assigned to four dietary groups in a randomized complete block design, consisting of eight replicates (cages) and five birds per replicate. Experimental diets contained four levels of yeast hydrolysate supplementation (0, 400, 800, or 1,200 mg.kg−1) derived from Saccharomyces cerevisiae (PROGUT® EXTRA; Hankkija Oy, Hyvinkää, Finland). Progut® extra was synthesized from spent brewery yeast through a rigorous process of strong acid hydrolysis, resulting in a final produced product that exhibits 70% solubility in water. No fraction of the hydrolysate is extricated after the hydrolysis, thereby ensuring that the product encompasses all bioactive components present within the yeast cells, including manno-oligosaccharides, β-glucan, peptides, and chitin structures. There were three-feeding phases: starter (0–10 d), grower (11–24 d), and finisher (25–35 d), and all diets were corn–soybean based (Table 1), and diets were analyzed using AOAC methods for proximate analysis (25) and HPLC for amino acids according to (26, 27). All diets were free of antimicrobial growth promotion.
2.2 Environmental data and heat stress
The ambient temperature (AM) was decreased gradually from 33°C for d 1 to 4, 30.5°C for d 5 to 7, 28.5°C for d 8 to 10, 27°C for d 11 to 15, and 24°C for d 16 to 24. A relative humidity (RH) of 33–42% was recorded, and a temperature–humidity index (THI) was calculated. On d 25, birds were exposed to chronic cyclic heat stress (35°C for 8 h/d from 8 a.m. to 4.00 p.m.) to mimic hot arid environments in broiler houses in Saudi Arabia as our laboratory previously suggested (28). The environmental temperature and humidity were recorded using EasyLog USB data loggers (Lascar Electronics, Whiteparish, Wiltshire, UK).
2.3 Sample collection and measurements
Body weights and feed intake were measured on d 0, 10, 24, and 35 on a cage basis. Feed conversion ratio (FCR) was calculated considering the weight of dead birds as feed intake divided by the body weight gain (g/g). The rectal temperature (°C) and respiratory rate (breaths/min) were measured on d 24 (before heat stress) and d 35 (after 10 d of heat exposure). After 10 d of heat exposure (d 35), birds close to the average weight were slaughtered as proposed in (28), and then, the carcasses were kept at 4°C in a refrigerator for 24 h. The weights of hot and cold carcasses and meat main portions (breast, legs, and wings) were measured to determine the absolute weights and relative weights as a percentage of body weights. The left breast muscles (Pectoralis major) were used for measuring meat quality (pH, drip-losing rate, and cooking loss). Muscle pH at 15 min and 24 h post-mortem was measured using a probe pH meter (Model: HI 8242C, Hanna Instruments Science and Technology, Beijing, China), and each breast was measured in duplicate at different locations and the values were averaged. The probe was calibrated before measuring using buffer solutions (4.01, 7.00, and 9.21) at room temperature. The initial and ultimate surface color of breast meat was determined using a colorimeter (CR-400 Chroma Meter, Konica Minolta, Tokyo, Japan). CIELAB: L* (lightness), a* (redness), and b* (yellowness) values were measured. After deboning, the entire breast muscles (Pectoralis major) were collected and the degree of hardness for woody breast (WB) was graded and scaled by hand palpation and scored using normal (0), mild (1), moderate (2), and severe (3) as proposed in Tijare et al. (29). After evaluation, filet samples were packed in bags (PA/PE, 90 μm) and stored at −20°C for further analysis. The water-holding capacity of meat has been measured by determining drip loss and cooking loss of the raw meat using two replicates of each sample. To measure cooking loss, the meat samples were defrosted at 4°C for 24 h and filets were cooked at 200 °C until the internal temperature of the meats reached 70 °C in an oven-searing (TRO45RDG-B5, Black and Decker Manufacturing Company, China). After cooking, samples were taken out, cooled at room temperature, wiped gently with paper towels, and weighed again. The values expressed as a percentage of the starting weight of sample before cooking as described by (30). To determine drip loss, the meat samples (~20 g) were weighed and immediately placed in a transparent polythene bag, hung from a hook, and stored at 4°C for 1 d. After storage, the sample was wiped gently with paper towels and weighed again. The values are expressed as a percentage as the percentage of the initial muscle weight ([(W1 – W2)/W1] × 100) (31).
2.4 Duodenal mRNA expression assay
A part of the middle section of duodenum tissues (~50 mg) were collected from one bird per replicate and rinsed with cold phosphate-buffered saline, were snap-frozen with liquid nitrogen, and were stored at −80°C. Total RNA was isolated from duodenal tissues using a reagent (the PureLink RNA Mini Kit) according to the manufacturer's instructions (Invitrogen, Carlsbad, CA). The procedures described by (32) were used to examine duodenal mRNA expression. The endogenous reference gene glyceraldehyde-3-phosphate dehydrogenase (GAPDH) was used, and primers based on chicken sequences were synthesized at Macrogen (Seoul, South Korea) as presented in Table 2. The PCRs were performed in duplicate, and the findings were normalized to GAPDH mRNA expression. Average gene expression relative to the endogenous control for each sample was calculated using the 2−ΔΔCt method (33).
2.5 Statistical analysis
The data were analyzed with a one-way ANOVA, Tukey's range test was adopted to compare means, and the woody breast data were subjected to the Kruskal–Wallis non-parametric test. Data were expressed as the mean ± SE, and it was expressed as significant when the p-value was < 0.05 (p < 0.05). Student's t-test was used to examine the differences between d 24 (before heat stress) and d 35 (the 10th day of heat exposure).
3 Results
3.1 Environmental data
The ambient temperature (AM), air relative humidity (RH), and the temperature–humidity index (THI) under a high-temperature environment are presented in Supplementary Table 1. The minimum AM, RH, and THI values were, on average, 24.50 ± 0.36, 33.50 ± 2.05%, and 71.02 ± 0.70, respectively, whereas the maximum values of AM, RH, and THI were, on average, 32.89 ± 1.43°C, 42.86 ± 0.81%, and 80.51 ± 1.66, respectively.
3.2 Stress indicators
The data on rectal temperature and respiratory rate are presented in Table 3. Indeed, on d 35 of age (10th day of heat exposure) birds showed an increase in rectal temperature (41.04 vs. 42.45°C; p < 0.001) coupled with a higher respiratory rate of 59.06 vs. 180 B/M; p < 0.001), while the addition of HY had no significant effect on rectal temperature or respiratory rate (p > 0.05).
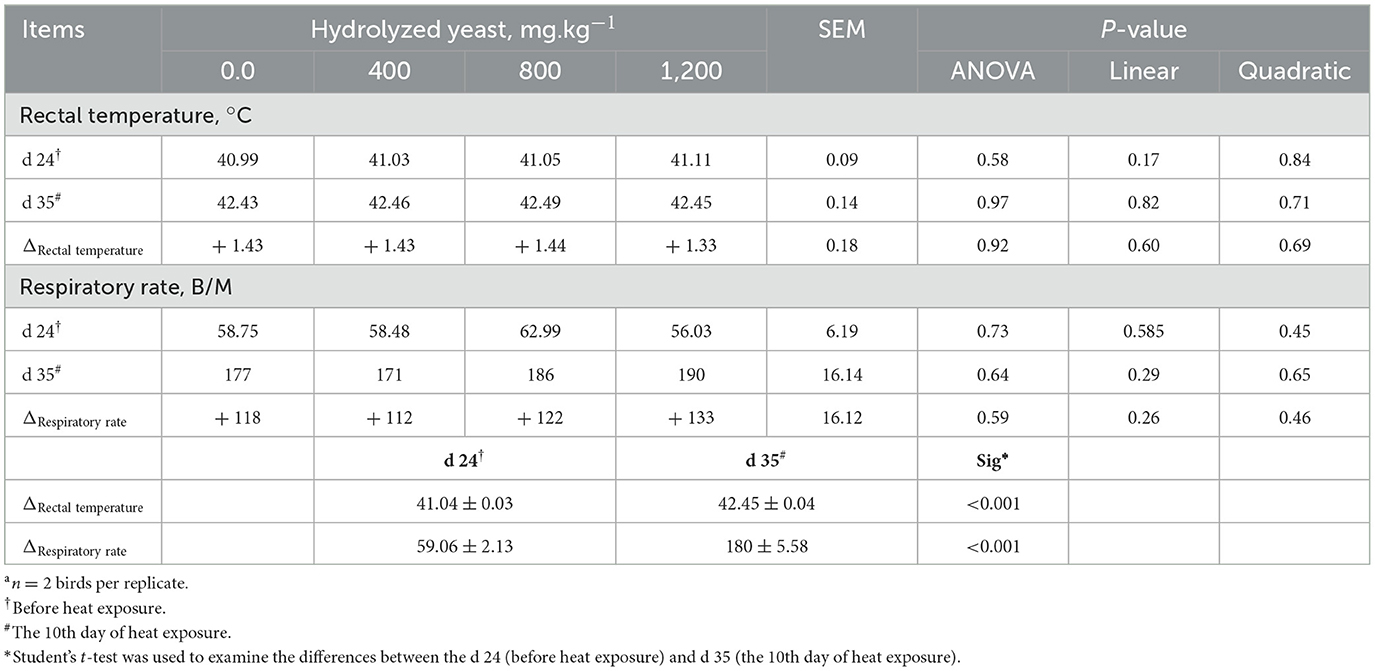
Table 3. Effects of hydrolyzed yeast on rectal temperature (°C) and respiratory rate (breaths/min) on d 24 and d 35 of agea.
3.3 Growth performance
The data on growth performance are presented in Table 4. The addition of HY had no effect on body weight, weight gain, and feed intake. The addition of HY at 800 mg.kg−1 numerically (P > 0.05) decreased FCR on days 11–24, 1–24, and 25–35 (heat stress period) and the overall FCR (d 0–35) by 7.80, 6.0, 2.50, and 5.0%, respectively. The addition of HY reduced the mortality rate (P = 0.005) during the heat stress period (d 25–35).
3.4 Carcass traits
As presented in Table 5, the addition of HY had no significant (P > 0.05) effect on carcass yield, fat pad, and meat portions (breast yield, legs yield, and wings yield). In addition, giblets yield (heart, liver, and gizzard) and the index of organs (spleen, bursa, and pancreas) were not changed (P > 0.05) by HY application.
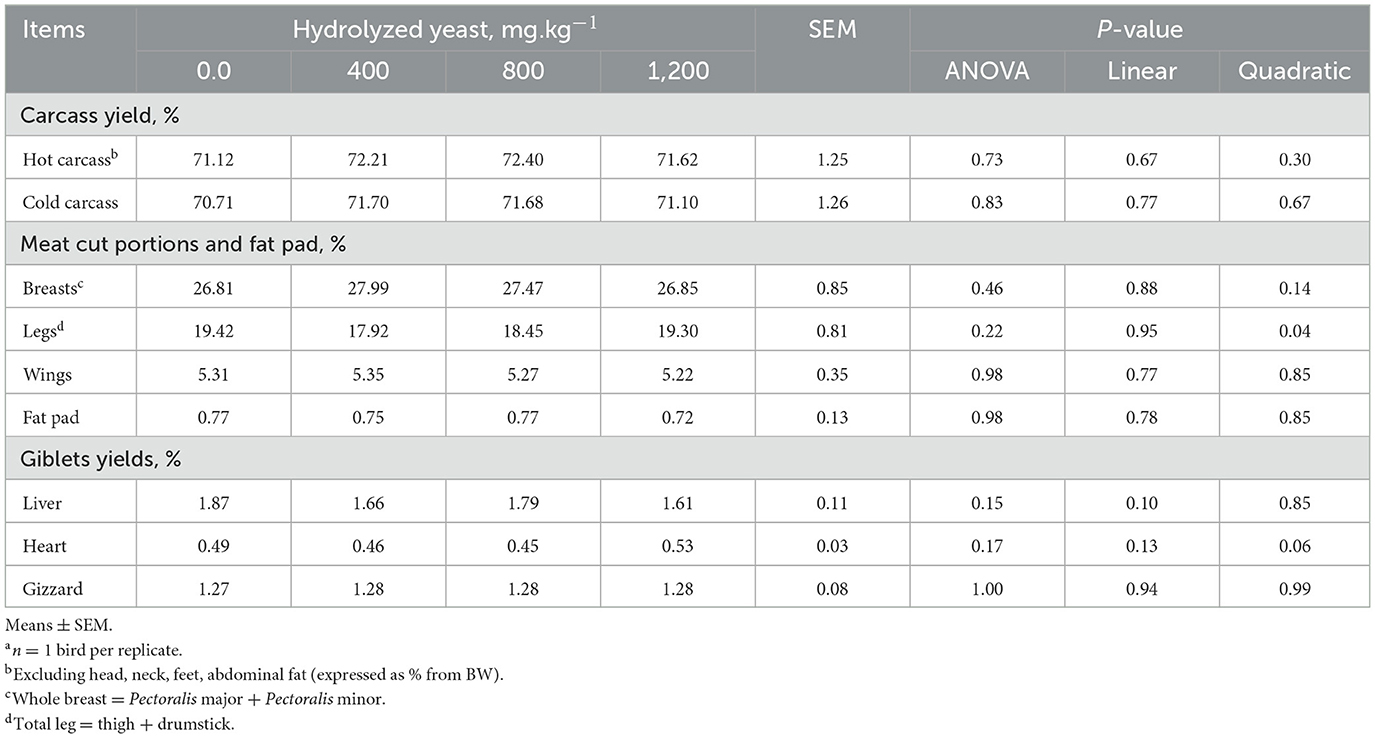
Table 5. Effects of hydrolyzed yeast on carcass yield and meat cut portions in heat-stressed broilersa.
3.5 Quality properties of breast filets (Pectoralis major) and woody breast scores
The data on meat quality are presented in Table 6. The added HY decreased (P < 0.001) drip loss of the Pectoralis major compared with the control diet group. The addition of HY at 400 and 1,200 mg.kg−1 decreased (P < 0.001) cooking loss in the Pectoralis major. No adverse effects (P > 0.05) were found in response to supplement dietary HY on initial or ultimate pH and color of breast filets among the dietary treatments.
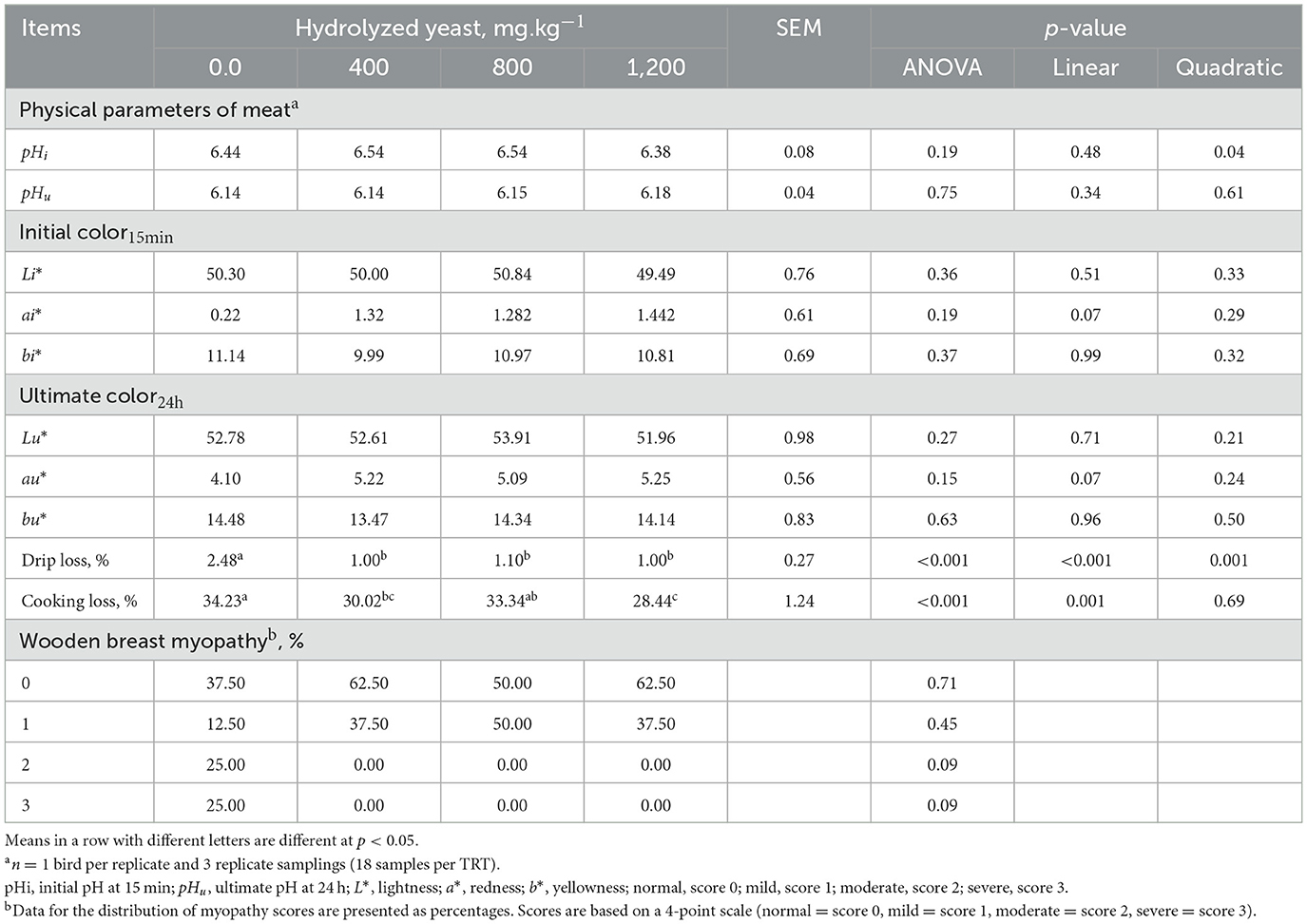
Table 6. Effects of hydrolyzed yeast on quality properties of Pectoralis Major in heat-stressed broilers.
3.6 Woody breast scores of Pectoralis major muscles
Woody breast myopathy scores are presented in Table 6 and Figures 1, 2. At d 35 of age, woody breast scores in all diets ranged from normal to severe. Supplementation of HY exhibited efficacy (P = 0.09) in diminishing woody breasts in terms of incidence and degree of severity.
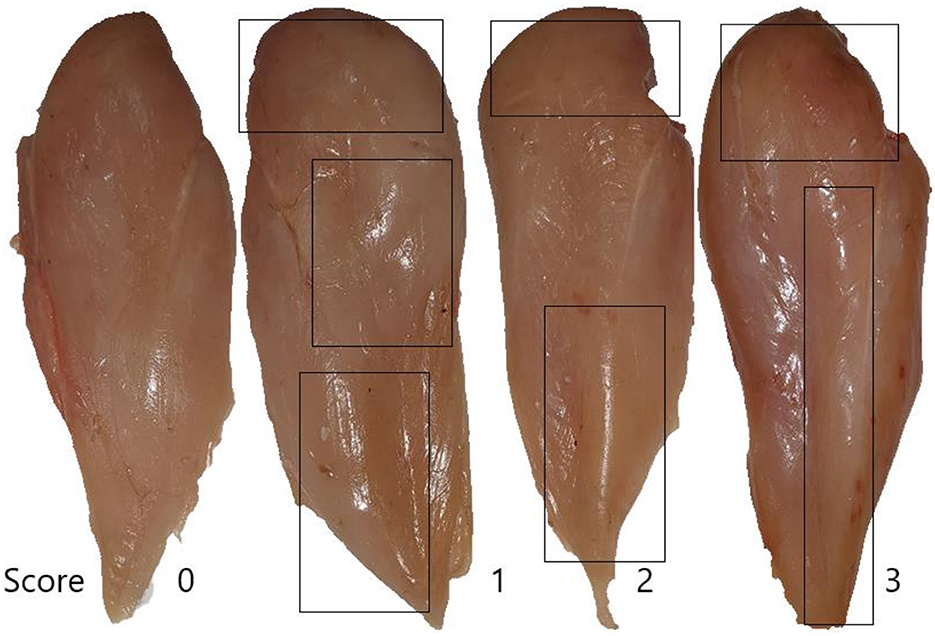
Figure 1. Woody scores are based on a 4-point scale (normal = score 0, mild = score 1, moderate = score 2, severe = score 3).
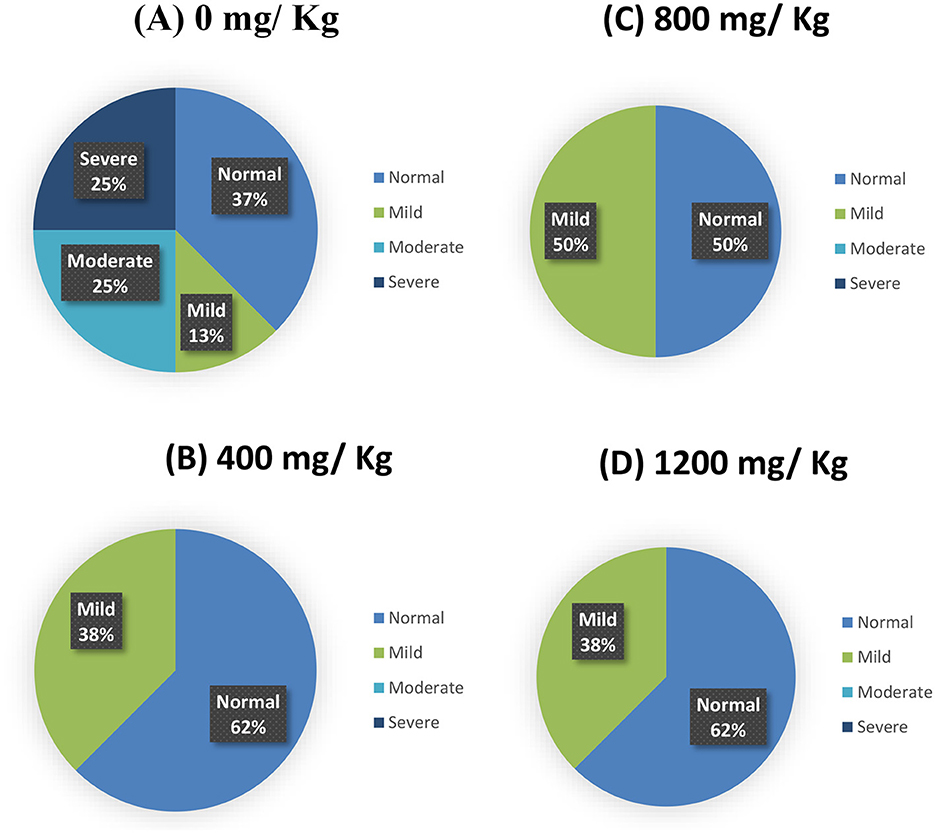
Figure 2. Woody breast scores (a percent distribution) for the incidence of wooden breast myopathy of male broiler chickens fed a hydrolyzed yeast. (A) Control diet (0); (B) HY at 400 mg.kg−1; (C) HY at 800 mg.kg−1; (D) HY at 1,200 mg.kg−1.
3.7 Duodenal mRNA expression
As presented in Figures 3, 4, supplementation of HY at 800 mg.kg−1 decreased (P = 0.04) duodenal mRNA expression of the avian β-defensin 10 (AvBD10) and increased (P < 0.05) mRNA expression of nuclear factor erythroid 2–related factor 2 (Nrf2), nuclear factor kappa-light-chain-enhancer of activated B cells (NF-κB), and secretory IgA (sIgA). The addition of HY at 400 and 800 mg.kg−1 decreased (P = 0.001) duodenal mRNA expression of copper and zinc superoxide dismutase (Cu-ZnSOD1). HY supplementation tended to decrease (P = 0.07) duodenal mRNA expression of HSP70.
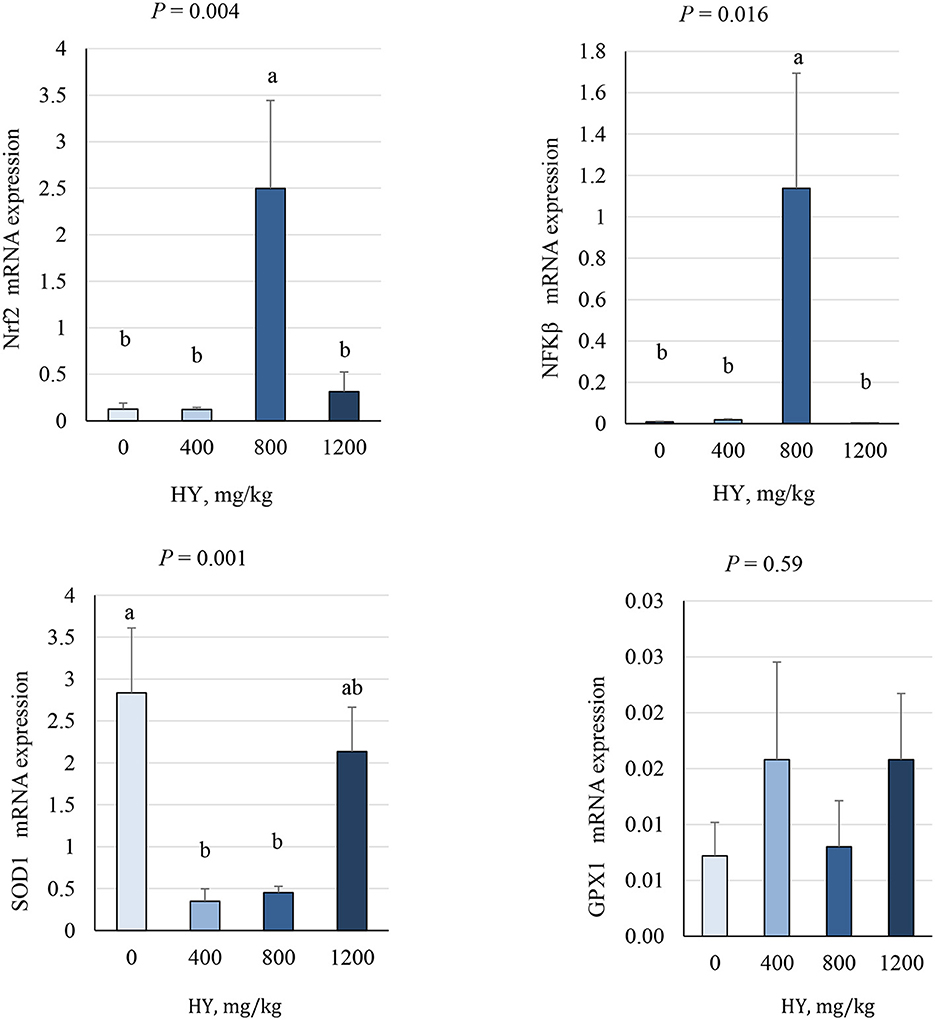
Figure 3. Effect of hydrolyzed yeast (HY) on the duodenal mRNA expression level of Nrf2, NF-κB, SOD1, and GPX1 of heat-stressed broiler chickens. Values are means ± SEM. Means on each bar with no common letter differ significantly at P < 0.05.
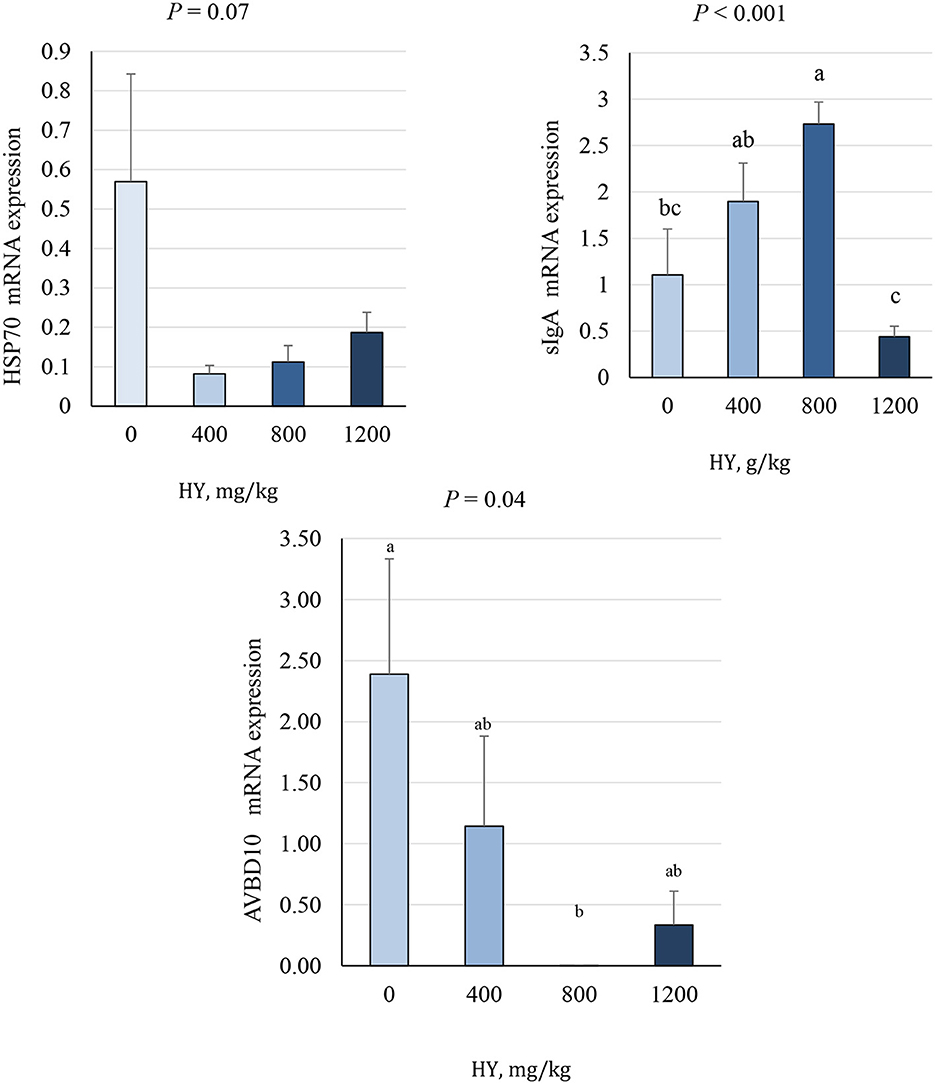
Figure 4. Effect of hydrolyzed yeast (HY) on the duodenal mRNA expression level of HSP70, sIgA, and AVBD10 of heat-stressed broiler chickens. Values are means ± SEM. Means on each bar with no common letter differ significantly at P < 0.05.
4 Discussion
The temperature–humidity index and the increase, on average, in the respiratory rate and rectal temperatures confirmed that the heat stress model was able to stimulate a heat stress challenge (24, 34, 35). According to our knowledge, no study has been reported on the effect of supplemental HY on the growth performance, intestinal redox homeostasis and health, and quality properties of breast filets of broilers in environments with high temperatures. The findings presented herein indicated that the addition of HY at 800 mg.kg−1 decreased FCR numerically. Previous studies reported different conclusions concerning the effect of Saccharomyces cerevisiae hydrolysate on the growth performance of broiler chickens. A recent study reported that the addition of Saccharomyces cerevisiae hydrolysate at different doses (500 mg.kg−1, d 0–28; 250 mg.kg−1, d 29–42) increased broiler body weight only at 28 d (36). In addition, feeding of enzymatically treated yeast did not influence growth performance during grower and finisher phases in broiler chickens subjected to the Eimeria challenge (37) or did not completely alleviate the effect of the coccidia challenge on broiler chickens (38). In the present study, heat stress challenge was adopted, and this may explain why HY did not completely alleviate the negative effects of heat stress on the growth performance. It seems that the response of Saccharomyces cerevisiae hydrolysate differs depending on age, the amount dosage, duration included, and diseases.
In the present study, the added hydrolyzed yeast resulted in the lowest drip loss. In addition, the added hydrolyzed yeast at concentrations of 400 and 1,200 mg.kg−1 resulted in the lowest cooking loss (from −12 to −17%; respectively) of breast filets compared with the control diet. Recently, dietary yeast hydrolysate beneficially affected antioxidant status in blood and liver in broilers translating into lower cooking loss of breast muscle (10). Similarly, it has been found that the added B. subtilis fmbJ improved oxidative stability in the serum and liver, resulting in decreased cooking loss and drip loss in the breast filets of broiler chickens (39). Cooking loss and drip loss are the methods for measuring water holding capacity in the meat industry that refers to the ability of meat to keep inherent or added moisture throughout processing and cooking and, consequently, leads to better protein functionality and greater cooking yields (40, 41). It has been indicated that the gut health (microbiome) can interact with the muscle called “the intestinal microbiota–muscle axis”, leading to a possibility to improve muscle characteristics, drip-losing rate, and energy metabolism of muscle (42–45).
Heat stress affects the negative expression of essential transcription factors [e.g., nuclear factor kappa-light-chain-enhancer of activated B cells (NF-κB) and nuclear factor erythroid 2-related factor 2 (Nrf2)], both playing vital roles in regulating antioxidant and anti-inflammatory responses in poultry (46–49). An imbalance between antioxidants and pro-oxidants can mediate the activation of NF-κB and Nrf2, which triggers an antioxidant response (50, 51), ultimately increasing the energy expenditure due to the need of bird for oxidative stress mitigation and thermoregulation. Both Nrf2 and NF-κB are regulated by the redox-sensitive factors, and the activation of NF-κB has been associated with enhanced inflammatory response, while Nrf2 is involved in cellular protection against oxidative stress and inflammation (52). On the other hand, it has been reported that increased NF-κB expression during the recovery period after heat shock help the clearance of damaged proteins or regulate protein quality control after heat stress (53). Furthermore, it has been indicated that the activation of NF-κB expression during low or moderate stresses can increase the expression of Nrf2 to improve antioxidant defenses (51). Furthermore, the addition of HY at 400 and 800 mg.kg−1 decreased duodenal mRNA expression of SOD1. It is well-acknowledged that the activation of a range of vitagenes (e.g., SOD, GSH, and HSP70) is required to maintain optimal redox balance in the cells and a reduction in the formation of oxidants and free radical (i.e., ROS) reduces the need for antioxidant enzyme production to reduce the oxidation activity (48, 51, 54). In the present study, the increased duodenal Nrf2 and NF-κB mRNA and decreased duodenal SOD1 mRNA indicated that HY supplementation at 800 mg.kg−1 maintains redox (antioxidant/prooxidant) balance under heat stress in broiler chickens.
In the present study, the addition of HY decreased the mortality rate during the heat stress period. The current result is reasonable, as found herein, the ability of HY to protect cells against oxidative stress by maintaining redox (antioxidant/prooxidant) balance. It has been reported that Saccharomyces cerevisiae and its derivatives improved the activity of antioxidant enzymes and decreased the production of malondialdehyde (49, 55). Furthermore, a reduction in mortality rates is observed herein, considering that Saccharomyces cerevisiae and its derivatives have antibacterial properties and consequently can improve health status and reduce mortality of birds under heat stress (9, 56). The present results showed that supplementation of HY at 800 mg.kg−1 decreased duodenal mRNA expression of AvBD10 and increased mRNA expression of sIgA. Avian β-defensin 10 has antimicrobial activity against S. typhimurium and E. coli (57). The downregulation of AvBD10 indicates the absence of S. Typhimurium and E. coli infection and shows that HY might have potential antimicrobial activity against microbial pathogens and might decrease the mortality caused by pathogens. It has been reported that AvBD10 exhibited bacteriostatic activity, rather than killing microbes such as Salmonella (58). In addition, following Eimeria maxima and Clostridium perfringens, the expression of AvBD10 was detected in the jejunum of broilers (59). It has been found that oral administration of Lactobacillus reuteri decreased the expression of AvBD10 in the intestine (60). Recently, fenugreek seeds downregulated ileal mRNA expression of AvBD10 and altered the cecal microbial community by increasing the population of good bacteria and decreasing bad bacteria (61). Therefore, supplementation of HY might modulate the magnitude of the immune response to protect against pathogenic microbes as evidenced by the decreased duodenal mRNA expression of AvBD10 and increased mRNA expression of sIgA. Current findings are in line with previous studies observing that supplementation of HY decreased microbial E. coli and Salmonella spp. and increased Lactobacillus spp and sIgA (8, 9, 62). To our knowledge, this is the first study to find a relationship between hydrolyzed yeast supplementation and mRNA expression of AvBD10.
5 Conclusion
The results suggest that hydrolyzed yeast supplementation to broiler chickens exposed to heat stress might improve intestinal redox homeostasis and decrease the mortality rate. The inclusion of 800 mg.kg−1 HY in the diet enhanced duodenal redox homeostasis, whereas 400–1,200 mg.kg−1 HY reduced mortality rate and exhibited lower drip loss values and reduced woody breast of Pectoralis major in terms of incidence and degree of severity.
Data availability statement
The datasets presented in this study can be found in online repositories. The names of the repository/repositories and accession number(s) can be found in the article/Supplementary material.
Ethics statement
The animal study was approved by All animal procedure was approved and performed in accordance with the Institutional Animal Care and Use Committee at King Saud University, Riyadh, Saudi Arabia (Approval no: KSUSE-21/62). The study was conducted in accordance with the local legislation and institutional requirements. Riyadh, Saudi Arabia.
Author contributions
AA-A: Data curation, Formal analysis, Investigation, Methodology, Writing – original draft, Writing – review & editing. RA: Conceptualization, Validation, Visualization, Writing – review & editing. MA-B: Data curation, Writing – review & editing. XD: Conceptualization, Validation, Visualization, Writing – review & editing. HK: Writing – review & editing. JV: Writing – review & editing. SS: Conceptualization, Validation, Writing – review & editing. MA: Conceptualization, Data curation, Funding acquisition, Investigation, Methodology, Project administration, Resources, Visualization, Writing – original draft, Writing – review & editing.
Funding
The author(s) declare financial support was received for the research, authorship, and/or publication of this article. This study was supported by Researchers Supporting Project Number (RSPD2024R731) and King Saud University (Riyadh, Saudi Arabia).
Conflict of interest
HK and JV are employees at Hankkija Oy.
The remaining authors declare that the research was conducted in the absence of any commercial or financial relationships that could be construed as a potential conflict of interest.
Publisher's note
All claims expressed in this article are solely those of the authors and do not necessarily represent those of their affiliated organizations, or those of the publisher, the editors and the reviewers. Any product that may be evaluated in this article, or claim that may be made by its manufacturer, is not guaranteed or endorsed by the publisher.
Supplementary material
The Supplementary Material for this article can be found online at: https://www.frontiersin.org/articles/10.3389/fvets.2024.1484150/full#supplementary-material
References
1. Forgetta V, Rempel H, Malouin F, Vaillancourt Jr R, Topp E, Dewar K, et al. Pathogenic and multidrug-resistant Escherichia fergusonii from broiler chicken. Poult Sci. (2012) 91:512–25. doi: 10.3382/ps.2011-01738
2. Helmy YA, Taha-Abdelaziz K, Hawwas HA, Ghosh S, AlKafaas SS, Moawad MM, et al. Antimicrobial resistance and recent alternatives to antibiotics for the control of bacterial pathogens with an emphasis on foodborne pathogens. Antibiotics. (2023) 12:274. doi: 10.3390/antibiotics12020274
3. Johnson CN, Kogut MH, Genovese K, He H, Kazemi S, Arsenault RJ. Administration of a postbiotic causes immunomodulatory responses in broiler gut and reduces disease pathogenesis following challenge. Microorganisms. (2019) 7:268. doi: 10.3390/microorganisms7080268
4. Żółkiewicz JA, Marzec MR, Feleszko W. Postbiotics—a step beyond pre-and probiotics. Nutrients. (2020) 12:2189. doi: 10.3390/nu12082189
5. Zamojska D, Nowak A, Nowak I, Macierzyńska-Piotrowska E. Probiotics and postbiotics as substitutes of antibiotics in farm animals: a review. Animals. (2021) 11:3431. doi: 10.3390/ani11123431
6. Araujo LF, Bonato M, Barbalho R, Araujo CS, Zorzetto PS, Granghelli CA, et al. Evaluating hydrolyzed yeast in the diet of broiler breeder hens. J Appl Poult Res. (2018) 27:65–70. doi: 10.3382/japr/pfx041
7. Perricone V, Sandrini S, Irshad N, Savoini G, Comi M, Agazzi A. Yeast-derived products: the role of hydrolyzed yeast and yeast culture in poultry nutrition—A review. Animals. (2022) 12:1426. doi: 10.3390/ani12111426
8. Sampath V, Han K, Kim IH. Influence of yeast hydrolysate supplement on growth performance, nutrient digestibility, microflora, gas emission, blood profile, and meat quality in broilers. J Anim Sci Technol. (2021) 63:563. doi: 10.5187/jast.2021.e61
9. Bortoluzzi C, Barbosa JG, Pereira R, Fagundes NS, Rafael JM, Menten JF. Autolyzed yeast (Saccharomyces cerevisiae) supplementation improves performance while modulating the intestinal immune-system and microbiology of broiler chickens. Front Sustain Food Syst. (2018) 2:85. doi: 10.3389/fsufs.2018.00085
10. Wang T, Cheng K, Yu C, Tong Y, Yang Z, Wang T. Effects of yeast hydrolysate on growth performance, serum parameters, carcass traits, meat quality and antioxidant status of broiler chickens. J Sci Food Agric. (2022) 102:575–83. doi: 10.1002/jsfa.11386
11. Wang T, Cheng K, Li Q, Wang T. Effects of yeast hydrolysate supplementation on intestinal morphology, barrier, and anti-inflammatory functions of broilers. Anim Biosci. (2022) 35:858. doi: 10.5713/ab.21.0374
12. Zhang ZY, Jia GQ, Zuo JJ, Zhang Y, Lei J, Ren L, et al. Effects of constant and cyclic heat stress on muscle metabolism and meat quality of broiler breast fillet and thigh meat. Poult Sci. (2012) 91:2931–7. doi: 10.3382/ps.2012-02255
13. Zaboli G, Huang X, Feng X, Ahn DU. Ahn. How can heat stress affect chicken meat quality?–a review. Poult Sci. (2019) 98:1551–6. doi: 10.3382/ps/pey399
14. Leishman EM, Ellis J, van Staaveren N, Barbut S, Vanderhout RJ, Osborne VR, et al. Baes Meta-analysis to predict the effects of temperature stress on meat quality of poultry. Poult Sci. (2021) 100:101471. doi: 10.1016/j.psj.2021.101471
15. Abasht B, Mutryn MF, Michalek RD, Lee WR. Oxidative stress and metabolic perturbations in wooden breast disorder in chickens. PLoS ONE. (2016) 11:e0153750. doi: 10.1371/journal.pone.0153750
16. Xing T, Pan X, Zhang L, Gao F. Hepatic oxidative stress, apoptosis, and inflammation in broiler chickens with wooden breast myopathy. Front Physiol. (2021) 12:659777. doi: 10.3389/fphys.2021.659777
17. Soglia F, Petracci M, Davoli R, Zappaterra M. A critical review of the mechanisms involved in the occurrence of growth-related abnormalities affecting broiler chicken breast muscles. Poult Sci. (2021) 100:101180. doi: 10.1016/j.psj.2021.101180
18. Dridi JS, Greene ES, Maynard CW, Brugaletta G, Ramser A, Christopher CJ, et al. Duodenal metabolic profile changes in heat-stressed broilers. Animals. (2022) 12:1337. doi: 10.3390/ani12111337
19. Burkholder KM, Thompson KL, Einstein ME, Applegate TJ, Patterson JA. Influence of stressors on normal intestinal microbiota, intestinal morphology, and susceptibility to Salmonella enteritidis colonization in broilers. Poult Sci. (2008) 87:1734–41. doi: 10.3382/ps.2008-00107
20. Quinteiro-Filho WM, Calefi AS, Cruz DS, Aloia TP, Zager A, Astolfi-Ferreira CS, et al. Heat stress decreases expression of the cytokines, avian β-defensins 4 and 6 and Toll-like receptor 2 in broiler chickens infected with Salmonella Enteritidis. Vet Immunol Immunopathol. (2017) 186:19–28. doi: 10.1016/j.vetimm.2017.02.006
21. Akbarian A, Michiels J, Degroote J, Majdeddin M, Golian A, De Smet S. Association between heat stress and oxidative stress in poultry; mitochondrial dysfunction and dietary interventions with phytochemicals. J Anim Sci Biotechnol. (2016) 7:37. doi: 10.1186/s40104-016-0097-5
22. Shakeri M, Cottrell JJ, Wilkinson S, Le HH, Suleria HA, Warner RD, et al. Growth performance and characterization of meat quality of broiler chickens supplemented with betaine and antioxidants under cyclic heat stress. Antioxidants. (2019) 8:336. doi: 10.3390/antiox8090336
23. Liu L, Ren M, Ren K, Jin Y, Yan M. Heat stress impacts on broiler performance: a systematic review and meta-analysis. Poult Sci. (2020) 99:6205–11. doi: 10.1016/j.psj.2020.08.019
24. Goel A, Ncho CM, Choi YH. Regulation of gene expression in chickens by heat stress. J Anim Sci Biotechnol. (2021) 12:1–13. doi: 10.1186/s40104-020-00523-5
25. AOAC International. Official Methods of Analysis of AOAC Int. 18th Ed,. Rev. 2nd Edn. Gaithersburg, MD: AOAC International (2006).
26. Azzam MM, Qaid MM, Al-Mufarrej SI, Al-Garadi MA, Albaadani HH, Alhidary IA. Rumex nervosus leaves meal improves body weight gain, duodenal morphology, serum thyroid hormones, and cecal microflora of broiler chickens during the starter period. Poult Sci. (2020) 99:5572–81. doi: 10.1016/j.psj.2020.08.023
27. Azzam MMM, Dong XY, Xie P, Wang C, Zou XT. The effect of supplemental L-threonine on laying performance, serum free amino acids, and immune function of laying hens under high-temperature and high-humidity environmental climates. J Appl Poult Res. (2011) 20:361–70. doi: 10.3382/japr.2010-00308
28. Al-Abdullatif A, Azzam MM. Effects of hot arid environments on the production performance, carcass traits, and fatty acids composition of breast meat in broiler chickens. Life. (2023) 13:1239. doi: 10.3390/life13061239
29. Tijare VV, Yang FL, Kuttappan VA, Alvarado CZ, Coon CN, Owens CM. Meat quality of broiler breast fillets with white striping and woody breast muscle myopathies. Poult Sci. (2016) 95:2167–73. doi: 10.3382/ps/pew129
30. Al-Abdullatif A, Hussein E, Suliman G, Akasha M, Al-Badwi M, Ali H, et al. Evaluating rice bran oil as a dietary energy source on production performance, nutritional properties and fatty acid deposition of breast meat in broiler chickens. Foods. (2023) 12:366. doi: 10.3390/foods12020366
31. Berri C, Besnard J, Relandeau C. Increasing dietary lysine increases final pH and decreases drip loss of broiler breast meat. Poult Sci. (2008) 87:480–4. doi: 10.3382/ps.2007-00226
32. Azzam MM, Al-Abdullatif A, Akasha M, Alhotan R, Suliman G, Lahaye L, et al. Effects of protected complex of biofactors and antioxidants on growth performance, serum biochemistry, meat quality, and intestinal antioxidant and immunomodulatory-related gene expressions of broiler chickens. Poult Sci. (2023) 102:102666. doi: 10.1016/j.psj.2023.102666
33. Livak KJ, Schmittgen TD. Analysis of relative gene expression data using real-time quantitative PCR and the 2(-Delta Delta C(T)) Method. Methods. (2001) 25:402–8. doi: 10.1006/meth.2001.1262
34. Samal L, Sejian V, Bagath M, Krishnan G, Manimaran A, Bhatta R. Different heat stress indices to quantify stress response in livestock and poultry. In: Rao GSL, Varma GG, Beena V, , editors. Livestock Meteorology 1st Edn. New Delhi: New India Publishing Agency (2017).
35. Lu Z, He XF, Ma BB, Zhang L, Li JL, Jiang Y, Zhou GH, Gao F. The alleviative effects and related mechanisms of taurine supplementation on growth performance and carcass characteristics in broilers exposed to chronic heat stress. Poult Sci. (2019) 98:878–86. doi: 10.3382/ps/pey433
36. Lin J, Comi M, Vera P, Alessandro A, Qiu K, Wang J, et al. Effects of Saccharomyces cerevisiae hydrolysate on growth performance, immunity function, and intestinal health in broilers. Poult Sci. (2023) 102:102237. doi: 10.1016/j.psj.2022.102237
37. Alagbe EO, Schulze H, Adeola O. Growth performance, nutrient digestibility, intestinal morphology, cecal mucosal cytokines and serum antioxidant responses of broiler chickens to dietary enzymatically treated yeast and coccidia challenge. J Anim Sci Biotechnol. (2023) 14:57. doi: 10.1186/s40104-023-00846-z
38. Kiarie EG, Mohammadigheisar M, Schulze H. Effects of early feeding of enzymatically treated yeast on growth performance, organ weights, intestinal histomorphology, and ceca microbial metabolites in broiler chickens subjected to Eimeria challenge. Poult Sci. (2022) 101:101967. doi: 10.1016/j.psj.2022.101967
39. Bai K, Huang Q, Zhang J, He J, Zhang L, Wang T. Supplemental effects of probiotic bacillus subtilis fmbJ on growth performance, antioxidant capacity, and meat quality of broiler chickens. Poult Sci. (2017) 96:74–82. doi: 10.3382/ps/pew246
40. Mir NA, Rafiq A, Kumar F, Singh V, Shukla V. Determinants of broiler chicken meat quality and factors affecting them: a review. J Food Sci Technol. (2017) 54:2997. doi: 10.1007/s13197-017-2789-z
41. Puolanne E. Chapter 8. Developments in our understanding of water-holding capacity in meat. In:Purslow PP, , editor. Woodhead Publishing Series in Food Science, Technology and Nutrition, New Aspects of Meat Quality. Woodhead Publishing, (2017). pp. 167–90. doi: 10.1016/B978-0-08-100593-4.00009-6
42. Grosicki GJ. Fielding RA, Lustgarten MS. Gut microbiota contribute to age-related changes in skeletal muscle size, composition, and function: biological basis for a gut-muscle axis. Calcif Tissue Int. (2018) 102:433–42. doi: 10.1007/s00223-017-0345-5
43. Qi R, Sun J, Qiu X, Zhang Y, Wang J, Wang Q, et al. The intestinal microbiota contributes to the growth and physiological state of muscle tissue in piglets. Sci Rep. (2021) 11:11237. doi: 10.1038/s41598-021-90881-5
44. Zhao J, Huang Y, Yu X. A narrative review of gut-muscle axis and sarcopenia: the potential role of gut microbiota. Int J Gen Med. (2021) 14:1263–73. doi: 10.2147/IJGM.S301141
45. Lei J, Dong Y, Hou Q, He Y, Lai Y, Liao C, Kawamura Y, Li J, Zhang B. Intestinal microbiota regulate certain meat quality parameters in chicken. Front Nutr. (2022) 9:747705. doi: 10.3389/fnut.2022.747705
46. Sahin K, Smith MO. Regulation of transcription factors by the epigallocatechin-3-gallate in poultry reared under heat stress. Worlds Poult Sci J. (2016) 72:299–306. doi: 10.1017/S0043933916000209
47. Sahin N, Hayirli A, Orhan C, Tuzcu M, Akdemir FA, Komorowski JR, et al. Effects of the supplemental chromium form on performance and oxidative stress in broilers exposed to heat stress. Poult Sci. (2017) 96:4317–24. doi: 10.3382/ps/pex249
48. Surai PF, Kochish II, Fisinin VI, Kidd MT. Antioxidant defence systems and oxidative stress in poultry biology: an update. Antioxidants. (2019) 8:235. doi: 10.3390/antiox8070235
49. Liu WC, Ou BH, Liang ZL, Zhang R, Zhao ZH. Algae-derived polysaccharides supplementation ameliorates heat stress-induced impairment of bursa of Fabricius via modulating NF-κB signaling pathway in broilers. Poult Sci. (2021) 100:101139. doi: 10.1016/j.psj.2021.101139
50. Li W, Kong AN. Molecular mechanisms of Nrf2-mediated antioxidant response. Mol Carcinog. (2009) 48:91–104. doi: 10.1002/mc.20465
51. Surai PF, Kochish II, Kidd MT. Redox homeostasis in poultry: regulatory roles of NF-κB. Antioxidants. (2021) 10:186. doi: 10.3390/antiox10020186
52. Yerra VG, Negi G, Sharma SS, Kumar A. Potential therapeutic effects of the simultaneous targeting of the Nrf2 and NF-κB pathways in diabetic neuropathy. Redox Biol. (2013) 1:394–7. doi: 10.1016/j.redox.2013.07.005
53. Nivon M, Abou-Samra M, Richet E, Guyot B, Arrigo AP. Kretz-Remy C.NF-κB regulates protein quality control after heat stress through modulation of the BAG3–HspB8 complex. J Cell Sci. (2012) 125:1141–51. doi: 10.1242/jcs.091041
54. Mishra B, Jha R. Oxidative stress in the poultry gut: Potential challenges and interventions. Front Vet Sci. (2019) 4:60. doi: 10.3389/fvets.2019.00060
55. Guo W, Gu X, Tong Y, Wang X, Wu J, Chang C. Protective effects of mannan/β-glucans from yeast cell wall on the deoxyniyalenol-induced oxidative stress and autophagy in IPEC-J2 cells. Int J Biol Macromol. (2019) 135:619–29. doi: 10.1016/j.ijbiomac.2019.05.180
56. Ahiwe EU, Dos Santos TT, Graham H, Iji PA. Can probiotic or prebiotic yeast (Saccharomyces cerevisiae) serve as alternatives to in-feed antibiotics for healthy or disease-challenged broiler chickens? A review. J Appl Poult Res. (2021) 30:100164. doi: 10.1016/j.japr.2021.100164
57. Yacoub HA, Elazzazy AM, Abuzinadah OA, Al-Hejin AM, Mahmoud MM, Harakeh SM. Antimicrobial activities of chicken β-defensin (4 and 10) peptides against pathogenic bacteria and fungi. Front Cell Infect Microbiol. (2015) 17:5–36. doi: 10.3389/fcimb.2015.00036
58. Mowbray CA, Niranji SS, Cadwell K, Bailey R, Watson KA, Hall J. Gene expression of AvBD6-10 in broiler chickens is independent of AvBD6, 9, and 10 peptide potency. Vet Immunol Immunopathol. (2018) 202:31–40. doi: 10.1016/j.vetimm.2018.06.007
59. Hong YH, Song W, Lee SH, Lillehoj HS. Differential gene expression profiles of β-defensins in the crop, intestine, and spleen using a necrotic enteritis model in 2 commercial broiler chicken lines. Poult Sci. (2012) 91:1081–8. doi: 10.3382/ps.2011-01948
60. Nii T, Jirapat J, Isobe N, Yoshimura Y. Effects of oral administration of Lactobacillus reuteri on mucosal barrier function in the digestive tract of broiler chicks. J Poult Sci. (2020) 57:67–76. doi: 10.2141/jpsa.0190035
61. Paneru D, Tellez-Isaias G, Bottje WG, Asiamah E, Abdel-Wareth AA, Salahuddin M, et al. Modulation of immune response and cecal microbiota by dietary fenugreek seeds in broilers. Vet Sci. (2024) 11:57. doi: 10.3390/vetsci11020057
Keywords: broilers, hydrolyzed yeast, intestinal redox and health, woody breast, heat stress
Citation: Al-Abdullatif AA, Alhotan RA, Al-Badwi MA, Dong X, Kettunen H, Vuorenmaa J, Sakr SA and Azzam MM (2024) Effects of hydrolyzed yeast on growth performance, intestinal redox homeostasis, and woody breast myopathy in heat-stressed broilers. Front. Vet. Sci. 11:1484150. doi: 10.3389/fvets.2024.1484150
Received: 22 August 2024; Accepted: 27 October 2024;
Published: 18 November 2024.
Edited by:
Arda Yıldırım, Gaziosmanpaşa University, TürkiyeReviewed by:
Jose L. Vicente-Salvador, Novozymes, United StatesArda Sözcü, Bursa Uludağ, University, Türkiye
Copyright © 2024 Al-Abdullatif, Alhotan, Al-Badwi, Dong, Kettunen, Vuorenmaa, Sakr and Azzam. This is an open-access article distributed under the terms of the Creative Commons Attribution License (CC BY). The use, distribution or reproduction in other forums is permitted, provided the original author(s) and the copyright owner(s) are credited and that the original publication in this journal is cited, in accordance with accepted academic practice. No use, distribution or reproduction is permitted which does not comply with these terms.
*Correspondence: Mahmoud M. Azzam, bWF6emFtQGtzdS5lZHUuc2E=