- State Key Laboratory of Herbage Improvement and Grassland Agro-Ecosystems, College of Pastoral Agriculture Science and Technology, Engineering Research Center of Grassland Industry, Ministry of Education, Lanzhou University, Lanzhou, China
Introduction: Standing milkvetch (Astragalus adsurgens) is widely distributed in the wild in Eurasia and North America and has been bred for cultivated forage in China. Yellow stunt and root rot disease caused by Alternaria gansuense is the primary disease of standing milkvetch. A. gansuense promotes the production of swainsonine in the plant. This study aimed to determine the safety of standing milkvetch that is infected with A. gansuense as forage for animals.
Methods: Two-week-old specific pathogen-free (SPF) male white mice were fed a commercial mouse feed (CMF), healthy plant feed (HPF) and diseased plant feed (DPF) for 3 or 6 weeks. We observed histological changes in the liver and kidney tissues of the mice and measured their daily feed intake, daily water intake, body weight, feed utilization, organ coefficients, and activities of serum enzymes.
Results: The results showed that the daily feed intake of the mice that were fed DPF and HPF was significantly higher (p < 0.05) than those fed CMF at 3 and 6 weeks. The highest increase was observed in the daily water intake of the mice fed HPF (p < 0.05) followed by DPF and CMF. However, the mice fed DPF gained the least weight (p < 0.05). There was a significantly higher percentage of liver weight to body weight of the mice fed DPF (p < 0.05) than those fed HPF for 3 weeks and those fed CMF for 3 and 6 weeks. There were significantly higher levels of concentrations of alanine aminotransferase in the mice fed DPF and HPF than those fed CMF for 3 weeks (p < 0.05) and 6 weeks (p < 0.01). However, there was no significant difference in the mice fed HPF than those fed DPF. There were significantly higher of lactate dehydrogenase concentration (p < 0.001), while the blood urea nitrogen was lower in the mice fed DPF than those fed HPF and CMF at 3 weeks. There was a significantly higher percentage of numbers of lymphocytes in the blood of the mice fed DPF (p < 0.05) than those fed HPF, but the percentages of monocytes and eosinophils were significantly lower. Comparatively, there were more apparent pathological changes in the liver and kidney tissues of the mice fed with DPF than in those fed with HPF.
Discussion: These findings indicate that standing milkvetch was toxic to white mice, and infection with A. gansuense increased its toxicity. Therefore, we conclude that standing milkvetch plants infected by A. gansuense must never be used as animal feed under any circumstances. Additionally, the amount of healthy standing milkvetch fed to animals should be appropriate, avoiding long-term or excessive feeding.
1 Introduction
Standing milkvetch (Astragalus adsurgens), which is widely planted in northern China, is a palatable and nutritious forage for livestock and poultry (1, 2). It grows rapidly and is characterized by tolerance to soil with low nutrients, wide adaptability, and strong resistance; therefore, it plays an important role in the restoration of degraded ecosystems (3, 4).
Fungal diseases are a major limiting factor of standing milkvetch in pastures (5). Yellow stunt and root rot (YSRR) of standing milkvetch is the most important fungal disease of standing milkvetch, and it was first reported in Huanxian, Gansu. The disease was later reported in Ningxia, Shaanxi, Inner Mongolia, Yunnan, and Beijing, which are the primary areas of standing milkvetch production. The incidence of the disease can be as high as 82.6%, with a disease index of 53.7 (6). YSRR is a systemic disease that causes various symptoms, including yellowing, crumpling or even defoliation, plant dwarfing, dark brown stalks in the late stages of the disease, and the rotting of stem bases, rhizomes, and main roots, as well as death, which seriously exacerbates the degradation of grasslands (7).
The causative pathogen of YSRR was identified as a new species in 2007 based on its morphological characteristics and named Embellisia astragali (8). Later, E. astragali was renamed Alternaria gansuense based on its molecular and morphological characteristics (9).
The pathogen A. gansuense is closely related to the locoweed endophyte A. sect. Undifilum spp., which produces poisonous swainsonine and causes locoism in livestock (9, 10). Previous studies have shown that both diseased plants infected by A. gansuense and healthy uninfected plants contain swainsonine, but its content is higher in diseased plants than in healthy ones (11).
Higher contents of swainsonine of locoweed that reach or exceed 0.001% can cause livestock poisoning with continuous feeding. However, a low content of swainsonine owing to a low intake of locoweed can lead to weight loss without causing livestock poisoning (12). The concentration of alkaline phosphatase (ALP) and aspartate aminotransferase (AST) increased in the serum of rats fed solely with the endophyte or locoweed, while the concentration of α-mannosidase (AMA) decreased (11). In addition, vacuolization appeared on the pancreatic, renal, and hepatic tissues of the rats that were fed locoweed (13). Swainsonine is one of the key toxins produced by locoweed (14–18) and causes enormous stockbreeding losses in China and the USA (19). A previous study revealed that locoweed plants free of endophytes were non-toxic (20). However, it is not clear whether healthy standing milkvetch and plants infected with A. gansuense can cause animal poisoning as locoweed does. Therefore, this study aimed to assess whether healthy standing milkvetch and plants infected with A. gansuense are safe for animals.
2 Materials and methods
2.1 Animals and feed
A total of 48 two-week-old specific pathogen-free (SPF) male white mice (Mus musculus) of the BABL/C variety, weighing 18–20 g, were obtained from the Animal Experimental Center, Lanzhou University, China, for the study. The experimental scheme was approved by the Lanzhou University College of Grassland Agricultural Science and Technology Ethics Committee.
Healthy and diseased standing milkvetch plants were collected from Huanxian County, Gansu Province, China. The diseased plants (infected with A. gansuense) had brown stems, while the healthy plants were free from any symptoms. The plants were oven-dried at 80°C for 24 h and then ground in a mill (FZ102, Yongguangming, China) using a 1-mm diameter screen. Commercial mouse feed (CMF) (Keao Xieli, China) was also ground with the same mill and separated into three parts. The diseased and healthy foliage powder was added to the first and second parts of the CMF powder at a weight of 20%, respectively, and no foliage powder was added to the third part. Each part was mixed thoroughly, and a fodder machine (KL120-200, Xinghui, China) was used to create a 40-mm long cylinder that was 12 mm in diameter. The three mouse feeds were then added to 25% water of the total weight and dried at 80°C for 12 h, respectively. The feeds that contained healthy and diseased standing milkvetch powder were named healthy plant feed (HPF) and diseased plant feed (DPF), respectively. The contents of crude protein, crude fat, crude fiber, moisture, ash, calcium, and phosphorus of the three mouse feeds were then measured (21).
2.2 Mice feeding with the three feed types
The study included 3-week and 6-week experiments to assay the serum enzymes and another 6-week experiment for the hematology assay. In each experiment, eight male mice were fed CMF, HPF, and DPF. For each experiment, two male mice were kept in a 45 cm × 30 cm × 28 cm plastic cage. The cages were randomly arranged in a block in a feeding instrument equipped with an aeration system where many pipes were individually connected to each cage (Fengshi, IVC-II, China). The instrument was kept at a room temperature of 18–22°C under a 12 h light/12 h dark photo period and 30–60% relative humidity. The room was disinfected with 40% formalin and UV light for 24 h before the experiment. The feeds were placed onto the net atop the cages, and distilled ion-ex water was supplied in the drinking bottles for the mice.
2.3 Measurement indicators during the feeding period
The daily feed intake and body weight of the two mice in cages were measured using an electronic balance (CP324S, Odolish, China), and the daily water intake was measured using a graduated flask at 9:00 a.m. every day. The eating, drinking, movement, and sleep of the mice were observed daily during the feeding period to determine any signs of poisoning. The daily feed and water intake were calculated as follows:
Daily feed intake = The feed weight fed the previous day - Remaining feed weight the next day.
Daily water intake = The water amount fed the previous day - Remaining water amount the next day.
2.4 Measurement indicators after the mice had been euthanized
2.4.1 Tissue weight and histology
The mice were euthanized at weeks 3 and 6, and the heart, liver, pancreas, spleen, and kidney tissues were excised and weighed. The tissues were subsequently observed to determine any pathogenic signs and then stored in glass jars in 10% formalin. A histological analysis was performed by embedding the tissues in paraffin, followed by sectioning, staining with hematoxylin and eosin, and observation under a microscope (Olympus, NC300-1, Tokyo, Japan).
2.4.2 Serum enzymes
Blood samples were obtained from the eyes of mice during both the 3-week and 6-week experiments (conducted for serum enzyme assay) and were allowed to incubate stationary for 12 h. The serum was separated by centrifugation (XKA-2200, Xiangyi, China). The activities of serum ALP, alanine aminotransferase (ALT), AST, and lactate dehydrogenase (LDH) and the content of blood urea nitrogen (BUN) were immediately assayed with an automatic biochemical analytical instrument (XL-300, Erba, Mannheim, Germany). α-Mannosidase was assayed using a mouse α-mannosidase ELISA kit (USCN Life Science, USA) and an auto ELISA detector (RT-6100, Rayto, USA) according to the manufacturer’s instructions.
2.4.3 Hematology
Blood samples were collected from the eyes of mice during the 6-week experiment (conducted for hematology assay) and were transferred into tubes containing malondialdehyde (MDA). The hematology analysis was conducted using an automatic animal blood analysis instrument (Hemavet 950, Drew Scientific, Plantation, FL, USA).
2.5 Statistical analysis
The feed and water intake data from the two mice in each cage were averaged. The feed utilization efficiency (FUE) was calculated using the weight gained and total intake (22) as follows:
In the formula, “the total food intake” represents the total amount of food consumed by the two mice in each cage, and the weight gain represents the combined total of the two mice in each cage.
The organ coefficient (OC) for each tissue was calculated using the weight of each tissue and body weight (23) as follows:
The means were subjected to a one-way ANOVA using SPSS 11.5.0 (SPSS Inc., Chicago, IL, USA) and compared using Duncan’s new multiple range test.
3 Results
3.1 Nutrient components of the feeds
The contents of crude protein and ash of the healthy and diseased plant feeds were slightly higher than those of the CMF. There were much higher contents of crude fiber in the two plant feeds, but their contents of crude fat and non-nutrient extracts were slightly lower than those in the CMF. However, there were no significant differences between the two types of plant feed (Table 1).
3.2 Feed and water intake, gained weight, and feed utilization
There was a significantly higher mean daily intake for the DPF and HPF than for the CMF during the 3-week (p < 0.01) and 6-week (p < 0.01) experiments. However, there were no significant differences between the DPF and HPF.
The mice fed DPF had the lowest mean daily water intake, while those fed the HPF had the highest in the 3-week (p < 0.05) and 6-week (p < 0.05) experiments.
The least amount of total weight gained by the mice fed the DPF occurred in weeks 3 and 6 (p < 0.05), but the highest amount occurred in those fed the CMF for 3 weeks and those fed the HPF for 6 weeks.
The utilization efficiency was the lowest for the DPF in all the experiments (p < 0.05), but the highest for the CMF in the 3-week experiment and for the HPF in the 6-week experiment (Table 2).
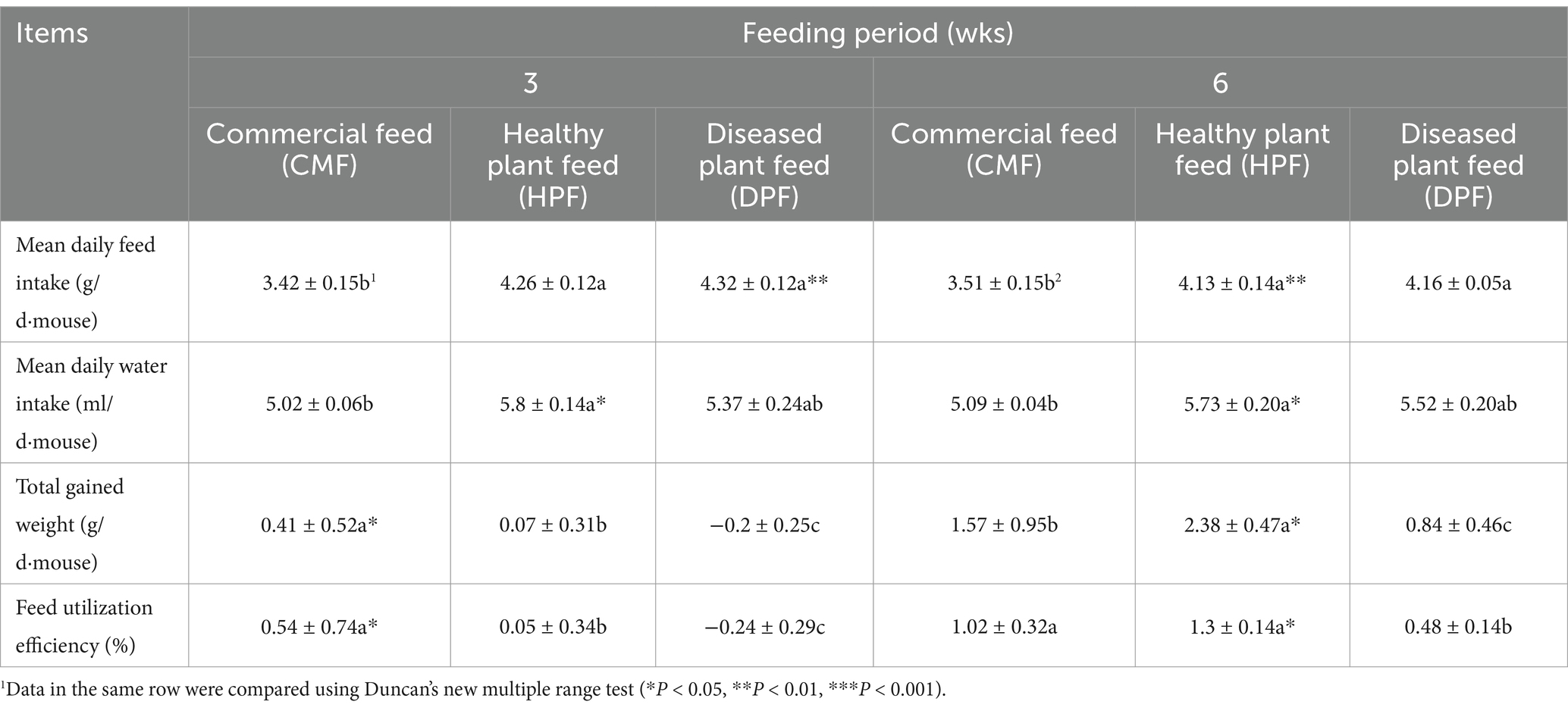
Table 2. Feed and water intake, gained weight and feed utilization efficiency of white mice fed with the feed contained 20% diseased standing milkvetch foliage infected by A. gansuense compared with fed with feed contained 20% healthy standing milkvetch foliage and a commercial feed for 3 and 6 wks.
3.3 Organ coefficient
Liver coefficients of the mice fed the DPF were significantly greater than those of mice fed the CMF for 3 weeks (p < 0.001) and 6 weeks (p < 0.05) (Figures 1, 2) and mice fed the HPF for 3 weeks (Figure 1). There was a significantly higher spleen coefficient in the mice fed the DPF (p < 0.01) than in those fed the CMF and HPF for 3 weeks (Figure 2). Furthermore, there was a significantly higher pancreas coefficient in the mice fed the DPF (p < 0.05) than in those fed the HPF for 3 weeks, but it was not significantly different (p > 0.05) from those fed the HPF for 6 weeks. There was no difference in the pancreas coefficient between the mice fed the DPF and those fed the CMF (Figures 1, 2).
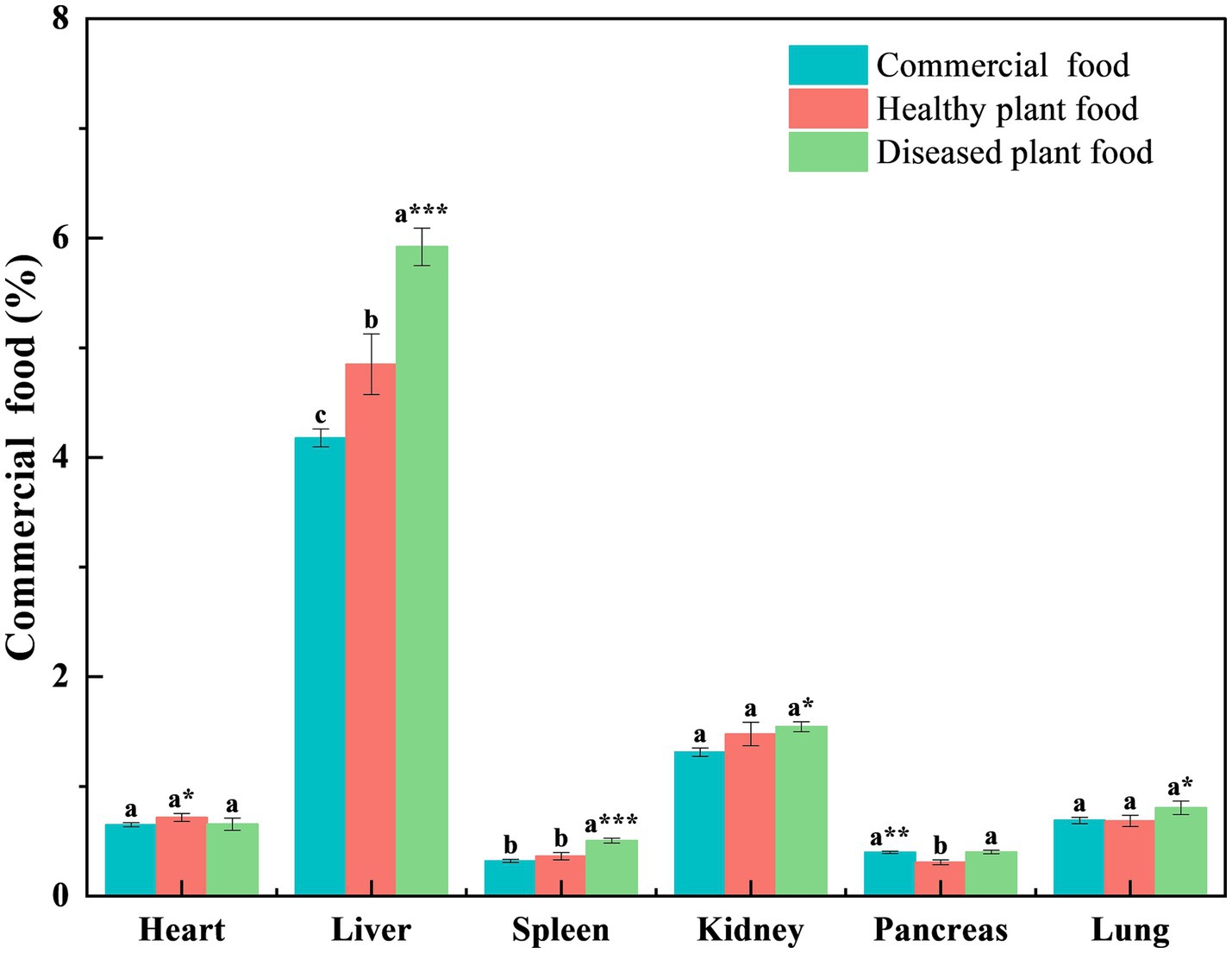
Figure 1. Organ coefficient of white mice fed with feed that contained 20% of standing milkvetch foliage infected with Alternaria gansuense compared to those fed with feed that contained 20% of healthy standing milkvetch foliage and a commercial feed for 3 weeks. Data (mean ± SE) within the same organ were compared using Duncan’s new multiple range test. *p < 0.05, **p < 0.01, and ***p < 0.001. Bars topped by the same lowercase letter do not differ significantly between treatments.
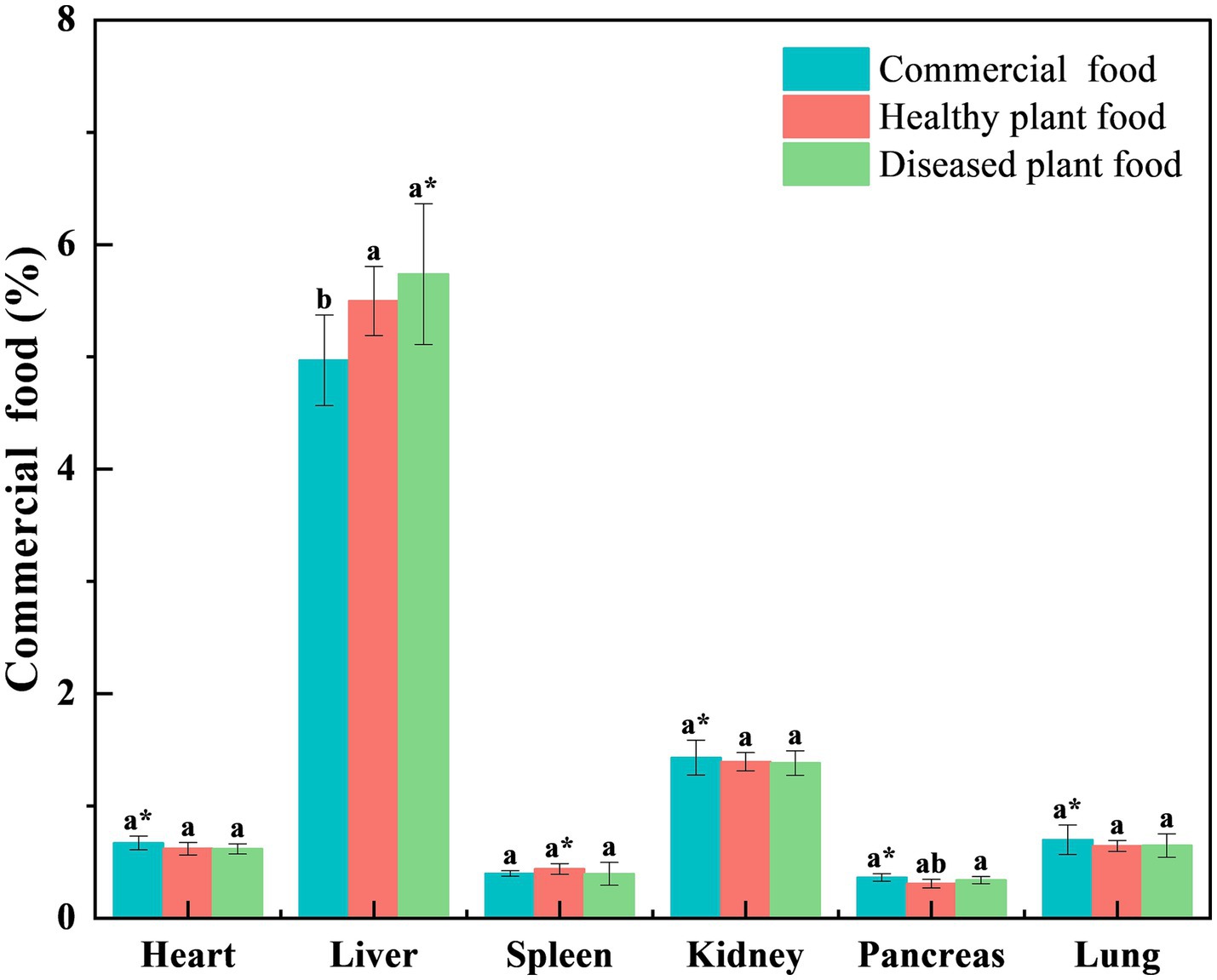
Figure 2. Organ coefficient of white mice fed with feed that contained 20% of standing milkvetch foliage infected with Alternaria gansuense compared to those fed with feed that contained 20% of healthy standing milkvetch foliage and a commercial feed for 6 weeks. Data (mean ± SE) within the same organ were compared using Duncan’s new multiple range test. *p < 0.05, **p < 0.01, and ***p < 0.001. Bars topped by the same lowercase letter do not differ significantly between treatments.
3.4 Serum enzyme activities
At 3 and 6 weeks of feeding, the ALT concentrations in the serum of mice were as follows: 36.37 ± 9.30 and 24.49 ± 2.68 U/L for the DPF group, 34.03 ± 9.69 and 29.41 ± 3.74 U/L for the HPF group, and 11.26 ± 0.91 and 14.83 ± 1.88 U/L for the CMF group, respectively. There were significantly higher concentrations of ALT in the mice fed DPF and HPF than in those fed CMF for 3 weeks (p < 0.05) and 6 weeks (p < 0.01). However, mice fed the HPF did not differ significantly from those fed the DPF.
At 3 and 6 weeks of feeding, the BUN concentrations were as follows: 2.15 ± 0.15 and 1.43 ± 0.30 mmol/L in the DPF group, 1.71 ± 0.16 and 1.79 ± 0.14 mmol/L in the HPF group, and 2.36 ± 0.18 and 1.44 ± 0.13 mmol/L in the CMF group, respectively. The BUN concentration in the mice fed the DPF was between the BUN concentrations of those fed the HPF and CMF, while the mice fed the HPF had a significantly lower (p < 0.05) concentration of BUN than those fed CMF for 3 weeks. There was no significant difference in the concentration of BUN between the groups at 6 weeks.
At 3 and 6 weeks of feeding, the LDH concentrations were as follows: 269.07 ± 14.09 and 166.30 ± 16.31 U/L in the DPF group, 154.50 ± 12.66 and 218.40 ± 27.59 U/L in the HPF group, and 210.04 ± 12.49 and 223.14 ± 14.48 U/L in the CMF group, respectively. The mice fed DPF had significantly higher levels of LDH concentration than those fed HPF and CMF, and the mice fed HPF had significantly lower levels of LDH concentration than those fed CMF for 3 weeks. There was no significant difference in the concentration of LDH between the groups at 6 weeks.
At 3 weeks of feeding, the AMA, AST, and ALP concentrations were as follows: 2.20 ± 0.05, 89.01 ± 8.56, and 53.15 ± 5.55 U/L in the DPF group, 2.03 ± 0.05, 73.01 ± 12.45, and 44.74 ± 7.48 U/L in the HPF group, and 2.23 ± 0.11, 62.99 ± 6.75, and 44.54 ± 7.73 in the CMF group, respectively. At 6 weeks of feeding, the AMA, AST, and ALP concentrations were as follows: 1.97 ± 0.07, 58.83 ± 10.98, and 55.41 ± 3.56 U/L in the DPF group, 2.26 ± 0.16, 70.01 ± 5.57, and 46.86 ± 8.96 U/L in the HPF group, and 2.08 ± 0.05, 62.70 ± 7.79, and 50.54 ± 6.63 in the CMF group, respectively. There were no significant differences in the AMA, AST, and ALP concentrations between the groups at 3 and 6 weeks (Table 3).
3.5 Hematology
There were significantly higher numbers of eosinophils in the blood (p < 0.05) in the mice fed the HPF than in those fed the CMF. However, the levels were similar to those fed the DPF. There was a significantly higher percentage of neutrophils in the blood of mice fed the DPF and HPF than in those fed the CMF, and there was no significant difference between the DPF and HPF (p < 0.001). There was a significantly higher percentage of lymphocytes in the blood of mice fed the DPF and CMF than in the mice fed the HPF, and there was no significant difference between the DPF and CMF (p < 0.001). The percentage of monocytes in the blood of mice fed the HPF was significantly higher than that of mice fed the DPF (p < 0.01). There was a significantly higher percentage of eosinophils in the blood of mice fed the HPF than in those fed the DPF and CMF, and there was no significant difference between the DPF and the CMF (p < 0.05). There was no significant difference in the numbers of white blood cells, neutrophils, lymphocytes, monocytes, basophils, red blood cells, and platelets in the blood of mice fed the DPF, HPF, and CMF (Table 4).
3.6 Clinical signs
No signs of poisoning were observed during the entire experiment.
3.7 Histology
The liver tissues of the mice fed the DPF and HPF were apparently larger than those fed the CMF, and some were dropsical with blood clots. There were no obvious changes in the organs.
Compared to the tissue sections of mice fed the CMF (Figures 3C,F), pathological changes appeared more severe in the liver and kidney tissues of the mice fed the DPF and HPF.
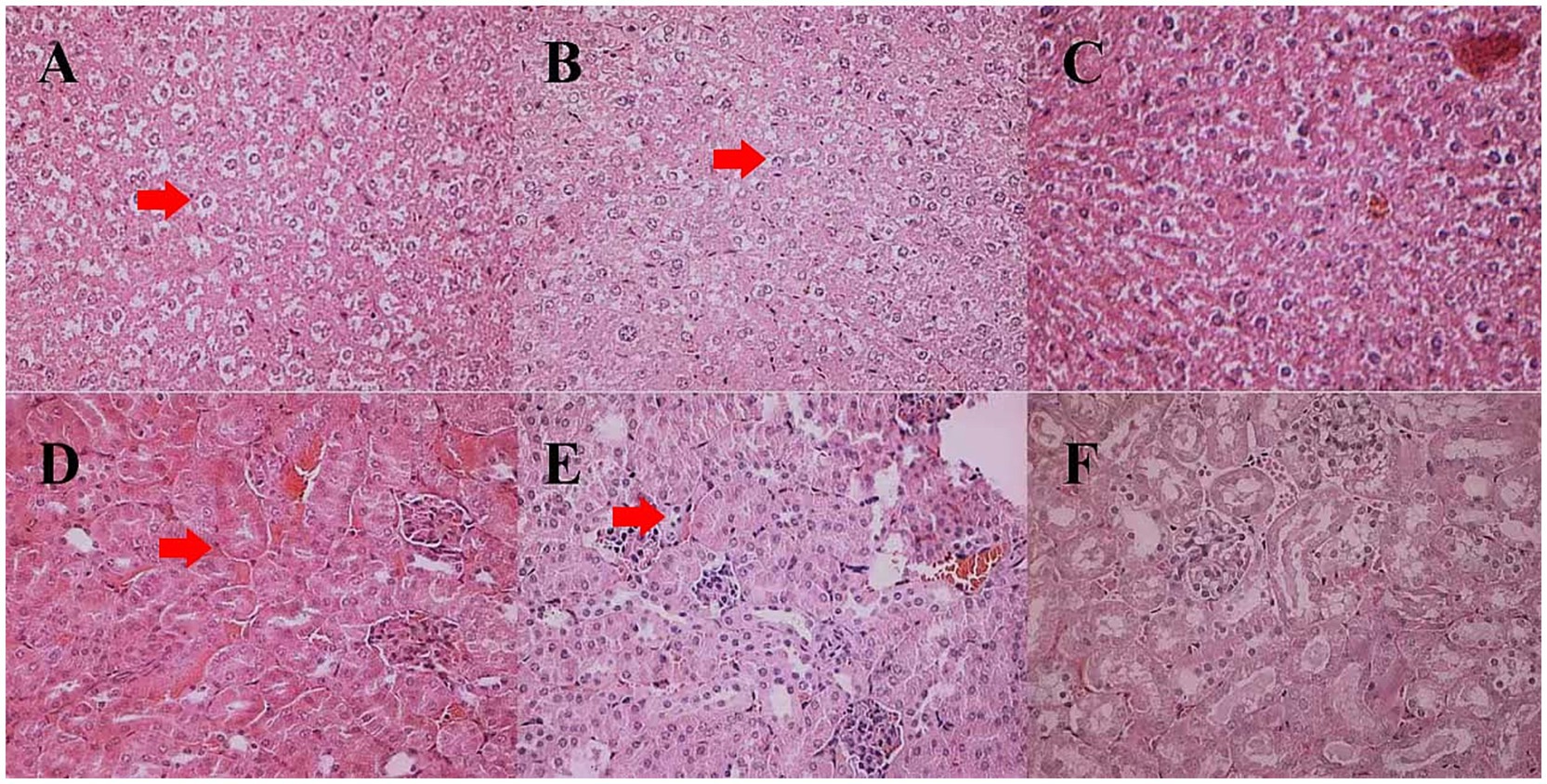
Figure 3. Histological changes in the liver and kidney tissues of white mice fed with feed that contained 20% of standing milkvetch foliage infected with Alternaria gansuense compared to those fed with feed that contained healthy standing milkvetch foliage and a commercial feed for 6 weeks. (A–C) Liver: healthy plant feed (HPF), diseased plant feed (DPF), and commercial feed (CMF), respectively. (D–F) Kidney: healthy plant feed (HPF), diseased plant feed (DPF), and commercial feed (CMF), respectively. In (A,B) the locations pointed to by red arrows show binucleated hepatocytes and vacuolation. In (D,E) the locations pointed to by red arrows show hypertrophy, hyperplasia, and proliferation of mesangial cells in the glomeruli.
The liver antra of the mice fed the HPF and DPF became narrower, with enlarged and swollen liver cells. Some cells at the edge of the liver lobule were necrotic, with double nucleoli or light-stained plasma. Cells in the center of the liver lobule were larger, with double nucleoli and large or small vacuoles (Figures 3A,B). Hypertrophy proliferation and hyperplasia were observed in the glomerular mesangial cells. Renal tubule epithelial cells were granularly degenerated, and the renal tubulointerstitial cells were dilated and congestive (Figures 3D,E).
4 Discussion
Standing milkvetch is a nutritive fodder that has been planted for a long time in China, although it is listed in the noxious plant catalog in the United States and Canada (10). This study found that healthy standing milkvetch plants were noxious to mice, as the liver coefficient and concentration of ALT were higher in the mice fed healthy standing milkvetch plants than those fed the CMF. Additionally, the viscera of the mice fed the HPF exhibited pathological changes. Many locoweeds (Oxytropis and Astragalus plants) are poisonous to livestock (13, 24). Their toxins are acknowledged to be selenium (25), nitrogenous compounds (26), and swainsonine (27).
In recent years, the toxicity of locoweed has primarily been attributed to swainsonine (28, 29). A series of obvious signs of poisoning were reported in chickens, which demonstrated that standing milkvetch is toxic to poultry (26). However, there were no evident signs of poisoning in rabbits and sheep (30). In these previous studies, hematology, activities of LDH, ALP, and ALT, and the content of hemoglobin were similar to those of the controls, although some pathological changes were observed on the viscera. Generally, the content of 3-nitropropionic acid (3-NPA) is lower in the stems, leaves, and flowers of standing milkvetch (31), and its digestion by ruminants transforms it into an innocuous compound. Therefore, some authors have considered standing milkvetch to be safe for animals and unlikely to induce toxicosis in livestock (26). However, this study found enlarged liver tissues in the mice fed the HPF. Additionally, it showed that healthy standing milkvetch plants were noxious to mice, as the liver coefficient and ALT concentration were higher in the mice fed healthy standing milkvetch plants than in those fed the CMF. Furthermore, the viscera of the mice fed the HPF exhibited pathological changes. The activity of alanine aminotransferase in the 3 week and 6 week experiments and the contents of BUN in the 3-week experiment, and the neutrophils, eosinophils, and lymphocytes in the 6-week experiment were abnormal compared to the mice fed the CMF. The decrease in BUN may correlate with liver damage (32). Eosinophils are often associated with allergies, while the increase in lymphocytes occurs because of inflammation (33). In this study, the hematology reports of the mice were compared to the reference ranges for the species (34), and no abnormalities were detected. Thus, standing milkvetch forage is harmful to animals, although no clear signs of poisoning were observed.
The DPF was more toxic than the HPF, as shown by the lower weight gain and feed utilization efficiency, the higher liver coefficient, and more severe pathological changes in most of the tissues of the mice fed DPF. Previous studies have shown that swainsonine is the primary toxic substance in standing milkvetch plants (35), and its content is higher in diseased plants than in healthy ones. A. gansuense can elevate the production of swainsonine (11). This is consistent with our research findings, and therefore, we conclude that swainsonine is the primary substance responsible for poisoning in mice. Swainsonine is an indolizidine alkaloid that is structurally similar to mannose and has a strong inhibitory effect on α-mannosidase. This inhibition disrupts the processing of N-glycans, as α-mannosidase is a key enzyme in the processing of N-glycan chains. By inhibiting α-mannosidase, swainsonine prevents the processing of glycoproteins, leading to the accumulation of unprocessed sugar chains at the mannose position. The accumulation of these sugar chains interferes with the normal function of glycoproteins, including their roles on the cell surface (35). The action mechanism of swainsonine leads to a series of biological effects, including the accumulation of complex oligosaccharides within cells, particularly in lysosomes, which can cause cellular vacuolation, especially in neurons (36). Additionally, swainsonine poisoning can affect the immune system and result in damage to the liver and kidneys (37). This substance can cause poisoning in the vast majority of animals, such as cattle, sheep, horses, and mice (38).
Research has demonstrated that endophytic fungi coexist symbiotically with plants in the genus Oxytropis, enabling them to produce swainsonine. If the endophytic fungi are removed from these plants, they cease to produce swainsonine, resulting in plants that are no longer toxic (39). Are endophytic fungi present in standing milkvetch plants? If present, would removing the endophytic fungi from the plant make it non-toxic? These questions will be the focus of future research.
In summary, our research results indicate that standing milkvetch is toxic to mice and that its toxicity is exacerbated upon infection by A. gansuense. Therefore, we conclude that standing milkvetch plants infected by A. gansuense must never be used as animal feed under any circumstances. Additionally, the amount of healthy standing milkvetch fed to animals should be appropriate, avoiding long-term or excessive feeding. The limitation of this study resides in the fact that the principal consumers of this feed are ruminant animals, while the toxicity of the feed was evaluated using white mice in this study. Future research ought to center on ruminant animals.
Data availability statement
The datasets presented in this study can be found in online repositories. The names of the repository/repositories and accession number(s) can be found in the article/supplementary material.
Ethics statement
The animal study was approved by the Animal Experimental Center, Lanzhou University, China. The study was conducted in accordance with the local legislation and institutional requirements.
Author contributions
BY: Conceptualization, Data curation, Formal analysis, Funding acquisition, Investigation, Methodology, Project administration, Resources, Software, Supervision, Validation, Visualization, Writing – original draft, Writing – review & editing. ZN: Conceptualization, Data curation, Formal analysis, Funding acquisition, Investigation, Methodology, Project administration, Resources, Software, Supervision, Validation, Visualization, Writing – original draft, Writing – review & editing. YL: Conceptualization, Data curation, Formal analysis, Funding acquisition, Investigation, Methodology, Project administration, Resources, Software, Supervision, Validation, Visualization, Writing – original draft, Writing – review & editing.
Funding
The author(s) declare that financial support was received for the research, authorship, and/or publication of this article. This study was funded by the National Key R & D Program of China (2022YFD1401103), the National Natural Science Foundation of China (No. 32061123004), the National Forestry and Grassland Administration (20220104), and The Earmarked Fund for CARS (CARS-34).
Acknowledgments
We thank Professor YL for his professional guidance. We would also like to thank MinShi for her kind support in our experimental operation.
Conflict of interest
The authors declare that the research was conducted in the absence of any commercial or financial relationships that could be construed as a potential conflict of interest.
Publisher’s note
All claims expressed in this article are solely those of the authors and do not necessarily represent those of their affiliated organizations, or those of the publisher, the editors and the reviewers. Any product that may be evaluated in this article, or claim that may be made by its manufacturer, is not guaranteed or endorsed by the publisher.
References
1. Li, YB, Song, ZQ, Zhang, T, Ding, CJ, and Chen, H. Gene expression variation of Astragalus adsurgens pall. Through discharge plasma and its activated water. Free Radic Biol Med. (2022) 182:1–10. doi: 10.1016/j.freeradbiomed.2022.02.016
2. Sun, QZ, Gui, R, Na, RS, Na, DMD, and Zhai, ZH. Study on productivity of Astragalus adsurgens pall. With different growth periods. Grassland China. (1999) 5:29–30.
3. Li, Y, Wei, WJ, Zhang, JW, Li, GD, and Gao, K. Structures and antipathogenic fungi activities of flavonoids from pathogen-infected. Nat Prod Res. (2019) 33:822–6. doi: 10.1080/14786419.2017.1413560
4. Wang, Z, and Wang, Q. Cultivating erect milkvetch (Astragalus adsurgens pall.) (leguminosae) improved soil properties in loess hilly and gullies in China. J Integr Agric. (2013) 12:1652–8.doi: 10.1016/S2095-3119(13)60394-0
5. Nan, ZB. Fungal diseases of cultivated grasses and forage legumes in loess plateau of eastern Gansu Province. Pratac Sci. (1990) 4:7
6. Li, YZ, and Nan, ZB. Symptomology and etiology of a new disease, yellow stunt, and root rot of standing milkvetch caused by Embellisia sp. in Northern China. Mycopathologia. (2007b) 163:327–34. doi: 10.1007/s11046-007-9002-2
7. Li, YZ. Studies of yellow stunt and root rot of standing milk-vetch (Astragalus adsurgens pall.). Lanzhou: Lanzhou University (2008).
8. Li, YZ, and Nan, ZB. A new species, Embellisia astragali sp. nov., causing standing milk-vetch disease in China. Mycologia. (2007a) 99:406–11. doi: 10.3852/mycologia.99.3.406
9. Liu, JL, Li, YZ, and Creamer, R. A re-examination of the taxonomic status of Embellisia astragali. Curr Microbiol. (2016) 72:404–9. doi: 10.1007/s00284-015-0962-z
10. Li, YZ, Creamer, R, Baucom, D, and Nan, ZB. Pathogenic Embellisia astragali on Astragalus adsurgens is very closely related to locoweed endophyte. Phytopathology. (2011) 101:S102–3.
11. Xu, N. Study on toxic substance of Astragalus adsurgens infected by Alternaria gansuense. Lanzhou: Lanzhou University (2017).
12. Cook, D, Gardner, DR, Welch, KD, Roper, JM, Ralphs, MH, and Green, BT. Quantitative PCR method to measure the fungal endophyte in locoweeds. J Agric Food Chem. (2009) 57:6050–4. doi: 10.1021/jf901028z
13. McLain-Romero, J, Creamer, R, Zepeda, H, Strickland, J, and Bell, G. The toxicosis of Embellisia fungi from locoweed (Oxytropis lambertii) is similar to locoweed toxicosis in rats. J Anim Sci. (2004) 82:2169–74. doi: 10.2527/2004.8272169x
14. Hamaguchi, J, Nakagawa, H, Takahashi, M, Kudo, T, Kamiyama, N, Sun, B, et al. Swainsonine reduces 5-fluorouracil tolerance in the multistage resistance of colorectal cancer cell lines. Mol Cancer. (2007) 6:58. doi: 10.1186/1476-4598-6-58
15. Ralphs, MH, Welsh, SL, and Gardner, DR. Distribution of locoweed toxin swainsonine in populations of. J Chem Ecol. (2002) 28:701–7. doi: 10.1023/A:1015228507950
16. Tulsiani, DRP, Broquist, HP, James, LF, and Touster, O. The similar effects of Swainsonine and locoweed on tissue Glycosidases and oligosaccharides of the pig indicate that the alkaloid is the principal toxin responsible for the induction of Locoism. Arch Biochem Biophys. (1984) 232:76–85. doi: 10.1016/0003-9861(84)90522-8
17. Wang, S, Panter, KE, Holyoak, GR, Liu, G, Molyneux, RJ, Evans, RC, et al. Evaluation of locoweed toxin (swainsonine) on bovine oocyte fertilization in vitro. Biol Reprod. (1999) 60:137–7.
18. Wang, Y, Gao, X, Peng, M, You, Y, and Wu, C. The mechanism of Swainsonine causing early pregnancy abnormal Decidualization and inducing abortion by changing glycosylation modification. Adv Rep Sci. (2018) 6:70–101. doi: 10.4236/arsci.2018.63007
19. Stegelmeier, BL, James, LF, Panter, KE, Gardner, DR, Pfister, JA, Ralphs, MH, et al. Dose response of sheep poisoned with locoweed (Oxytropis sericea). J Vet Diagn Invest. (1999) 11:448–56. doi: 10.1177/104063879901100510
20. Romero, J, Creamer, R, Ralphs, MH, and Gardner, DR. Association of a fungal endophyte with seed tissue and locoweed toxicity. Phytopathology. (2002) 92:S70
21. Chen, YY, Zhu, N, Chen, XY, Liu, GB, Li, YK, Guo, YQ, et al. Evaluation of pigeon pea leaves (Cajanus cajan) replacing alfalfa meal on growth performance, carcass trait, nutrient digestibility, antioxidant capacity and biochemical parameters of rabbits. J Anim Physiol Anim Nutr. (2019) 103:1265–73. doi: 10.1111/jpn.13119
22. Patience, JF, Rossoni-Serao, MC, and Gutiérrez, NA. A review of feed efficiency in swine: biology and application. J Anim Sci Biotechnol. (2015) 6:33. doi: 10.1186/s40104-015-0031-2
23. Li, K, Cao, ZJ, Guo, Y, Tong, C, Yang, SH, Long, M, et al. Selenium yeast alleviates Ochratoxin A-induced apoptosis and oxidative stress via modulation of the PI3K/AKT and Nrf2/Keap1 signaling pathways in the kidneys of chickens. Oxidative Med Cell Longev. (2020) 2020:ID4048706:1–12. doi: 10.1155/2020/4048706
24. Braun, K, Romero, J, Liddell, C, and Creamer, R. Production of swainsonine by fungal endophytes of locoweed. Mycol Res. (2003) 107:980–8. doi: 10.1017/S095375620300813x
25. Davis, AM. Selenium uptake in Astragalus and Lupinus species. Agron J. (1986) 78:727–9. doi: 10.2134/agronj1986.00021962007800040033x
26. Wang, JH. Study on safe of animal and poultry fed with Astragalus adsurgens. J Anim Sci Vet Med. (1989) 4:34–40.
27. Cook, D, Gardner, DR, Grum, D, Pfister, JA, Ralphs, MH, Welch, KD, et al. Swainsonine and endophyte relationships in Astragalus mollissimus and Astragalus lentiginosus. J Agric Food Chem. (2011) 59:1281–7. doi: 10.1021/jf103551t
28. Taylor, JB, and Strickland, JR. Appearance and disappearance of swainsonine in serum and milk of lactating ruminants with nursing young following a single dose exposure to swainsonine (locoweed; Oxytropis sericea). J Anim Sci. (2002) 80:2476–84. doi: 10.2527/2002.8092476x
29. Wang, S, Guo, R, Su, YX, Yang, C, Guo, YZ, Tan, CJ, et al. Swainsonine promotes apoptosis by impairing lysosomal function and inhibiting autophagic degradation in rat primary renal tubular epithelial cells. Chem Biol Interact. (2021) 336:109319. doi: 10.1016/j.cbi.2020.109319
30. Wu, ZL, Li, SQ, and Wang, Q. Dynamic change of toxin produced by Astragalus adsurgens. Chin J Grassland. (1991) 2:59–60.
31. Feng, P, Sun, QZ, Zheng, HY, Ye, SX, Yu, Z, and Xue, JG. Nutrient and poisonous composition in the mixed silage of maize and Astragalus adsurgens pall. With varied proportions. J Anim Vet Adv. (2012) 11:1532–7. doi: 10.3923/javaa.2012.1532.1537
32. Golab, F, Kadkhodaee, M, Zahmatkesh, M, Ghaznavi, R, Arab, HA, Seifi, B, et al. Hepatic changes during various periods of reperfusion after induction of renal ischemia in rats. Transplant Proc. (2009) 41:2749–50. doi: 10.1016/j.transproceed.2009.07.006
33. Niedzwiecki, M, Yamada, Y, Inci, I, Weder, W, and Jungraithmayr, W. Decrease of airway allergies after lung transplantation is associated with reduced basophils and eosinophils. Transplant Proc. (2016) 48:2140–6. doi: 10.1016/j.transproceed.2016.02.084
34. Trumel, C. Schalm's veterinary hematology. Vet Clin Pathol. (2023) 52:132–3. doi: 10.1111/vcp.13201
35. Wu, CC, Han, TS, Lu, H, and Zhao, BY. The toxicology mechanism of endophytic fungus and swainsonine in locoweed. Environ Toxicol Pharmacol. (2016) 47:38–46. doi: 10.1016/j.etap.2016.08.018
36. Wu, CC, Wang, WL, Liu, XX, Ma, F, Cao, DD, Yang, XW, et al. Pathogenesis and preventive treatment for animal disease due to locoweed poisoning. Environ Toxicol Pharmacol. (2014) 37:336–47. doi: 10.1016/j.etap.2013.11.013
37. Klein, JLD, Roberts, JD, George, MD, Kurtzberg, J, Breton, P, Chermann, JC, et al. Swainsonine protects both murine and human haematopoietic systems from chemotherapeutic toxicity. Br J Cancer. (1999) 80:87–95. doi: 10.1038/sj.bjc.6690326
38. Molyneux, RJ, Lee, ST, Gardner, DR, Panter, KE, and James, LF. Phytochemicals: the good, the bad and the ugly? Phytochemistry. (2007) 68:2973–85. doi: 10.1016/j.phytochem.2007.09.004
Keywords: diseased plant, feeding experiment, hematology, histology, serum enzyme, toxicity
Citation: Yang B, Nan ZB and Li YZ (2025) Toxicity of standing milkvetch infected with Alternaria gansuense in white mice. Front. Vet. Sci. 11:1477970. doi: 10.3389/fvets.2024.1477970
Edited by:
Ana M. Molina-López, University of Cordoba, SpainReviewed by:
Yi Ma, Jiangsu University, ChinaAlexandra Dreanca, University of Agricultural Sciences and Veterinary Medicine of Cluj-Napoca, Romania
Selinay Basak Erdemli-Kose, Mehmet Akif Ersoy University, Türkiye
Copyright © 2025 Yang, Nan and Li. This is an open-access article distributed under the terms of the Creative Commons Attribution License (CC BY). The use, distribution or reproduction in other forums is permitted, provided the original author(s) and the copyright owner(s) are credited and that the original publication in this journal is cited, in accordance with accepted academic practice. No use, distribution or reproduction is permitted which does not comply with these terms.
*Correspondence: Yan Zhong Li, bGl5emhAbHp1LmVkdS5jbg==