- Department of Aquaculture, Faculty of Fisheries, Recep Tayyip Erdoğan University, Rize, Turkey
In aquaculture, interest in natural essential oils is increasing alongside synthetic anesthetic agents. In this context, the anesthetic efficacy of nutmeg essential oil, which had not been previously tested, was investigated in common carp (Cyprinus carpio). The study, conducted using three different concentrations (800 μL/L “LC”, 1,200 μL/L “MC”, and 1,400 μL/L “HC”), found that induction times were <3 min for MC and HC, while LC had a longer induction time, exceeding acceptable levels. Within the first 4 h, white blood cells, red blood cells, hemoglobin, and hematocrit levels increased to >5 103/μL, >1 106/μL, >6 g/dL, and >12%, respectively. However, they returned to control levels after 8 h. Histological signs were more severe with higher concentrations, and necrosis was only observed in the HC group. Alkaline comet assay results showed DNA migration only in the HC group. According to the PROMETHEE multi-criteria decision-making model, the LC concentration is suitable for sedation, while the MC concentration should be used for deep anesthesia. The current study demonstrates that nutmeg essential oil can be used as an alternative to commercial synthetic anesthetic agents.
1 Introduction
In aquaculture, fish undergo routine procedures including fish-grading, transportation, vaccination, tagging, and surgery. These activities elevate stress levels in fish, significantly affecting their physiology and survival (1, 2). Stress negatively impacts fish physiology, causing slower growth, suppressed immune function, and inhibited reproduction (3, 4). Reduced fish welfare directly affects fish health and growth, leading to economic losses in the aquaculture industry (5). Thus, anesthetic agents are widely used in aquaculture to reduce stress and eliminate pain sensitivity. Effective sedation and anesthesia can help mitigate the negative effects associated with the hypothalamic-pituitary-interrenal (HPI) axis, which is central to physiological stress responses (6). Beyond safeguarding fish welfare, anesthesia is employed to immobilize fish during demanding procedures such as blood sampling and spawning of large broodstock. This practice not only alleviates the workload but also minimizes the risk of potential injuries. Additionally, anesthetics are often used during fish transportation to decrease metabolic activity and prevent mechanical damage.
The most commonly used anesthetics in aquaculture include Tricaine methanesulfonate (MS-222), Benzocaine, Quinaldine, 2-Phenoxyethanol (2-PE), Metomidate, Aqui-S, and clove oil (8, 83). Due to the negative effects of synthetic anesthetics on fish and their potential residues affecting humans, researchers in recent years have been investigating the anesthetic properties of plant-based essential oils (7, 9–11). The use of natural essential oils as anesthetic agents for fish is gaining increasing attention due to their potential benefits over synthetic alternatives (12). Synthetic anesthetics, while effective, can leave harmful residues that affect both aquatic environments and human health. In contrast, essential oils, derived from natural sources such as clove or lavender, offer a more eco-friendly and sustainable option (7, 10). These natural anesthetics are often less toxic, degrade more rapidly in water, and reduce the risk of harmful residues (13). Additionally, essential oils can effectively induce anesthesia while minimizing stress and injury to the fish. Their application not only enhances fish welfare during procedures like handling and transportation but also aligns with the growing emphasis on environmental sustainability and reducing chemical pollutants in aquaculture practices.
As a natural anesthetic, nutmeg, native to the Maluku Islands of Indonesia, is also distributed in India, Sri Lanka, South Africa, and the United States (14, 15). While nutmeg is primarily used as a spice (16), it is also extracted to produce essential oil, which possesses antioxidant, antimicrobial, anti-inflammatory, anticancer, and aphrodisiac properties (17–20). However, while there is one study on the potential of nutmeg powder as an anesthetic agent (21), there have been no studies conducted on its essential oil for this purpose. The reports on the narcotic use of nutmeg (22, 23) have led to the idea that this substance might induce anesthesia effects when used in appropriate concentrations. Myristicin, an important component of nutmeg, can exhibit adverse effects such as hallucinations, tachycardia, drowsiness, numbness, and blurred vision (24). Therefore, as a first step, it is necessary to investigate the potential effects of myristicin to understand how the ingestion of large amounts of nutmeg could impact patients in the field of anesthesia (25).
The effectiveness of an anesthetic agent is evaluated based on several factors, including induction time, physiological effects, and cost analysis. Responses to anesthetics can vary among different fish species, so the efficacy of an anesthetic may differ from one species to another. The common carp (Cyprinus carpio), a warm-water fish with a low trophic level, is one of the most significant species in the aquaculture industry (26). Due to its high adaptability to environmental conditions, it is considered a valuable fish for aquaculture. In many European countries, common carp constitutes more than 80% of total fish production (27). Therefore, to increase the acceptability of a previously unused natural anesthetic agent, it is essential to focus on a significant species in aquaculture. In light of this motivation, the current study focuses on the applicability and effects of nutmeg, a naturally occurring agent with anesthetic potential, on common carp. In addition, it aims to determine optimal concentration of the nutmeg oil by using a multi-criteria decision-making method (PROMETHEE).
2 Material and method
2.1 Nutmeg (Myristica fragrans)
The nutmeg (Myristica fragrans) essential oil was purchased from a commercial corporation (Doalinn, Türkiye). Since it does not dissolve in water on its own, it was dissolved in a 1:10 ratio using 96% ethanol. The content of nutmeg oil is presented in Table 1. Analyses were performed on a GC–MS (Shimadzu QP2010 Ultra) device with a 30 m 5-Ms column. The temperatures of transfer line and ion source were 280 and 275°C, respectively. Qualitative analysis was done using NIST and Wiley libraries integrated in the device. The GC-MS analysis was carried out as an outsourced service at the Central Research Laboratory Application and Research Center in Recep Tayyip Erdoğan University. Accordingly, α-pinene, β-pinene, and sabinene are the main components (>10%) of nutmeg essential oil.
2.2 Experimental trial
The current study was approved by the Ethical Committee of Recep Tayyip Erdoğan University, Türkiye (Decision No: 2024/05). The trials were conducted at the Recep Tayyip Erdoğan University Aquaculture Research and Application Center. In the study, the fish were exposed to three different anesthetic concentrations of 800 (LC), 1,200 (MC), and 1,400 (HC) μL/L, respectively. The concentrations of nutmeg oil were determined based on induction and recovery times as a result of a preliminary study. As in our previous study, ethanol was added to the control group at the highest concentration, and this is considered negligible as an anesthetic (7). A total of 120 fish (46.8 ± 4.9 g) were used, with each group consisting of three replicates and ten fish per replicate. For adaptation, the fish were placed in the trial tanks (12 different tanks with 50 L volume) 7 days before the study and were not fed during the last 48 h. The adaptation system was a flowthrough system with 7.2 ± 0.3 pH and 8 ± 0.9 mg/L DO. The water source for the study was groundwater with a constant temperature of 18°C. Induction time was determined by observing reactions such as a complete lack of response to stimuli (handling), abnormal operculum movements, and loss of balance (28). The fish behavioral responses to anesthesia were monitored over a 7-min period. Recovery was defined as the moment when the fish began to swim steadily and responded to stimuli. After induction, the anesthetized fish were immediately placed in 500 L tanks with vigorous aeration using an air pump (DO 9.8 ± 0.7 mg/L) for recovery. Induction and recovery procedures were performed individually for each fish. To eliminate observer bias, both the recovery and induction times were recorded by a single observer with a stopwatch.
2.3 Hematological examination
For hematological studies, blood samples were taken from the anesthetized fish at 2, 4, and 8 h after initial anesthesia, without additional anesthetic operation. Samples were collected from the control group without anesthesia. Five fish were randomly chosen (the fish from which blood was drawn were excluded from the study) and blood was collected from caudal vein with a 1 mL syringe. Then, blood samples were placed in EDTA K3 tubes to prevent clotting. Red blood cells (RBC), white blood cells (WBC), hemoglobin (HGB), and hematocrit (HCT) were measured with an automatic hematological analyzer (Prokan-6800VET, Shenzhen, China). The instrument was calibrated with blank blood samples of the common carp before the study (29).
2.4 Histological evaluation
Only gill tissues were considered for histological examination. Three fish (the fish from which blood was drawn were excluded from the study), exposed to the anesthesia, were euthanized (mechanical stunning), and gill tissues (second lamella) were later taken. Control fish were euthanized with mechanical stunning. Tissues were fixed in 10% neutral buffer formalin for 2 days and then transferred to 50% ethanol. Gills were subjected to alcohol series and placed into the liquid paraffin at 65°C for 12 h. Then, tissues were blocked in the paraffin and sectioned at a thickness of 5 μm using a microtome. Preparations were transferred at 65°C to remove paraffin and subjected again to ethanol and xylene series. Afterwards, samples were stained with hematoxylin and eosin and covered with Entellan® (MERCK/107961.0500) and cover-slip. Histological changes were observed with light microscope and imaged (30).
2.5 Alkaline comet assay
A maximum of 10 μL blood sample was taken from the caudal vein of both anesthetized and control fish. An alkaline (pH > 13) environment was provided for erythrocyte DNA damage assessment (31). Blood samples taken from three fish (from each group) were mixed with 1 mL Phosphate Buffed Saline (PBS-Ca ++, MG ++ free). Subsequently, 15 μL of this cell suspension (~10,000 cells) was taken and mixed with 75 μL of 0.5% low melting point (LMP) agarose. In the further stage, the frosted glass slides were covered with three layers: (1) 1% normal melting point (NMP) agarose, (2) LMP + cell suspension, and (3) 100 μL LMP agarose. These layers were then allowed to solidify at 4°C. The samples were incubated in lysing solution (2.5 m NaCl, 100 mm EDTA, 10 mm Tris, PH 10.0–10.5, 1% Triton X-100 and 10% DMSO) for 8 h to allow for cell lysis. The slides were then placed in a horizontal gel electrophoresis unit containing fresh cold alkaline electrophoresis buffer and cell migration was achieved for 30 min at 25 V and 300 mA. After this step, samples were treated for 10 min with neutralized buffer. Slides were washed with distilled water for 10 min and stained with 0.5 μg/ml ethidium bromide. Distilled water was used to prevent over staining. All procedures up to this stage were carried out at +4°C and in a dark environment. For the positive control, hydrogen peroxide (H2O2) treatment (three fish) was applied for 10 min. For each sample, three slides were examined under the fluorescent microscope (Leica DMR HC, Germany). In addition, cells were analyzed using Comet ScoreTM 2.0 Software (Tritek Crop., Sumemeruck, VA, USA).
2.6 PROMETHEE decision model
The optimal concentration was determined based on a multi-criterion decision-making (MCDM). The Preference Ranking Organization Method for Enrichment (PROMETHEE) model comprises four fundamental steps: (1) investigation of the appropriate anesthetic concentration for common carp, (2) determination of evaluation criteria and weights for each concentration group, (3) scoring the different concentration groups depending on evaluation criteria, and (4) decision on the best anesthetic concentration. Each alternative was weighted according to several evaluation criteria. Each evaluation criterion contains a weight value, and the sum of these values is equal to “1” (32).
According to the MCDM principle, alternatives should be evaluated based on the specified criteria. These criteria are used by the decision-maker when assessing and reaching a conclusion. To determine the optimal anesthetic concentration, evaluation criteria are considered based on feasibility, cost, effectiveness of the anesthetic agent, and the physiological condition of the fish. In the present study, 15 different evaluation criteria were identified. These criteria were categorized under five main headings: (1) induction and recovery times, (2) cost analysis, (3) hematological parameters, (4) histological changes, and (5) DNA comet assay. The weight value for each criterion was determined based on its importance factor through expert opinions. Thus, the weight values were determined by evaluating the responses received from 10 different professionals in the field of aquaculture (32). The evaluation criteria and their weights are presented in Table 2. For cost analysis, one liter of anesthetic was considered to cost 900 USD ($) and was normalized based on the amount of anesthetic used for each fish species. The average value of blood parameters at the eighth hour for the relevant group was evaluated. For histological changes, a five-point Likert scale indicating the severity of lesions was used. The PROMETHEE decision model reaches results in seven steps (Supplementary material 1).
2.7 Statistical analysis
All data are presented as the means ± standard deviation (SD). The Kolmogorov-Smirnov test was conducted to assess the normality of the distribution. Significant differences between groups were determined with One-way ANOVA and Tukey tests. Differences were considered statistically significant when the calculated p-value was <0.05. All analyses were performed in SPSS software (Version 23, IBM Corp., Armonk, New York, USA).
3 Results
3.1 Effectiveness of anesthetic agent
The concentration of nutmeg essential oil is significant for induction and recovery times (p < 0.05). Fish in the LC group were induced significantly longer, taking more than 5 min (Figure 1). Conversely, fish in the HC group recovered in a significantly longer time (<5 min) compared to other groups.
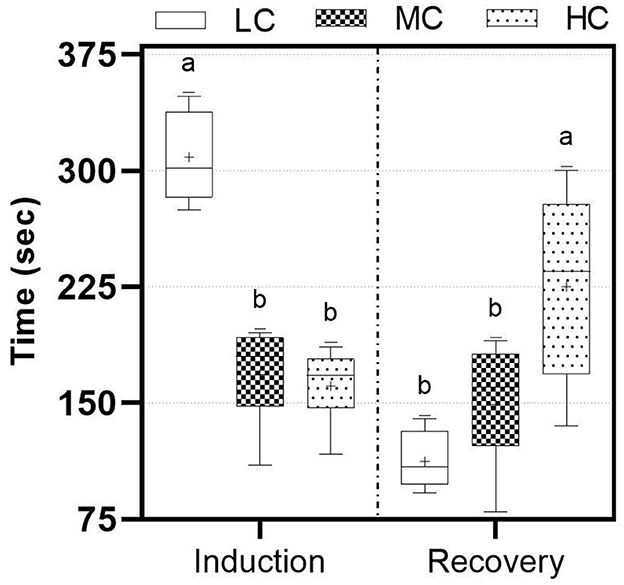
Figure 1. Induction and recovery times according to different nutmeg essential oil concentration. LC, 800 μL/L; MC, 1,200 μL/L; HC, 1,400 μL/L. Lowercase letters represent statistical differences.
3.2 Hematological results
Blood samples taken at the 2nd, 4th, and 8th hours from anesthetized fish demonstrate the temporal response of the fish's blood parameters (Figure 2). Blood parameters for WBC, RBC, HGB, and HCT increased at the 4th hour and returned to control levels at the 8th hour. The critical peak for all blood parameters was identified between the 5th and 6th hour across all groups. No significant differences in blood parameters were observed at any time points among the concentration groups (p > 0.05).
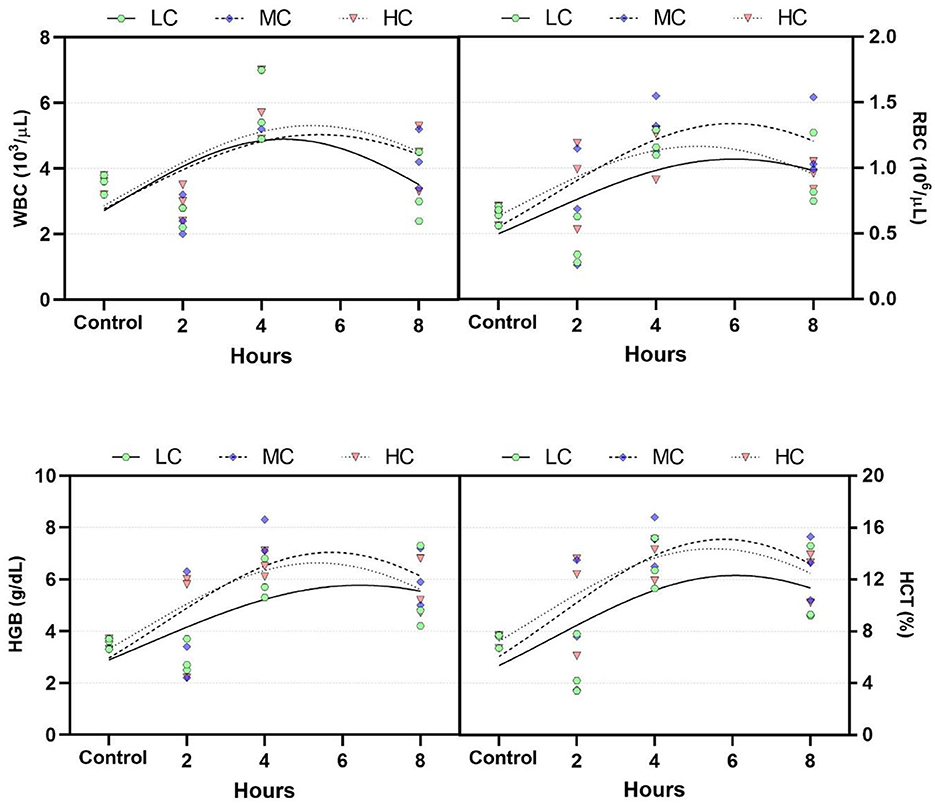
Figure 2. Changes in blood parameters over time. LC, 800 μL/L; MC, 1,200 μL/L; HC, 1,400 μL/L. WBC, white blood counts; LYM, lymphocyte counts; RBC, red blood counts; HGB, hemoglobin concentration.
3.3 Histological studies
The gill tissues of fish anesthetized with different concentrations of nutmeg essential oil, as well as control fish, were histologically evaluated (Figure 3). Hyperplasia was observed in the gills of control fish. Additionally, hyperplasia and epithelial lifting were noted in the LC and MC groups. In the HC group, epithelial lifting and necrotic cells were prominently observed (Table 3).
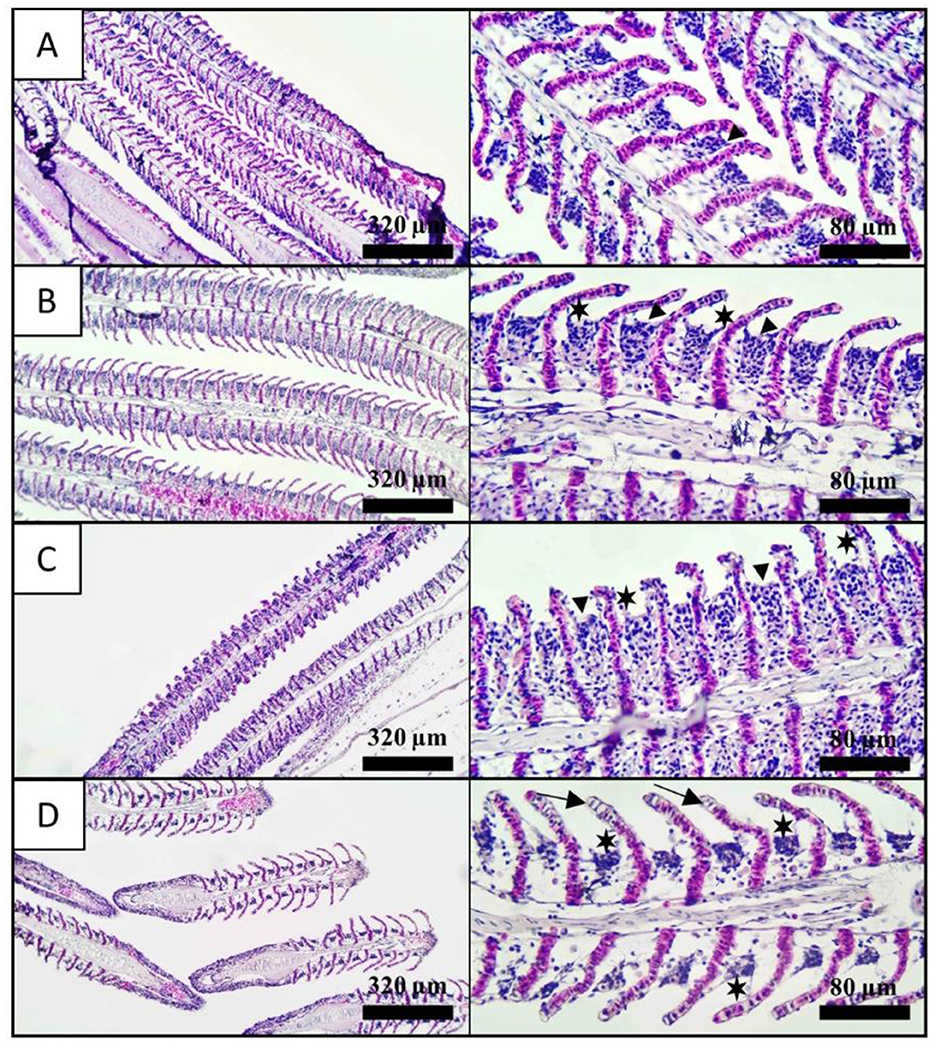
Figure 3. Histological observation of gill tissues exposed nutmeg essential oil with different concentrations. (A) Control, (B) 800 μL/L, (C) 1,200 μL/L, and (D) 1,400 μL/L. Arrowhead: hyperplasia, star: epithelial lifting, and arrow: necrosis. Left side and right side in the figure represents 10X and 40X magnification, respectively.
3.4 DNA damage
The potential genotoxic effects of the anesthetic agent were evaluated using the alkaline comet assay method (Figure 4). No DNA migration was observed in the control, LC, and MC groups, whereas DNA damage was notably observed in the blood cells of the HC group. Similar to the positive control treated with H2O2, the HC group exhibited significantly higher tail DNA%, olive moment, tail length, and tail moment (p < 0.01) (Table 4).
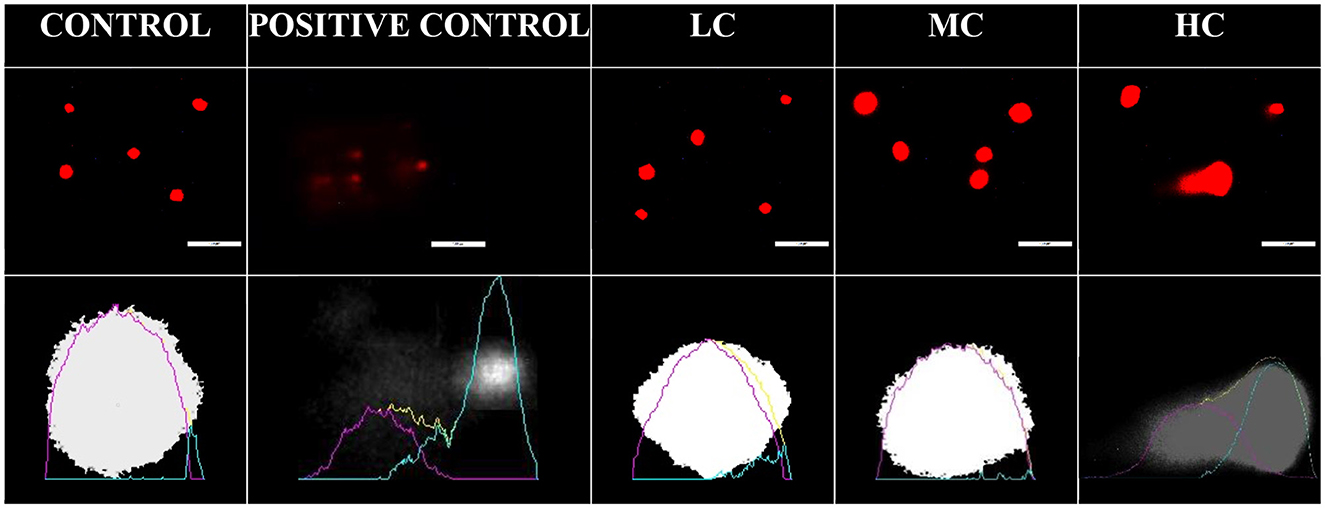
Figure 4. Blood cell DNA migration from control and anesthesia groups. LC, 800 μL/L; MC, 1,200 μL/L; HC, 1,400 μL/L. Positive control was treated with H2O2 solution.
3.5 Multi-criteria decision model
According to all physiological responses of the fish, anesthesia cost, and induction/recovery times, the optimal anesthesia concentration was determined based on the PROMETHEE decision model (Table 5). For common carp, the most suitable anesthesia concentration is 800 μL/L, followed by 1,200 and 1,400 μL/L. According to the distribution of the criteria affecting the ranking, C2, C3, C4, and C8 are the criteria that make LC the preferred concentration (Figure 5). However, C1 in the LC group, which represents induction time, is not at acceptable levels in terms of the applicability of the anesthesia.
4 Discussion
For an anesthetic agent to be considered applicable, the main factors are that it should not be toxic to animals, should not adversely affect human health during the procedure, and should have suitable induction and recovery times (33). The chemical composition of the essential oil should be investigated, and the anesthetic effects and toxicity of these components must be determined. In the present study, sabinene, which constitutes the largest fraction, is frequently used in the pharmaceutical industry and exhibits antifungal (34) and anti-inflammatory effects (35). On the other hand, α-Pinene and β-Pinene, which are part of the chemical composition of nutmeg essential oil, have been shown to be in essential oil of various plants and produce anesthetic effects in numerous studies (36–41). These components, which bind to the benzodiazepine site of the GABAA receptor complexes in the postsynaptic neurons of the central nervous system, can induce anesthesia in fish via inhibitory postsynaptic potential (IPSP) (42–44). Exposure duration and the concentration of the anesthetic agent can cause stress in the fish and lead to high operational costs (45). Induction time and recovery time are crucial for both minimizing stress on the fish and expediting the process. Past experiences have indicated that induction time should be under 3 min, and recovery time should be under 5 min (46). In the present study, the LC concentration increased the induction time to over 3 min and produced a sedative effect in the fish. In addition, the fish in the MC group were induced and recovered within the appropriate time range. Although the HC group exhibited a rapid induction time, the recovery time occasionally extended to 5 min. Statistical analysis indicates that the MC group is more suitable for both induction and recovery compared to the other groups with longer times. Induction and recovery times may vary depending on the fish species and trophic level (47, 48). In our previous study with Danube sturgeon, it was demonstrated that chamomile oil at lower concentrations induced and recovered the fish in similar time range (7). The use of nutmeg in powder form, as opposed to essential oil, resulted in significantly longer induction and recovery times for common carp (21). This phenomenon can be attributed to the more complete and rapid transfer of anesthetic components from the essential oil into the water. Although a concentration of 300 μL/L of nutmeg seed oil is considered the most suitable for fish transfer, the induction times were still several times higher than acceptable levels (49).
Hematological parameters are among the most rapid indicators of fish response to toxic substances (50). An improperly adjusted concentration of an anesthetic agent can have toxic effects (51). Consequently, fish exposed to the anesthetic may exhibit changes in blood parameters. In the present study, blood parameters were examined over time, showing a continuous increase in WBC, RBC, HGB, and HCT levels during the first 6 h, and returned to near control levels by the eighth hour. This suggests that with a longer observation period, the levels would fully return to control values. Additionally, opercular movement in anesthetized fish slows down (52). This leads to hypoxia, which, in turn, results in elevated catecholamine levels and, ultimately, higher release of RBC, HGB, and HCT from the spleen (53). The results show a similar pattern of HGB, HCT, and RBC in all exposed groups which suggests stress and likely hypoxia caused by gill irritations (epithelial lifting). In aquaculture, the most commonly used natural anesthetic, clove oil, similarly increased blood parameters in fish during the acute phase, with levels returning to normal in subsequent periods (7, 54–57).
Gills are the first organ exposed to water in fish (58). Therefore, although the histological examination of gills may not be fully sufficient to assess the complete stress response of fish in anesthesia studies, it can still be considered an important stress indicator (59, 60). In the current study, hyperplasia was observed in the gill tissues of fish, including the control group, though with lower severity compared to the anesthetized groups. On the other hand, epithelial lifting signs were only seen in the gills of fish subjected to anesthesia. Additionally, necrotic cells were prominently present in the gills of the LC group. Ultimately, as the concentration of the anesthetic increased, the histological changes in the fish also became more pronounced. Hyperplasia and hypertrophy in gill tissues are non-specific responses commonly exhibited by fish to acute and chronic damage (61). These symptoms are generally considered an initial defense mechanism and are classified as mildly reparable (62). In fact, these initial defense responses can be more pronounced in fish anesthetized with clove oil compared to those treated with the synthetic anesthetic agent MS222 (63). Similarly, the use of natural anesthetic agents has been previously reported to induce hyperplasia and epithelial lifting in fish (10, 64).
The alkaline comet assay test is a reliable method for providing information on the genotoxicity of the relevant substance, based on the ICH S2(R1) guideline (65). DNA strand breakage in fish occurs both in vivo and in vitro in laboratory settings, and it reflects the cells' metabolic activation capacity (66). In the present study, DNA migration was not observed in fish from the control, LC, and MC groups. On the other hand, in the HC group, DNA migration was significantly observed, similar to the positive control. A previously published study has reported conflicting results regarding essential oils and DNA migration in fish, with some studies indicating that essential oils do not induce DNA migration (67), while others have reported that they do (68, 69). Although essential oils are safe at low concentrations, they can exhibit toxic effects when used at high concentrations (51). In previous studies, inconsistencies in the genotoxic effects of essential oils were directly related to the concentrations used. For example, the mutagenic effect of clove oil is dependent on its concentration (70). Clove oil can act as either an antioxidant or a pro-oxidant agent, depending on its concentration, thus potentially serving as a geno-protective agent (71, 72). Eugenol, which is also found in nutmeg oil, has been reported to be genotoxic in various eukaryotic cells, including human cells (73). Although the exact mechanism is not fully understood (68), this genotoxicity has been partially attributed to the oxidative stress induced by eugenol (74). Most essential oils are cytotoxic without being mutagenic and generally do not pose long-term genotoxic risks. Therefore, many of them are reported to be non-carcinogenic and even to enhance apoptosis of tumor cells (75).
The PROMETHEE method, a commonly used multi-criteria decision-making model across various fields, is designed to provide the best option among conflicting criteria (76). Considering the effectiveness of different concentrations of nutmeg essential oil on fish, the optimal concentration has been determined. According to the model, the best score was observed in the LC group, followed by the MC and HC groups, respectively. The criteria that make the LC group highly preferable are C2, C3, C4, and C8, which have the highest weights. However, given that the induction time for anesthesia should be <3 min (46) for the applicability of an anesthetic agent, it is more reasonable to consider LC as a sedation concentration rather than an anesthesia concentration. In fish transport, sedative concentrations are generally used to reduce sensitivity to mechanical stimuli, swimming activity, and stress (77–79). The use of essential oils for sedation reduces stress response, physical damage, and ammonia in water (80–82). Therefore, the LC concentration of nutmeg can be used as a sedative concentration that does not induce stress in fish. Among the other concentrations, the MC group is considered suitable for anesthesia due to its induction and recovery times falling within an acceptable range and its more favorable effects on fish physiology. On the other hand, the HC group presents major limiting factors for suitability due to its histological and genotoxic effects. Additionally, the high cost and extended recovery time negatively impact both the economic feasibility and the practicality of using HC.
5 Conclusions
The current study reveals the anesthetic effects of nutmeg essential oil on common carp, a topic previously unexplored. The anesthetic effects of nutmeg essential oil are attributed to α-Pinene and β-Pinene. The induction times for fish in the LC group remained above acceptable levels. As a result of hematological, histological, and genotoxic studies, nutmeg essential oil has emerged as a promising alternative to synthetic anesthetic agents. However, the most concerning aspect of the study is the regions where nutmeg oil is distributed, which contributes to its high cost. Since nutmeg is predominantly a plant found in far east countries, the availability and applicability of this anesthetic agent in countries like India, Pakistan, and Indonesia are of particular importance. In future studies, it is essential to investigate stress parameters such as plasma cortisol and glucose levels to further validate these findings.
Data availability statement
The original contributions presented in the study are included in the article/Supplementary material, further inquiries can be directed to the corresponding author.
Ethics statement
The animal study was approved by Ethical Committee of Recep Tayyip Erdoğan University, Türkiye (Decision No: 2024/05). The study was conducted in accordance with the local legislation and institutional requirements.
Author contributions
MM: Conceptualization, Data curation, Formal analysis, Investigation, Methodology, Validation, Writing – original draft, Writing – review & editing.
Funding
The author(s) declare that financial support was received for the research, authorship, and/or publication of this article. This study was supported by the Research Fund of the Recep Tayyip Erdoğan University (FBA-2024-1645).
Acknowledgments
I would like to thank the scientific and administrative staff at Recep Tayyip Erdoğan University, Rize, Turkey Fisheries Research Center for their valuable assistance. In addition, I would like to thank my mother, Perihan Minaz, for being the visionary behind this idea. This study has been supported by the Recep Tayyip Erdoğan University Development Foundation (Grant Number: 02024009016053).
Conflict of interest
The author declares that the research was conducted in the absence of any commercial or financial relationships that could be construed as a potential conflict of interest.
Publisher's note
All claims expressed in this article are solely those of the authors and do not necessarily represent those of their affiliated organizations, or those of the publisher, the editors and the reviewers. Any product that may be evaluated in this article, or claim that may be made by its manufacturer, is not guaranteed or endorsed by the publisher.
Supplementary material
The Supplementary Material for this article can be found online at: https://www.frontiersin.org/articles/10.3389/fvets.2024.1477357/full#supplementary-material
References
1. Harmon TS. Methods for reducing stressors and maintaining water quality associated with live fish transport in tanks: a review of the basics. Rev Aquac. (2009) 1:58–66. doi: 10.1111/j.1753-5131.2008.01003.x
2. Husen MA, Sharma S. Efficacy of anesthetics for reducing stress in fish during aquaculture practices - a review. Kathmandu Univ J Sci Eng Technol. (2014) 10:104–23. doi: 10.3126/kuset.v10i1.63691
3. Ciji A, Akhtar MS. Stress management in aquaculture: a review of dietary interventions. Rev Aquac. (2021) 13:2190–247. doi: 10.1111/raq.12565
4. Schreck CB, Tort L. The concept of stress in fish. Fish Physiol. (2016) 35:1–34. doi: 10.1016/B978-0-12-802728-8.00001-1
5. Ashley PJ. Fish welfare: current issues in aquaculture. Appl Anim Behav Sci. (2007) 104:199–235. doi: 10.1016/j.applanim.2006.09.001
6. Brønstad A. Good anesthesia practice for fish and other aquatics. Biology. (2022) 11:1355. doi: 10.3390/biology11091355
7. Ak K, Minaz M, Er A, Aslankoç R. The using potential of a new natural anesthetic agent on rainbow trout (Oncorhynchus mykiss): chamomile oil (Matricaria chamomilla). Aquaculture. (2022) 561:738742. doi: 10.1016/j.aquaculture.2022.738742
8. Aydin B, Barbas LAL. Sedative and anesthetic properties of essential oils and their active compounds in fish: a review. Aquaculture. (2020) 520:734999. doi: 10.1016/j.aquaculture.2020.734999
9. Can E, Sümer E. Anesthetic and sedative efficacy of peppermint (Menta piperita) and lavender (Lavandula angustifolia) essential oils in blue dolphin cichlid (Cyrtocara moorii). Turkish J Vet Anim Sci. (2019) 43:334–41. doi: 10.3906/vet-1809-22
10. Yigit NO, Metin S, Didinen BI, Didinen H, Ozmen O. Effect of lavander (Lavandula angustifolia) and laurel (Laurus nobilis) essential oils as anesthesics in rainbow trout (Oncorhynchus mykiss). Aquaculture. (2022) 557:738328. doi: 10.1016/j.aquaculture.2022.738328
11. Yigit NO, Kocaayan H. Efficiency of thyme (Origanum onites) and coriander (Coriandrum sativum) essential oils on anesthesia and histopathology of rainbow trout (Oncorhynchus mykiss). Aquaculture. (2023) 562:738813. doi: 10.1016/j.aquaculture.2022.738813
12. Haihambo W, Gabriel NN, Abasubong KP, Gabriel RN. Alternative fish anaesthetics: perspective on their application in african aquaculture and fisheries research. Emerg Sustain Aquac Innovat Afr. (2023) 543–52. doi: 10.1007/978-981-19-7451-9_26
13. Martins T, Valentim A, Pereira N, Antunes LM. Anaesthetics and analgesics used in adult fish for research: a review. Lab Anim. (2019) 53:325–41. doi: 10.1177/0023677218815199
14. Ashokkumar K, Simal-Gandara J, Murugan M, Dhanya MK, Pandian A. Nutmeg (Myristica fragrans Houtt.) essential oil: a review on its composition. Biological, and pharmacological activities. Phyther Res. (2022) 36:2839–51. doi: 10.1002/ptr.7491
15. Francis SK, James B, Varughese S, Nair MS. Phytochemical investigation on Myristica fragrans stem bark. Nat Prod Res. (2019) 33:1204–8. doi: 10.1080/14786419.2018.1457670
16. Abourashed EA, El-Alfy AT. Chemical diversity and pharmacological significance of the secondary metabolites of nutmeg (Myristica fragrans Houtt.). Phytochem Rev. (2016) 15:1035–56. doi: 10.1007/s11101-016-9469-x
17. Das S, Kumar Singh V, Kumar Dwivedy A, Kumar Chaudhari A, Upadhyay N, Singh A, et al. Assessment of chemically characterised Myristica fragrans essential oil against fungi contaminating stored scented rice and its mode of action as novel aflatoxin inhibitor. Nat Prod Res. (2020) 34:1611–5. doi: 10.1080/14786419.2018.1519826
18. Hiranrat A, Hiranrat W. Myristigranol, a new diarylpropane derivative from the wood of Myristica fragrans. Nat Prod Res. (2019) 33:2958–63. doi: 10.1080/14786419.2018.1512988
19. Nikolic V, Nikolic L, Dinic A, Gajic I, Urosevic M, Stanojevic L, et al. Chemical composition, antioxidant and antimicrobial activity of nutmeg (Myristica fragrans Houtt) seed essential oil. J Essent Oil Bear Plants. (2021) 24:218–27. doi: 10.1080/0972060X.2021.1907230
20. Purkait S, Bhattacharya A, Bag A, Chattopadhyay RR. Antibacterial and antioxidant potential of essential oils of five spices. J Food Qual Hazards Control. (2018) 5:61–71. doi: 10.29252/jfqhc.5.2.6
21. Al-Niaeem KS, Mohammed FA, Al-Hamadany QH. The anaesthetic effect of nutmeg powder, Myrisitca fragrans on young common carp, Cyprinus carpio. Biol Appl Environ Res. (2017) 1:279–86. doi: 10.13140/RG.2.2.35019.28963
22. Grover JK, Khandkar S, Vats V, Dhunnoo Y, Das D. Pharmacological studies on Myristica fragrans- antidiarrheal, hypnotic, analgesic and hemodynamic (blood pressure) parameters. Methods Find Exp Clin Pharmacol. (2002) 24:675–80. doi: 10.1358/mf.2002.24.10.802317
24. Sangalli BC, Chiang W. Toxicology of nutmeg abuse. J Toxicol Clin Toxicol. (2000) 38:671–8. doi: 10.1081/CLT-100102020
25. Leiter E, Hitchcock G, Godwin S, Johnson M, Sedgwick W, McCall S, et al. Evaluation of the anxiolytic properties of Tetrahydropalmatine, a Corydalis yanhusuo compound, in the male Sprague-Dawley rat. J Am Assoc Nurse Anesthesiol. (2011) 79:109–15.
26. Khan MN, Shahzad K, Chatta A, Sohail M, Piria M, Treer T. A review of introduction of common carp Cyprinus carpio in Pakistan: origin, purpose, impact and management. Croat J Fish. (2016) 74:71–80. doi: 10.1515/cjf-2016-0016
27. Rahman MM. Role of common carp (Cyprinus carpio) in aquaculture production systems. Front Life Sci. (2015) 8:399–410. doi: 10.1080/21553769.2015.1045629
28. Iwama GK. Stress in fish. Ann N Y Acad Sci. (1998) 851:304–10. doi: 10.1111/j.1749-6632.1998.tb09005.x
29. Minaz M, Er A, Ak K, Nane ID, Ipek ZZ, Kurtoglu IZ, et al. Short-term exposure to bisphenol A (BPA) as a plastic precursor: hematological and behavioral effects on Oncorhynchus mykiss and Vimba vimba. Water Air Soil Pollut. (2022) 233:1–12. doi: 10.1007/s11270-022-05585-x
30. Kurtoglu IZ, Kayis S, Ak K, Gencoglu S, Duzgun A, Er A. Histopathology of rainbow trout (Oncorhynchus mykiss) and sturgeon (Acipenser baerii) exposed to sublethal concentrations of cement. Fresenius Environ Bull. (2016) 25:3523–7.
31. Er A, Kayiş S. Acute toxicity of pozzolanic cement on two crustacean species, water flea (Daphnia magna) and Gammarus komareki. Bull Environ Contam Toxicol. (2022) 108:309–14. doi: 10.1007/s00128-021-03345-x
32. Demirel YE, Simsek E, Ozturk E, Kitis M. Selection of priority energy efficiency practices for industrial steam boilers by PROMETHEE decision model. Energy Effic. (2021) 14:1–20. doi: 10.1007/s12053-021-10007-8
33. Can E, Kizak V, Seyhaneyildiz Can S, Özçiçek E. Anesthetic potential of geranium (Pelargonium graveolens) oil for two cichlid species, Sciaenochromis fryeri and Labidochromis caeruleus. Aquaculture. (2018) 491:59–64. doi: 10.1016/j.aquaculture.2018.03.013
34. Yamasaki Y, Kunoh H, Yamamoto H, Akimitsu K. Biological roles of monoterpene volatiles derived from rough lemon (Citrus jambhiri Lush) in citrus defense. J Gen Plant Pathol. (2007) 73:168–79. doi: 10.1007/s10327-007-0013-0
35. Valente J, Zuzarte M, Gonçalves MJ, Lopes MC, Cavaleiro C, Salgueiro L, et al. Antifungal, antioxidant and anti-inflammatory activities of Oenanthe crocata L. essential oil. Food Chem Toxicol. (2013) 62:349–54. doi: 10.1016/j.fct.2013.08.083
36. Bodur T, Afonso JM, Montero D, Navarro A. Assessment of effective dose of new herbal anesthetics in two marine aquaculture species: Dicentrarchus labrax and Argyrosomus regius. Aquaculture. (2018) 482:78–82. doi: 10.1016/j.aquaculture.2017.09.029
37. da Cunha MA, de Barros FMC, de Oliveira Garcia L, de Lima Veeck AP, Heinzmann BM, Loro VL, et al. Essential oil of Lippia alba: a new anesthetic for silver catfish, Rhamdia quelen. Aquaculture. (2010) 306:403–6. doi: 10.1016/j.aquaculture.2010.06.014
38. Kasanen JP, Pasanen AL, Pasanen P, Liesivuori J, Kosma VM, Alarie Y. Stereospecificity of the sensory irritation receptor for nonreactive chemicals illustrated by pinene enantiomers. Arch Toxicol. (1998) 72:514–23. doi: 10.1007/s002040050536
39. Mercier B, Prost J, Prost M. The essential oil of turpentine and its major volatile fraction (alpha- and beta-pinenes): a review. Int J Occup Med Environ Health. (2009) 22:331–42. doi: 10.2478/v10001-009-0032-5
40. Raknak K, Rattanarom P, Praphairaksit N. Transformation of mugwort (Artemisia vulgaris) oil into nanoemulsion as an ethanol-free herbal anesthetic for surgery on koi carp (Cyprinus carpio). Aquac Int. (2022) 30:409–18. doi: 10.1007/s10499-021-00808-0
41. Tondolo JSM, De Amaral LP, Simões LN, Garlet QI, Schindler B, Oliveira TM, et al. Anesthesia and transport of fat snook centropomus parallelus with the essential oil of nectandra megapotamica (Spreng.). Mez Neotrop Ichthyol. (2013) 11:667–74. doi: 10.1590/S1679-62252013000300020
42. Aoshima H, Hamamoto K. Potentiation of GABAA receptors expressed in xenopus oocytes by perfume and phytoncid. Biosci Biotechnol Biochem. (1999) 63:743–8. doi: 10.1271/bbb.63.743
43. Kessler A, Sahin-Nadeem H, Lummis SCR, Weigel I, Pischetsrieder M, Buettner A, et al. GABAA receptor modulation by terpenoids from Sideritis extracts. Mol Nutr Food Res. (2014) 58:851–62. doi: 10.1002/mnfr.201300420
44. Khumpirapang N, Chaichit S, Jiranusornkul S, Pikulkaew S, Müllertz A, Okonogi S. In vivo anesthetic effect and mechanism of action of active compounds from Alpinia galanga oil on Cyprinus carpio (koi carp). Aquaculture. (2018) 496:176–84. doi: 10.1016/j.aquaculture.2018.07.017
45. Feng G, Zhuang P, Zhang L, Kynard B, Shi X, Duan M, et al. Effect of anaesthetics MS-222 and clove oil on blood biochemical parameters of juvenile Siberian sturgeon (Acipenser baerii). J Appl Ichthyol. (2011) 27:595–9. doi: 10.1111/j.1439-0426.2011.01711.x
46. Ross LG, Ross B. Anaesthetic and Sedative Techniques for Aquatic Animals. Ames, IO: Blackwell Publishing (2008).
47. Mirghaed AT, Yasari M, Mirzargar SS, Hoseini SM. Rainbow trout (Oncorhynchus mykiss) anesthesia with myrcene: efficacy and physiological responses in comparison with eugenol. Fish Physiol Biochem. (2018) 44:919–26. doi: 10.1007/s10695-018-0481-5
48. Yousefi M, Hoseini SM, Aydin B, Taheri Mirghaed A, Kulikov EV, Drukovsky SG, et al. Anesthetic efficacy and hemato-biochemical effects of thymol on juvenile Nile tilapia, Oreochromis niloticus. Aquaculture. (2022) 547:737540. doi: 10.1016/j.aquaculture.2021.737540
49. Lili W, Wahyudin MF, Suryana AAH, Haetami K. The used of natural anesthetic materials nutmeg seed oil in the transportation of Sumatran Fish Fingerlings (Puntius tetrazona). Asian J Fish Aquat Res. (2021) 15:1–10. doi: 10.9734/ajfar/2021/v15i430333
50. Akram R, Iqbal R, Hussain R, Ali M. Effects of bisphenol a on hematological, serum biochemical, and histopathological biomarkers in bighead carp (Aristichthys nobilis) under long-term exposure. Environ Sci Pollut Res (2021) 1:1–16. doi: 10.21203/rs.3.rs-186716/v1
51. Sinha S, Jothiramajayam M, Ghosh M, Mukherjee A. Evaluation of toxicity of essential oils palmarosa, citronella, lemongrass and vetiver in human lymphocytes. Food Chem Toxicol. (2014) 68:71–7. doi: 10.1016/j.fct.2014.02.036
52. Cooke SJ, Suski CD, Ostrand KG, Tufts BL, Wahl DH. Behavioral and physiological assessment of low concentrations of clove oil anaesthetic for handling and transporting largemouth bass (Micropterus salmoides). Aquaculture. (2004) 239:509–29. doi: 10.1016/j.aquaculture.2004.06.028
53. Clauss TM, Dove ADM, Arnold JE. Hematologic disorders of fish. Vet Clin North Am Exot Anim Pract. (2008) 11:445–62. doi: 10.1016/j.cvex.2008.03.007
54. Gholipour Kanani H, Mirzargar SS, Soltani M, Ahmadi M, Abrishamifar A, Bahonar A, et al. Anesthetic effect of tricaine methanesulfonate, clove oil and electroanesthesia on lysozyme activity of Oncorhynchus mykiss. Iran J Fish Sci. (2011) 10:393–402.
55. Mohammadi M, Khara H. Effect of different anesthetic agents (clove oil, tricaine methanesulfonate, ketamine, tobacco) on hematological parameters and stress indicators of rainbow trout Oncorhynchus mykiss, Walbaum, 1792. Comp Clin Path. (2015) 24:1039–44. doi: 10.1007/s00580-014-2027-2
56. Uçar A, Atamanalp M. The effects of natural (clove oil) and synthetical (2-phenoxyethanol) anesthesia substances on hematology parameters of rainbow trout (Oncorhynchus mykiss) and brown trout (Salmo trutta fario). J Anim Vet Adv. (2010) 9:1925–33. doi: 10.3923/javaa.2010.1925.1933
57. Velísek J, Svobodova Z, Piackova V, Groch L, Nepejchalova L. Effects of clove oil anaesthesia on common carp (Cyprinus carpio L.). Vet Med. (2005) 50:269–75. doi: 10.17221/5623-VETMED
58. Nilsson GE. Gill remodeling in fish – a new fashion or an ancient secret? J Exp Biol. (2007) 210:2403–9. doi: 10.1242/jeb.000281
59. Bodur T, Oktavia IS, Sulmartiwi L. Effective concentration of herbal anaesthetics Origanum vulgare L. oil and its effects on stress parameters in Nile tilapia (Oreochromis niloticus). Vet Med Sci. (2024) 10:e1492. doi: 10.1002/vms3.1492
60. Bodur T, León-Bernabeu S, Navarro A, Tort L, Afonso JM, Montero D. Effects of new plant based anesthetics Origanum sp. and Eucalyptus sp oils on stress and welfare parameters in Dicentrarchus labrax and their comparison with clove oil. Aquaculture. (2018) 495:402–8. doi: 10.1016/j.aquaculture.2018.06.021
61. de Oliveira CPB, Lemos CHP, Felix e Silva A, de Souza SA, Albinati ACL, Lima AO, et al. Use of eugenol for the anaesthesia and transportation of freshwater angelfish (Pterophyllum scalare). Aquaculture. (2019) 513:734409. doi: 10.1016/j.aquaculture.2019.734409
62. Raskovic B, Poleksic V, Zivic I, Spasic M. Histology of carp (Cyprinus carpio L) gills and pond water quality in semiintensive production. Bulg J Agric Sci. (2010) 16:253–62.
63. Jia Y, Xie T, Gao Y, Qin H, Guan C. Anesthetics efficacy and physiological response of MS222 and clove oil in spotted knifejaw Oplegnathus punctatus. Aquac Reports. (2022) 25:101201. doi: 10.1016/j.aqrep.2022.101201
64. Brandão FR, Farias CFS, de Melo Souza DC, de Oliveira MIB, de Matos LV, Majolo C, et al. Anesthetic potential of the essential oils of Aloysia triphylla, Lippia sidoides and Mentha piperita for Colossoma macropomum. Aquaculture. (2021) 534:736275. doi: 10.1016/j.aquaculture.2020.736275
65. Frötschl R. Experiences with the in vivo and in vitro comet assay in regulatory testing. Mutagenesis. (2015) 30:51–7. doi: 10.1093/mutage/geu069
66. Mitchelmore CL, Chipman JK. DNA strand breakage in aquatic organisms and the potential value of the comet assay in environmental monitoring. Mutat Res Fundam Mol Mech Mutagen. (1998) 399:135–47. doi: 10.1016/S0027-5107(97)00252-2
67. Kampke EH, de Souza Barroso ME, Marques FM, Fronza M, Scherer R, Lemos MF, et al. Genotoxic effect of Lippia alba (Mill.) N. E. Brown essential oil on fish (Oreochromis niloticus) and mammal (Mus musculus). Environ Toxicol Pharmacol. (2018) 59:163–71. doi: 10.1016/j.etap.2018.03.016
68. Golomazou E, Malandrakis EE, Kavouras M, Karatzinos T, Miliou H, Exadactylos A, et al. Anaesthetic and genotoxic effect of medicinal plant extracts in gilthead seabream (Sparus aurata L.). Aquaculture. (2016) 464:673–82. doi: 10.1016/j.aquaculture.2016.08.017
69. Nascimento HS, Crispim BA, Francisco LFV, Merey FM, Kummrow F, Viana LF, I et al. Genotoxicity evaluation of three anesthetics commonly employed in aquaculture using Oreochromis niloticus and Astyanax lacustris. Aquac Rep. (2020) 17:100357. doi: 10.1016/j.aqrep.2020.100357
70. Shoeibi S, Rahimifard N, Pirouz B, Yalfani R, Pakzad S, Mirab Samiee S, et al. Mutagenicity of four natural flavors: clove, cinnamon, thyme and Zataria multiflora Boiss. J Med Plants. (2009) 8:89–96.
71. Atsumi T, Murakami Y, Shibuya K, Tonosaki K, Fujisawa S. Induction of cytotoxicity and apoptosis and inhibition of cyclooxygenase-2 gene expression, by curcumin and its analog, α-diisoeugenol. Anticancer Res. (2005) 25:4029–36.
72. Miyazawa M, Hisama M. Antimutagenic activity of phenylpropanoids from clove (Syzygium aromaticum). J Agric Food Chem. (2003) 51:6413–22. doi: 10.1021/jf030247q
73. Cortés-Rojas DF, de Souza CRF, Oliveira WP. Clove (Syzygium aromaticum): a precious spice. Asian Pac J Trop Biomed. (2014) 4:90–6. doi: 10.1016/S2221-1691(14)60215-X
74. Martins C, Rueff J, Rodrigues AS. Genotoxic alkenylbenzene flavourings, a contribution to risk assessment. Food Chem Toxicol. (2018) 118:861–79. doi: 10.1016/j.fct.2018.06.020
75. Bakkali F, Averbeck S, Averbeck D, Idaomar M. Biological effects of essential oils – a review. Food Chem Toxicol. (2008) 46:446–75. doi: 10.1016/j.fct.2007.09.106
76. De Smet Y, Mareschal B, Verly C. Extending the PROMETHEE II method to continuous and combinatorial multi-objective optimization problems: a first model. in IEEM 2009 - IEEE International Conference on Industrial Engineering and Engineering Management. Hong Kong: IEEE (2009). p. 1608–11.
77. Mirghaed TA, Ghelichpour M, Hoseini SM. Myrcene and linalool as new anesthetic and sedative agents in common carp, Cyprinus carpio- Comparison with eugenol. Aquaculture. (2016) 464:165–70. doi: 10.1016/j.aquaculture.2016.06.028
78. Souza DFC, Baldissera MD, Baldisserotto B, Heinzmann BM, Martos-Sitcha JA, Mancera JM. Essential oils as stress-reducing agents for fish aquaculture: a review. Front Physiol. (2019) 10:785. doi: 10.3389/fphys.2019.00785
79. Teixeira RR, de Souza RC, Sena AC, Baldisserotto B, Heinzmann BM, Couto RD, et al. Essential oil of Aloysia triphylla in Nile tilapia: anaesthesia, stress parameters and sensory evaluation of fillets. Aquac Res. (2017) 48:3383–92. doi: 10.1111/are.13165
80. Becker AG, Luz RK, Mattioli CC, Nakayama CL, de Souza e Silva W, de Oliveira Paes Leme F, et al. Can the essential oil of Aloysia triphylla have anesthetic effect and improve the physiological parameters of the carnivorous freshwater catfish Lophiosilurus alexandri after transport? Aquaculture. (2017) 481:184–90. doi: 10.1016/j.aquaculture.2017.09.007
81. Cupp AR, Hartleb CF, Fredricks KT, Gaikowski MP. Effectiveness of eugenol sedation to reduce the metabolic rates of cool and warm water fish at high loading densities. Aquac Res. (2016) 47:234–42. doi: 10.1111/are.12485
82. Hohlenwerger JC, Baldisserotto B, Couto RD, Heinzmann BM, da Silva DT, Caron BO, et al. Essential oil of Lippia alba in the transport of Nile tilapia. Ciência Rural. (2016) 47:e20160040. doi: 10.1590/0103-8478cr20160040
Keywords: DNA damage, fish, hematology, herbal anesthetic agent, histology
Citation: Minaz M (2024) A new herbal anesthetic agent for common carp (Cyprinus carpio) sedation and anesthesia: nutmeg (Myristica fragrans) essential oil. Front. Vet. Sci. 11:1477357. doi: 10.3389/fvets.2024.1477357
Received: 07 August 2024; Accepted: 30 September 2024;
Published: 14 October 2024.
Edited by:
Luis Manuel Félix, Universidade de Trás-os-Montes e Alto Douro, PortugalReviewed by:
Türker Bodur, Akdeniz University, TürkiyeMalgorzata Witeska, University of Natural Sciences and Humanities of Siedlce, Poland
Copyright © 2024 Minaz. This is an open-access article distributed under the terms of the Creative Commons Attribution License (CC BY). The use, distribution or reproduction in other forums is permitted, provided the original author(s) and the copyright owner(s) are credited and that the original publication in this journal is cited, in accordance with accepted academic practice. No use, distribution or reproduction is permitted which does not comply with these terms.
*Correspondence: Mert Minaz, mert.minaz@erdogan.edu.tr