- 1Jiangsu Agri-Animal Husbandry Vocational College, Taizhou, China
- 2Institute of Veterinary Medicine, Jiangsu Academy of Agricultural Sciences, Nanjing, China
Bovine respiratory disease complex (BRDC) represents a global acute respiratory condition that imposes substantial economic burdens on the cattle industry due to its high morbidity and mortality rates. Various factors contribute to the development of BRDC, including pathogen infections, environmental stresses, weaning of calves, and herd relocation. Viral pathogens, notably bovine respiratory syncytial virus (BRSV) and bovine viral diarrhea virus (BVDV), play a critical role in the etiology of BRDC, with single or combined viral infections being particularly clinically significant. In this study, we developed a duplex TaqMan-based real-time RT-PCR assay targeting the conserved regions of the F gene of BRSV and the 5′ UTR sequence of BVDV. The limits of detection for BRSV and BVDV were 6.83 copies/μL and 5.24 copies/μL, respectively. Our validation data suggest the assay has excellent sensitivity, specificity and reproducibility. Testing of clinical samples revealed prevalence of BRSV and BVDV in local farms in Jiangsu Province, China. This study provides an efficient diagnostic tool for the epidemiological investigation of BRDC.
1 Introduction
Bovine respiratory disease complex (BRDC) is a systemic respiratory ailment in cattle, characterized by symptoms such as fever, nasal discharge, lethargy, dyspnea, and coughing (1). As a multifactorial and multi-etiologic syndrome, BRDC can be exacerbated by complex interactions among host factors, pathogen exposure, and environmental factors (2). Environmental conditions like tough weather, weaning, transportation, and overcrowding negatively impact both immune and non-immune defense mechanisms in cattle (3, 4). These circumstances can lead to an overall suppression of the host’s immune system, facilitating bacterial colonization in the lower respiratory tract, particularly when viral infections are present (5).
Currently, several viral pathogens, including BRSV, BVDV, IBR, bovine coronavirus (BCoV), and bovine parainfluenza virus type 3 (BPIV-3), have been identified as key contributors to the development of BRDC, with single or combined viral infections being particularly clinically significant (6, 7). Among these, BRSV and BVDV are of particular concern due to their high prevalence and significant role in causing severe respiratory illness (7–10). In China, the epidemiological situation for BRSV and BVDV underscores the importance of accurate and timely diagnosis, given that these viruses are endemic and contribute to substantial economic losses within the livestock industry (7–10).
Early and efficient diagnosis of BRSV and BVDV is crucial for controlling the spread of BRDC and mitigating economic impacts. Traditional diagnostic methods, such as virus isolation, enzyme-linked immunosorbent assay (ELISA), hemagglutination inhibition test, serum neutralization test, and SYBR Green PCR, while valuable, have limitations in terms of sensitivity, specificity, and operational complexity (11). The fluorescent quantitative PCR (qPCR) assay, on the other hand, offers a robust alternative with its ease of operation, superior sensitivity and specificity, and the capability for multiplex detection. These features make qPCR especially advantageous for rapid and accurate identification of multiple pathogens in a single reaction, thereby streamlining the diagnostic process and enabling more effective management of BRDC outbreaks.
2 Materials and methods
2.1 Viral strains and nucleic acid
Viral nucleic acids of BCoV (GenBank No. MZ603735), BPIV3 (GenBank No. MH552577), bovine rotavirus (BoRV, GenBank No. JN790188), bovine astrovirus (BoAstV, GenBank No. NC_024297), and BVDV (GenBank No. KF501393), were kept by the Institute of Veterinary Medicine, Jiangsu Academy of Agricultural Sciences.
2.2 Reagents and instruments
Nucleic acid extraction was conducted using the AxyPrep Body Fluid Viral DNA/RNA Miniprep Kit (AxyGen, Union City, CA, United States). RT-PCR was performed using One Step PrimeScript™ RT-PCR Kit (Takara Bio, Beijing, China). Primers and probes were diluted in TE buffer (Solarbio, Beijing, China). PCR amplification was carried out on an ABI StepOne Plus™ Real-Time PCR System (Thermo Fisher Scientific, Waltham, MA, United States).
2.3 Primers and probes
All available sequences of BRSV and BVDV were retrieved from GenBank for alignment using DNAMAN. Highly conserved regions from the BRSV gF gene and the BVDV 5′ UTR sequence were selected for primer and probe design (Beacon Designer 7.0 software). Sequences of primers and probes are listed in Table 1.
2.4 Nucleic acid extraction
Nucleic acid extraction from cell cultures or clinical samples was performed using the Axygen Body Fluid Viral DNA/RNA Miniprep Kit, following the manufacturer’s protocol. In summary, 1 mL of 1x PBS was added to a microcentrifuge tube containing either a nasal swab or 0.5 g of a fecal sample and thoroughly mixed. The mixture was then centrifuged at 3,000 rpm for 3 min, after which 200 μL of the supernatant was used for the extraction process. This supernatant was combined with an equal volume of lysis buffer and incubated at room temperature for 5 min. Subsequently, 75 μL of V-N buffer was added to the mixture, which was then subjected to another round of centrifugation to collect the supernatant for nucleic acid isolation. The purified nucleic acid was finally eluted in 50 μL of elution buffer and stored at −80°C for subsequent analyses.
2.5 Standard plasmid construction
Recombinant plasmids pUC57-BRSV-F and pUC57-BVDV-5′ UTR, which contain the target fragments of BRSV and BVDV respectively, were synthesized as standard plasmids by General Biosystems Corp. Ltd. (Anhui, China).
2.6 Optimization of the singleplex RT-PCR assay
The reaction system was set up as recommended by the One Step PrimeScript™ RT-PCR Kit manual: 10 μL of 2 × One-Step RT-PCR buffer III, 0.4 μL of Ex Taq HS (5 U/μL), 0.4 μL of PrimeScript RT Enzyme Mix II, 0.8 μL of each primer set (10 μM), 0.8 μL of probe (10 μM), 0.4 μL of ROX (50×), 2 μL of nucleic acid template, and 5.2 μL of RNase free water. The total volume for each reaction is 20 μL. The reaction parameters are as follows: reverse transcription reaction: 42°C for 5 min, followed by 95°C for 10 s, 1 cycle; PCR program: 95°C for 5 s, 60°C for 30 s, 40 cycles; fluorescence signals were captured during annealing process. Six gradients of annealing temperature were set for optimization (50°C, 52°C, 54°C, 56°C, 58°C, 60°C).
2.7 Optimization of the duplex RT-PCR assay
In comparison with the singleplex RT-PCR, working concentrations for primers and probes were further optimized using the matrix method to screen for the optimal reaction system.
2.8 Specificity test of the duplex RT-PCR assay
To assess assay specificity, in silico analysis was performed with an online tool named Primer-BLAST.1 Furthermore, nucleic acids of BCoV, BPIV3, BoRV, BoAstV, BVDV viral strains were extracted and tested with the duplex RT-PCR assay.
2.9 Sensitivity test of the duplex RT-PCR assay
To assess assay sensitivity, 10-fold serial dilutions of 1:1 mixture of standard plasmids containing BRSV and BVDV targets were carefully tested in triplicate. The copy number for each dilution was calculated with an online software based on plasmid size and nucleic acid concentration.2 Limit of detection (LOD) as well as PCR amplification efficiency were determined based on standard curves generated.
2.10 Repeatability test of the duplex RT-PCR assay
To verify repeatability and reproducibility of the established duplex RT-PCR assay, both intra-assay and inter-assay tests were performed. Plasmid mixtures with three different concentrations (1.11 × 104 copies/μL, 1.11 × 105 copies/μL, and 1.11 × 106 copies/μL) were prepared as samples. For the intra-assay, each sample was tested in triplicate. For the inter-assay, triplicate tests for all three samples were performed on three different dates. The coefficient of variation (CV) was calculated for each set of triplicates by dividing the standard deviation (SD) by the mean of the measurements, and then multiplying by 100 to express it as a percentage. Statistical analysis included the use of an analysis of variance (ANOVA) to assess the significance of any observed variations among the replicates and across different testing days.
2.11 Clinical samples collection
Twenty-three bovine fecal samples and 50 bovine nasal swabs were randomly collected from pastures and dairy farms in Jiangsu Province, respectively. All samples were preserved by the Institute of Veterinary Medicine, Jiangsu Academy of Agricultural Sciences. All clinical samples were tested using both the duplex and singleplex RT-PCR assays established in this study.
3 Results
3.1 Development and optimization of the duplex real-time RT-PCR assay
The optimized annealing temperature for singleplex real-time PCR is 50°C. After optimization with the matrix method, the final concentrations of primers and probes are 0.6 μmol/L and 0.4 μmol/L for BRSV, 0.2 μmol/L and 0.2 μmol/L for BVDV, respectively (Table 2). The optimized reaction procedure was as follows: reverse transcription reaction: 42°C for 5 min, followed by 95°C for 10 s, 1 cycle; PCR program: 95°C for 5 s, 50°C for 30 s (annealing), 40 cycles, with fluorescence signals collected during the annealing step.
The judgement criteria are as follows: the test is valid only when both the negative and positive controls work. If the sample has no fluorescence amplification curve, it is considered negative; if the sample has a Ct value less than 35 with a fluorescence amplification curve, it is considered positive; if the sample has a Ct value between 35 and 40 with a fluorescence amplification curve, it is considered suspect and needs to be re-extracted and re-tested for confirmation. If the Ct value is again between 35 and 40 with an amplification curve, the sample is considered positive.
3.2 Sensitivity and standard curves of the duplex real-time RT-PCR assay
To assess the sensitivity of the assay, 10-fold serial dilutions of 1:1 mixture of standard plasmids containing BRSV and BVDV targets (ranging from 6.83 × 108 to 6.83 × 100 copies/μL and from 5.24 × 108 to 5.24 × 100 copies/μL for BRSV and BVDV, respectively) were carefully tested in triplicate. The standard curves for both singleplex and duplex assays was generated by plotting the Ct values against logarithm of the template quantities (Figure 1). PCR amplification efficiencies were all between 90 and 100.30% (90.02, 93.17% for BRSV singleplex and duplex assays, respectively, 94.71, 100.30% for BVDV singleplex and duplex assays, respectively). At the same time, correlation coefficients (R2) were all above 0.999 (1, 0.9992 for BRSV singleplex and duplex assays, respectively, 0.9996, 0.9993 for BVDV singleplex and duplex assays, respectively). These data indicate no inhibition of PCR sensitivity in the multiplex assay compared to the singleplex assays. The limit of detection for both BRSV and BVDV targets was less than 10 copies (Table 3).
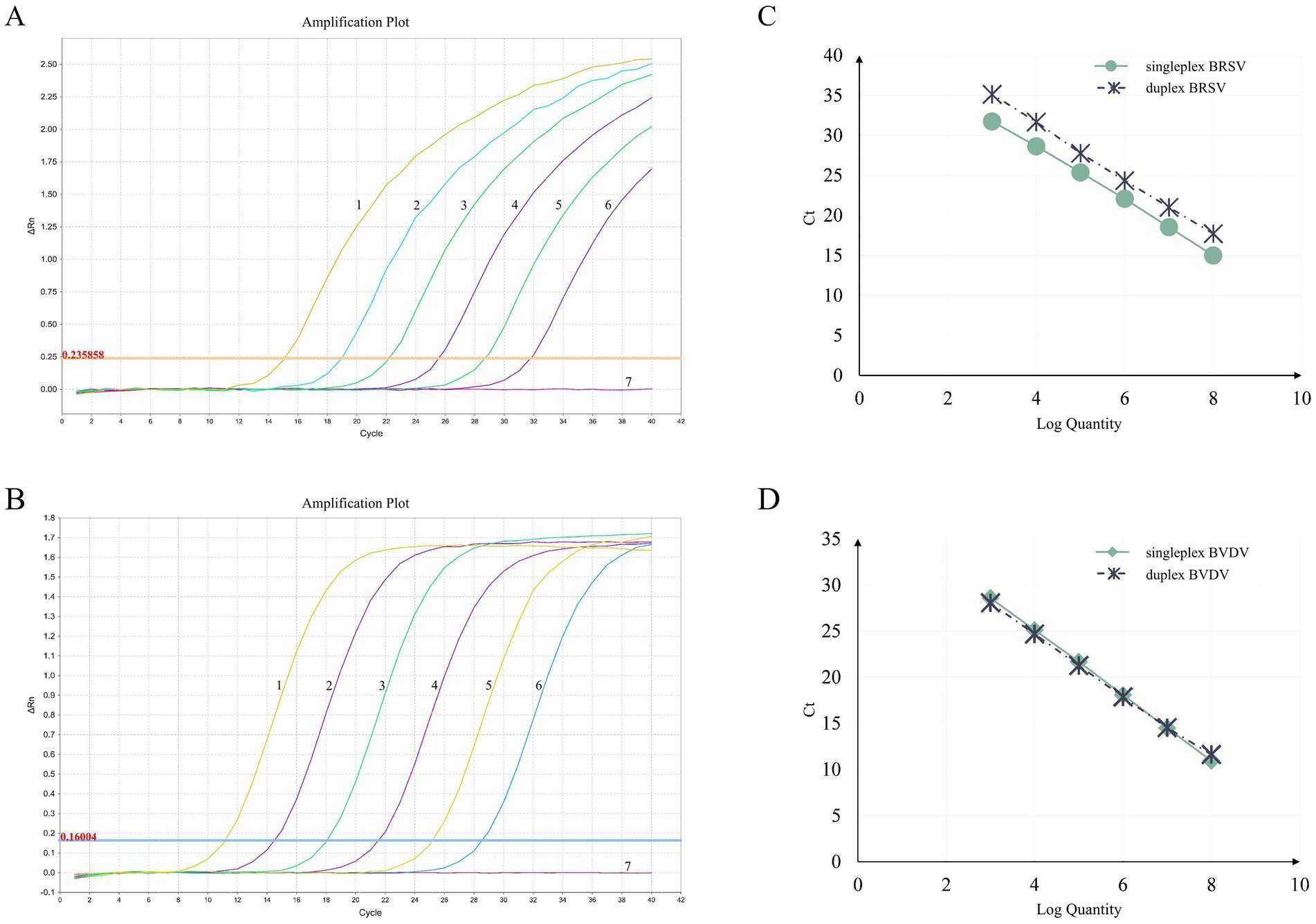
Figure 1. Amplification curves and standard curves of the duplex RT-PCR assay. The amplification curves for BRSV (A) and BVDV (B) were generated using recombinant standard plasmids pUC57-BRSV-F and pUC57-BVDV-5′ UTR, respectively. The standard curves (C,D) were generated from the amplification curves. In (A,B), the plasmid concentrations for curves 1 to 6 ranged from 6.83 × 108 to 6.83 × 103 copies/μL and from 5.24 × 108 to 5.24 × 103 copies/μL for BRSV and BVDV, respectively. Curve 7 represents the negative control.
3.3 Specificity of the duplex real-time RT-PCR assay
The specificity of the primers and probes was first assessed by in silico analysis using the NCBI primer-BLAST function, which determined a unique target for each set of the assay. Subsequently, the assay specificity was evaluated using specific viral targets (standard plasmids containing BRSV or BVDV, and nucleic acid of a BVDV viral strain) and non-target viral pathogens (nucleic acids of multiple bovine viral strains, including BCoV, BPIV3, BoRV and BoAstV). The assay specifically detected BRSV and BVDV while no positive signals were generated from any of the other non-target pathogens, indicating good specificity of the assay (Figure 2).
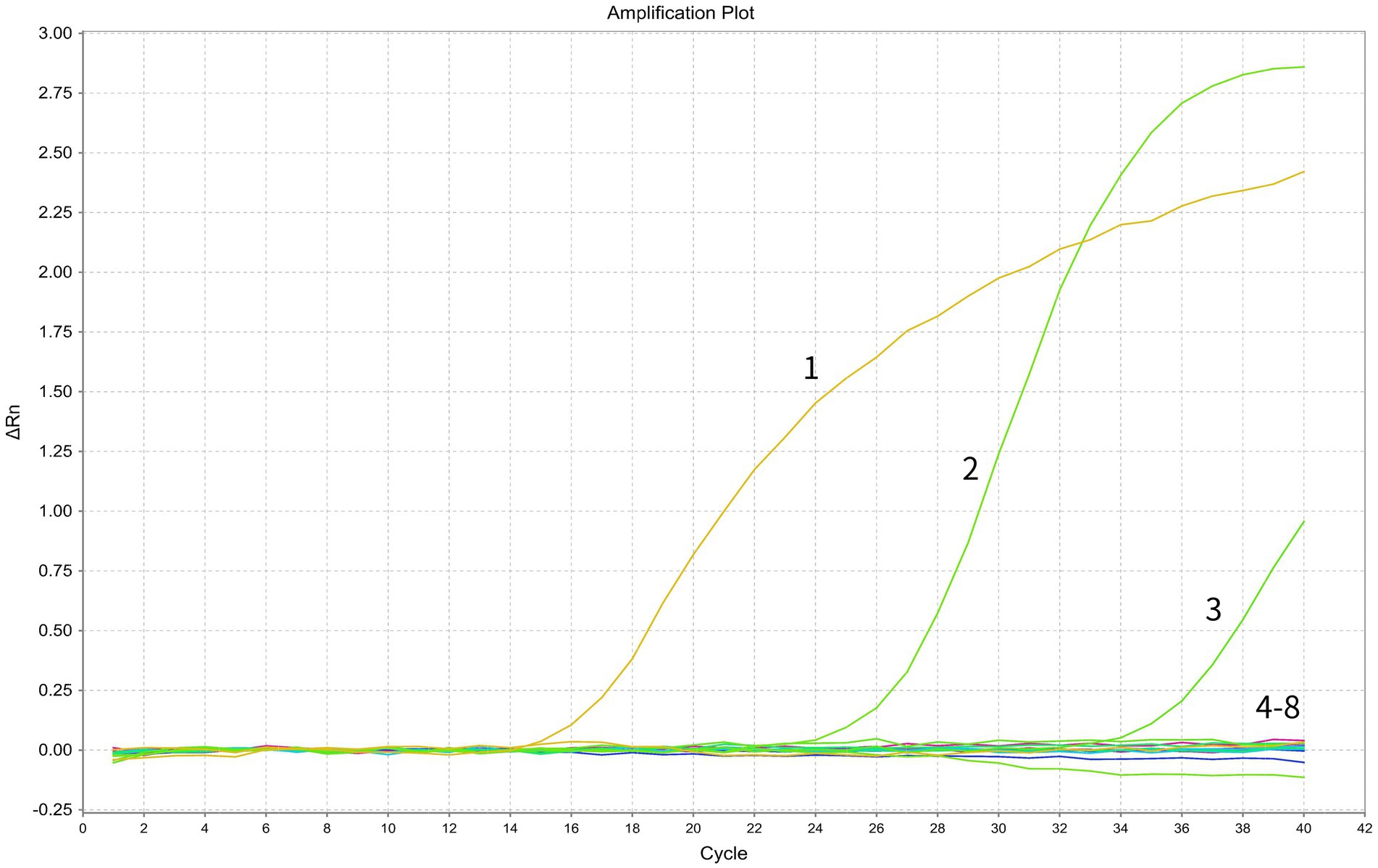
Figure 2. Specificity test of the duplex RT-PCR assay. In the displayed amplification plot, the x-axis represents the cycle number, while the y-axis represents the ΔRn value. Details of the amplification curves are as follows: 1, BRSV standard plasmid; 2, BVDV standard plasmid; 3, BVDV viral nucleic acid; 4–7, viral nucleic acids of BCoV, BPIV3, BoRV, and BoAstV; 8, negative control.
3.4 Repeatability and reproducibility of the duplex real-time RT-PCR assay
The intra-assay and inter-assay variations of Ct values were carefully determined. The analysis revealed that the coefficients of variation (CV) for both BRSV and BVDV are below 0.02% (Table 4), which suggests that the developed duplex real-time RT-PCR assay has high repeatability and stability.
3.5 Application and detection of the duplex real-time RT-PCR assay
A total of 73 clinical samples were tested with the duplex real-time RT-PCR assay. The positive rates for BRSV and BVDV were 5.48% (4/73) and 8.22% (6/73), respectively. No co-infections were detected. To confirm the data, all clinical samples were also tested with the singleplex real-time RT-PCR assay. The results showed a 100% match.
4 Discussion
BRDC is a significant and costly issue in the global cattle industry, due to its complex etiology and the involvement of multiple pathogens (12, 13). Among these, BRSV and BVDV are frequently identified in co-infection, which can lead to increased morbidity and mortality rates (7–10).
BRSV, an enveloped, single-stranded, negative-sense RNA virus, is known for its high morbidity, particularly when combined with other viral or bacterial infections (14). A recent study in China reported a 18.65% positivity rate for BRSV across 23 farms in 11 provinces (9), while another study in Sichuan Province found an even higher average positive rate of 64.57% among yaks (15). BVDV, on the other hand, is an enveloped, single-stranded, positive-sense RNA virus that can be transmitted both vertically and horizontally (16). It has been shown to have a high seroprevalence in East China, with a 77.8% positive rate for BVDV antibodies in bulk tank milk samples, and a lower, but still concerning, 1.86% antigen-positive rate in individual cows. In our study, we observed a positive rate of 8.22% for BRSV and 5.48% for BVDV, without any cases of mixed infection (17).
The multifactorial nature of BRDC makes its management challenging, underscoring the need for rapid and accurate diagnostic tools. Conventional methods, such as serological tests and single-target PCR, have limitations, including cross-reactivity, low sensitivity, and the inability to detect multiple pathogens simultaneously. The development of a duplex TaqMan-based fluorescence quantitative RT-PCR assay for the simultaneous detection of BRSV and BVDV addresses some of these shortcomings. This method offers several advantages over traditional approaches, including: (1) high specificity and sensitivity: the use of specific primers and probes allows for the precise identification of target viruses, reducing the likelihood of false positives or negatives; (2) reproducibility and repeatability: standardized conditions ensure consistent results, which is critical for both routine diagnostics and epidemiological studies; (3) economic and systematic convenience: by detecting two viruses in a single reaction, this assay reduces the cost and time associated with running separate tests, making it more practical for large-scale screening; (4) multiplexing capability: the ability to test for multiple targets simultaneously increases the efficiency of surveillance and monitoring efforts, especially in areas where co-infections are common.
However, the design and validation of such a multiplex assay are not without challenges. One of the key difficulties lies in optimizing the amplification conditions to achieve balanced and efficient detection of all targets. This involves carefully selecting primers and probes that do not cross-react and have similar annealing temperatures, as well as ensuring that the PCR conditions are optimized for all targets. Additionally, the presence of multiple targets in a single reaction can sometimes lead to competition for reagents, which can affect the sensitivity and specificity of the assay. Another significant challenge is ensuring that the assay maintains its performance characteristics across different laboratories and sample types. This requires rigorous standardization and validation processes. Standardization involves developing detailed protocols and guidelines that can be consistently followed by different laboratories. Validation, on the other hand, includes extensive testing to confirm the assay’s accuracy, precision, sensitivity, and specificity. This often involves the use of well-characterized reference materials and the inclusion of positive and negative controls to monitor the performance of the assay over time.
In a summary, a duplex TaqMan-based fluorescence quantitative RT-PCR assay for the simultaneous detection of two bovine respiratory viruses, BRSV and BVDV, was well established. Its high specificity, sensitivity, and reproducibility, coupled with the economic and systematic benefits, make it a valuable tool for both clinical diagnostics and epidemiological investigations. By overcoming the limitations of conventional assays, this method provides a robust platform for the improved management and control of BRDC.
Data availability statement
The raw data supporting the conclusions of this article will be made available by the authors, without undue reservation.
Author contributions
FH: Conceptualization, Data curation, Formal analysis, Funding acquisition, Writing – original draft. JF: Data curation, Formal analysis, Funding acquisition, Visualization, Writing – original draft. JC: Data curation, Formal analysis, Visualization, Writing – original draft. DZ: Writing – review & editing. BC: Writing – review & editing. YL: Writing – review & editing. CL: Conceptualization, Funding acquisition, Investigation, Project administration, Resources, Writing – review & editing.
Funding
The author(s) declare that financial support was received for the research, authorship, and/or publication of this article. This work was supported by Jiangsu Agri-Animal Husbandry Vocational College Key Technology Innovation Funding Project for Industrial Development (NSF2022ZR03), Nanjing Science and Technology Innovation Funding Project for Overseas Researchers (025083501230360), Shandong Provincial Natural Science Foundation, China (ZR2020MC019 and ZR2021LZY030), National Undergraduate Training Program for Innovation and Entrepreneurship (X202410452564), Jiangsu Academy of Agricultural Sciences Basic Scientific Research Special Project (ZX(24)2012) and Jiangsu Independent Innovation Project (CX(24)3002).
Conflict of interest
The authors declare that the research was conducted in the absence of any commercial or financial relationships that could be construed as a potential conflict of interest.
Publisher’s note
All claims expressed in this article are solely those of the authors and do not necessarily represent those of their affiliated organizations, or those of the publisher, the editors and the reviewers. Any product that may be evaluated in this article, or claim that may be made by its manufacturer, is not guaranteed or endorsed by the publisher.
Footnotes
1. ^https://www.ncbi.nlm.nih.gov/tools/primer-blast/
2. ^https://www.technologynetworks.com/tn/tools/copynumbercalculator
References
1. Ferraro, S, Fecteau, G, Dubuc, J, Francoz, D, Rousseau, M, Roy, JP, et al. Scoping review on clinical definition of bovine respiratory disease complex and related clinical signs in dairy cows. J Dairy Sci. (2021) 104:7095–108. doi: 10.3168/jds.2020-19471
2. McGill, JL, and Sacco, RE. The immunology of bovine respiratory disease: recent advancements. Vet Clin North Am Food Anim Pract. (2020) 36:333–48. doi: 10.1016/j.cvfa.2020.03.002
3. Earley, B, Buckham Sporer, K, and Gupta, S. Invited review: relationship between cattle transport, immunity and respiratory disease. Animal. (2017) 11:486–92. doi: 10.1017/S1751731116001622
4. Peel, DS. The effect of market forces on bovine respiratory disease. Vet Clin North Am Food Anim Pract. (2020) 36:497–508. doi: 10.1016/j.cvfa.2020.03.008
5. Gaudino, M, Nagamine, B, Ducatez, MF, and Meyer, G. Understanding the mechanisms of viral and bacterial coinfections in bovine respiratory disease: a comprehensive literature review of experimental evidence. Vet Res. (2022) 53:70. doi: 10.1186/s13567-022-01086-1
6. Bi, YY, Song, LL, Xue, Y, Li, JB, Li, CH, Jia, AQ, et al. Detection of serum antibodies against major pathogens leading to BRDC in large-scale dairy farms in four provinces, Northern China. China Anim Health Insp. (2020) 12:9–13. doi: 10.3969/j.issn.1005-944X.2020.12.003
7. Guo, T, Zhang, J, Chen, X, Wei, X, Wu, C, Cui, Q, et al. Investigation of viral pathogens in cattle with bovine respiratory disease complex in Inner Mongolia, China. Microb Pathog. (2021) 153:104594. doi: 10.1016/j.micpath.2020.104594
8. Jia, S, Yao, X, Yang, Y, Niu, C, Zhao, Y, Zhang, X, et al. Isolation, identification, and phylogenetic analysis of subgroup III strain of bovine respiratory syncytial virus contributed to outbreak of acute respiratory disease among cattle in Northeast China. Virulence. (2021) 12:404–14. doi: 10.1080/21505594.2021.1872178
9. Chang, Y, Yue, H, and Tang, C. Prevalence and molecular characteristics of bovine respiratory syncytial virus in beef cattle in China. Animals. (2022) 12:3511. doi: 10.3390/ani12243511
10. Zhou, Y, Shao, Z, Dai, G, Li, X, Xiang, Y, Jiang, S, et al. Pathogenic infection characteristics and risk factors for bovine respiratory disease complex based on the detection of lung pathogens in dead cattle in Northeast China. J Dairy Sci. (2023) 106:589–606. doi: 10.3168/jds.2022-21929
11. Chen, XD, and Hao, YQ. Prokaryotic expression of bovine viral diarrhea virus E2 protein and development of an indirect ELISA method. Chin J Anim Infect Dis. (2021) 29:74–80. doi: 10.19958/j.cnki.cn31-2031/s.2021.04.008
12. Loy, JD, Leger, L, Workman, AM, Clawson, ML, Bulut, E, and Wang, B. Development of a multiplex real-time PCR assay using two thermocycling platforms for detection of major bacterial pathogens associated with bovine respiratory disease complex from clinical samples. J Vet Diagn Invest. (2018) 30:837–47. doi: 10.1177/1040638718800170
13. Akyüz, E, Merhan, O, Aydın, U, Sezer, M, Atlı, K, Büyük, E, et al. Pentraxin-3, endothelin-1, some biochemical parameters and hematology in bovine respiratory disease complex. Iran J Vet Res. (2023) 24:143–50. doi: 10.22099/IJVR.2023.46494.6674
14. Sarmiento-Silva, RE, Nakamura-Lopez, Y, and Vaughan, G. Epidemiology, molecular epidemiology and evolution of bovine respiratory syncytial virus. Viruses. (2012) 4:3452–67. doi: 10.3390/v4123452
15. Chang, Y, Yue, T, and Yue, H. Molecular epidemiological investigation of yak-derived bovine respiratory syncytial virus. Acta Vet Et Zoontech Sin. (2023) 54:2030–2041. doi: 10.11843/j.issn.0366-6964.2023.05.024
16. Wang, Y, and Pang, F. Diagnosis of bovine viral diarrhea virus: an overview of currently available methods. Front Microbiol. (2024) 15:1370050. doi: 10.3389/fmicb.2024.1370050
Keywords: bovine respiratory disease complex (BRDC), bovine respiratory syncytial virus (BRSV), bovine viral diarrhea virus (BVDV), TaqMan, duplex real-time RT-PCR
Citation: Hao F, Fu J, Chen J, Zhu D, Cai B, Li Y and Liu C (2024) Establishment of a duplex TaqMan-based real time RT-PCR assay for simultaneous detection of BRSV and BVDV. Front. Vet. Sci. 11:1473408. doi: 10.3389/fvets.2024.1473408
Edited by:
Shao-Lun Zhai, Guangdong Academy of Agricultural Sciences, ChinaReviewed by:
Zhanbo Zhu, Heilongjiang Bayi Agricultural University, ChinaWaqas Ahmad, University of Veterinary and Animal Sciences, Pakistan
Ninnet Gómez-Romero, National Autonomus University of Mexico, Mexico
Copyright © 2024 Hao, Fu, Chen, Zhu, Cai, Li and Liu. This is an open-access article distributed under the terms of the Creative Commons Attribution License (CC BY). The use, distribution or reproduction in other forums is permitted, provided the original author(s) and the copyright owner(s) are credited and that the original publication in this journal is cited, in accordance with accepted academic practice. No use, distribution or reproduction is permitted which does not comply with these terms.
*Correspondence: Chuanmin Liu, bGNtMTAyOEAxMjYuY29t
†These authors have contributed equally to this work and share first authorship