- 1School of Public Health, Dali University, Dali, China
- 2Yunnan Institute of Endemic Disease Control and Prevention, Dali, China
- 3State Key Laboratory for Infectious Disease Prevention and Control, Collaborative Innovation Center for Diagnosis and Treatment of Infectious Diseases, National Institute for Communicable Disease Control and Prevention, Chinese Center for Disease Control and Prevention, Beijing, China
Anaplasma phagocytophilum (A. phagocytophilum) is an emerging zoonotic pathogen causing human granulocytic anaplasmosis, linked to small mammal reservoirs that harbor various zoonotic pathogens, underscoring their importance in public health and ecology. This study seeks to determine the prevalence of A. phagocytophilum in small mammals using PCR, then sequence and genotype positive samples, and assess infection risk factors. Small mammals were seasonally captured and a nested polymerase chain reaction (nested-PCR) was conducted targeting the 16S rRNA gene on spleen samples to detect A. phagocytophilum infection from three counties in western Yunnan province, China. Positive samples were sequenced and genotyped, revealing genetic diversity and regional clustering of the pathogen. A total of 1,605 small mammals belonging to 30 species, 18 genera, 6 families, 3 orders were captured seasonally and screened in this region, yielding a 0.93% infection rate with A. phagocytophilum (15/1605). Significant variations in infection rates were observed across different species, counties, and habitats. The 16Sr RNA genes of A. phagocytophilum were categorized into two distinct clades, indicating notable genetic diversity. The identification of genetic variants in spleen samples underscores the potential public health risk and the critical importance of the One Health approach in disease surveillance. Our findings emphasize the necessity for continuous monitoring and highlight the value of nested-PCR testing on spleen samples for accurate prevalence assessment.
1 Introduction
Anaplasma phagocytophilum (A. phagocytophilum), belonging to the order Rickettsiales, family Anaplasmataceae, genera Anaplasma, is a zoonotic tick-borne pathogen transmitted by infected ticks (1, 2). A. phagocytophilum primarily infects human granulocytes, such as neutrophils, leading to human granulocytic anaplasmosis (HGA) (3). As a zoonotic disease, the rapid increase of Anaplasmosis prevalence in Europe, America, Africa, and Asia, has aroused widespread concern (4–8). Since clinical signs are unspecific and animals infected with A. phagocytophilum may not show any clinical signs at all, diagnosis of granulocytic anaplasmosis can be challenging. In China, seroprevalence studies have indicated that infections with A. phagocytophilum in humans have been documented across several provinces. And a study showed that 20% of the tested individuals (64/323) were positive to A. phagocytophilum and that the incidence was higher in male (22%) than female (16%) (9). However, it is plausible that the actual number of reported cases is underestimated (9–11).
Persistent infection in reservoir hosts is crucial for sustaining the natural transmission cycle of A. phagocytophilum and defining its infection range (10). This pathogen has been identified in a wide range of animal hosts, including dogs, cats, and small mammals (12–15). Small mammals, in particular, serve as reservoirs for numerous zoonotic pathogens, underscoring their importance in public health and ecological research. In general, small mammals, especially in the USA, are important reservoirs of the A. phagocytophilum strain pathogenic to humans. And their short lifespan is likely to reduce their epidemiological importance as reservoir hosts, which remains hotly debated (11). Therefore, it is crucial to monitor the infection of A. phagocytophilum in small mammals as a potential threat to the health of humans, domestic and wild animals.
Yunnan province is characterized by a complex terrain with subtropical plateau monsoon, maintains a high biodiversity of small mammals that may play important role on A. phagocytophilum transmission. However, few surveillance studies have been conducted in this region. The available data are limited to investigations on Anaplasma infection in domestic in the northwestern of Yunnan (16). This study aims to detect the A. phagocytophilum infection in wild small mammals, characterize their genotypes, and evaluate the environmental factors of A. phagocytophilum infection on small mammal from three counties in western Yunnan province.
2 Materials and methods
2.1 Sampling sites and small mammals trapping
Using the convenient sampling method, seven villages or towns were selected as sampling sites: three from Lianghe (Mangdong, Hexi and Nangsong), two from Jianchuan (Shilong and Dongshan) and one from Yulong (Wenbi Mountain). The location of three sampling sites was drawn with ArcGIS 10.5 (Figure 1). In each season, small mammals were captured seasonally by snap traps (15 × 8 cm, Metal rat trap clip, China) and peanut as bait set at woodland, cultivated land, thickets in 57 sample locations from seven villages or towns, with a total of 200 snap traps per sample location were placed for three consecutive nights during December 2015 and October 2016. Mammal species were identified according to morphological characteristics in the local Centers for Disease Control (CDC) laboratory and a necropsy was performed, with recordings of gender, habitats and sampling seasons. Spleen tissue samples from each specimen were collected using aseptic techniques and stored at −40°C for DNA extraction.
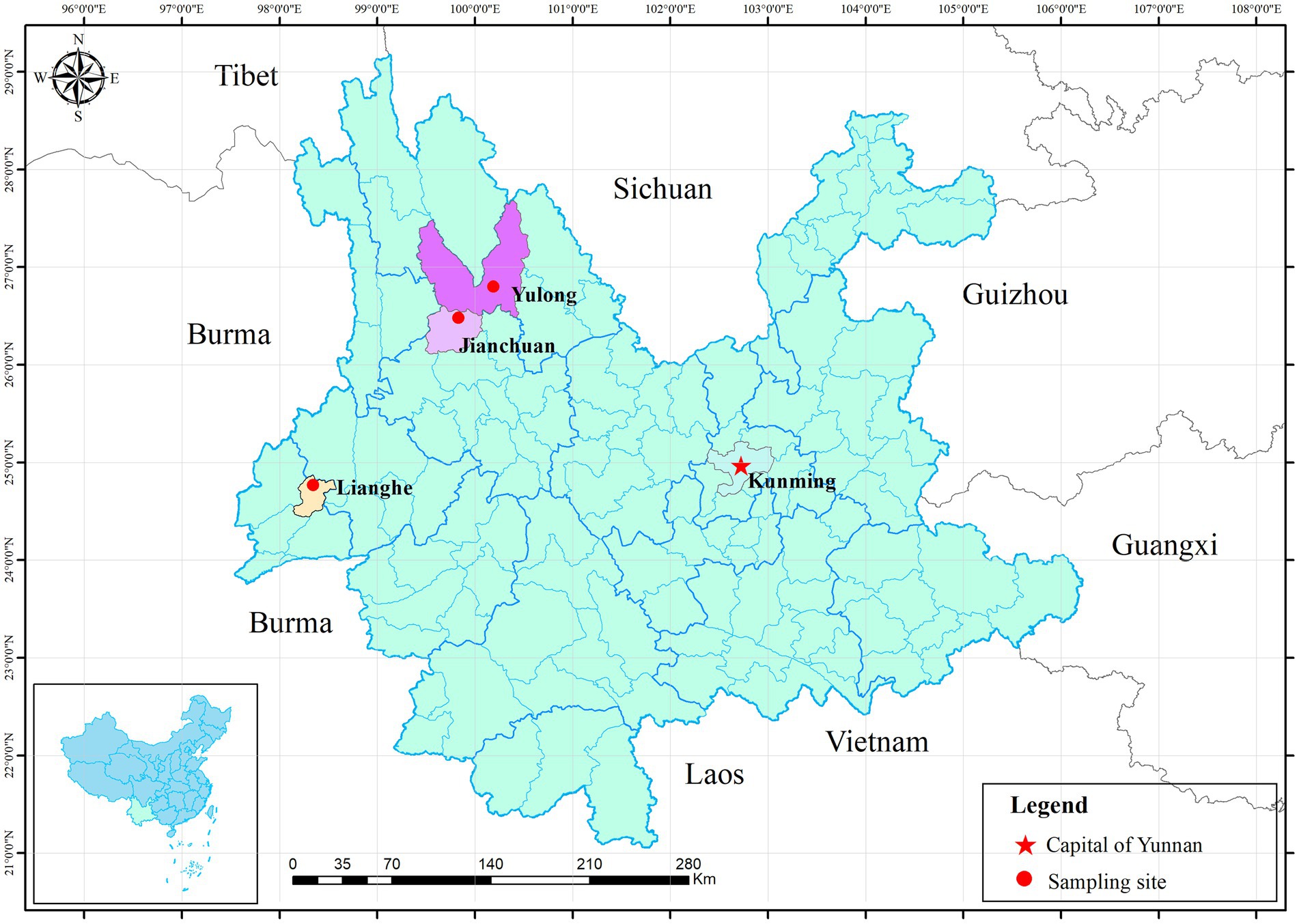
Figure 1. Location of three sampling sites in Yunnan province. Three red dots represent three sampling counties. The red five-pointed star represents Kunming, the capital of Yunnan province.
2.2 DNA extraction and nested polymerase chain reaction (nested-PCR) analysis
DNA was extracted from spleen issue using the magnetic beads tissue/cell genome DNA isolation kit (AU19014, Beijing BioTeke Corporation, China) according to manufacturer’s instructions. DNA concentrations were determined by the ultra-micro nucleic acid ultraviolet tester (NanoDrop 1,000, Thermo Fisher Scientific, USA). As the extract concentration was ≥50 μg/mL, and A260/A280 was 1.8 to 2.0, this template was selected for detection (12). Nested PCR was used for specfication based on 16S rRNA gene amplification. The first round of primers used the generic gene of Anaplasma and Ehrlichia, and the second round was performed using the specific primers for the A. phagocytophilum (Table 1) (13). These primers were synthesized at a concentration of 10 μM (Sangon Biotech, Shanghai, China).
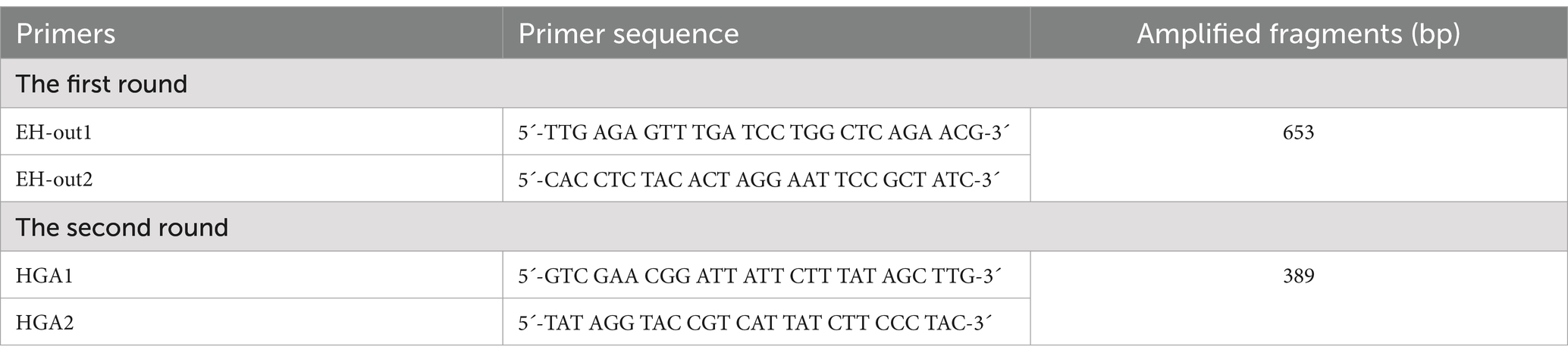
Table 1. The detection primers of Anaplasma phagocytophilum based on the 16S rRNA gene in nested PCR.
The first round PCR reactions were performed in 25 μL volumes. This included 12.5 μL Dream Taq Green PCR Master Mix (K1081, Thermo Fisher Scientific, Waltham, MA, USA), 0.5 μL each of first round primers EH-out1 and EH-out2 (10 μmol/L), 5 μL DNA template, and 6.5 μL sterile deionized water. Cycling protocol was as follows: initial denaturation at 95°C for 3 min, followed by 35 cycles of denaturation at 95°C for 30 s, annealing at 55°C for 40 s, extension at 72°C for 50 s, and a final extension at 72°C for 7 min. The second round PCR reactions were performed in 30 μL volumes, including 15 μL Dream Taq Green PCR Master Mix, 0.6 μL each of second round primers HGA1 and HGA2, 0.5 μL template (the first-round PCR product), and 13.3 μL sterile deionized water. PCR conditions for the second round were the same as those for the first round, except the annealing temperature was 57°C rather than 55°C. The whole process was controlled by both positive and negative controls. Positive controls consisted of the first PCR amplified positive product for A. phagocytophilum confirmed by sequencing in this study, and negative controls consisted in sterile water. Second-round PCR products of 3 μL were electrophoresed on 1.5% agarose gels under the condition of 120 V for 45 min, and visualized under a Gel imaging system (G: BOX F3, Syngene, Frederick, MD, USA). The amplied products about 389 bp were confirmed as A. phagocytophilum positive and then sequenced in both directions by Shanghai Sangon Biotech.
2.3 Sequence analysis and phylogenetic analysis
The sequences were compared with published gene sequences obtained from the GenBank database, using the BLAST Sequence Similarity Searching tool.1 Sequences analysis was carried out using the MegAlign program in DNASTAR package. A Phylogenetic tree was constructed by a neighbor-joining method with 1,000 bootstrap replications in MEGA 7.0 software.
2.4 Statistical analysis
Data on geography and laboratory parameters was collected using Microsoft Excel 2016. Depending on their constituent ratio, wild small mammals were classified as dominant (>10%) or other (≤10%). In calculating the A. phagocytophilum infection rate, we used the number of wild small mammals infected with A. phagocytophilum as the numerator, and the number of wild small mammals whose DNA had been successfully extracted as a denominator. Differences of A. phagocytophilum infection in small mammals among species, gender, habitat, sampling seasons and counties were conducted by Fisher’s Exact Test under R software (version 4.0.1). All p-values were 2-tailed, and p<0.05 was considered statistically significant.
2.5 Ethics approval
The study has been approved the Medical Ethics Committee of Dali University (no. MECDU-201507-21). The small mammals captured were allowed and no painful.
3 Results
3.1 Species of wild small mammals and A. phagocytophilum infection
A total of 1,605 wild small mammals were collected at three counties from western Yunnan province in this study. These wild small mammals were classified into 30 species, 18 genera, six families, three orders; Apodemus chevrieri (29.35%, 471/1605) and Eothenomys miletus (19%, 305/1605) were the dominant species. In Lianghe, a total of 407 wild small mammals were identified; the dominant species were Rattus tanezumi (24.57%, 100/407), Rattus steini (19.9%, 81/407), Mus pahari (10.81%), and Niviventer fulvescens (10.07%, 41/407). Jianchuan and Yulong have the same dominant rat species. In Jianchuan, the dominant species were Ap. chevrieri (44.14%, 286/648), E. miletus (31.94%, 207/648), and Apodemus draco (10.65%, 69/648). In Yulong, the dominant species were Ap. chevrieri (33.64%, 185/550), E. miletus (16.55%, 91/550), and Ap. draco (16%, 88/550).
Fifteen out of 1,605 samples were positive according to the 16Sr RNA gene with an overall prevalence of 0.93% (Table 2). Based on the agarose gel electrophoresis results of nPCR, five of 30 species were positive with a molecular weight of about 389 bp (Figure 2). Different species showed different rates of A. phagocytophilum infection, ranging from 0.21 to 20%. Rattus nitidus showed the greastest prevalence of A. phagocytophilum (20.0%, 3/15), followed by R. tanezumi (5.77%, 6/104), R. steini (4.88%, 4/82), E. miletus (0.33%, 1/305), and Ap. chevrieri (0.21%, 1/471). The infection rate of A. phagocytophilum in wild small mammals from Lianghe and Yulong counties was 3.19% (13/407) and 0.36% (2/550), respectively. However, no positive test was found in Jianchuan (0%, 0/648). Three of the 17 species of wild small mammals were positive in Lianghe; the prevalence of A. phagocytophilum was highest in R. nitidus (21.43%, 3/14), followed by R. tanezumi (6%, 6/100), and was lowest in R. steini (4.94%, 4/81). The positive species of wild small mammals of Yulong were E. miletus (1.1%, 1/91) and Ap. chevrieri (0.54%, 1/185); the remaining species were not detected. In Jianchuan, E. miletus and Ap. chevrieri were captured in large numbers, but no positive A. phagocytophilum was detected (Table 2).
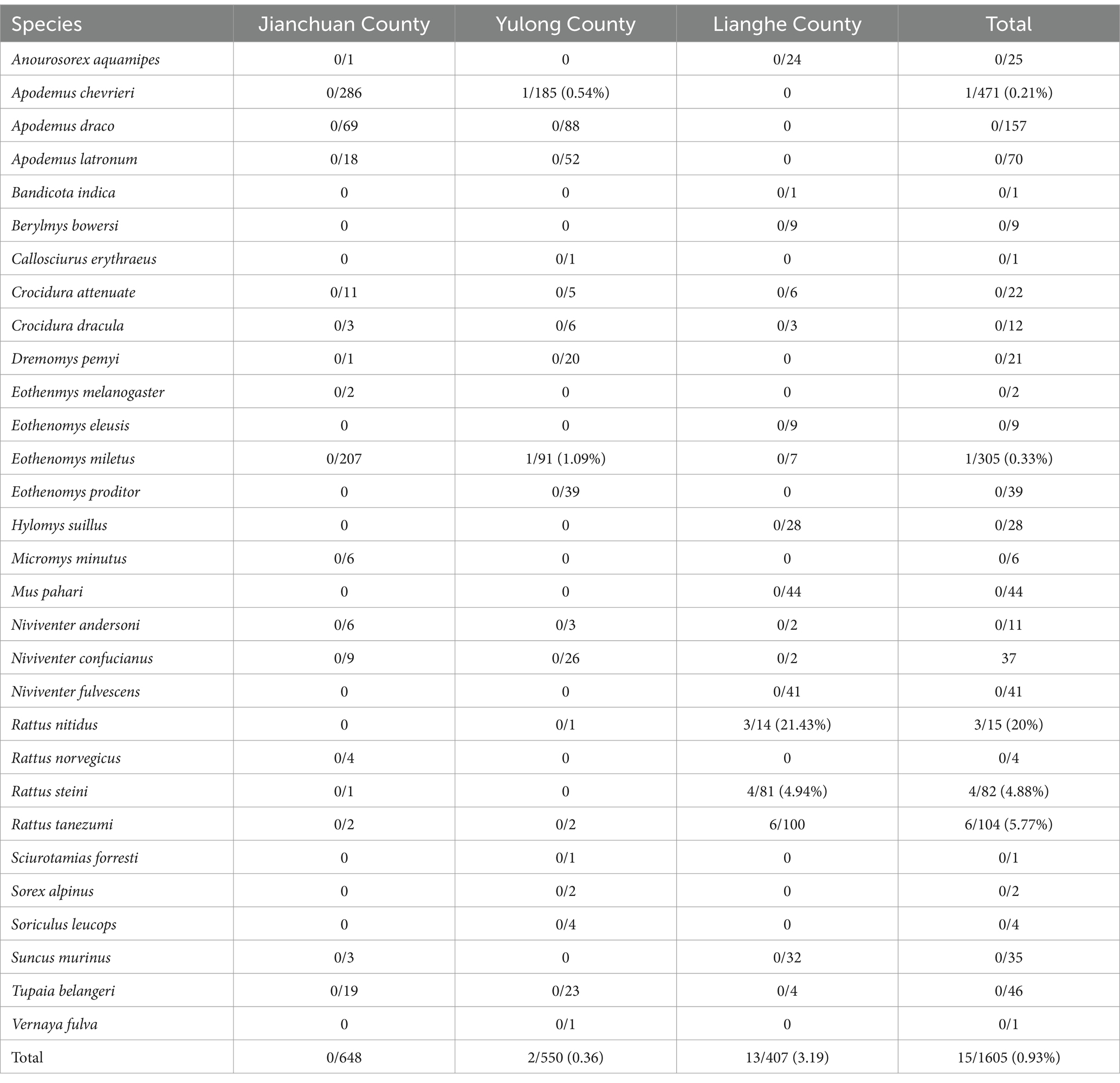
Table 2. Distribution of infection on wild small mammals captured from western Yunnan province [positive/n (%)].
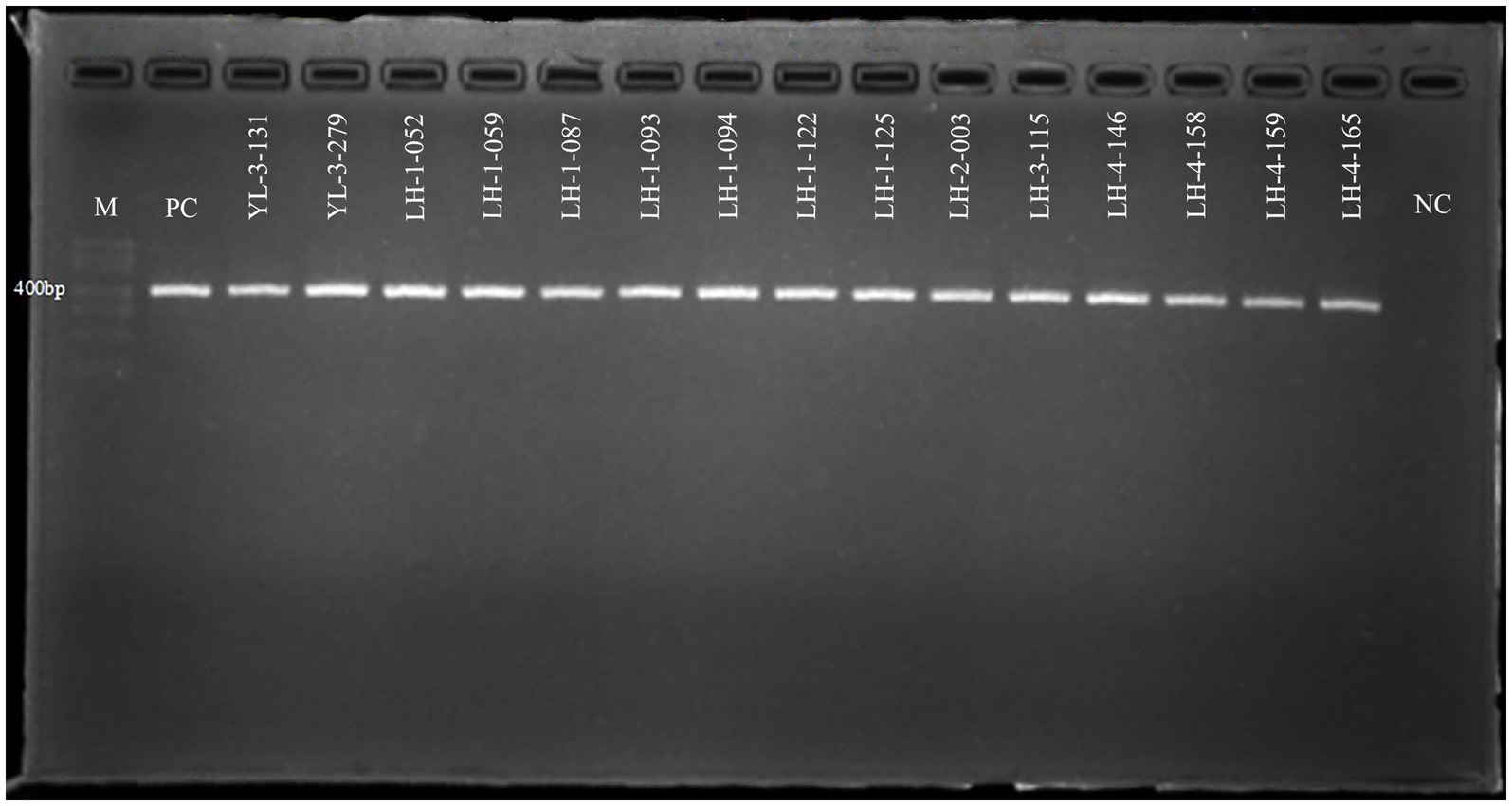
Figure 2. Electropherogram of Anaplasma phagocytophilum positive products by nested-PCR. Marker shows in the picture from top to bottom: 700, 600, 500, 400, 300, 200, 100 bp. Fifteen positive amplification products in this study were showed, which were YL-3-131 ~ LH-4-165 in turn. M, DNA marker; PC, positive control; NC, negative control; YL, Yulong; LH, Lianghe.
3.2 Phylogenetic analysis based on the 16S rRNA gene of A. phagocytophilum
Out of 15 sample sequences (13 from Lianghe, 2 from Yulong), the 16S rRNA gene nucleotide homology and phylogenetic analysis were performed. Fifteen positive sample sequences aligned with 12 reference ones, which demonstrated 84.3–100% identity to A. phagocytophilum strains (Figure 3). All sequences of Lianghe county (LH-1-052, LH-1-059, LH-1-087, LH-1-093, LH-1-094, LH-1-122, LH-1-125, LH-2-003, LH-3-115, LH-4-146, LH-4-158, LH-4-159, LH-4-165) were identical to each other and to a sequence from Yulong (YL-3-131). The other sequence from Yulong (YL-3-279) shared 97.2% similarity with these strains (Figure 3).
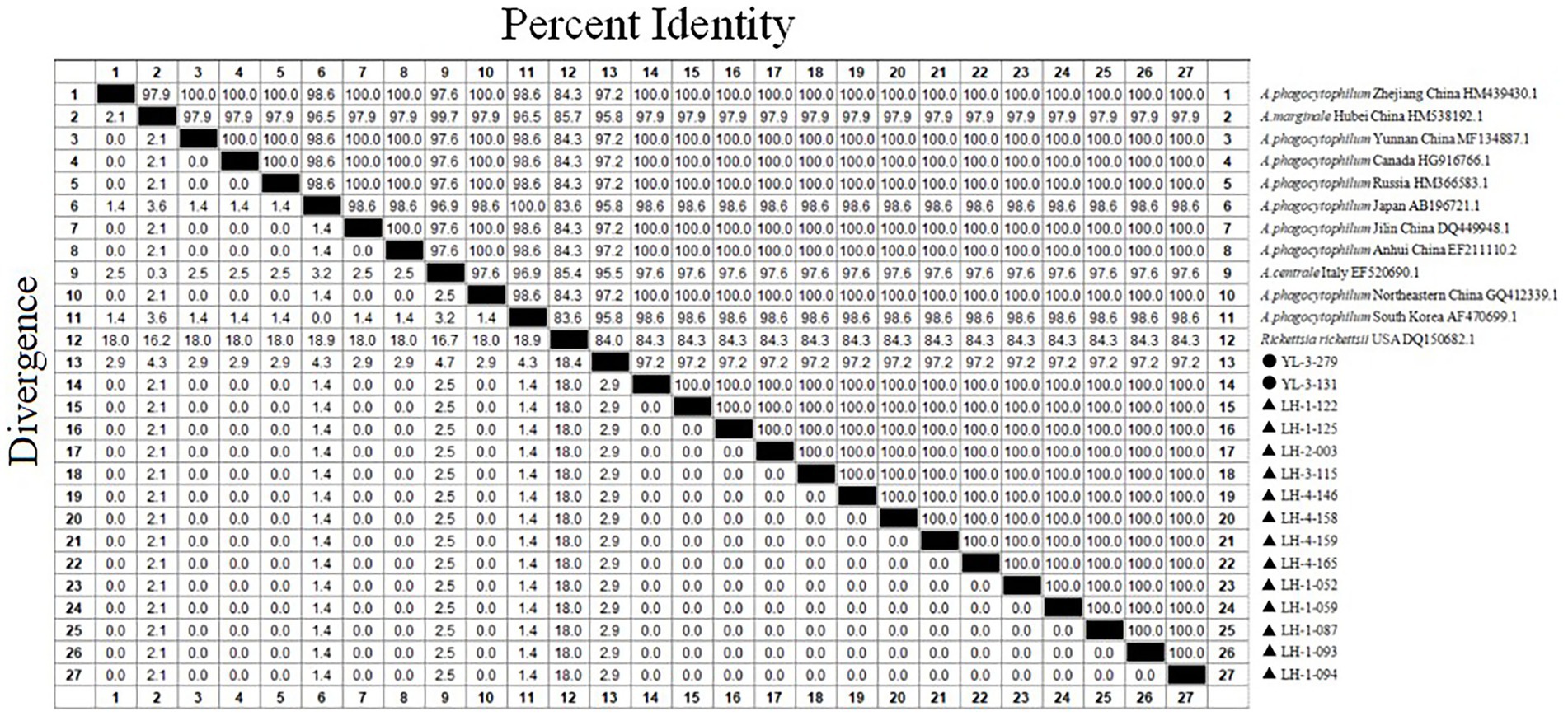
Figure 3. The 16S rRNA nucleotide homology matrix of Anaplasma phagocytophilum strain and reference strain in western Yunnan province. ▲, positive samples from Lianghe; ●, positive samples from Yulong.
The phylogenetic tree revealed that all sequences from Lianghe and YL-3-131 clustered together, along with seven A. phagocytophilum sequences retrieved from GenBank (Anhui, Jilin, Zhejiang, Yunnan, North eastern China, Canada, and Russia). The other sequence from Yulong (YL-3-279) was clustered into an independent small branch, while Rickettsia rickettsii USA DQ150682.1 was at the periphery (Figure 4). The positive R. nitidus (3 strains), R. tanezumi (6 strains), R. steini (4 strains) all originated from Lianghe; the positive E. miletus (1 strains) and Ap. chevrieri (1 strains) all originated from Yulong. While no positive samples were found in 648 wild small mammals collected from Jianchuan.
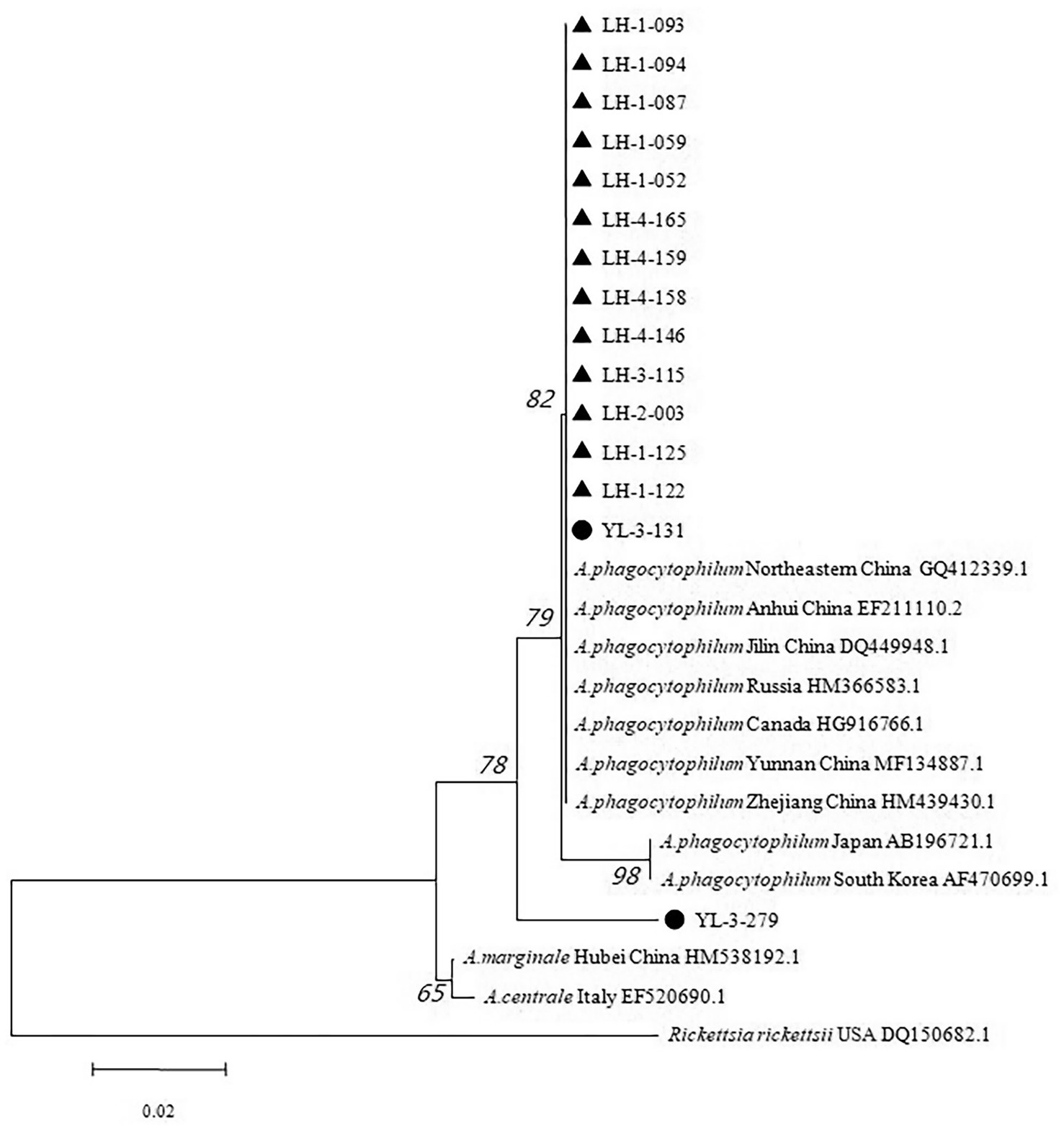
Figure 4. Phylogenetic tree based on 16S rRNA gene of Anaplasma phagocytophilum. ▲, positive samples from Lianghe; ●, positive samples from Yulong.
3.3 Associated environmental and seasonal factors for A. phagocytophilum infection
The related factors of A. phagocytophilum infection are showed in Table 3. Compared with Yulong and Jianchuan counties, Lianghe county had a higher infection prevalence among wild small mammals (p < 0.001). Compared to the different species of wild small mammals, the rate of A. phagocytophilum infection of the dominant species is higher than the other species (p = 0.006). The infection rate of A. phagocytophilum was 1.45% in the woodland, and no infection was found in the other two habitats (Cultivated land and thickets). Nevertheless, the infection rate of A. phagocytophilum in wild small mammals was not significantly different in gender and sampling seasons (p > 0.05) (Table 3).
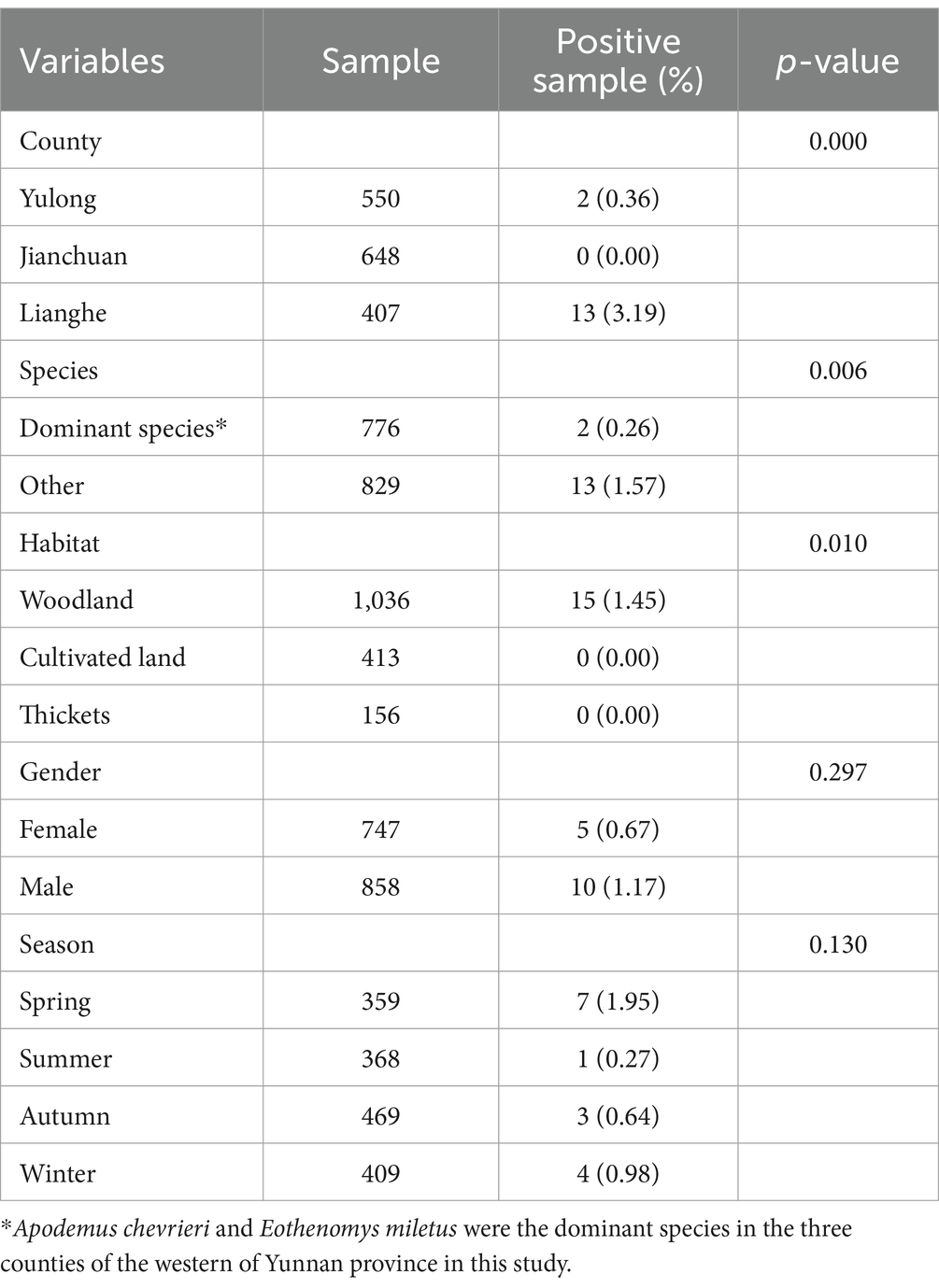
Table 3. An analysis of factors affecting Anaplasma phagocytophilum infection rates in wild small mammals from western Yunnan province.
4 Discussion
In this study, a total of 1,605 wild small mammals were collected from three counties of the western of Yunnan province, and molecular detection and phylogenetic analysis of 15 A. phagocytophilum were performed. The study concerning A. phagocytophilum infection data from 407 wild small mammals in Lianghe County has been published in Chinese academic journals (14). Notably, we found that Lianghe County had the highest infection rate of A. phagocytophilum, with significant rates in R. nitidus, R. tanezumi, and R. steini at 20, 5.77, and 4.88%, respectively. These data highlight the potential key role of these species in the transmission of A. phagocytophilum.
The infection rate of A. phagocytophilum in Lianghe was the highest among three counties, where the 16S rRNA gene identical sequences of A. phagocytophilum were recovered from R. nitidus, R. tanezumi, R. steini, which shared 100% identity with a human case of HGA first reported in Anhui (EF211110.2) and a rodent sequence of A. phagocytophilum previously reported in Yunnan (MF134887.1). This result may be due to the temperature and humidity in Lianghe, which is conducive for tick growth and reproduction. Lianghe county is in the southwestern part of the Hengduan mountain, and has a rich water resource and lush vegetation due to its south subtropical monsoon climate. In the middle of the Hengduan mountain, Jianchuan and Yulong counties are separated by a low-lying valley. Yulong county has a low-latitude highland South Asian monsoon climate. Jianchuan is dominated by pine forests, which has a low-latitude highland monsoon climate. We know that geographical environment is one of the key conditions for the long-term survival of small mammals and ticks, which indirectly affects the transmission and host storage capacity of A. phagocytophilum. A variety of geographical conditions and climates support a wide variety of small mammals, each with its own storage capacity for A. phagocytophilum. It is possible that the south Asian monsoon climate in Lianghe provides ideal conditions for ticks and A. phagocytophilum to survive and spread. However, the association between A. phagocytophilum infection in wild small mammals and meteorological factors, including temperature, humidity, precipitation, and altitude, requires further investigation to elucidate the potential impacts of these environmental variables on the pathogen’s prevalence and transmission dynamics. In a word, this indicates that the potential for A. phagocytophilum infection in humans warrants serious consideration and underscores the necessity for enhanced surveillance of small mammals.
The genetic analysis of the two Yulong strains has elucidated a dichotomy in their phylogenetic clustering, suggesting a potential divergence in their evolutionary pathways and ecological interactions. Notably, one of the Yulong strains (YL-3-131) demonstrated a high degree of sequence similarity with the Lianghe and Anhui (EF211110.2) strains. This observation raises the possibility of zoonotic transmission to humans, considering the established propensity of the Anhui strains for human infection. The genetic proximity indicates that the Yulong strain may exhibit analogous pathogenic characteristics and transmission mechanisms, thereby necessitating a thorough investigation into its potential public health implications. In contrast, the other Yulong strain (YL-3-279) formed an independent phylogenetic branch, deviating from the genetic clustering observed for the Lianghe-like strain. The distinctive positioning of YL-3-279 in the phylogenetic tree may be attributed to two factors. One plausible explanation is the presence of a short sequence or partial mutation in the amplified product of the positive sample, which could have introduced bias in the genetic characterization. Alternatively, YL-3-279 may constitute a novel genotype of A. phagocytophilum, thereby illustrating the genetic diversity inherent within the species and underscoring the complexity of its evolutionary trajectory. The identification of distinct genetic variants within the Yulong strains highlights the imperative for a thorough examination of their genetic diversity. This examination should encompass the potential associations between genetic variants and their respective rodent hosts, in addition to the tick vectors implicated in their transmission. Moreover, the geographical origin of these strains may significantly influence their genetic composition, indicating a potential eco-evolutionary interaction among the pathogen, its vectors, and the local environment. Consequently, the phylogenetic analysis of the Yulong strains has revealed a complex genetic landscape that warrants further investigation. The potential for zoonotic transmission, the presence of novel genotypes, and the interactions among genetic variants, rodent hosts, tick vectors, and geographical origins necessitate a multidisciplinary approach to fully elucidate the pathogen’s biology and its public health implications.
Our present study evaluated the host, environmental factors and genetic diversity of A. phagocytophilum in small mammals from three counties of Yunnan in China. Previous studies demonstrated that infections with A. phagocytophilum occurred widely among the residents and domestic animals in Yunnan province. The seropositivity rates were 7.59% in healthy donors, 4.49% in acute undifferentiated fever patients, and 15.56% in newly enrolled college students in Yunnan province (15, 17). Another study involving nine provinces of China reported a seroprevalence of 0.6% among rural residents in Yunnan also suggested underestimating the number of reported cases (18). Another study of domestic animals showed the infection rate with A. phagocytophilum ranged from 6.29 to 41.08% (dogs: 6.29%; sheep: 38.10%; cattle: 41.08%) (16). Another study in Korea showed that the infection rate of A. phagocytophilum was 1.7% in ticks removed from humans (19). These evidences suggest that the presence of A. phagocytophilum in the natural environment should not be disregarded, as there exists a potential risk of transmission to humans. However, few studies on A. phagocytophilum infection on wild small mammals were reported in the western of Yunnan Province. The infection of A. phagocytophilum in small mammals in our study was 0.93% (15/1605). More importantly, the R. nitidus, R. tanezumi, and R. steini exhibited significantly higher infection rates of 20, 5.77, and 4.88%, respectively. These findings suggest that these wild small animals may serve as principal reservoir hosts for A. phagocytophilum in China. The findings also indicate the necessity of enhancing surveillance and research concerning the infection status of wild small animals in the region to remain vigilant against the potential threat they may pose to human health. Given the transmission pathways and clinical manifestations of HGA, further investigation and the implementation of preventive measures are especially critical.
Furthermore, the findings indicate that the prevalence of A. phagocytophilum infection in small mammals varies according to habitat type, with the highest infection rates observed in woodland areas. This variation is likely attributable to differences in tick vector density and habitat preference. Consequently, individuals engaging in activities within woodland environments should implement appropriate protective measures to minimize their risk of tick bites. No significant gender differences in the infection rates of A. phagocytophilum among wild small mammals were observed in our study, which contradicts the findings of previous studies. Prior studies have suggested that the infection rate of A. phagocytophilum is associated with both the gender and body weight of the host animals (20). The discrepancy in our results may stem from similar activity ranges and tick exposure for both male and female wild small mammals, suggesting that gender-based differences may be less significant than in previous studies due to uniform environmental interactions with the vector. The infection rate of A. phagocytophilum for wild small mammals did not show any significant difference between seasonal variations in this study. As is known to all, ticks are the main vectors of A. phagocytophilum, whose activity depends on the humidity and temperature. Seasonal changes such as rainfall or temperature have a significant impact on the host animals, the habitat and the tick vector’s feeding habits (21). A previous study imply that the ruminant and bird hosts have the characteristics of seasonal dynamics of tick-borne pathogens because of their abundance and temporal population fluctuations, but not in wild small mammals (22). This result may be explained that these tick vectors may spend all their life in their hosts’ burrows, where the environmental conditions are buffered and rely less on climatic conditions (20). Even so, seasonal changes with A. phagocytophilum infection should be paid more attention to avoid the occurrence and epidemic of natural focal diseases. In other words, the range of tick habitats may be expanding rapidly due to global warming, increasing the likelihood of tick-borne diseases emerging and reemerging.
This study has also some limitations. The study investigated only three counties in western Yunnan, with limited sampling points. But these three counties are rich in small mammals, which are the representative places of plague focus in Yunnan Province (Lianghe County: the commensal rodent plague focus, Jianchuan and Yulong: the wild rodent plague focus) (23). As a result of this abundance, all rodent-borne diseases may be at risk. Then, ticks are important reservoirs and vectors for A. phagocytophilum, and no ticks were found on the surface of the body of any small mammals during the survey. Then a comprehensive survey of the seasonal occurrence of the small mammals in consecutive years of A. phagocytophylum infection has not yet been carried out. It is not known about tick infestation or whether there is a correlation between tick infestation rates and small mammal infestation, which deserve further study. In addition, our study acknowledges the limitations in exploring the direct impacts of environmental variables on tick ecology and disease transmission dynamics. We recommend that future research incorporate more detailed environmental data, including long-term climate records and tick population monitoring, to better understand these complex relationships. Lastly, we did not investigate the prevalence of A. phagocytophilum infection in the human of this area, this also is what we are going to do next. However, several advantages exist in our study. Thirty species of small mammals were captured in this study, which were not limited to the dominant species in the area. Due to the extensive sampling of animals, this study provides a comprehensive reflection of the infection status of A. phagocytophilum in small mammals within the region. Secondly, the detection of A. phagocytophilum infection demonstrated significant disparities across spatial, species-specific, and habitat-related dimensions. These variations imply that ecological factors may play a critical role in shaping the transmission dynamics and prevalence of the pathogen. Recognizing such disparities is essential for advancing a nuanced understanding of the pathogen’s ecology and for pinpointing potential hotspots of infection. Furthermore, our research suggests the potential existence of a novel genotype of A. phagocytophilum within the Yulong region. This hypothesis is supported by observed genetic variations in the pathogen strains when compared to established genotypes. The identification of a new genotype could significantly enhance our understanding of the pathogen’s evolutionary history, its adaptability to diverse hosts and environments, and its potential implications for public health. Future research should utilize advanced molecular methodologies to elucidate the genetic diversity and phylogenetic relationships among various strains. These genetic insights are crucial for guiding targeted surveillance initiatives, forecasting disease transmission, and formulating effective intervention strategies.
5 Conclusion
This study has identified five species of wild small mammals in western Yunnan Province harboring infections of A. phagocytophilum, specifically R. tanezumi, R. steini, R. nitidus, E. miletus, and Ap. chevrieri. The genetic diversity of A. phagocytophilum, coupled with the observed variations in infection rates across the three sampling areas and different habitats, highlights the complex interplay between ecological factors and disease prevalence. The detection of A. phagocytophilum in these small mammals is significant as it indicates a potential sylvatic reservoir that could impact human health, particularly in areas where human-mammal interactions are frequent, such as woodlands. The genetic variability observed within the pathogen may influence its virulence, transmission dynamics, and the efficacy of any future intervention strategies. Given the potential for A. phagocytophilum to be transmitted to humans, it is imperative that public health authorities maintain vigilant surveillance of wild small mammal, particularly in ecologically diverse regions like western Yunnan Province. Continued monitoring will not only inform us about the prevalence of the pathogen but also provide insights into the effectiveness of natural barriers to pathogen transmission. In conclusion, our study provides valuable insights into the ecological factors influencing A. phagocytophilum infections in wild small mammals. The implications of these findings for public health are significant, warranting further research and proactive disease management strategies.
Data availability statement
The datasets presented in this study can be found in online repositories. The names of the repository/repositories and accession number(s) can be found in the article/supplementary material.
Ethics statement
The animal study was approved by the Medical Ethics Committee of Dali University. The study was conducted in accordance with the local legislation and institutional requirements.
Author contributions
J-jZ: Data curation, Formal analysis, Funding acquisition, Software, Visualization, Writing – original draft, Writing – review & editing, Methodology. H-zZ: Data curation, Formal analysis, Methodology, Software, Visualization, Writing – original draft. R-dH: Investigation, Methodology, Writing – original draft. DY: Data curation, Formal analysis, Methodology, Writing – original draft. MH: Investigation, Writing – original draft. Z-xL: Investigation, Writing – original draft. D-mL: Methodology, Writing – original draft. J-xY: Conceptualization, Funding acquisition, Investigation, Project administration, Resources, Supervision, Validation, Writing – review & editing.
Funding
The author(s) declare that financial support was received for the research, authorship, and/or publication of this article. This research was funded by the National Natural Science Foundation of China (No. 81860565), the project of “Talent Support Program in Yunnan” (No. YNWR-MY-2019-008), the Science and Technology Innovation Team of Natural Focal Diseases Epidemiology in University of Yunnan Province [Yunnan Provincial Department of Education issued (2020) No. 102], and the Doctoral Research Fund Grants of Dali University (KYBS2023011).
Acknowledgments
The authors would like to thank Jianchuan Station for Endemic Disease Control and Prevention, Lianghe Center for Disease Control and Prevention, and Yulong Center for Disease Control and Prevention for assistance in the progress of the project.
Conflict of interest
The authors declare that the research was conducted in the absence of any commercial or financial relationships that could be construed as a potential conflict of interest.
Publisher’s note
All claims expressed in this article are solely those of the authors and do not necessarily represent those of their affiliated organizations, or those of the publisher, the editors and the reviewers. Any product that may be evaluated in this article, or claim that may be made by its manufacturer, is not guaranteed or endorsed by the publisher.
Footnotes
References
1. Mansfield, KL, Cook, C, Ellis, RJ, Bell-Sakyi, L, Johnson, N, Alberdi, P, et al. Tick-borne pathogens induce differential expression of genes promoting cell survival and host resistance in ixodes ricinus cells. Parasit Vectors. (2017) 10:81. doi: 10.1186/s13071-017-2011-1
2. Madison-Antenucci, S, Kramer, LD, Gebhardt, LL, and Kauffman, E. Emerging tick-borne diseases. Clin Microbiol Rev. (2020) 33:e18–83. doi: 10.1128/CMR.00083-18
3. Artigas-Jeronimo, S, Gonzalez-Garcia, A, de la Fuente, J, Blanda, V, Shekarkar, AM, Villar, M, et al. Low netosis induced in anaplasma phagocytophilum-infected cells. Vaccines. (2022) 10:1756. doi: 10.3390/vaccines10101756
4. Stokes, W, Lisboa, LF, Lindsay, LR, and Fonseca, K. Case report: anaplasmosis in Canada: locally acquired Anaplasma phagocytophilum infection in Alberta. Am J Trop Med Hyg. (2020) 103:2478–80. doi: 10.4269/ajtmh.20-0603
5. Lehane, A, Maes, SE, Graham, CB, Jones, E, Delorey, M, and Eisen, RJ. Prevalence of single and coinfections of human pathogens in ixodes ticks from five geographical regions in the United States, 2013-2019. Ticks Tick Borne Dis. (2021) 12:101637. doi: 10.1016/j.ttbdis.2020.101637
6. Sosa-Gutierrez, CG, Cervantes-Castillo, MA, Laguna-Gonzalez, R, Lopez-Echeverria, LY, Ojeda-Ramirez, D, and Oyervides, M. Serological and molecular evidence of patients infected with Anaplasma phagocytophilum in Mexico. Diseases. (2021) 9:37. doi: 10.3390/diseases9020037
7. Markowicz, M, Schotta, AM, Hoss, D, Kundi, M, Schray, C, Stockinger, H, et al. Infections with tickborne pathogens after tick bite, Austria, 2015-2018. Emerg Infect Dis. (2021) 27:1048–56. doi: 10.3201/eid2704.203366
8. Wang, F, Yan, M, Liu, A, Chen, T, Luo, L, Li, L, et al. The seroprevalence of Anaplasma phagocytophilum in global human populations: a systematic review and meta-analysis. Transbound Emerg Dis. (2020) 67:2050–64. doi: 10.1111/tbed.13548
9. Zhang, S, Hai, R, Li, W, Li, G, Lin, G, He, J, et al. Seroprevalence of human granulocytotropic anaplasmosis in central and southeastern China. Am J Trop Med Hyg. (2009) 81:293–5. doi: 10.4269/ajtmh.2009.81.293
10. Kolo, A. Anaplasma species in Africa-a century of discovery: a review on molecular epidemiology, genetic diversity, and control. Pathogens. (2023) 12:702. doi: 10.3390/pathogens12050702
11. Tomassone, L, Berriatua, E, De Sousa, R, Duscher, GG, Mihalca, AD, Silaghi, C, et al. Neglected vector-borne zoonoses in Europe: into the wild. Vet Parasitol. (2018) 251:17–26. doi: 10.1016/j.vetpar.2017.12.018
12. Olson, ND, and Morrow, JB. DNA extract characterization process for microbial detection methods development and validation. BMC Res Notes. (2012) 5:668. doi: 10.1186/1756-0500-5-668
13. China, GOOT. Technical guidelines for prevention and control of human granulocytic anaplasmosis (provisional). Chin J Exp Clin Inf Dis. (2010) 4:350–65.
14. Hong-Ze, Z, Jun-Jie, Z, Dan, Y, Ru-Dan, H, Mei, H, Zheng-Xiang, L, et al. Investigation of Anaplasma phagocytophilum infection in wild small mammals in the plague foci of Lianghe county, Yunnan province. Chin J Zoonoses. (2019) 35:1057–62. doi: 10.3969/j.issn.1002-2694.2019.00.164
15. Wang, F, Ma, M, Luo, S, Yan, M, Tao, L, Liu, A, et al. Seroprevalence of tick-borne Anaplasma phagocytophilum infection in healthy adult population and patients with acute undifferentiated fever from the Yunnan province of China. Vector Borne Zoonotic Dis. (2019) 19:576–81. doi: 10.1089/vbz.2018.2389
16. Ming-Guo, Y, Jia-Fu, J, Zi-Hou, G, Na, J, Li, T, Zhi-Hai, H, et al. Molecular epidemiological investigation of anaplasma in domestic in the northwest of Yunnan province, China. Chin J Zoonoses. (2020) 36:678–84. doi: 10.3969/j.issn.1002-2694.2020.00.103
17. Yu-Hui, C, Yan-Hong, W, Qun, X, Zhi-Fen, Y, Rui-Yun, H, Ai-Hua, L, et al. Serological seroepidemiological investigation of Anaplasma phagocytophilum in newly-enrolled college students in Yunnan province. China Med Pharm. (2015) 5:214–6.
18. Zhang, L, Liu, H, Xu, B, Zhang, Z, Jin, Y, Li, W, et al. Rural residents in China are at increased risk of exposure to tick-borne pathogens Anaplasma phagocytophilum and Ehrlichia chaffeensis. Biomed Res Int. (2014) 2014:313867. doi: 10.1155/2014/313867
19. Kim, YJ, Seo, JY, Kim, SY, and Lee, HI. Molecular detection of Anaplasma phagocytophilum and ehrlichia species in ticks removed from humans in the republic of Korea. Microorganisms. (2022) 10:1224. doi: 10.3390/microorganisms10061224
20. Perez, G, Bastian, S, Chastagner, A, Agoulon, A, Plantard, O, Vourc'H, G, et al. Ecological factors influencing small mammal infection by Anaplasma phagocytophilum and Borrelia burgdorferi s.l. in agricultural and forest landscapes. Environ Microbiol. (2017) 19:4205–19. doi: 10.1111/1462-2920.13885
21. Chvostac, M, Spitalska, E, Vaclav, R, Vaculova, T, Minichova, L, and Derdakova, M. Seasonal patterns in the prevalence and diversity of tick-borne Borrelia burgdorferi sensu lato, Anaplasma phagocytophilum and Rickettsia spp. in an urban temperate forest in south western Slovakia. Int J Environ Res Public Health. (2018) 15:994. doi: 10.3390/ijerph15050994
22. Shi, L, Qin, J, Zheng, H, Guo, Y, Zhang, H, Zhong, Y, et al. New genotype of Yersinia pestis found in live rodents in Yunnan province, China. Front Microbiol. (2021) 12:628335. doi: 10.3389/fmicb.2021.628335
Keywords: Anaplasma phagocytophilum , small mammals, genetic diversity, Yunnan province, infection rate
Citation: Zhu J-j, Zhang H-z, Hong R-d, Yu D, Hong M, Liu Z-x, Li D-m and Yin J-x (2024) Prevalence and genetic diversity of Anaplasma phagocytophilum in wild small mammals from western Yunnan province, China. Front. Vet. Sci. 11:1472595. doi: 10.3389/fvets.2024.1472595
Edited by:
Fabrizio Bertelloni, University of Pisa, ItalyReviewed by:
Marina Žekić, Scientific Veterinary Institute Novi Sad, SerbiaIngo Schäfer, Laboklin GmbH & Co. KG, Germany
Copyright © 2024 Zhu, Zhang, Hong, Yu, Hong, Liu, Li and Yin. This is an open-access article distributed under the terms of the Creative Commons Attribution License (CC BY). The use, distribution or reproduction in other forums is permitted, provided the original author(s) and the copyright owner(s) are credited and that the original publication in this journal is cited, in accordance with accepted academic practice. No use, distribution or reproduction is permitted which does not comply with these terms.
*Correspondence: Jia-xiang Yin, Y2hpbmF5anhAaG90bWFpbC5jb20=
†These authors have contributed equally to this work and share first authorship