- 1Laboratory of Biodiversity and Genetic Resources, National Center for Biotechnology, Astana, Kazakhstan
- 2Laboratory of Parasitology, Department of Veterinary Medicine, S. Seifullin Kazakh Agrotechnical Research University, Astana, Kazakhstan
- 3Consortium of Hunting, Tourist and Fishing Farms "Adal Zher", Temirtau, Kazakhstan
- 4Institute of Parasitology, Justus Liebig University Giessen, Giessen, Germany
Introduction: The presence of gastrointestinal nematodes, including zoonotic ascarids, in wild canids, felids and mustelids as definitive hosts in Central Asian countries has been documented in many studies based on traditional morphological methods. In contrast, relevant data for the badger are scarce. The aim of this study was the molecular identification of ascarid nematodes from five wild carnivore species in different regions of Kazakhstan.
Methods: A total of 211 adult ascarids were collected from gray wolves (Canis lupus, 8 of 83 infected with 2–6 Toxascaris leonina), red foxes (Vulpes vulpes, 26 of 53, with 2–8 Toxascaris leonina), corsac foxes (Vulpes corsac, 6 of 11, 3–6 Toxascaris leonina), lynx (Lynx lynx, 2 of 3, with 2–5 Toxocara cati) and badgers (Meles meles, 2 of 4, with 2–7 Baylisascaris melis). Genomic DNA was extracted from the worms and ribosomal DNA, including the first and second internal transcribed spacer genes, was amplified by polymerase chain reaction using specific oligonucleotide primers and then sequenced.
Results: Toxascaris leonina, but not Toxocara canis, was molecularly identified in the wild canids, Toxocara cati in the lynx and Baylisascaris melis in the badger. The maximum likelihood phylogenetic tree showed three distinct clades: the canid Toxascaris leonina was placed in one clade, Toxocara cati in another and Baylisascaris melis in a third.
Discussion: The study provides the world’s first molecular data and phylogenetic analysis of Baylisascaris melis, identified for the second time since its description over 100 years ago. This species was shown to be genetically distinct from other Baylisascaris spp. (B. columnaris, B. procyonis, B. transfuga, B. devosi). The possible zoonotic significance of ascarids from wild carnivores is discussed in the light of conditions in Central Asia.
1 Introduction
Members of the genera Toxocara, Toxascaris, and Baylisascaris comprise the spectrum of ascarid nematodes (order Ascaridida: family Ascarididae) of terrestrial mammals, including the carnivores Canidae, Felidae, and Mustelidae (1, 2). Their adult stages parasitize the small intestines of the definitive host, which contaminates the environment by excreting worm eggs in feces. The eggs embryonate, can survive for months or years, and are ingested by another animal. Paratenic hosts (e.g., in Toxocara spp.) or intermediate hosts (in Baylisascaris spp.) may be facultatively involved, e.g., prey rodents. After oral ingestion of infective eggs, larvae penetrate the intestinal mucosa and migrate to the liver and other tissues, including the brain (3, 4). The infection can also be transmitted to humans (known as ‘toxocariasis’) (5). For example, the seroprevalence of toxocariasis in humans has been reported to be 11% in eastern Kazakhstan (6) and up to 54% in western Siberian regions of Russia (7). Depending on the ascarid species and the number of eggs ingested, the infection may be latent, but may also cause clinical symptoms (larva migrans syndrome) (4, 8). Contamination of the environment with ascarid eggs by domestic and wild carnivores is known in principle (4, 9, 10), but its impact in Central Asia is still unknown.
A number of studies have documented the occurrence and prevalence of helminth infections, including ascarids, in wild canids and felids in Kazakhstan [(e.g., 11–14)] and neighboring countries [(e.g., 15–19)]. In these studies, for example, wolves and red foxes were infected with Toxocara canis in 39% and 8–30% respectively, and with Toxascaris (T.) leonina in 38% and 6–78% respectively; Toxocara cati was present in 86% of lynx. In contrast, there are only two reports on the helminth fauna of badgers from Uzbekistan (17, 18), but no data from Kazakhstan. All these studies were carried out using traditional morphological methods. However, in field studies where the species identification of roundworms is based solely on their morphological features, the diagnosis is sometimes at least questionable, e.g., in badger (18–20). These diagnostic problems can be solved using molecular methods that have been available for many years. Such methods confirm or modify the taxonomic classification and can also be used to study the phylogenetic relationships of parasites such as ascarids, detect their genetic diversity and explain epidemiological results [(e.g., 2, 21–24)]. Therefore, the aim of the present study was to molecularly confirm the morphological species diagnosis of roundworms from five wild carnivore species in different regions of Kazakhstan, including wolf, red fox, corsac fox, lynx and badger, and to provide baseline data for future investigations.
2 Materials and methods
2.1 Ethical approval
The study had been approved by the local Animal Ethics Committee (extract from Protocol No. 1 dated 24 July 2019) prior to commencement and was conducted in accordance with the World Medical Association Code of Ethics (Declaration of Helsinki) for animal research.1
2.2 Sample collection
Adult wild carnivores, including 83 gray wolves (Canis lupus), 53 red foxes (Vulpes vulpes), 11 corsac foxes (Vulpes corsac), 3 European lynx (Lynx lynx) and 4 badgers (Meles meles) were available for this study. They had been shot by hunters in different regions of Kazakhstan (Figure 1) between December 2019 and October 2023. The gastrointestinal tract of each animal, frozen until examination, was examined for helminths as described by Skrjabin (25). Adult roundworms were collected, washed in physiological saline, morphologically identified to species (26, 27) and preserved in 70% ethanol.
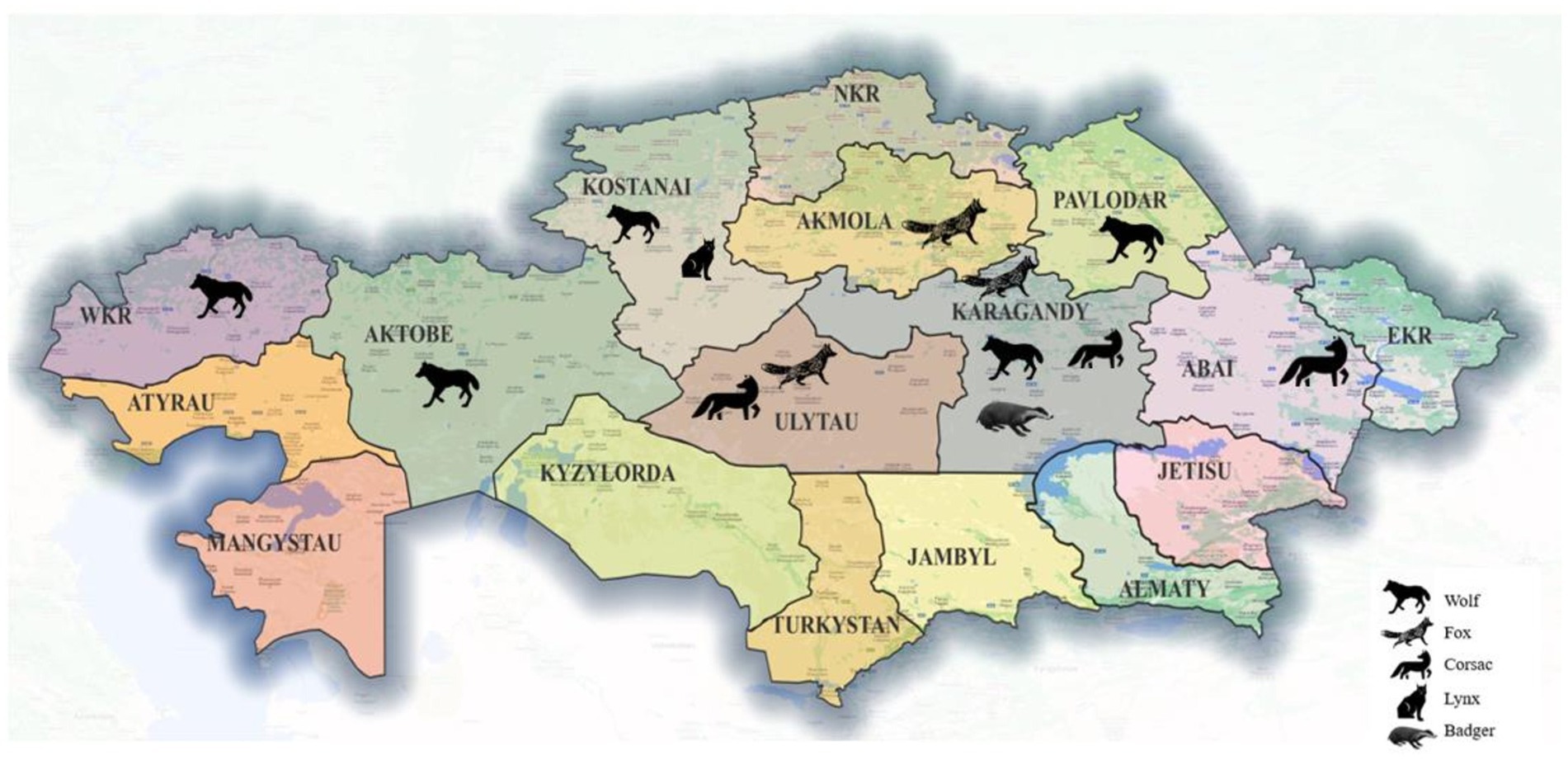
Figure 1. Map of Kazakhstan showing the provinces and the geographical origin of the host species collected.
2.3 DNA extraction
Following morphological specification, one worm from each ascarid-positive animal was randomly selected for molecular analysis. A small piece of this specimen was cut off and homogenized, and the homogenate was subjected to the standard phenol-chloroform method supplemented with proteinase K, to extract genomic DNA (gDNA). The DNA was then precipitated with ethanol (28), purified, dissolved in ddH2O and stored at −70°C for subsequent analysis.
2.4 PCR analysis
First, a polymerase chain reaction (PCR) was performed using the universal NC13/NC2 primer pair to amplify worm gDNA (21). PCR was performed in a 25 μL reaction mixture containing 10× Taq buffer with (NH4)2SO4, 2.5 mM MgCl2, 1 U Taq DNA polymerase and 200 μM dNTPs (Thermo Scientific, Carlsbad, CA, USA), 10 pmol of each primer and 20 ng of extracted gDNA as a template. DNA segments were amplified using thermal cycling reactions for 30 cycles of denaturation (94°C for 30 s), annealing (55°C for 30 s) and extension (72°C for 30 s). The resulting amplification products were separated by electrophoresis on a 1.5% agarose gel prepared with 1× TAE buffer solution containing 8 ng/μL ethidium bromide. This was followed by species-specific PCR targeting the partial internal transcribed spacer 2 (ITS2) ribosomal DNA (rDNA) gene of Toxocara canis, Toxocara cati and T. leonina using the primer pairs Tcan1/NC2, Tcat1/NC2 and Tleo1/NC2, respectively, (21). All PCRs were performed as described by Jacobs et al. (21). For the identification of Baylisascaris sp. a primer pair targeting the ITS1-5.8S-ITS2 rDNA genes was used under the conditions described by Franssen et al. (29). The sequences of all primers used are shown in Table 1.
2.5 Sequencing analysis and phylogeny
Two positive amplification products were randomly selected from each host species for sequencing and genotyping. The respective amplicons were purified using a Quick PCR Purification Kit (Invitrogen, Lithuania) according to the manufacturer’s protocols. Sequencing was performed according to the Seq Studio Genetic Analyzer manual (Thermo Fisher Scientific Applied Biosystems, USA). The nucleotide sequences were visually checked using the Bio Capt program (version 11.0) and then analyzed by BLAST search against the GenBank database.2 Finally, the nucleotide sequences were aligned using the Clustal W program, and the relationships of the taxa were analyzed with 1,000 bootstrap replicates by the maximum likelihood method with MEGA11 (30). For the inference method, the nearest neighbor Interaction (NNI) was used. The tree for Baylisascaris species was rooted by the outgroup Anisakis nascettii (JX486104).
2.6 Statistical analysis
Explorative data analysis was performed using the BIAS statistical software (31). The observed prevalence, mean intensity and abundance of each ascarid species were calculated as described by Bush et al. (32).
3 Results
A total of 211 adult ascarids were collected from 154 host animals. Based on morphology, three species were identified: wolves (9.6% infected), red foxes (49.1%) and corsac foxes (55%) were infected only with T. leonina, lynx (66%) and badgers (50%) were infected only with Toxocara cati and Baylisascaris (B.) melis, respectively. Their mean intensity and abundance were low (Table 2). Adult Toxocara canis were not found in any of the hosts.
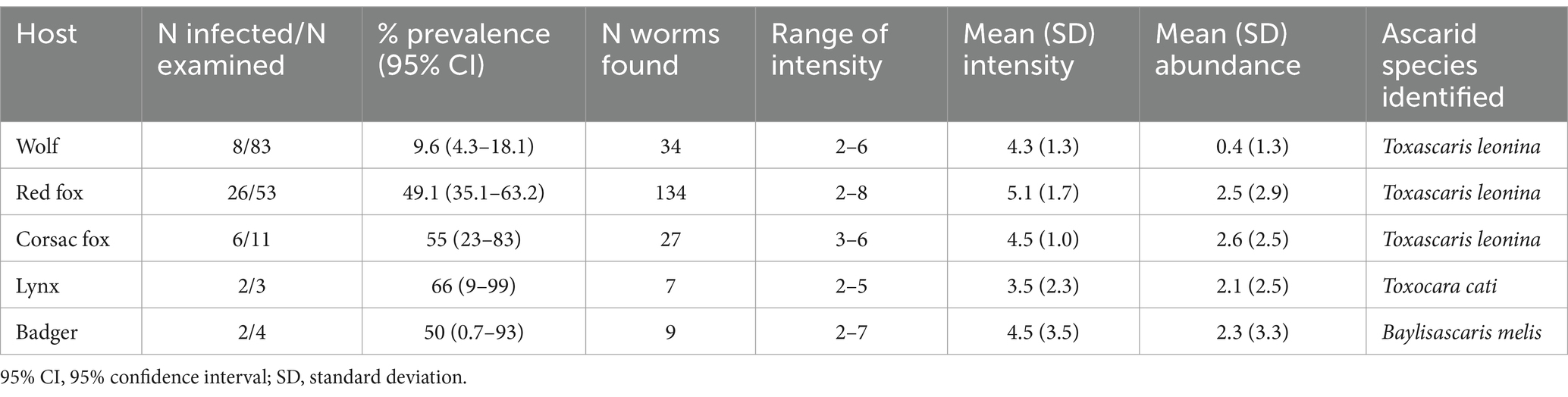
Table 2. Prevalence, intensity and abundance of adult ascarid species on the basis of morphology in wild carnivores in Kazakhstan.
The first PCR performed with the universal primer pair NC13/NC2 showed that the length of the PCR products from the ascarids of canids (wolf, red fox, and corsac fox) was different from that of the PCR products from the worms of lynx and badger (Figure 2).
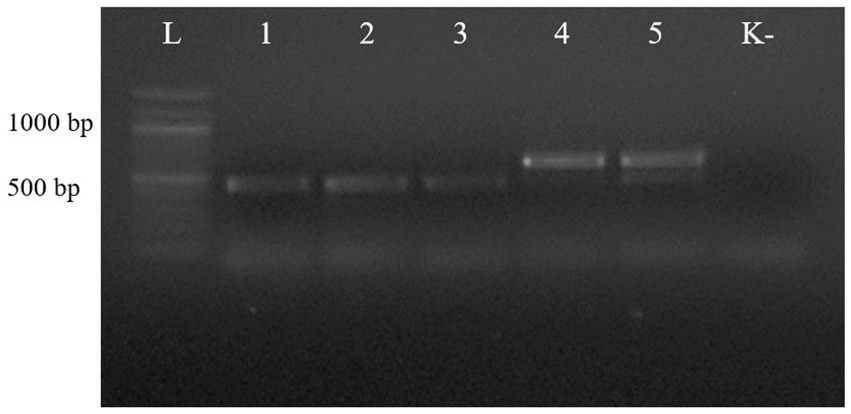
Figure 2. Electrophoresis of PCR products of gDNA from representative ascarid samples using the universal primer pair NC13/NC2: lane L: DNA marker; lanes 1–5: gDNA from ascarids collected from red fox (1), wolf (2), corsac fox (3), lynx (4) and badger (5); lane K: negative control (ddH2O).
The second PCR, performed with the respective species-specific primer pairs targeting the ITS2 rDNA region, identified T. leonina in canids and Toxocara cati in lynx (Figure 3). The primer pair specific for Toxocara canis gave no results in any sample (data not shown). Ribosomal ITS2 amplicons were obtained from six T. leonina isolates (232–261 bp), two each from wolf, red fox and corsac fox, and from two Toxocara cati isolates (375 and 434 bp) from lynx. The badger ascarids were identified as Baylisascaris sp. using a primer on the ribosomal ITS1-5.8S-ITS2 region and by comparison of the nucleotide sequences obtained with references from the GenBank database. Ribosomal ITS1-5.8S-ITS2 amplicons of 511 bp and 842 bp in length were obtained from two B. melis isolates. Nucleotide sequence data for all isolates have been deposited in the NCBI GenBank database under the accession numbers shown in Table 3.
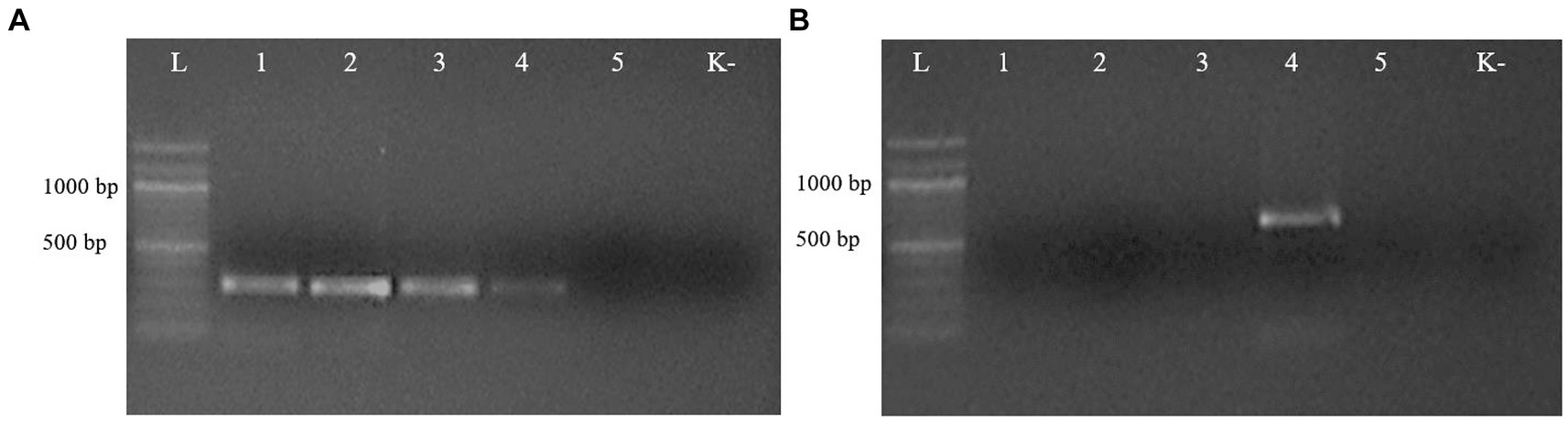
Figure 3. Electrophoresis of PCR products of gDNA from representative ascarid samples using the primer pairs Tleo1/NC2 (A) and Tcat1/NC2 (B), species-specific for Toxascaris leonina and Toxocara cati, respectively. Lane L: DNA marker; lanes 1–5: DNA from ascarids collected from red fox (1), wolf (2), corsac fox (3), lynx (4) and badger (5); lane K: negative control (ddH2O).
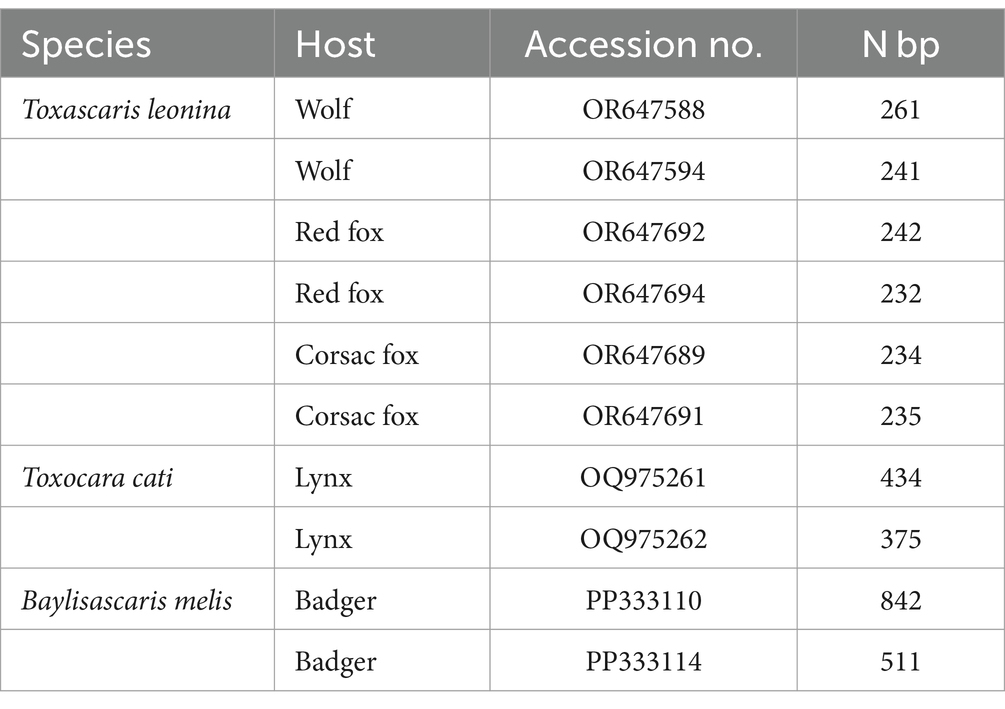
Table 3. GenBank accession no. and number of nucleotide base pairs of representative samples of adult ascarids from this study.
Nucleotide sequences from representative ascarid samples of the five host species were used to construct the maximum likelihood phylogenetic tree. Three distinct clades were identified: T. leonina from canids was placed in one clade with bootstrap values ranging from 46 to 96, Toxocara cati from lynx in another and B. melis from badgers in a third (Figure 4). Maximum tree analyses of the ribosomal ITS1-5.8S-ITS2 gene sequence showed that the two B. melis isolates formed a clade with the four reference species Baylisascaris columnaris, Baylisascaris procyonis, Baylisascaris transfuga, and Baylisascaris devosi. Both B. melis isolates showed slight genetic differences (Figure 5).
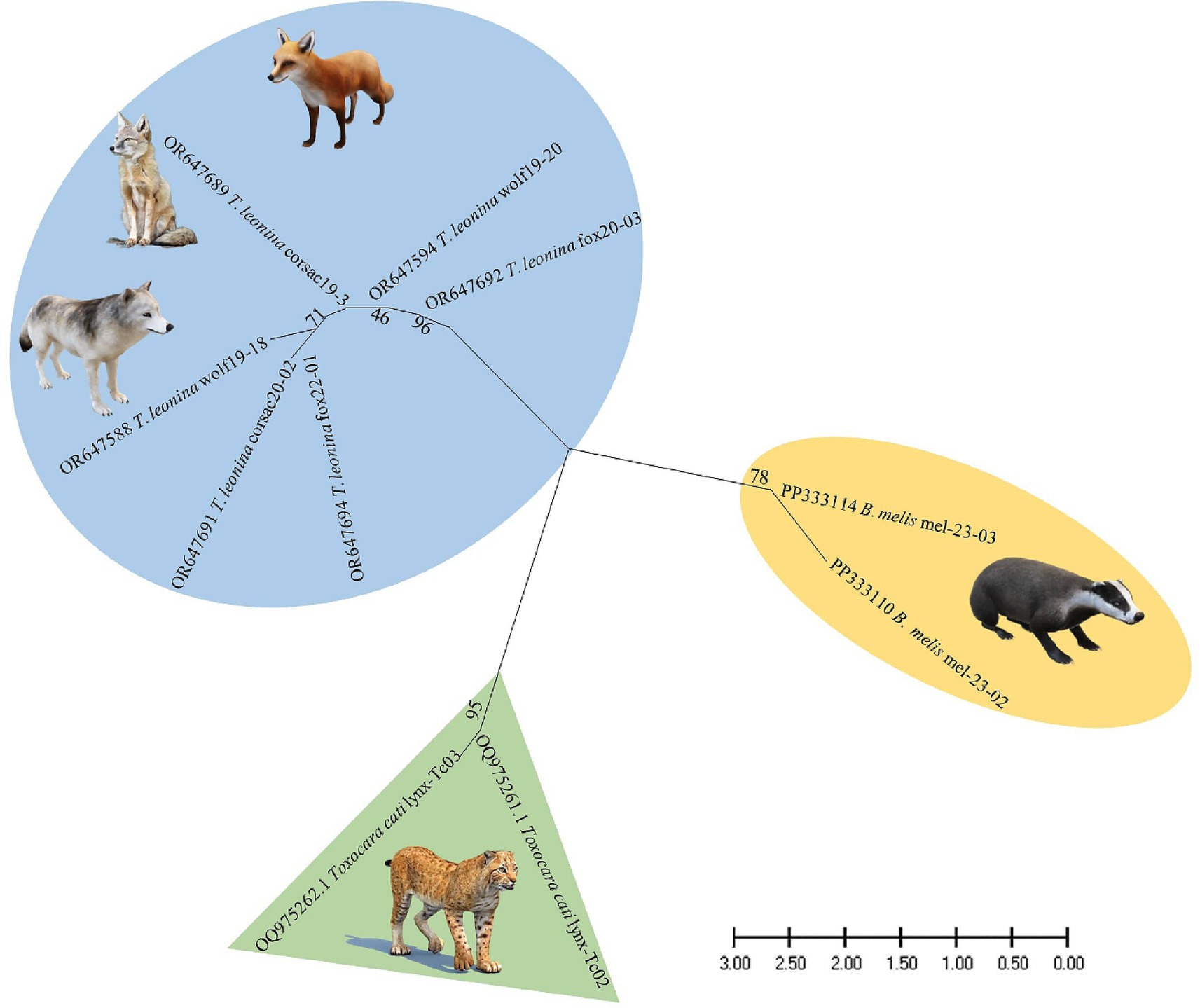
Figure 4. Maximum likelihood phylogeny constructed from nucleotide data of representative ascarid samples from wild carnivores in Kazakhstan. Numbers along the branches show bootstrap values resulting from different analyses in percent; scale: estimated number of nucleotide substitutions per site; species name and host species after the GenBank accession no.
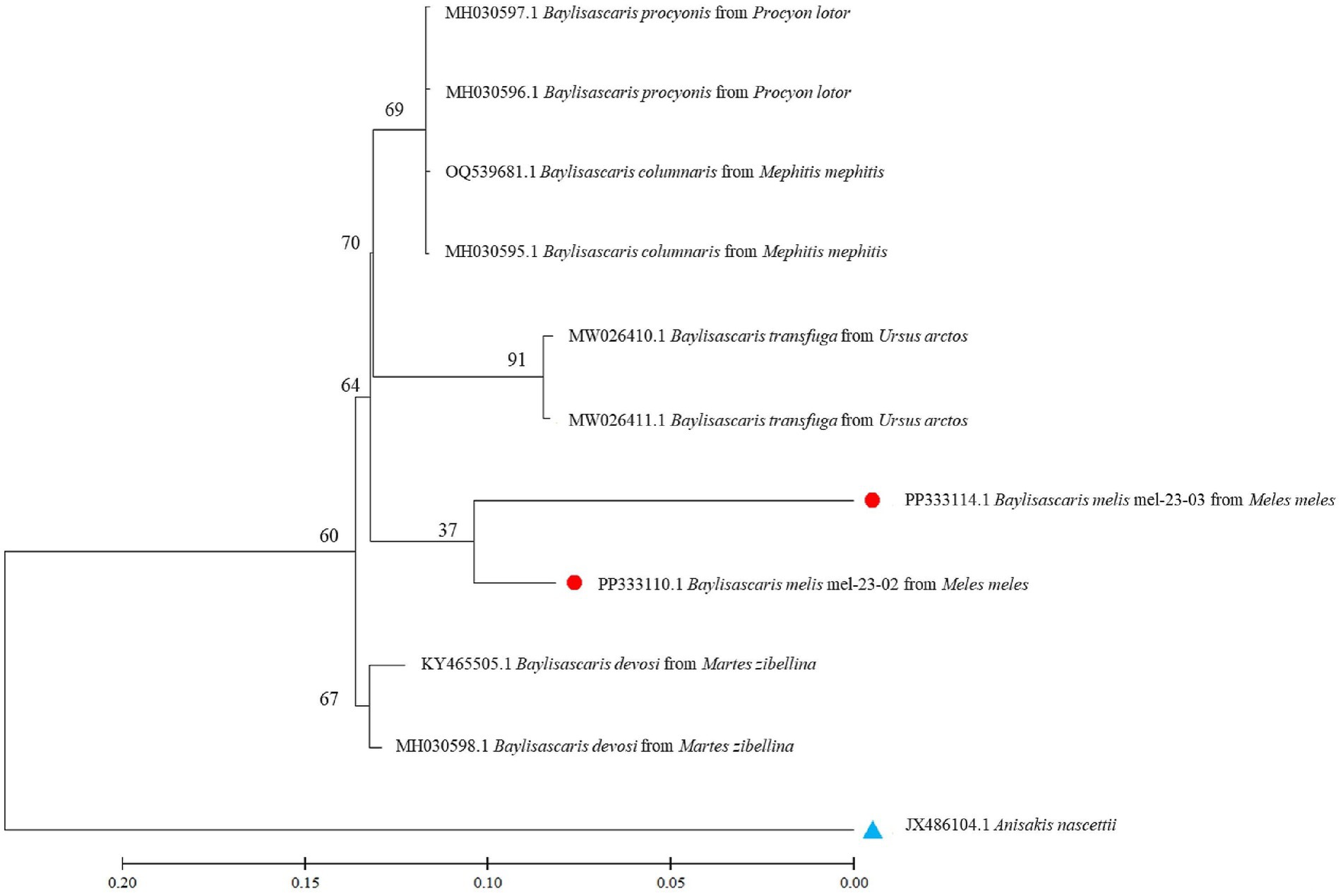
Figure 5. Maximum likelihood tree based on ribosomal ITS1-5.8S-ITS2 gene regions from several Baylisascaris spp. available in the GenBank and two isolates from this study. Numbers along the branches show bootstrap values resulting from different analyses in percent; scale: estimated number of nucleotide substitutions per site; species name and host species after the GenBank accession no.; red dots: isolates from this study; blue triangle: outgroup.
4 Discussion
In this study, five wild carnivore species in Kazakhstan were examined for their respective ascarid species. The species found, their prevalence, intensity and abundance partly differ from those of other Kazakh studies. This is not surprising as the regions of origin of the sampled hosts were different. It should also be noted that the data presented (as from previous studies) are not representative. They are based on a relatively small number of non-randomly selected hosts in a few regions of Kazakhstan, a large country of 2,725,000 km2, where, for example, the wolf and red fox populations are estimated to be 30,000 and 75,000, respectively, (33, 34). It is also well documented that the ascarid fauna of wild carnivores varies between landscapes (e.g., steppe, foothills, mountains) (19, 35, 36), which may be explained by local differences in prey availability (10). Furthermore, lynx are protected species and their killing requires justified exemptions. It is therefore quite difficult to study representative samples of these wild carnivores in such large countries.
Nevertheless, it is the first study to use molecular methods to identify ascarid nematodes from Central Asian countries. Phylogenetic analysis revealed three distinct species: Toxocara cati, T. leonina, and B. melis (Figure 4), confirming the morphological diagnosis.
In the three canid hosts, only T. leonina was identified, but not Toxocara canis. This is consistent with previous findings, based on traditional morphological methods, that T. leonina was the dominant ascarid species in corsac foxes in Kazakhstan (11), wild canids in southern Siberia (15), and stray dogs in Eurasian regions (37). It may be due to the higher cold tolerance of T. leonina eggs compared to Toxocara canis eggs, which favors this roundworm species in colder regions (37). However, it should be noted that the worms in the present study were obtained from adult hosts. This may have biased the results, as Toxocara canis is known to be mainly found in young canids (1, 26). In fact, other studies in Kazakhstan and neighboring countries have reported that wolves, red foxes or corsac foxes are infected with both ascarid species (12–14, 16–18).
Toxocara cati was the only ascarid species found in lynx. This was consistent with most reports from different countries (10, 15, 18), although occasionally T. leonina was also reported from this felid species (2, 38).
Wild canids and felids (as well as their domestic relatives) infected with ascarids contaminate the environment by excreting worm eggs in feces. The embryonated eggs are a potential source of infection for domestic dogs and cats and for paratenic hosts, including humans, who may ingest these eggs (1, 9, 10). In the light of the results presented, this infection risk can be assessed as follows: (a) T. leonina is considered a negligible parasite from a veterinary and zoonotic point of view (37). (b) In contrast, Toxocara cati is pathogenic in its definitive feline host (39) and also in paratenic hosts: Experimental studies have shown that after ingestion of Toxocara cati eggs, larvae migrate into tissues, including the brain, causing pathomorphological alterations in mice and pigs and abnormal neurobehaviour in mice (40, 41). It should therefore be considered as a potential cause of neural larval migrans symptoms in humans (42). However, lynx are likely to be a negligible source of Toxocara cati infection to humans, at least in Central Asia. This is because the lynx prefers to live in forested areas, which provide sufficient cover for hunting and abundant prey without much contact with human settlements (43). (c) Toxocara canis may be present in wolves and red foxes (see above), although not in this study. These wild canids are more synanthropic than the lynx, and their range extends close to human settlements (10, 44). This increases the risk of successful transmission of their parasites, including the zoonotic Toxocara canis, to domestic animals and humans (1, 9, 10).
This study also presents the first molecular data and provides the first phylogenetic analysis of B. melis worldwide. The badger ascarid was shown to be genetically distinct from Baylisascaris spp. of other carnivores: B. columnaris (definitive host: skunk [Mephitis spp.]), B. procyonis (raccoon [Procyon lotor]), B. transfuga (bears [Ursus spp.]) and B. devosi (marten [Martes spp.], fisher [Pekania pennanti], wolverine [Gulo gulo]) (Figure 5). This also confirms the morphological differentiation by Sprent (45) and supports the hypothesis (46) that the ascarids found in North American badgers (Taxidea taxus), which have been described as B. columnaris, are in fact B. melis. The significance of the slight genetic differences between the two B. melis isolates analyzed remains to be investigated. The phylogenetic analysis also showed that B. procyonis and B. columnaris form a clade. This confirms previous results suggesting that they are closely related species or that the former is even a synonym of the latter (47, 48).
Interestingly, there is little information on the geographical distribution and prevalence of B. melis in badger populations in Eurasia. First described over 100 years ago in Belgium (49), this is the second unequivocal identification of this species. This nematode had not been mentioned in any relevant study in central, western or southern European countries. There are two studies from Italy and Switzerland reporting only unspecified “ascarid” eggs or worms in a few badgers (Table 4). In contrast, ascarids have been collected from badgers in Uzbekistan, Azerbaijan and Caucasian Russia and morphologically identified as Toxocara canis, B. columnaris or B. devosi (Table 4). However, it is most likely that these worms were misidentified and were actually B. melis; the molecular results support this assumption. Thus, data from the literature and the results presented here suggest that B. melis may occur primarily, if not exclusively, in badger populations of western and central Asia. The reasons for this are still unknown.
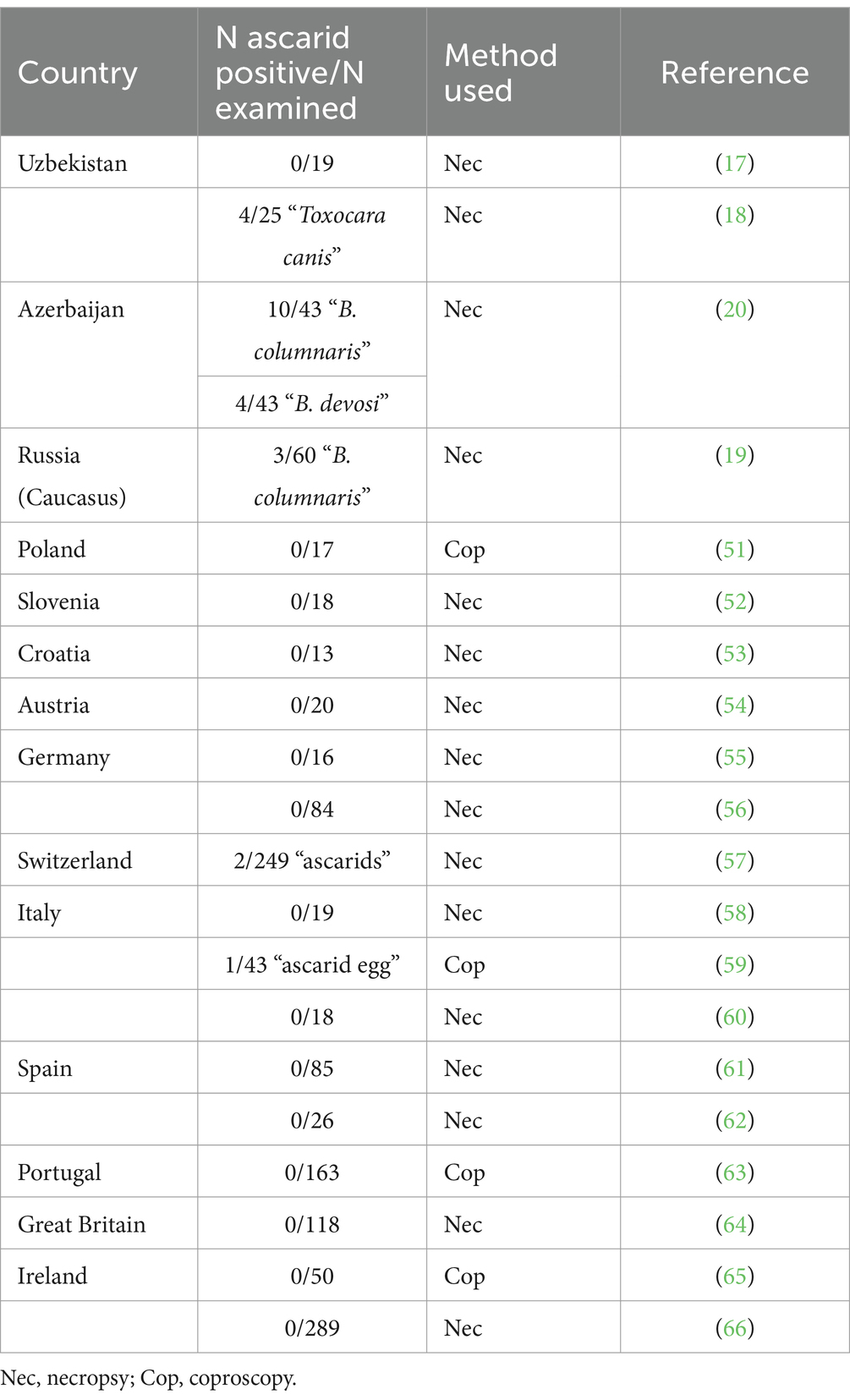
Table 4. Results of previous studies on intestinal helminths, including ascarids, in badgers in Eurasia.
It should be noted that B. melis is able to infect rodents (facultative intermediate hosts) under experimental conditions: It was highly pathogenic and caused fatal neural larva migrans symptoms in the American ground squirrel (Urocitellus armatus); mice (Mus musculus) did not develop clinical symptoms, but their brains and other tissues contained B. melis larvae (50). Whether this can also occur in Central Asian ground squirrel species (Spermophilus spp.) or other rodents under natural conditions does not seem impossible and requires further study. In any case, based on the clinical and pathological findings in rodents, a zoonotic significance of B. melis cannot be excluded and should be further investigated.
This study concludes by identifying ascarid nematodes from five distinct wild carnivore species in Central Asia within the phylogenetic framework. The study also presents the world’s first molecular data on B. melis from badger. It provides further insights into the classification and genetic diversity of ascarids. It reiterates the need for molecular methods to complement traditional morphological methods as a basic diagnostic tool in the future, for example in studies of the fauna, diversity, ecology and epidemiology of wildlife parasites, especially potential zoonotic agents. For future research, we are also considering collecting feces from wild carnivores to detect roundworm infection, which would increase the sample size.
Data availability statement
The datasets presented in this study can be found in online repositories. The names of the repository/repositories and accession number(s) can be found at: https://www.ncbi.nlm.nih.gov/nuccore/OR647588, OR647588; https://www.ncbi.nlm.nih.gov/nuccore/OR647594, OR647594; https://www.ncbi.nlm.nih.gov/nuccore/OR647692, OR647692; https://www.ncbi.nlm.nih.gov/nuccore/OR647694, OR647694; https://www.ncbi.nlm.nih.gov/nuccore/OR647689, OR647689; https://www.ncbi.nlm.nih.gov/nuccore/OR647691, OR647691; https://www.ncbi.nlm.nih.gov/nuccore/OQ975261, OQ975261; https://www.ncbi.nlm.nih.gov/nuccore/OQ975262, OQ975262; https://www.ncbi.nlm.nih.gov/nuccore/PP333110, PP333110; https://www.ncbi.nlm.nih.gov/nuccore/PP333114, PP333114.
Ethics statement
The animal study was approved by the local Animal Ethics Committee (extract from Protocol No. 1 dated 24 July 2019) prior to commencement and was conducted in accordance with the World Medical Association Code of Ethics (Declaration of Helsinki) for animal research (http://ec.europa.eu/environment/chemicals/lab_animals/legislation_en.htm). The study was conducted in accordance with the local legislation and institutional requirements.
Author contributions
RU: Data curation, Formal analysis, Methodology, Software, Validation, Visualization, Writing – original draft, Writing – review & editing. ASm: Data curation, Formal analysis, Investigation, Methodology, Software, Validation, Visualization, Writing – original draft, Writing – review & editing. LL: Data curation, Formal analysis, Methodology, Validation, Writing – original draft, Writing – review & editing. ASh: Data curation, Formal analysis, Methodology, Software, Writing – original draft, Writing – review & editing. AAB: Data curation, Formal analysis, Visualization, Writing – original draft, Writing – review & editing. APB: Data curation, Investigation, Methodology, Software, Writing – original draft, Writing – review & editing. CB: Conceptualization, Formal analysis, Investigation, Methodology, Resources, Software, Supervision, Validation, Visualization, Writing – original draft, Writing – review & editing. VK: Conceptualization, Data curation, Formal analysis, Funding acquisition, Methodology, Project administration, Resources, Supervision, Writing – original draft, Writing – review & editing.
Funding
The author(s) declare that financial support was received for the research, authorship, and/or publication of this article. This research was financially supported by the Ministry of Education and Science of the Republic of Kazakhstan under project #AP08052252 for 2020–2022. The molecular genetic work was also supported by the Initiative Scientific Project #0121PKI0192 for 2021–2024, Kazakhstan.
Acknowledgments
The authors are grateful to Alexander Lyalchenko for collecting a number of host animals. We also thank our master students for their help in identifying and counting the parasites.
Conflict of interest
The authors declare that the research was conducted in the absence of any commercial or financial relationships that could be construed as a potential conflict of interest.
Publisher’s note
All claims expressed in this article are solely those of the authors and do not necessarily represent those of their affiliated organizations, or those of the publisher, the editors and the reviewers. Any product that may be evaluated in this article, or claim that may be made by its manufacturer, is not guaranteed or endorsed by the publisher.
Footnotes
References
1. Otranto, D, and Deplazes, P. Zoonotic nematodes of wild carnivores. Int J Parasitol Parasit Wildl. (2019) 9:370–83. doi: 10.1016/j.ijppaw.2018.12.011
2. Xie, Y, Li, Y, Gu, X, Liu, Y, Zhou, X, Wang, L, et al. Molecular characterization of ascaridoid parasites from captive wild carnivores in China using ribosomal and mitochondrial sequences. Parasit Vectors. (2020) 13:382. doi: 10.1186/s13071-020-04254-4
3. Wu, T, and Bowman, DD. Visceral larval migrans of Toxocara canis and Toxocara cati in non-canid and non-felid hosts. Adv Parasitol. (2020) 109:63–88. doi: 10.1016/bs.apar.2020.02.001
4. Kazacos, KR. Baylisascaris larva Migrans. Reston: U.S. Geological Survey Circular 1412 (2016). 122 p.
5. Ma, G, Rostami, A, Wang, T, Hofmann, A, Hotez, PJ, and Gasser, RB. Global and regional seroprevalence estimates for human toxocariasis: a call for action. Adv Parasitol. (2020) 109:275–90. doi: 10.1016/bs.apar.2020.01.011
6. Torgerson, PR, Rosenheim, K, Tanner, I, Ziadinov, I, Grimm, F, Brunner, M, et al. Echinococcosis, toxocarosis and toxoplasmosis screening in a rural community in eastern Kazakhstan. Trop Med Int Health. (2009) 14:341–8. doi: 10.1111/j.1365-3156.2009.02229.x
7. Akhmadishina, LV, Ruzina, MN, Lukasheva, MA, Kyuregyan, KK, Mikhailov, MI, and Lukashev, AN. Seroprevalence and incidence of human toxocarosis in Russia. Adv Parasitol. (2020) 109:419–32. doi: 10.1016/bs.apar.2020.01.015
8. Auer, H, and Walochnik, J. Toxocariasis and the clinical spectrum. Adv Parasitol. (2020) 109:111–30. doi: 10.1016/bs.apar.2020.01.005
9. Otranto, D, Cantacessi, C, Dantas-Torres, F, Brianti, E, Pfeffer, M, Genchi, C, et al. The role of wild canids and felids in spreading parasites to dogs and cats in Europe. Part II: helminths and arthropods. Vet Parasitol. (2015) 213:24–37. doi: 10.1016/j.vetpar.2015.04.020
10. Holland, CV. A walk on the wild side: a review of the epidemiology of Toxocara canis and Toxocara cati in wild hosts. Int J Parasitol Parasit Wildl. (2023) 22:216–28. doi: 10.1016/j.ijppaw.2023.10.008
11. Tazieva, ZK. Helminths of predatory mammals (Canidae and Mustelidae) of Kazakhstan. (PhD Biol. Sci. thesis [abstract]). Alma-Ata: University (1970). 24 p In Russian.
12. Abdybekova, AM, and Torgerson, PR. Frequency distributions of helminths of wolves in Kazakhstan. Vet Parasitol. (2012) 184:348–51. doi: 10.1016/j.vetpar.2011.09.004
13. Abdybekova, A. About helminth fauna of corsac foxes inhabiting the south of Kazakhstan. GISAP Biol Vet Med Agricult Sci. (2014) 3:39–41.
14. Abirova, IM, Elsugalisva, NZ, Zhumagalisva, GK, and Gusmanov, MG. Helminthofauna of the fox (Vulpes vulpes) and corsac (Vulpes corsac). Biol Sci Kazakhstan. (2021) 3:28–35. In Kazakh
15. Bykova, AM. Helminths of predatory mammals (Canidae, Felidae, Mustelidae) in the Omsk region and their ecological-faunistic analysis. (PhD Biol. Sci. thesis [abstract]). Tyumen: All-Russian Scientific Research Institute Veterinary Entomology and Arachnology (2007). 21 p In Russian.
16. Ziadinov, I, Deplazes, P, Mathis, A, Mutunov, B, Abdykerimov, K, Nurgaziev, R, et al. Frequency distribution of Echinococcus multilocularis and other helminths of foxes in Kyrgyzstan. Vet Parasitol. (2010) 171:286–92. doi: 10.1016/j.vetpar.2010.04.006
17. Safarov, AA, Akramova, FD, Turgunov, SN, Saidova, SO, Mirzaeva, AU, Berdibaev, AS, et al. Fauna of helminths of predatory mammals (Canidae, Mustelidae, Felidae) of Uzbekistan. Sci Rev Biol Sci. (2023) 4:39–52. doi: 10.17513/srbs.1340, In Russian
18. Shakarboev, EB, and Berdibaev, AS. Ecological and faunistic analysis of helminths of wild mammals from the order Carnivora in Karakalpakstan. Adv Anim Vet Sci. (2023) 11:1801–9. doi: 10.17582/journal.aavs/2023/11.11.1801.180954
19. Itin, GS, and Kravchenko, VM. Helminthic cenoses of the Eurasian badger (Meles meles, L., 1758) in the North-Western Caucasus. Theory Pract Combat Parasitic Dis. (2023) 24:195–8. doi: 10.31016/978-5-6048555-6-0.2023.24.194-198
20. Fataliyev, GH. Helminthofauna of the badger (Meles meles L. 1758) and detection of new nematodes Trichocephalus sp. nov. Fataliyev, 2010 (Trichocephalata: Trichocephalidae) on the territory of the republic of Azerbaijan. For Chron. (2019) 4:7–11.
21. Jacobs, DE, Zhu, X, Gasser, RB, and Chilton, NB. PCR-based methods for identification of potentially zoonotic ascaridoid parasites of the dog, fox and cat. Acta Trop. (1997) 68:191–200. doi: 10.1016/s0001-706x(97)00093-4
22. Li, L, Lü, L, Nadler, SA, Gibson, DI, Zhang, LP, Chen, HX, et al. Molecular phylogeny and dating reveal a terrestrial origin in the early carboniferous for ascaridoid nematodes. Syst Biol. (2018) 67:888–900. doi: 10.1093/sysbio/syy018
23. Fogt-Wyrwas, R, Dabert, M, Jarosz, W, Rząd, I, Pilarczyk, B, and Mizgajska-Wiktor, H. Molecular data reveal cryptic speciation and host specificity in Toxascaris leonina (Nematoda: Ascarididae). Vet Parasitol. (2010) 266:80–3. doi: 10.1016/j.vetpar.2019.01.002
24. Fava, NMN, Cury, MC, Santos, HA, Takeuchi-Storm, N, Strube, C, Zhu, XQ, et al. Phylogenetic relationships among Toxocara spp. and Toxascaris sp. from different regions of the world. Vet Parasitol. (2020) 282:109133. doi: 10.1016/j.vetpar.2020.109133
25. Skrjabin, KI. Method of complete helminthological dissection of vertebrates, including humans. Moscow: State University (1928) 40–48. In Russian.
26. Deplazes, P, Eckert, J, Mathis, A, Samson-Himmelstjerna, V, and Zahner, H. Parasitology in veterinary medicine. Wageningen: Academic Publishers (2016). 653 p.
27. Kontrimavičius, VN. Helminths of mustelids and trends in their evolution. Moscow: Nauka (1969) 232–234. In Russian.
28. Bowles, J, and McManus, DP. Genetic characterization of the Asian Taenia, a newly described taeniid cestode of humans. Am J Trop Med Hyg. (1994) 50:33–44.
29. Franssen, F, Xie, K, Sprong, H, and van der Giessen, J. Molecular analysis of Baylisascaris columnaris revealed mitochondrial and nuclear polymorphisms. Parasit Vectors. (2013) 6:124. doi: 10.1186/1756-3305-6-124
30. Tamura, K, Stecher, G, and Kumar, S. MEGA11: molecular evolutionary genetics analysis version 11. Mol Biol Evol. (2021) 38:3022–7. doi: 10.1093/molbev/msab120
32. Bush, AO, Lafferty, KD, Lotz, JM, and Shostak, AW. Parasitology meets ecology on its own terms: Margolis Shostak AW revisited. J Parasitol. (1997) 83:575–83.
33. World Population Review. Gray wolf population by country. (2024). Available at: https://worldpopulationreview.com/country-rankings/gray-wolf-population-by-country (Accessed June 10, 2024).
34. World Population Review. Red fox population by country. (2024). Available at: https://worldpopulationreview.com/country-rankings/fox-population-by-country (Accessed June 10, 2024).
35. Fataliev, GG. Helminthofauna of wild canids in Azerbaijan and ways of its formation. Parazitologiya. (2011) 45:129–39. In Russian
36. Ponamarev, NM, and Kostyukov, MA. Study on helminths of red foxes in the Altai region. Bull Altay State Agrarian Univ. (2012) 1:57–9. In Russian
37. Bauer, C, Lider, LA, Ussenbayev, AE, Seitkamzina, DM, Zhanabayev, AA, Maksimov, P, et al. Toxascaris leonina in dogs – a nematode species of high prevalence in some regions of Eurasia. Vet Parasitol Reg Stud Rep. (2024) 48:100986. doi: 10.1016/j.vprsr.2024.100986
38. Kołodziej-Sobocińska, M, Yakovlev, Y, Schmidt, K, Hurníková, Z, Ruczyńska, I, Bednarski, M, et al. Update of the helminth fauna in Eurasian lynx (Lynx lynx) in Poland. Parasitol Res. (2018) 117:2613–21. doi: 10.1007/s00436-018-5953-0
39. Dillon, AR, Tillson, DM, Hathcock, J, Brawner, B, Wooldridge, A, Cattley, R, et al. Lung histopathology, radiography, high-resolution computed tomography, and bronchio-alveolar lavage cytology are altered by Toxocara cati infection in cats and is independent of development of adult intestinal parasites. Vet Parasitol. (2013) 193:413–26. doi: 10.1016/j.vetpar.2012.12.045
40. Janecek, E, Waindok, P, Bankstahl, M, and Strube, C. Abnormal neurobehaviour and impaired memory function as a consequence of Toxocara canis- as well as Toxocara cati-induced neurotoxocarosis. PLoS Negl Trop Dis. (2017) 11:E0005594. doi: 10.1371/journal.pntd.0005594
41. Poulsen, CS, Yoshida, A, Wellbrant, TT, Leifsson, PS, Skallerup, P, Thamsborg, SM, et al. Migratory pattern of zoonotic Toxocara cati and T. canis in experimentally infected pigs. Eur J Clin Microbiol Inf Dis. (2024) 43:587–96. doi: 10.1007/S10096-024-04753-7
42. Maciag, L, Morgan, ER, and Holland, C. Toxocara: time to let cati ‘out of the bag’. Trends Parasitol. (2022) 38:280–9. doi: 10.1016/j.pt.2021.12.006
43. Sidorovich, V. Behaviour and ecology of the Eurasian Lynx. Minsk: Publishing House Four Quarters (2022). 344 p.
44. Poyarkov, AD, Korablev, MP, Bragina, E, and Hernandez-Blanco, JA. Overview of current research on wolves in Russia. Front Ecol Evol. (2022) 10:869161. doi: 10.3389/fevo.2022.869161
45. Sprent, JFA. Notes on Ascaris and Toxascaris, with a definition of Baylisascaris gen. Nov. Parasitology. (1968) 58:185–98. doi: 10.1017/S0031182000073534
46. Sapp, SGH, Gupta, P, Martin, MK, Murray, MH, Niedringhaus, KD, Pfaff, MA, et al. Beyond the raccoon roundworm: the natural history of non-raccoon Baylisascaris species in the New World. Int J Parasitol Parasites Wildl. (2017) 6:85–99. doi: 10.1016/j.ijppaw.2017.04.003
47. Camp, LE, Radke, MR, Shihabi, DM, Pagana, C, Yang, G, and Nadler, SA. Molecular phylogenetics and species-level systematics of Baylisascaris. Int J Parasitol Parasit Wildl. (2018) 7:450–62. doi: 10.1016/j.ijppaw.2018.09.010
48. Gu, XH, Chen, HX, Hu, JJ, and Li, L. Morphology and ASAP analysis of the important zoonotic nematode parasite Baylisascaris procyonis (Stefahski and Zarnowski, 1951), with molecular phylogenetic relationships of Baylisascaris species (Nematoda: Ascaridida). Parasitology. (2024) 151:200–12. doi: 10.1017/S0031182023001312
49. Gedoelst, CR. Sur une espece nouvelle d’ascaride, parasite du blaireau. C R Seances Soc Biol Fil. (1920) 83:1291–2.
50. Tiner, JD. Fatalities in rodents caused by larval Ascaris in the central nervous system. J Mammal. (1953) 34:153–67. doi: 10.2307/1375616
51. Górski, P, Zalewski, A, and Lakomy, M. Parasites of carnivorous mammals in Białowieza primeval Forest. Wiad Parazytol. (2006) 52:49–53.
52. Brglez, J. Some endohelminths in badgers, Meles meles L., in Slovenia. Zbornik Biotehniške Fakultete Univerze Edvarda Kardelja v Ljubljani, Veterinarstvo. (1988) 25:251–7. In Slovenian
53. Bujanić, M, Martinković, F, Štimac, I, Škvorc, N, and Konjević, D. Gastrointestinal parasites of wild badger (Meles meles) at Medvednica Nature Park – preliminary results. Proc Int Symp Anim Sci. (2019) 30.
54. Baumann, T. Endoparasitenfauna des Dachses in Österreich. (MS Vet Med thesis). Vienna: University of Veterinary Medicine (2014). 61 p.
55. Stubbe, M. Zur Biologie der Raubtiere eines abgeschlossenen Waldgebietes. Z Jagdwiss. (1965) 11:73–102.
56. Loos-Frank, B, and Zeyhle, E. The intestinal helminths of the red fox and some other carnivores in Southwest Germany. Z Parasitenk. (1982) 67:99–113. doi: 10.1007/BF00929518
57. Akdesir, E, Origgi, FC, Wimmershoff, J, Frey, J, Frey, CF, and Ryser-Degiorgis, MP. Causes of mortality and morbidity in free-ranging mustelids in Switzerland: necropsy data from over 50 years of general health surveillance. BMC Vet Res. (2018) 14:195. doi: 10.1186/s12917-018-1494-0
58. Magi, M, Banchi, C, Barchetti, A, and Guberti, V. The parasites of the badger (Meles meles) in the north of Mugello (Florence, Italy). Parassitologia. (1999) 41:533–6.
59. Maestrini, M, Berrilli, F, Di Rosso, A, Coppola, F, Procesi, IG, Mariacher, A, et al. Zoonotic Giardia duodenalis genotypes and other gastrointestinal parasites in a badger population living in an anthropized area of Central Italy. Pathogens. (2022) 11:906. doi: 10.3390/pathogens11080906
60. Di Cerbo, AR, Manfredi, MT, Bregoli, M, Ferro Milone, N, and Cova, M. Wild carnivores as source of zoonotic helminths in North-Eastern Italy. Helminthologia. (2008) 45:13–9. doi: 10.2478/s11687-008-0002-7
61. Torres, J, Miquel, J, and Motjé, M. Helminth parasites of the Eurasian badger (Meles meles L.) in Spain: a biogeographic approach. Parasitol Res. (2001) 87:259263. doi: 10.1007/s004360000316
62. Millán, J, Sevilla, I, Gerrikagoitia, X, García-Pérez, AL, and Barral, M. Helminth parasites of the Eurasian badger (Meles meles L.) in the Basque Country (Spain). Eur J Wildl Res. (2004) 50:37–40. doi: 10.1007/s10344-003-0032-x
63. Rosalino, LM, Torres, J, and Santos-Reis, M. A survey of helminth infection in Eurasian badgers (Meles meles) in relation to their foraging behaviour in a Mediterranean environment in Southwest Portugal. Eur J Wildl Res. (2006) 52:202–6. doi: 10.1007/s10344-006-0033-7
64. Jones, GW, Neal, C, and Harris, EA. The helminth parasites of the badger (Meles meles) in Cornwall. Mamm Rev. (1980) 10:163–4. doi: 10.1111/j.1365-2907.1980.tb00237.x
65. Stuart, P, Golden, O, Zintl, A, De Waal, T, Mulcahy, G, McCarthy, E, et al. A coprological survey of parasites of wild carnivores in Ireland. Parasitol Res. (2013) 112:3587–93. doi: 10.1007/s00436-013-3544-7
Keywords: Baylisascaris melis , Toxascaris leonina , Toxocara cati , wild carnivores, mustelids, molecular identification, phylogeny, Kazakhstan
Citation: Uakhit R, Smagulova A, Lider L, Shevtsov A, Berber AA, Berber AP, Bauer C and Kiyan V (2024) Molecular identification of Baylisascaris melis (Gedoelst, 1920) from the Eurasian badger (Meles meles) and ascarids from other wild carnivores in Kazakhstan. Front. Vet. Sci. 11:1452237. doi: 10.3389/fvets.2024.1452237
Edited by:
Rodrigo Morchón García, University of Salamanca, SpainReviewed by:
Serena Cavallero, Sapienza University of Rome, ItalyAlfonso Balmori-de la Puente, University of Salamanca, Spain
Copyright © 2024 Uakhit, Smagulova, Lider, Shevtsov, Berber, Berber, Bauer and Kiyan. This is an open-access article distributed under the terms of the Creative Commons Attribution License (CC BY). The use, distribution or reproduction in other forums is permitted, provided the original author(s) and the copyright owner(s) are credited and that the original publication in this journal is cited, in accordance with accepted academic practice. No use, distribution or reproduction is permitted which does not comply with these terms.
*Correspondence: Vladimir Kiyan, dnNraXlhbkBnbWFpbC5jb20=