- 1Institute for Diagnostic and Interventional Radiology, Pediatric and Neuroradiology, Rostock University Medical Center, Rostock, Germany
- 2Faculty of Medicine Carl Gustav Carus, University Center for Orthopedics, Trauma and Plastic Surgery, Technische Universität Dresden, Dresden, Germany
- 3Department of Diagnostic and Interventional Neuroradiology, School of Medicine and Health, Technical University of Munich, Munich, Germany
- 4Department of Anesthesia and Intensive Care, University Hospital Rostock, Rostock, Germany
- 5Medical Biology and Electron Microscopy Centre, Rostock University Medical Center, Rostock, Germany
- 6Department Life, Light and Matter, University of Rostock, Rostock, Germany
- 7Department of Orthopedics and Orthopedic Surgery, University Medicine Greifswald, Greifswald, Germany
- 8Department of Ophthalmology, Rostock University Medical Center, Rostock, Germany
- 9Research Laboratory for Biomechanics and Implant Technology, Department of Orthopedics, Rostock University Medical Center, Rostock, Germany
- 10Core Facility Multimodal Small Animal Imaging, Rostock University Medical Center, Rostock, Germany
Introduction: The chicken egg, with its in ovo compartments, is a widely used and popular animal model in experimental studies. This study aimed to quantify the volumes of the yolk/yolk sac, amniotic fluid, and chicken embryo in ovo using non-invasive ultra-high-field magnetic resonance imaging (UHF-MRI).
Materials and methods: In total, 64 chicken eggs were examined using a 7 T UHF-MRI scanner, acquiring T2-weighted anatomical images of the entire egg from developmental day 1 to 16 (D1-D16). Four eggs were scanned each developmental day, and the volumes of the yolk/yolk sac, amniotic fluid, and embryo were quantitatively assessed.
Results: UHF-MRI facilitated the in ovo quantitative assessment of the yolk/yolk sac starting from D1 and the embryo from D5 onward. The yolk/yolk sac volume increased from D1 to D6 before progressively decreasing until D14. The amniotic cavity could be detected on D6, with its fluid volume increasing steadily until D14. The embryo’s volume increased consistently throughout the developmental period, reaching its peak at D16.
Discussion: UHF-MRI allows in vivo assessment of embryonic development, providing non-invasive, longitudinal insights into the volumes of the yolk/yolk sac, amniotic fluid, and chicken embryo. The investigation method described in this study may provide a standardized model for biomedical research in the developing chicken embryo, supporting various experimental applications.
Introduction
Ultra-high-field magnetic resonance imaging (UHF-MRI) with magnetic field strength (B0) of 7 Tesla (T) and above is an excellent technique for non-invasive and non-destructive imaging. This technique offers excellent soft tissue contrast and high in-plane resolution in the submillimeter range. The higher magnetic field strength enhances resolution and reduces scan time, enabling detailed examination of delicate anatomical structures, such as the embryonic brain or eye, which are often smaller than a few millimeters (1–3). As a non-invasive imaging technique, UHF-MRI allows in ovo and in vivo studies of chicken embryos throughout their entire developmental period, as demonstrated previously (4–9).
During the 21-day developmental period before hatching, the egg contents and surrounding eggshells provide all necessary resources except oxygen and heat (10, 11). Due to its short developmental period and relatively easy handling, the chicken egg, with its in ovo compartments, is a widely used and popular animal model for experimental studies (12–21).
During development, three extraembryonic membranes are formed, including the yolk/yolk sac membrane, the amnion, and the chorioallantoic membrane (CAM) (22, 23). All essential nutrients, including proteins, lipids, carbohydrates, and minerals, are provided by the yolk/yolk sac. The yolk/yolk sac is important for blood cell synthesis, especially erythrocytes. The amnion encloses the embryo and protects it from mechanical and thermal shocks. Before hatching, the embryo ingests the amniotic fluid as a source for water and nutrients. During development, the allantois fuses with the chorion to form the CAM. This heavily vascularized compartment is used as a respiratory organ along the eggshell and is involved in the acid–base balance (24).
Furthermore, this compartment is important for the excretion and storage of waste products from the embryo (25, 26). A key resource for the early growth of the embryo is the albumen, a source of water and proteins (10–12). Parolini et al. decided to inject into the albumen rather than into the yolk because of the amphipathic properties of perfluorooctanesulfonate and its high binding affinity to proteins, such as lipoproteins and albumin (12).
As mentioned above, all compartments play an important role in the embryonic development of the chicken. Therefore, this animal model has been frequently used to evaluate possible impacts on the developmental processes that occur after changes and manipulations of these compartments in ovo, e.g., after injections (12–17).
Over the decades, CAM has been employed to study angiogenesis, tumor growth, metastasizing potential, and cardiovascular development, as the chick embryo is naturally immunodeficient (13, 14). Other studies showed that the chicken embryo is a suitable model to explore the developmental toxicity of various substances and compounds at relevant concentrations for humans (15). Briels et al. injected perfluorooctanesulfonate and its chlorinated polyfluoroalkyl ether sulfonate alternative F53-B, separately and as mixtures, into the yolk sac at the beginning of the developmental period (15).
Petrovová et al. examined agrochemicals’ effects and potential embryotoxicity, including pesticides, by injecting a cholinesterase inhibitor, bendiocarb, in the chicken embryo (16). In 2010, de Siqueira Bueno et al. injected embryotoxic air pollutants into the air sac of the chicken embryo and observed induced abnormalities in embryo–fetal development (17).
All studies have in common that they calculated the doses of the injected substances in relation to the total weight of the egg without focusing on the volumes of the injected compartments. However, this approach does not consider developmental differences in the targeted compartment in relation to the embryo size and growth, thus making an accurate delivery of the tested substances questionable. In addition, some authors assume a uniform distribution of the applied compounds throughout all egg compartments after injecting persistent organic pollutants in the allantois (18), again neglecting the considerable changes in these compartments with respect to embryo nutrition and growth.
Quantifying the volumes of the yolk, yolk sac, and amniotic fluid in avian eggs is essential for establishing a standardized animal model in developmental biology. These measurements provide critical insights into nutrient utilization and key stages of embryonic growth, enabling precise monitoring of developmental patterns. Tracking these volumes also enhances our understanding of physiological processes related to embryonic hydration, nutrition, and overall health. Moreover, such data establishes baseline metrics for normal development, supporting comparative studies and allowing researchers to identify anomalies or assess the effects of experimental interventions (10–17, 22, 23).
Therefore, to improve the injection and achieve more accurate concentrations in future studies, it is important to know the volumes of the compartments during the developmental period. Therefore, this study aimed to quantify the volumes of yolk/yolk sac, amniotic fluid, and the chicken embryo over the entire developmental period in ovo and in vivo by using non-invasive UHF-MRI.
Materials and methods
Animal model
All animals were handled in accordance with the ARVO statement for the Use of Animals in Ophthalmic and Vision Research, and the experiments complied with national legislation for the protection of animals. Sixty-four fertilized chicken eggs (White Leghorn), obtained from a commercial hatchery (Valo BioMedia, Osterholz-Scharmbeck, Germany), were stored at room temperature (20°C) for 3 days prior to the start of incubation. All 64 eggs were simultaneously incubated (Heka-Turbo 168, HEKA, Rietberg, Germany) at optimal conditions: 37.8°C and 60% relative humidity, as recommended by the manufacturer of the incubator. Each day (D1-D16), four eggs were scanned once, respectively, and incubation was terminated. For euthanasia, the chicken eggs were cooled on crushed ice for 60 min in total. Afterward, the eggs were opened following decapitation. None of the embryos hatched. After euthanasia, the yolk sac was extracted, and the volume was measured manually in a water-filled measurement cylinder, as detailed below.
Ultra-high-field MR imaging
In vivo imaging was conducted on a 7 T MRI scanner (BioSpec 70/30, Bruker Biospin MRI GmbH, Ettlingen, Germany) equipped with a BGA-12S HP gradient (bore size of 11 cm) and a circularly polarized volume coil (rat body coil with 112 mm/72 mm outer/inner diameter, Bruker Biospin). First, a fast T2-weighted (T2w) localizer was acquired. Following the localizer, a high-resolution T2w turbo spin-echo sequence (TurboRARE – Rapid Acquisition with Relaxation Enhancement) of the entire egg was performed in coronal planes. Imaging parameters were time of repetition (TR)/time of echo (TE) 3,740/27 ms; matrix size 368 × 368; field of view (FOV) 40 × 40 mm; in-plane resolution 0.150 × 0.150 mm; slice thickness 1.0 mm; no slice gap; time of acquisition (TA), 10:38 min.
Motion artifacts became more pronounced from D8 onward, impairing image quality. Therefore, the eggs were removed from the incubator and placed on crushed ice for at least 20 min to minimize motion inside the egg, and afterward, an MRI was performed. Following that, the eggs were terminated. As demonstrated in different studies (2, 4–6), eggs can typically be returned to the incubator after 40–60 min on crushed ice without adversely affecting development, allowing incubation to continue. This well-established procedure is essential for preventing motion artifacts. Temperature was monitored during the entire scanning time using an MR-compatible thermometer (1,025 T, Monitoring & Gating system, Small Animal Instruments, United States) as described previously (4, 5).
MR image evaluation
For further workup, the MR datasets were transferred to an ITK-Snap workstation (Ver. 3.8.0) (27), and contours of the yolk/yolk sac, amniotic cavity with its fluid, and the chicken embryo were manually defined on all respective slides of raw data (see Figure 1).
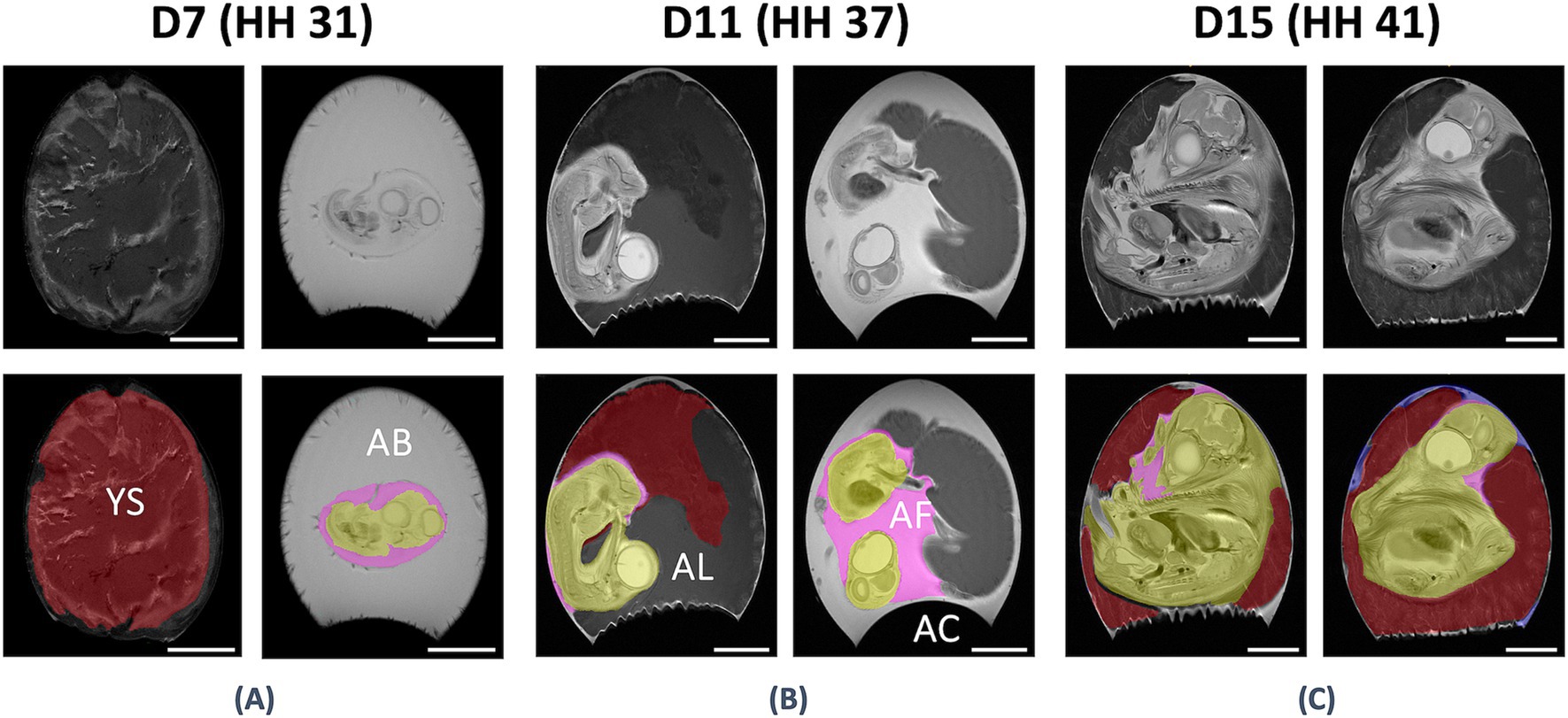
Figure 1. Anatomical evaluation of the in-ovo compartments using UHF-MRI. Anatomical assessment of the in ovo compartments at D7 / HH stage 31 (A), D11 / HH stage 37 (B), and D15 / HH stage 41 (C) on coronal T2-weighted UHF-MR images with (bottom) and without (top) segmentation. It was possible to differentiate between the yolk/yolk sac (YS, red), amniotic fluid (AF, pink), albumen (AB), allantois (AL), air chamber (AC), and the chicken embryo (CE) on all respective slides of raw MRI data. Bar ≙ 10 mm.
To evaluate the reproducibility of the analysis, this procedure was repeated three times, and mean values were calculated for each region of interest.
Evaluation of yolk sac ex vivo
After MRI, the embryos were euthanized, and the yolk sac was collected separately. A 100 mL measuring cylinder (EcoLab E-1266, neoLab Migge GmbH, Heidelberg, Germany) was filled with 50 mL of clear water (20° C), and the yolk sac was placed into the cylinder. The volume was determined by measuring the increase in water level within the cylinder. This established procedure for volume determination is referred to as the Archimedean method (28, 29).
Statistical analysis
Volume values of yolk/yolk sac, amniotic fluid, and the chicken embryo are provided as mean ± standard deviation using IBM® SPSS® Advanced Statistics 22.0. A regression line was determined with an R2 closest to 1 for the amniotic fluid and the chicken embryo. To ascertain whether there is a significant difference between the volumes of the yolk sac in ovo and ex ovo, a paired t-test was conducted based on the assumption of a p-value of <0.05.
Results
All eggs were successfully incubated. MRI identified changes in inner egg structures starting from D1 and identified changes in the embryo from D5 onward. Quantification of the yolk and, subsequently, the yolk sac was feasible from D1 onward, while the amniotic cavity could be evaluated from D6 onward. All results are shown in Table 1.
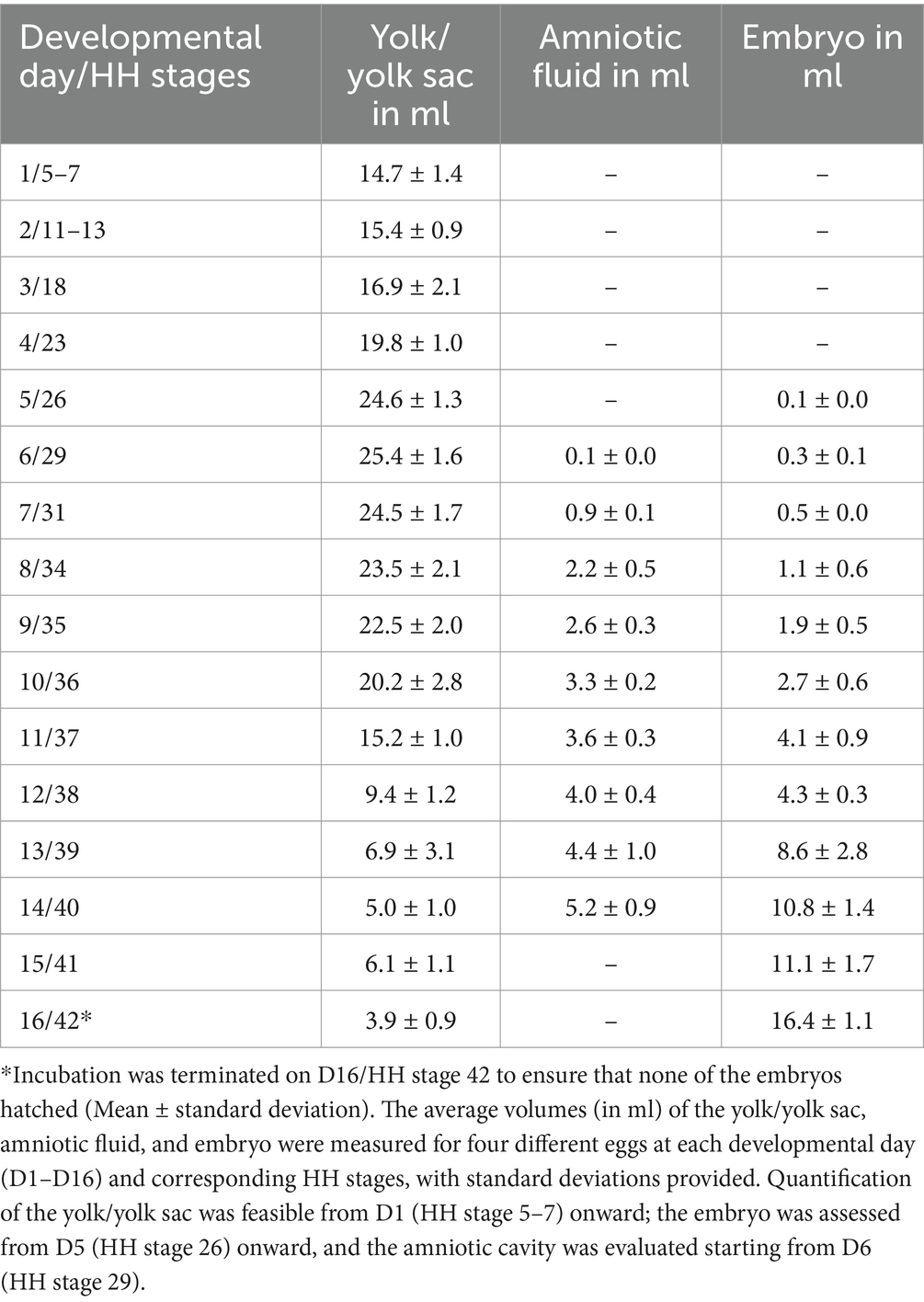
Table 1. Quantitative evaluation of the volume of the yolk/yolk sac, the amniotic fluid, and the chicken embryo in ml from D1 to D16.
Yolk/yolk sac
First, the volume slowly increased between D1 with (mean ± standard deviation: 14.7 mL ± 1.4 mL) to (25.4 ± 1.6) ml on D6. Afterward, the volume consistently decreased until D14 with (5.0 ± 1.0) ml. The most significant change could be observed between D10 and D12, from a volume of (20.2 ± 2.8) ml to (9.4 ± 1.2) ml. A minor increase of the volume was observed on D15 to (6.1 ± 1.1) ml before it decreased again to (3.9 ± 0.9) ml on D16 (see Table 1; Figure 2A).
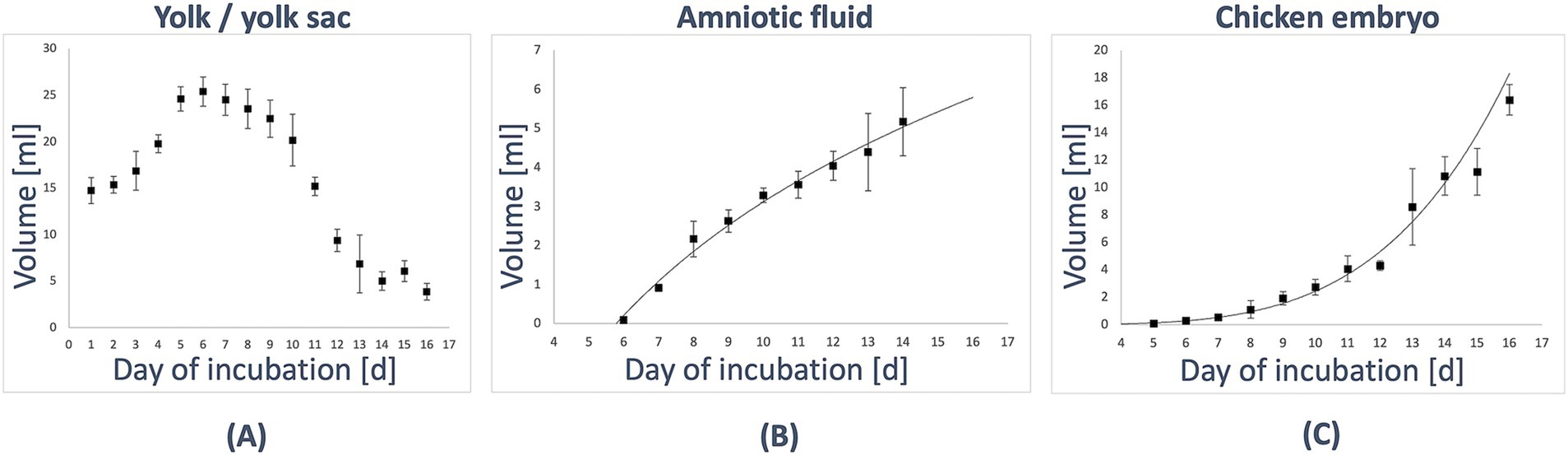
Figure 2. Volumes of the in-ovo compartments during development. (A) The yolk/yolk sac was identified on D1 (HH stage 5–7) in ovo. Initially, its volume gradually increased from (14.7 ± 1.4) ml on D1 (HH stage 5–7) to (25.4 ± 1.6) ml on D6 (HH stage 29). Subsequently, the volume consistently decreased, reaching (5.0 ± 1.0) ml by D14 (HH stage 40). (B) The amniotic cavity was detectable starting on D6 (HH stage 29), with an initial fluid volume of (0.1 ± 0.0) ml. From this point, the volume increased logarithmically (R2 = 0.987), reaching (5.2 ± 0.9) ml by D14 (HH stage 40). (C) The volume of the embryo increased exponentially (R2 = 0.989), starting from (0.1 ± 0.0) ml on D5 (HH stage 26) and growing to (16.4 ± 1.1) ml by D16 (HH stage 42).
After MRI, the eggs were opened, and it was possible to separate the yolk sac with its thin membrane without damage from D12 onward. The volume was measured on D12 with (10.3 ± 0.5) ml and decreased to (5.8 ± 1.7) ml on D14. Moreover, in the in ovo measurement, a minor increase of the volume was observed on D15 to (6.8 ± 0.5) ml before it decreased again to (5.3 ± 1.0) ml on D16 (see Table 2; Figure 3). No significant differences could be observed based on the assumption of a p-value of <0.05.
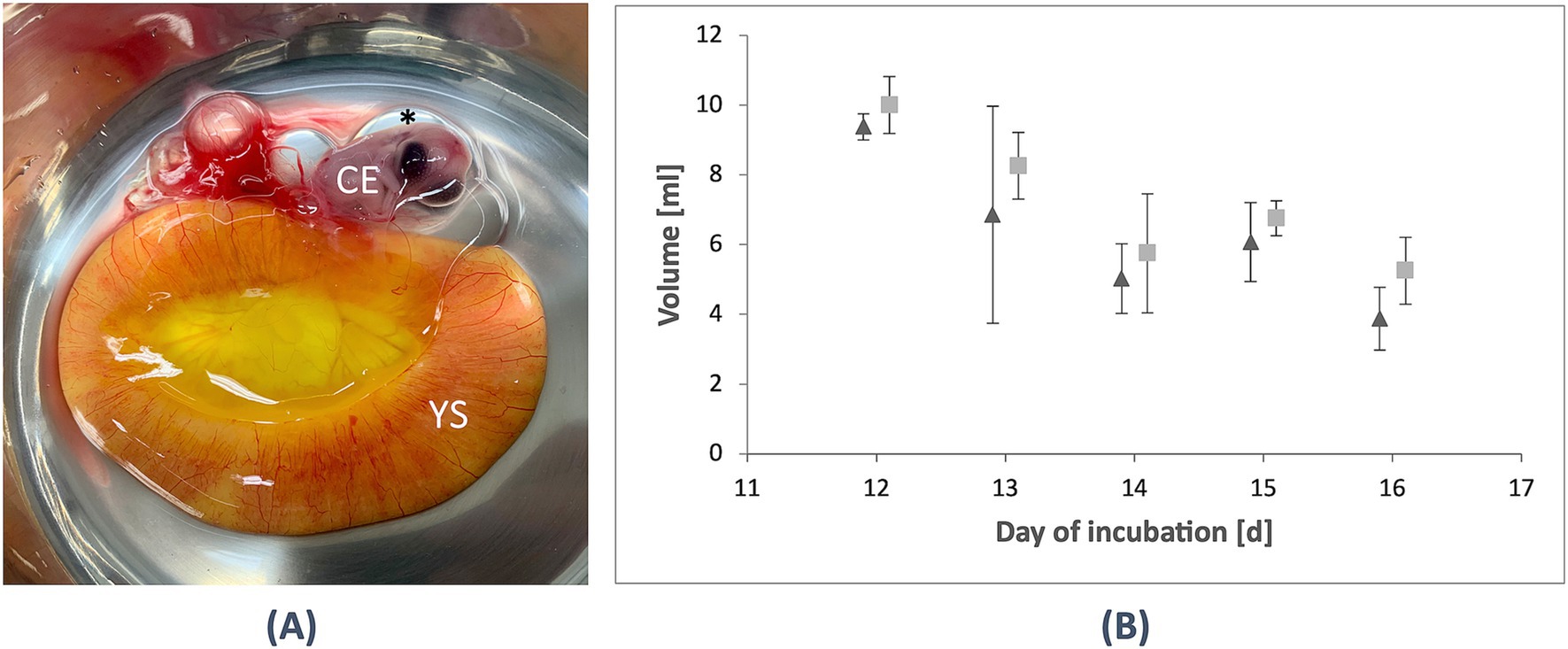
Figure 3. Evaluation of the yolk sac in ovo and ex ovo. (A) After MRI, incubation was terminated, and the eggs were opened. From D12 / HH stage 38 onward, it was possible to extract the yolk sac (YS) and observe the chicken embryo (CE) and the amniotic cavity (*). (B) A comparison was made between the yolk sac volume in ovo (triangle) and ex ovo (rectangle) from D12–16 (HH stage 38–42). While the in ovo measurements showed slightly smaller volumes overall, a minor increase in the ex ovo volumes was observed, peaking on D15 (HH stage 41) before decreasing again on D16 (HH stage 42). These findings contribute to the anatomical evaluation of in ovo compartments using UHF-MRI.
Amniotic cavity
The amniotic cavity could be observed with a fluid volume of (0.1 ± 0.0) ml on D6. Afterward, it increased in a logarithmic way (R2 = 0.987) to (5.2 ± 0.9) ml on D14 (see Table 1; Figure 2B).
Embryo
The volume of the embryo was first segmented on D5, with measurements indicating an exponential increase (R2 = 0.989) from (0.1 ± 0.0) ml on D5 to (16.4 ± 1.1) ml on D16. Significant growth changes were observed, particularly between D12 and D13, where the volume increased from (4.3 ± 0.3) ml to (8.6 ± 2.8) ml, and between D15 and D16, where it grew from (11.1 ± 1.7) ml to (16.4 ± 1.1) ml. Detailed data are presented in Table 1; Figure 2C.
Discussion
In contrast to the morphological and functional longitudinal development of the chicken embryo, investigation of the in-ovo development of the compartments is scarce. In 1951, Hamburger and Hamilton (HH) provided 46 chronological stages that allowed the developing chick to be accurately characterized during all embryonic stages but did not focus on the in-ovo compartments (19).
To date, there have been several approaches for studying the impact of different injections into the respective embryonic compartments, especially yolk/yolk sacs, amniotic fluid, and chicken embryos (12–18, 20). The amniotic injection route is the most popular because of its fast distribution, as substances may be quickly absorbed orally and through mucosal surfaces (30). Nevertheless, an application through the chorioallantoic membrane or the amniotic cavity may lead to reduced hatchability compared to applications where the yolk sac route or the extraembryonic cavity is used (31). Injection of the air sac is usually made in an early stage of development, while in a later stage, the amniotic route is preferred, as the embryo starts to consume amniotic fluids (32).
The majority of the above-mentioned studies share the approach of calculating the doses of injected substances relative to the total weight of the egg, rather than considering the specific volumes of the injected compartments.
UHF-MRI allowed the quantitative assessment of the yolk/yolk sac starting from D1 and the embryo from D5 onward. The volume of the yolk/yolk sac first increased between D1 and D6, which might have been caused by the water flux from the albumen into the yolk/yolk sac (23). The amniotic cavity could be observed on D6, and its fluid volume increased to D14. In this study, one of the reasons for the growth is the transfer of albumen into the amniotic cavity (21).
There was an increasing volume of the embryo during the observed developmental period. The volumes we obtained in our study are similar in range compared to the few values already known from the literature. In contrast with our study, Adriaensen et al. quantified the volume of the yolk sac, amniotic fluid, and chicken embryo but solely on D11, 13, and 15 using MRI (21). On D11, the volume of amniotic fluid was summarized as about (3.6 ± 0.30) ml in our study and about (3.42 ± 0.25) ml as described by Adriaensen et al., which is quite similar. In this study, the volume of the embryo and the yolk sac is about (16.84 ± 1.13) ml in total at D13. We were able to quantify the volume of the embryo and yolk sac at D13 with about (16.50 ± 2.90) ml, which is in accordance with the results of the study mentioned above. We focused on the same compartments, and it was possible to image and quantify the respective volumes and obtain these measurements longitudinally over the entire developmental period until D16.
In another study by Starck et al., the volume of the yolk/yolk sac of ostrich eggs was evaluated at selected developmental time points, and it was possible to observe an increase of the volume in the first 3 weeks with a following decrease until the end of development after 7 weeks (33). Compared to this study, our measured values of the yolk/yolk sac volume are much smaller because the ostrich egg is much larger than the chicken egg, but the trend of the results is similar. This might be also because the yolk is digested by the embryo. Moreover, our observed increase in the embryo volume over the development period is in accordance with general growth and changes in the embryonic weight as described in the literature (19, 34). Referring to this and to our best knowledge, we found no further information in the literature yet.
To validate consistency between in ovo and ex ovo measurements, embryos were euthanized after MRI, and the yolk sac was collected separately. We demonstrate an excellent correlation between the MRI-based volumetry and the physical measurements after opening the egg. It has also been observed in ovo that a minor increase in the volume of the yolk sac was observed by the physical volumetry before it decreased again but with no significant differences (see Table 2 and Figure 3).
We decided to stop the incubation on D16 to ensure that none of the embryos hatched. Otherwise, we managed to overview nearly the entire developmental period except for 4 days. In addition, most of the above-mentioned studies dealing with in-ovo injections focused on the first two-thirds of the developmental period. It remains to be seen whether the measurements of the last 4 days of development are relevant for experimental studies, as they are generally not included in current studies.
Manders et al. blindly targeted five embryonic compartments (amniotic cavity, chicken embryo, allantois, albumen, and yolk/yolk sac) with needles of four different lengths by inoculating methylene blue. Three compartments (air chamber, CAM, and blood vessels) were prepared within sight. The visual inspection after breaking the eggs yielded low success rates (20). The reason might be, on the one hand, the constant change in volume of the different compartments and, on the other hand, the variable positions within the egg. It might even be necessary to perform an MRI before every injection to evaluate the exact position. Therefore, our study would greatly benefit future studies and help obtain more accurate injections and, subsequently, more reliable experimental results.
Our study has some limitations. First, one influence might be the cooling of the eggs to prevent motion artifacts after D8, which could have a minor impact on the volumes of the compartments and structures inside the egg, even if it does not influence embryonic development (35, 36). Secondly, although the in-plane resolution of UHF-MRI is excellent, it was sometimes challenging, due to the slice thickness, to differentiate the small structures inside the egg, e.g., the feathers of the embryo. This limitation could be overcome by acquiring isotopic MRI datasets, but there would be a significant increase in acquisition time.
Due to artifact formation, volumetric analysis of air-filled compartments using MRI is challenging. Therefore, we did not focus on the air chamber, which gradually enlarges during development. Isotropic 3D T2-weighted imaging could be attempted to quantify the air sac in future studies.
Finally, the number of embryos used in this study is small, but we demonstrated, as a proof of principle, that the longitudinal volumetric analysis of the amniotic fluid, yolk/yolk sac, and embryo is feasible using MRI. Due to the small number of eggs, we did not correlate our results with the weight and size of the eggs. This could be part of further studies, as there are different types of chicken eggs regarding size and weight (37).
Due to the results of our study, it is possible to conduct targeted injections more precisely and improve the quality of future experiments. For example, it is possible to add Gadobutrol (Gadovist® 1.0 mmoL/mL; Bayer Vital GmbH, Berlin, Germany; 0.0004 mM) as a contrast agent in order to verify the correct injection in the respective compartment using T1-Fast Low-Angle Shot (T1 FLASH) weighted MRI (see Figures 4A,B). It is also possible to evaluate the success of some injections (e.g., chromium ions) without an extra contrast agent and only by the intensity change inside the respective compartment (see Figures 4C,D). MRI could also quantify the compartments’ volumes before and after injections to verify the application site and applicated fluid volume.
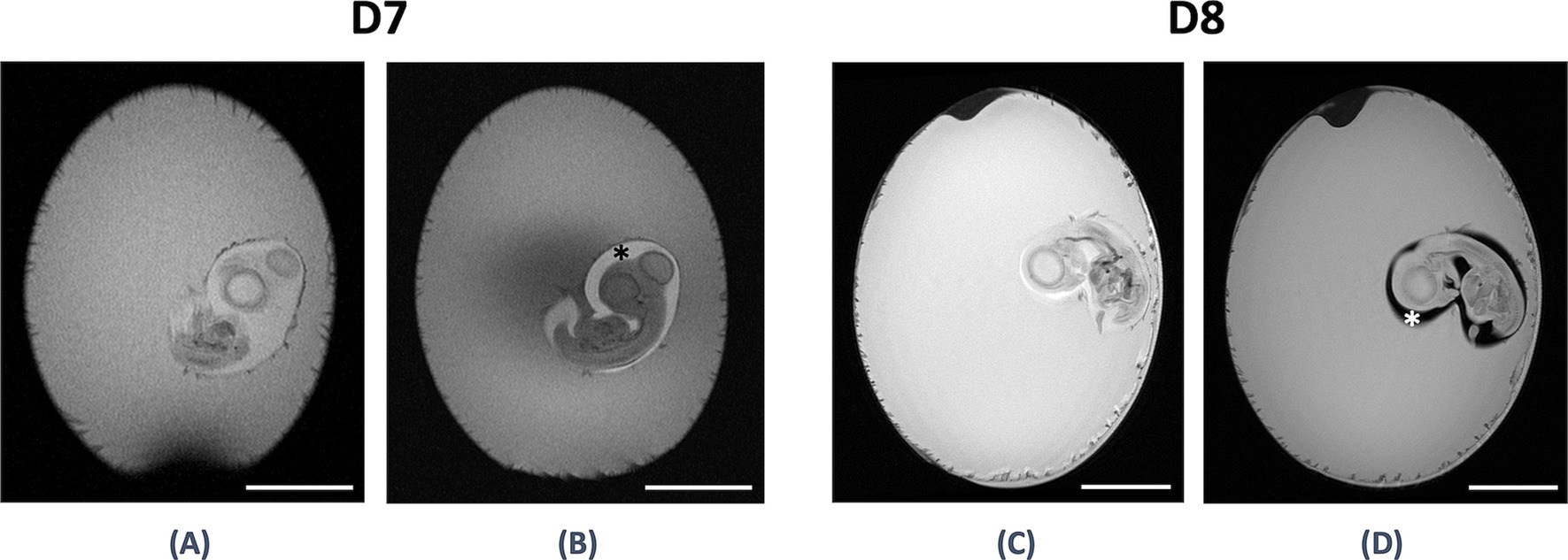
Figure 4. Amniotic cavity before and after injection of Gadovist® and chromium ion solution. T1-FLASH-weighted images acquired before (A) and 5 min after (B) the injection of 20 μL of Gadovist® (0.0004 mM)-NaCl solution into the amniotic cavity (indicated by a black asterisk) demonstrated a slight increase in the intensity of the amniotic fluid compared to the albumen. Fast spin echo T2-weighted (TurboRARE) MRI images acquired before (C) and after (D) the injection of 5 μL of a chromium (III) ion solution (11 mM) into the amniotic cavity (indicated by a white asterisk) showed a clear decrease in the intensity of the amniotic fluid surrounding the embryo. The hyperintense fluid seen in (C) transitioned to hypointense fluid in (D). Scale bar ≙ 10 mm.
In summary, UHF-MRI allows longitudinal volumetric analysis of different compartments of the chicken egg non-invasively and non-destructively, in vivo and in ovo. This will enable future studies to estimate or even determine the exact individual volume of the respective compartment before an invasive procedure like an injection and to optimize experimental procedures.
Data availability statement
The raw data supporting the conclusions of this article will be made available by the authors, without undue reservation.
Ethics statement
The animal study was approved by “Landesamt für Landwirtschaft, Lebensmittelsicherheit und Fischerei Mecklenburg-Vorpommern (LALLF MV, Germany),” and all in ovo experiments were in compliance with the European directive for protection of animals used for experimental and scientific purposes issued by the Directorate General for Environment (Directive 2010/63/EU of the European Parliament and of the Council of 22 September 2010 on the Protection of Animals used for Scientific Purposes. Official Journal L 276: 33–79 (revising Directive 86/609/EEC)) and the respective German interpretation “Tierschutz-Versuchstierverordnung “(1 August 2013, BGBl. I S. 3125, 3,126), which was updated by article 394 on 31 August 2015 (BGBl. I S. 1474). Accordingly, in ovo experiments do not require any special additional allowance as long as the embryos are sacrificed before hatching, as done in this study. The study was conducted in accordance with the local legislation and institutional requirements.
Author contributions
FS: Conceptualization, Data curation, Writing – original draft, Writing – review & editing. HS: Formal analysis, Writing – original draft, Writing – review & editing. JK: Data curation, Writing – original draft, Writing – review & editing. MF: Conceptualization, Project administration, Supervision, Writing – original draft, Writing – review & editing. IL: Methodology, Validation, Writing – original draft, Writing – review & editing. OS: Conceptualization, Supervision, Visualization, Writing – original draft, Writing – review & editing. AJ-H: Conceptualization, Formal analysis, Writing – original draft, Writing – review & editing. SL: Supervision, Validation, Writing – original draft, Writing – review & editing. TL: Formal analysis, Validation, Writing – original draft, Writing – review & editing. JS: Conceptualization, Data curation, Formal analysis, Writing – original draft, Writing – review & editing.
Funding
The author(s) declare financial support was received for the research, authorship, and/or publication of this article. We acknowledge financial support by Deutsche Forschungsgemeinschaft and Universität Rostock/Universitätsmedizin Rostock within the Open Access Publishing funding program.
Conflict of interest
The authors declare that the research was conducted in the absence of any commercial or financial relationships that could be construed as a potential conflict of interest.
The author(s) declared that they were an editorial board member of Frontiers, at the time of submission. This had no impact on the peer review process and the final decision.
Publisher’s note
All claims expressed in this article are solely those of the authors and do not necessarily represent those of their affiliated organizations, or those of the publisher, the editors and the reviewers. Any product that may be evaluated in this article, or claim that may be made by its manufacturer, is not guaranteed or endorsed by the publisher.
References
1. Richdale, K, Wassenaar, P, Teal Bluestein, K, Abduljalil, A, Christoforidis, JA, Lanz, T, et al. 7 tesla MR imaging of the human eye in vivo. J Magn Reson Imaging. (2009) 30:924–32. doi: 10.1002/jmri.21959
2. Bain, MM, Fagan, AJ, Mullin, JM, McNaught, I, McLean, J, and Condon, B. Noninvasive monitoring of chick development in ovo using a 7T MRI system from day 12 of incubation through to hatching. J Magn Reson Imaging. (2007) 26:198–201. doi: 10.1002/jmri.20963
3. Lindner, T, Langner, S, Graessl, A, Rieger, J, Schwerter, M, Muhle, M, et al. High spatial resolution in vivo magnetic resonance imaging of the human eye, orbit, nervus opticus and optic nerve sheath at 7.0 tesla. Exp Eye Res. (2014) 125:89–94. doi: 10.1016/j.exer.2014.05.017
4. Streckenbach, F, Klose, R, Langner, S, Langner, I, Frank, M, Wree, A, et al. Ultrahigh-field quantitative MR microscopy of the chicken eye in vivo throughout the in Ovo period. Mol Imaging Biol. (2019) 21:78–85. doi: 10.1007/s11307-018-1208-9
5. Klose, R, Streckenbach, F, Hadlich, S, Stahnke, T, Guthoff, R, Wree, A, et al. Ultra-high-field MRI in the chicken embryo in Ovo - a model for experimental ophthalmology. Klin Monatsbl Augenheilkd. (2017) 234:1458–62. doi: 10.1055/s-0043-120675
6. Lindner, T, Klose, R, Streckenbach, F, Stahnke, T, Hadlich, S, Kühn, JP, et al. Morphologic and biometric evaluation of chick embryo eyes in ovo using 7 tesla MRI. Sci Rep. (2017) 7:2647. doi: 10.1038/s41598-017-02755-4
7. Woelders, H, de Wit, A, Lourens, A, Stockhofe, N, Engel, B, Hulsegge, I, et al. Bioelectromagnetics (2017) 38:186–203. doi: 10.1002/bem.22026,
8. Pawlak, K, Nieckarz, Z, Sechman, A, Wojtysiak, D, Bojarski, B, and Tombarkiewicz, B. Effect of a 1800 MHz electromagnetic field emitted during embryogenesis on chick development and hatchability. Anat Histol Embryol. (2018) 47:222–30. doi: 10.1111/ahe.12346
9. Augustianath, T, Evans, DA, and Anisha, GS. Teratogenic effects of radiofrequency electromagnetic radiation on the embryonic development of chick: A study on morphology and hatchability. Res Vet Sci. (2023) 159:93–100. doi: 10.1016/j.rvsc.2023.04.015
12. Parolini, M, Colombo, G, Valsecchi, S, Mazzoni, M, Possenti, CD, Caprioli, M, et al. Potential toxicity of environmentally relevant perfluorooctane sulfonate (PFOS) concentrations to yellow-legged gull Larus michahellis embryos. Environ Sci Pollut Res. (2016) 23:426–37. doi: 10.1007/s11356-015-5248-2
13. Ribatti, D. The chick embryo chorioallantoic membrane as a model for tumor biology. Exp Cell Res. (2014) 328:314–24. doi: 10.1016/j.yexcr.2014.06.010
14. Weiss, A, van Beijnum, JR, Bonvin, D, Jichlinski, P, Dyson, PJ, Griffioen, AW, et al. Low-dose angiostatic tyrosine kinase inhibitors improve photodynamic therapy for cancer: lack of vascular normalization. J Cell Mol Med. (2014) 18:480–91. doi: 10.1111/jcmm.12199
15. Briels, N, Ciesielski, TM, Herzke, D, and Jaspers, VLB. Developmental toxicity of Perfluorooctanesulfonate (PFOS) and its chlorinated Polyfluoroalkyl ether sulfonate alternative F-53B in the domestic chicken. Environ Sci Technol. (2018) 52:12859–67. doi: 10.1021/acs.est.8b04749
16. Petrovová, E, Maženský, D, Vdoviaková, K, Massanyi, P, Luptáková, L, and Smrčo, P. Effect of bendiocarb on development of the chick embryo. J Appl Toxicol. (2010) 30:397–401. doi: 10.1002/jat.1509
17. De Siqueira Bueno, HM, Souza Leão Martins, R, Pannuti, C, dos Santos, RN, Sowmy, T, Barbosa Junior, F, et al. Metal embryotoxicity from urban particles in Sao Paulo city: an experimental study in chicken embryos. Ecotoxicol Environ Saf. (2010) 73:1385–90. doi: 10.1016/j.ecoenv.2010.02.016
18. Yadav, A, Verhaegen, S, Hadera, MG, Berntsen, HF, Berg, V, Lyche, JL, et al. Peripherally administered persistent organic pollutants distribute to the brain of developing chicken embryo in concentrations relevant to human exposure. Neurotoxicology. (2022) 88:79–87. doi: 10.1016/j.neuro.2021.10.013
19. Hamburger, V, and Hamilton, HL. A series of normal stages in the development of the chick embryo. J Morphol. (1951) 88:49–92. doi: 10.1002/jmor.1050880104
20. Manders, TTM, Matthijs, MGR, Veraa, S, van Eck, JHH, and Landman, WJM. Success rates of inoculation of the various compartments of embryonated chicken eggs at different incubation days. Avian Pathol. (2021) 50:61–77. doi: 10.1080/03079457.2020.1834503
21. Adriaensen, H, Parasote, V, Castilla, I, Bernardet, N, Halgrain, M, Lecompte, F, et al. How egg storage duration prior to incubation impairs egg quality and chicken embryonic development: contribution of imaging technologies. Front Physiol. (2022) 13:1–12. doi: 10.3389/fphys.2022.902154
24. Givisiez, PEN, Moreira Filho, ALB, Santos, MRB, Oliveira, HB, Ferket, PR, Oliveira, CJB, et al. Chicken embryo development: metabolic and morphological basis for in ovo feeding technology. Poult Sci. (2020) 99:6774–82. doi: 10.1016/j.psj.2020.09.074
25. Visschedijk, AH. The air space and embryonic respiration. I. The pattern of gaseous exchange in the fertile egg during the closing stages of incubation. Br Poult Sci. (1968) 9:173–84. doi: 10.1080/00071666808415707
26. Reijrink, IAM, van Duijvendijk, LAG, Meijerhof, R, Kemp, B, and van den Brand, H. Influence of air composition during egg storage on egg characteristics, embryonic development, hatchability, and chick quality. Poult Sci. (2010) 89:1992–2000. doi: 10.3382/ps.2009-00610
27. Yushkevich, PA, Piven, J, Hazlett, HC, Smith, RG, Ho, S, Gee, JC, et al. User-guided 3D active contour segmentation of anatomical structures: significantly improved efficiency and reliability. NeuroImage. (2006) 31:1116–28. doi: 10.1016/j.neuroimage.2006.01.015
28. Boersma, PD, and Rebstock, GA. Calculating egg volume when shape differs: when are equations appropiate? J Field Ornithol. (2010) 81:442–8. doi: 10.1111/j.1557-9263.2010.00300.x
29. Shi, P, Chen, L, Quinn, BK, Yu, K, Miao, Q, Guo, X, et al. A simple way to calculate the volume and surface area of avian eggs. Ann N Y Acad Sci. (2023) 1524:118–31. doi: 10.1111/nyas.15000
30. Oliveira, GS, McManus, C, and dos Santos, VM. Control of Escherichia coli in poultry using the in Ovo injection technique. Antibiotics. (2024) 13:205. doi: 10.3390/antibiotics13030205
31. Das, R, Mishra, P, and Jha, R. In ovo feeding as a tool for improving performance and gut health of poultry: a review. Front Vet Sci. (2021) 8:754246. doi: 10.3389/fvets.2021.754246
32. Berrocoso, JD, Kida, R, Singh, AK, Kim, YS, and Jha, R. Effect of in ovo injection of raffinose on growth performance and gut health parameters of broiler chicken. Poult Sci. (2017) 96:1573–80. doi: 10.3382/ps/pew430
33. Starck, JM. Morphology of the avian yolk sac. J Morphol. (2021) 282:959–72. doi: 10.1002/jmor.21262
34. Burke, WH, and Sharp, PJ. Sex differences in body weight of chicken embryos. Poult Sci. (1989) 68:805–10. doi: 10.3382/ps.0680805
35. Heidrich, A, Würbach, L, Opfermann, T, and Saluz, HP. Motion-artifact-free in vivo imaging utilizing narcotized avian embryos in Ovo. Mol Imaging Biol. (2011) 13:208–14. doi: 10.1007/s11307-010-0355-4
36. Chen, L, Wang, Z, Fu, X, Wang, S, Feng, Y, Coudyzer, W, et al. Dynamic 3D morphology of chick embryos and allantois depicted nondestructively by 3.0T clinical magnetic resonance imaging. Poult Sci. (2023) 102:102902. doi: 10.1016/j.psj.2023.102902
Keywords: in ovo cavities, in ovo development, ultra-high field MRI, animal model, chicken embryo
Citation: Streckenbach F, Schön H, König J, Frank M, Langner I, Stachs O, Jonitz-Heincke A, Langner S, Lindner T and Schätzel J (2024) Longitudinal volumetric analysis of in ovo compartments in chicken eggs using ultra-high-field magnetic resonance imaging. Front. Vet. Sci. 11:1450572. doi: 10.3389/fvets.2024.1450572
Edited by:
Hussein M. El-Husseiny, Tokyo University of Agriculture and Technology, JapanReviewed by:
Qixiao Jiang, Qingdao University, ChinaSittipon Intarapat, Mahidol University, Thailand
Copyright © 2024 Streckenbach, Schön, König, Frank, Langner, Stachs, Jonitz-Heincke, Langner, Lindner and Schätzel. This is an open-access article distributed under the terms of the Creative Commons Attribution License (CC BY). The use, distribution or reproduction in other forums is permitted, provided the original author(s) and the copyright owner(s) are credited and that the original publication in this journal is cited, in accordance with accepted academic practice. No use, distribution or reproduction is permitted which does not comply with these terms.
*Correspondence: Felix Streckenbach, RmVsaXguc3RyZWNrZW5iYWNoQG1lZC51bmktcm9zdG9jay5kZQ==