- 1Department of Infectious Diseases and Public Health, Jockey Club College of Veterinary Medicine and Life Sciences, City University of Hong Kong, Hong Kong, Hong Kong SAR, China
- 2Department of Veterinary Clinical Sciences, Centre for Animal Health and Welfare, Jockey Club College of Veterinary Medicine and Life Sciences, City University of Hong Kong, Hong Kong, Hong Kong SAR, China
Identifying risk factors associated with the carriage of antimicrobial-resistant (AMR) bacteria in dogs is crucial to understanding their epidemiology and for developing and refining targeted control measures. However, relevant data is scattered and conflicting findings have been reported. This systematic review aimed to compile risk factors associated with the carriage of AMR-Enterobacterales in dogs worldwide and to identify relevant knowledge gaps for directing future research. A systematic review was conducted according to PRISMA guidelines, searching PubMed, CABi, and Scopus databases for studies reporting risk factors for acquiring AMR-Enterobacterales in dogs. After screening peer-reviewed, English-language studies by title/abstract, eligible studies were subjected to a full-text assessment, data extraction, risk-of-bias assessment, and qualitative synthesis. In the initial search, 774 articles were identified, including 274 duplicates. After screening by title/abstract, 77 articles were subjected to full-text review, from which 40 were eventually selected for data extraction, including 29 cross-sectional, six cohort, and five case-control studies. The most frequently investigated risk factors for AMR-Enterobacterales carriage in dogs were antimicrobial use (28 of 40), age (24), sex (22), hospitalization (19), and feeding raw diet (14). Of these, antimicrobial use was the most common risk factor significantly associated with AMR-Enterobacterales (19/28), followed by raw diet (9/14) and hospitalization (8/19). Our synthesis emphasized the importance of increasing awareness regarding the prudent use of critically important antimicrobials (CIAs), such as fluoroquinolones, in companion animal practices, strengthening infection prevention and control procedures in veterinary clinics and hospitals and educating caregivers about the potential risks of feeding raw diets in order to reduce the burden of AMR-bacteria in dogs.
1 Introduction
Antimicrobial resistance (AMR) has been listed among the top 10 global public health threats by the World Health Organization (WHO) (1). According to a review published in 2019, AMR directly caused more than 1.2 million deaths and was indirectly associated with nearly five million deaths in humans (2). In the absence of effective control measures, deaths due to AMR could reach 10 million people annually by 2050 (3).
Antimicrobial resistance is a critical One Health issue due to the extensive use of antimicrobials and the potential transmission of resistant bacteria and their genes between humans, animals, and their ecological niche (4, 5). Companion animals, particularly dogs and cats, have been identified as potential reservoirs and carriers of AMR-bacteria mainly due to the frequent administration of antimicrobial drugs in small animal practices and their close contact with humans (6). AMR-bacteria with identical or similar genotypes have been isolated from companion animals and their caregivers, indicating their likely transmission between humans and companion animals (7–9). This source of AMR-bacteria is of growing concern because the pet population is increasing globally, strong bonds are formed between animals and their caregivers, and they are often treated as family members with frequent close physical contact (10, 11).
The mechanisms of AMR selection and spread vary among different bacterial species and can be complex and multifactorial (12). The order Enterobacterales, includes Escherichia coli and Klebsiella pneumoniae, which are classified in the critical priority group by WHO due to their significant AMR threat to public health and high prevalence (1). These organisms pose a considerable challenge in the global fight against AMR, warranting focused monitoring and research to mitigate their negative impact on animals and public health.
The primary risk factors identified for the carriage or infection with AMR-Enterobacterales in dogs include antimicrobial use, feeding raw meat diets, and hospitalization (13–15). Some studies have also identified age, sex, and breed as potential risk factors; however, there are conflicting findings among some studies and the factors investigated vary. Identifying important risk factors contributing to AMR-Enterobacterales carriage is necessary to better understand their epidemiology and to develop tailored prevention and control strategies. The objectives of this systematic review were to compile published evidence on risk factors for the carriage of AMR-Enterobacterales in dogs reported worldwide and to identify relevant knowledge gaps for directing future research.
2 Methods
This systematic review was conducted according to the Preferred Reporting Items for Systematic Reviews and Meta-Analyses (PRISMA) guidelines (16).
2.1 Eligibility criteria
Studies that reported any risk factors for carrying or detecting AMR-Enterobacterales in dogs worldwide were included in our systematic review. The search was limited to peer-reviewed studies published in English. There were no date or geographical limitations for inclusion. Studies involving dogs only or dogs together with other animal species (mixed species) were included; however, the relevant data were extracted for dogs only. Studies on non-Enterobacterales bacteria, published in languages other than English, purely experimental, reviews, and gray literature were excluded.
2.2 Search strategy
The last search for eligible articles was performed in December 2023 using three electronic databases (PubMed, CABi, and Scopus) and three main domains with relevant search terms ((dog OR dogs OR canine*) AND (antimicrobial resistan* OR AMR OR antibiotic resistan*) AND (Risk factor* OR protective factor*)). The initial search string was kept wide to capture all possibly relevant articles, and an expert librarian at City University of Hong Kong was consulted to confirm and adjust the string to be compatible with each database.
2.3 Screening and study selection
In the initial search, identified studies from the three databases were exported to EndNote V 20.6 (Clavariate Analytics, 2020) and duplicates were removed. The remaining studies were uploaded to Rayyan QCRI software (http://rayyan.qcri.org) (17), and each study was screened by title/abstract, followed by a full-text review of each identified eligible study to ensure relevance. Two reviewers independently conducted the screening and selection process. Any disagreements were resolved by a third independent reviewer. All steps of the study screening and selection process are summarized in Figure 1.
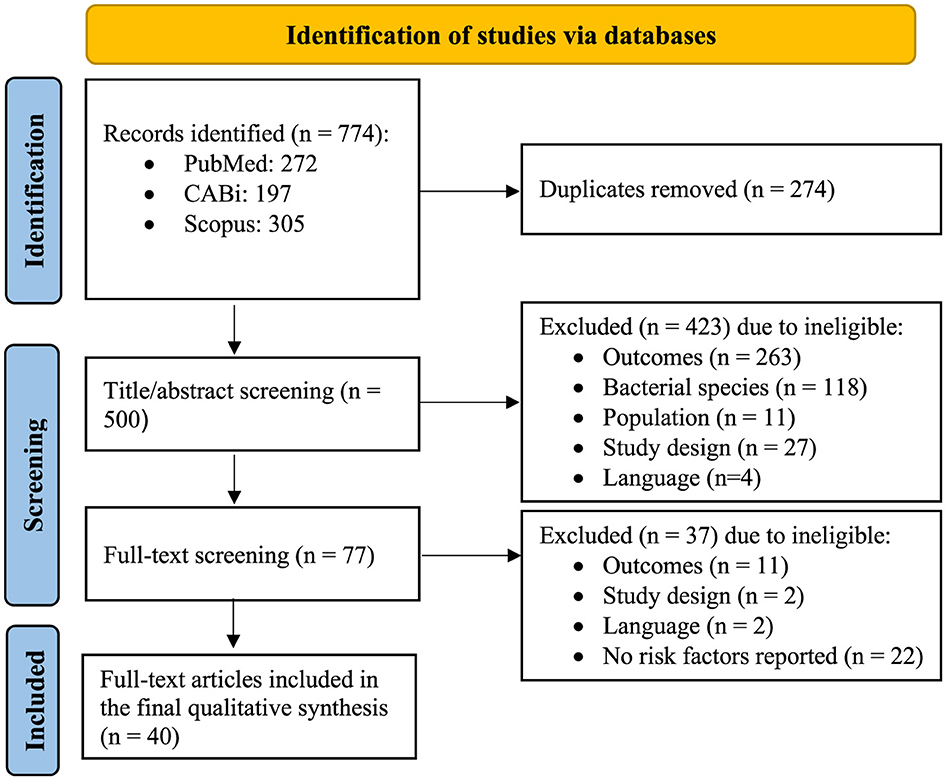
Figure 1. PRISMA flowchart indicating the study selection process (adapted from http://prisma-statement.org/PRISMAStatement/FlowDiagram.aspx).
2.4 Data extraction
The following variables and information were extracted from the body and Supplementary material of eligible studies and entered into a data extraction table in Microsoft Excel™ designed for this study: (1) General study characteristics (title, authors, journal name, year of publication, country study was performed, objectives), (2) Methodology (study type, study duration, sampling methods), (3) Animal population characteristics (species, number, sex, age, breed, neutering status), (4) Bacterial species isolated, (5) AMR patterns (e.g., ESBL), (6) Antimicrobial susceptibility test (AST) conducted, (7) AMR genes detected, (8) Statistical analysis conducted and (9) Factors investigated (risk and protective factors investigated).
2.5 Classification of risk factors
All investigated predictor variables for AMR-Enterobacterales, including risk/protective factors (hereafter, referred to as “risk factors”), extracted from the final selected studies were classified into nine main categories based on their similarities in order to provide clear summary assessments (Table 1). Most studies investigated a large number of predictor variables and were included in multiple risk factor categories. The main categories, subcategories, and statistical significance of associations between reported risk factors and the occurrence of AMR-Enterobacterales are summarized in Table 1, and with more details (e.g., all studied predictor variables per subcategory) in Supplementary Table 1.
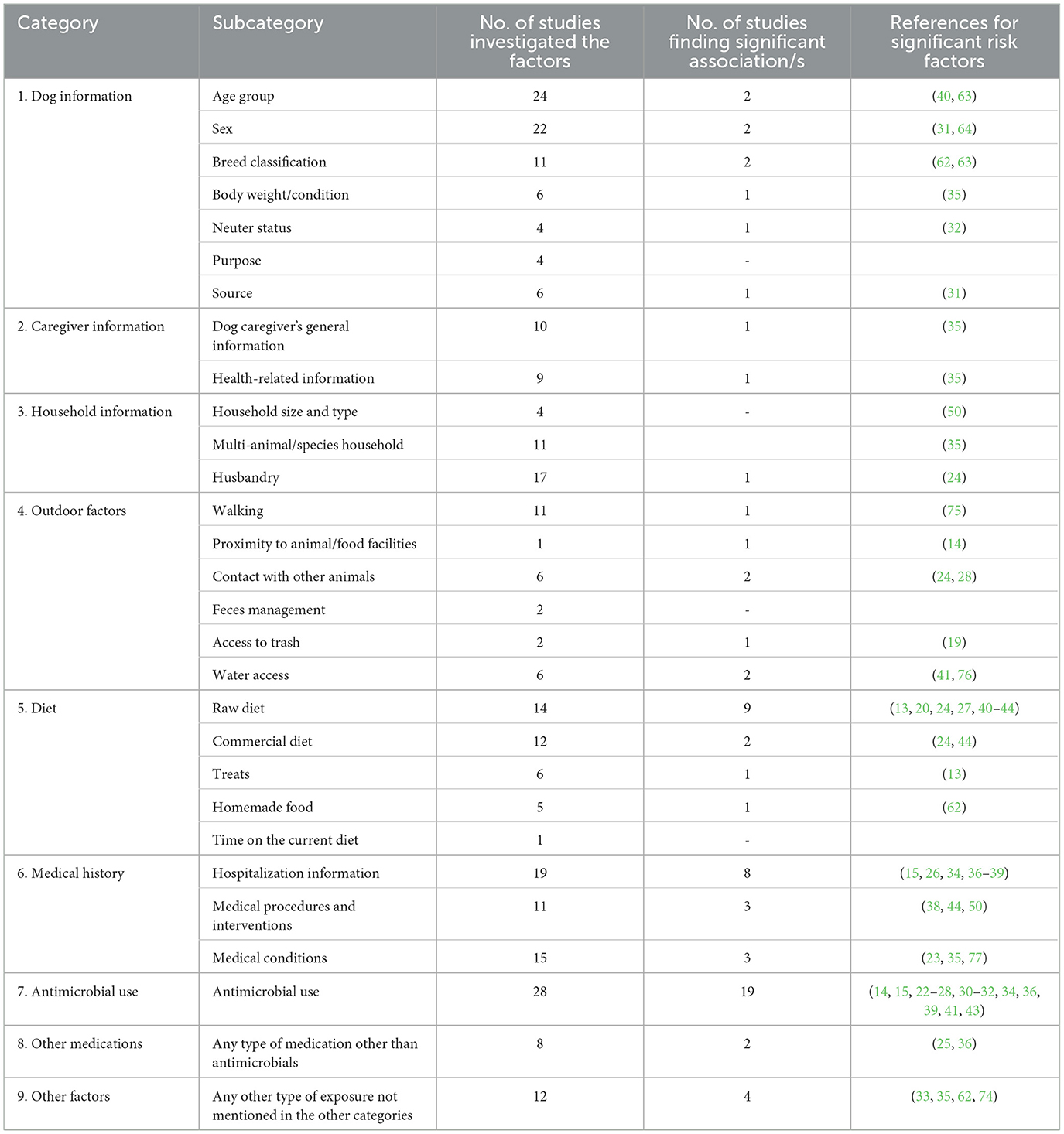
Table 1. Defined classifications for reported variables in the 40 selected studies with the number of studies investigating the factors and those finding statistically significant associations with the carriage of AMR-Enterobacterales in dogs.
2.6 Quality assessment of included studies
The quality of the included studies was evaluated using the guide notes in the Cochrane Handbook for Systematic Reviews of Interventions (18), which were adapted for our study types. This is a domain-based evaluation including 10 main quality assessment domains (aim, study population, study power, masking, case definition, diagnostic classification, indication of precision, completeness of results, believable conclusions, and consistency). Critical appraisals were made separately for each domain. Risk-of-bias for each domain was defined as high, low, or unclear, based on a detailed review of each selected study.
3 Results
3.1 Study selection
In total, 774 articles were identified in our initial search of the three databases, including 272 in PubMed, 197 in CABi, and 305 in Scopus. The workflow and corresponding numbers of the articles at each stage are summarized in Figure 1. After removing duplicates, 500 unique articles remained for title/abstract screening. Subsequently, 77 articles were selected for full-text review, of which, 37 were excluded due to: (1) not reporting any risk factors (22/37), (2) irrelevant outcomes (11/37), (3) study design (2/37), and (4) language (2/37) (Figure 1). Finally, 40 studies were eligible for complete data extraction.
3.2 Characteristics of included studies
All 40 studies included in the final qualitative synthesis were observational, including 29 cross-sectional, six cohort, and five case-control studies. All studies were published between 2006 and 2023 and were most frequently conducted in Europe (16/40), followed by North America (8/40), Asia (8/40), South America (4/40), Australia (3/40), and Africa (1/40).
Most studies (26/40) followed a non-random sampling strategy, and 10 reported random sampling for participant selection. Four studies did not provide explicit details about sampling methods (19–21). Age of the studied dogs ranged between 1 month and 17 years.
E.coli was the most frequently investigated and isolated bacteria in these studies (30/40), followed by Klebsiella (5/40), Salmonella (3/40), Citrobacter (3/40). Nine studies (of 40) reported the concurrent isolation of multiple bacterial species as follows: 3 reported concurrent E. coli and Salmonella; 3 others isolated E. coli and Klebsiella; one study detected E. coli and Citrobacter; one study E. coli, Klebsiella, Citrobacter and Morganella; and the remaining one reported E. coli, Klebsiella, Citrobacter, Proteus, and Enterobacter. No studies reported the detection of Shigella, Yersinia, or Serratia.
The most commonly reported AMR type was extended-spectrum beta-lactamase (ESBL)-producing E. coli (19/40). Eight studies reported multidrug resistant (MDR)-E. coli. All studies conducted AST, including disk diffusion (22/40) and minimum inhibitory concentration (MIC) (18/40). Over half (24/40) used breakpoints recommended by the Clinical and Laboratory Standards Institute guidelines (CLSI), while 4/40 used the European Committee on Antimicrobial Susceptibility Testing guidelines (EUCAST), 7/40 reported both EUCAST and CLSI, 3/40 applied the British Society for Antimicrobial Chemotherapy guidelines (BSAC), and 2/40 did not report using any specific guidelines.
3.3 Reported risk factors
The most commonly investigated predictor variables for AMR-Enterobacterales carriage in dogs were antimicrobial use (28/40), age (24/40), sex (22/40), hospitalization (19/40) and raw diet (14/40) (Table 1). The most frequent statistically significant risk factor identified was previous antimicrobial use (19/28). Five studies reported previous use of antibiotics (without specifying the antibiotic classes) to be significantly linked with ESBL- and carbapenem-resistant-E. coli (22–26). With respect to the time frame of antibiotic use, six studies revealed a positive association between the timing of antimicrobial administration (from 10 days to one year) with ESBL, ampicillin-resistant (AmpC), and 3GCR-E. coli carriage (14, 27–31).
For specific antimicrobial classes, seven studies investigated specific classes of antimicrobials, of which, four reported the prior use of fluoroquinolones as significantly associated with ESBL, quinolone-resistant, and MDR-E. coli (15, 32–34). One study associated fluoroquinolones, cephalosporins, penicillin beta-lactamase with 3-GCR, AmpC-producing, and fluoroquinolone-resistant E. coli (35). One study reported fluoroquinolones and cephalosporins to be associated with ESBL-E. coli (32), while another study associated using cephalosporins and nitroimidazole with MDR-E.coli (36).
Lastly, one study showed that antimicrobial use in any household animal species in the past 6 months and purchase of antimicrobials from a pet food store were significantly associated with 3-GCR and ESBL-E. coli (14).
Other common risk factors found to be significantly associated with AMR- Enterobacterales carriage in dogs were raw diet (9/14) and hospitalization (8/19). Long duration of hospitalization (≥3 days) increased the risk of acquiring ESBL-E. coli, ESBL-K. pneumoniae and MDR-E. coli (4/8) (15, 36–38). In addition, being hospitalized and attending a referral consultation were found to be risk factors for ESBL- and AmpC-producing E. coli, respectively (3/8) (34, 35, 39). Feeding raw meat to dogs was associated with 3-GCR- and ESBL-E. coli in nine studies (13, 20, 27, 40–44), of which, two reported a significant association between raw chicken consumption and AMR Salmonella and E.coli (43, 44). However, no significant association was identified between the frequency of raw food consumption and the occurrence of AMR-bacteria carriage in these studies.
The odds ratios and corresponding confidence intervals for each factor (statistically significant or not), where reported in the 40 studies, are included in Supplementary Table 2.
3.4 Quality assessment (risk-of-bias)
The domain “masking” was removed from our analysis because it is not relevant to observational studies. The number of articles with low, unclear, or high risk-of-bias (of 40) for each domain is summarized in Figure 2. The main factors leading to a high risk of bias included non-random, small, convenient sampling (62.5% of studies), and the lack of information regarding the potential variables that could lead to bias (50%). In addition, in 80% of the studies, authors did not report how the sample size was calculated; therefore, the risk of bias regarding the study power was unclear.
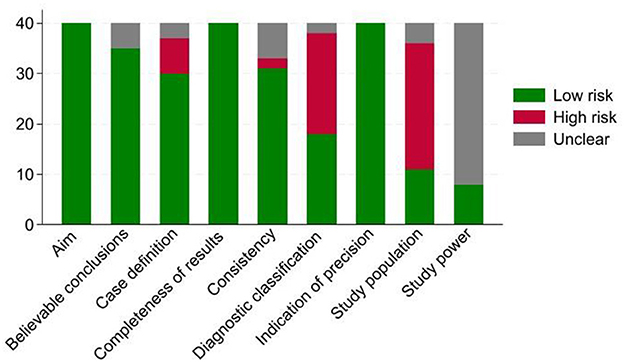
Figure 2. Frequency distribution of our 40 systematically reviewed studies categorized as having high, low, or unclear risk-of-bias by each domain; the framework was adapted from (78).
4 Discussion
To the best of our knowledge, this is the first comprehensive review synthesizing the risk factors associated with the carriage of AMR-Enterobacterales in dogs on a global scale. By compiling a diverse range of risk factors, we aimed to provide an overview of risk factors that could contribute to the acquisition and spread of AMR in dogs worldwide. The most commonly reported risk factor was antimicrobial use. Fluoroquinolones, cephalosporins, penicillin beta-lactamases, and nitroimidazoles were among the antimicrobial classes significantly associated with the third-generation cephalosporin-resistant, AmpC-producing, and quinolone-resistant-E. coli (35). This finding is likely due to the extensive use of these drugs in companion animal practices and veterinary medicine in general (45). In fact, fluoroquinolones are regarded as “highest priority, critically important antimicrobials” (CIAs) by the WHO, World Organization for Animal Health, and European Medicines Agency with high likelihood and consequences (risk) of AMR development (46, 47).
In addition to antimicrobial use, our study revealed several other medically important risk factors that may impact the carriage of AMR-Enterobacterales in dogs. Hospitalization was the third most common risk factor reported. Prolonged hospital stay is associated with an increased probability of exposure to contaminated surfaces and objects, contact with other hospitalized animals and interaction with the hospital personnel (48). These factors are known to contribute to the transmission of AMR-bacteria in human-based studies as well (49). Two studies have conducted an in-depth analysis of risk factors in veterinary hospitals, examining specific hospital units, such as oncology, neurology, orthopedics, cardiology and others (37, 50). Among these factors, exposure to anesthesia, surgery, diagnostic imaging and intubation were found to have a significant association with the acquisition of carbapenem-resistant and blaNDM − 5 carbapenem-resistant E. coli (50). These findings are consistent with other published studies in humans, which have highlighted the significance of medical services and devices in patient care as a contributing factor to the transmission of infections and AMR-bacteria (51, 52). However, unlike in human healthcare settings, in veterinary medicine, reusing some devices, such as endotracheal tubes, is claimed to be relatively common which can pose a serious risk regarding the acquisition and spread of AMR-pathogens (50). These findings call for strengthening infection prevention and control procedures, and implementing active surveillance in veterinary clinics and hospitals, particularly in developing countries.
A significant association between chronic or recurrent diseases and MDR-E. coli and ESBL-E. coli was reported in two studies (out of 14), where dogs with chronic urinary tract infections (UTIs) previously treated with antimicrobials were found to be eight times more likely to carry MDR-E. coli compared to dogs with acute UTIs and no history of prior antimicrobial treatment (23, 35). These findings suggested that the prolonged use of antimicrobials for the treatment of chronic diseases can substantially contribute to an increased risk of acquiring AMR-E. coli infections. Interestingly, annual vaccination and heartworm preventive treatment within the past 6 months demonstrated a significant protective effect against AMR-Salmonella spp. and AMR-E.coli (53). Other studies have not reported this association, suggesting that these variables may have served as proxies for consistent exposure to veterinary care (44).
Some studies have shown that lifestyle and dietary choices can significantly influence resistance patterns. For example, nine (out of 14) studies that investigated raw diet, found a significant association between the consumption of raw meat and AMR-Salmonella spp., clavulanate-amoxicillin and third-generation cephalosporin-resistant E.coli. Consuming raw poultry meat was reported in two of these studies (43, 44). The high prevalence of ESBL-E.coli in chicken meat has been well-documented (54, 55). The administration of antimicrobials in food-producing animals, for growth promotion and/or disease treatment, has been identified as a significant risk factor in the spread of AMR-bacteria within the food chain (56, 57). In addition, the presence of environmentally persistent Salmonella in the pet food factory setting has been well documented, and biofilm formation may serve as a potential mechanism contributing to their long-term survival (58). The beliefs held by some pet owners that raw food is more “natural” and “healthier” compared to processed commercial foods may pose a risk to the animals through the acquisition and spread of various pathogens and AMR-bacteria (59, 60). This raises public health concerns due to the often frequent close contact between owners, pets, and their shared environment. Collective efforts to educate caregivers about the potential risks of raw feeding have been made in some developed countries by their respective veterinary associations; however, not adequate at a global scale (61). Caregivers should be educated on the potential risks of giving raw meat or meat products to their pets. In contrast, feeding supplements (e.g., vitamins and minerals), herbal products, proprietary dog treats and homemade cooked diets showed a protective effect against ciprofloxacin-resistant and MDR-E.coli (13, 24, 44, 62). The authors highlighted the scarcity of research investigating the protective effects of food supplements, particularly in small animal medicine. However, it is assumed that caregivers, who provide supplements to their dogs, care more about their health (i.e., a confounding factor effect). The observed protective associations of dog treats and homemade cooked diets can be attributed to the elimination of E. coli in the production process, particularly when heat treatment is used (24).
After antimicrobial use, age was the most commonly investigated factor (24/40). A higher prevalence of AMR was found in E. coli isolated from dogs older than 10 years compared to those isolated from younger dogs (1–3 years old) (27, 63). This was attributed to suppressed immunity in geriatric dogs and selection pressure from prior antimicrobial use in younger dogs (63). Sex was also a commonly investigated factor (22/40), with only two studies reporting significant associations with AMR-bacteria (53, 64). One study demonstrated that female dogs were at higher risk of carrying pAmpC-producing E. coli (31), potentially due to a higher incidence of bacterial infections in the lower urinary tract. In contrast, the other study reported a significantly higher prevalence of cephalosporin-resistant Enterobacterales in males (64), with no explanation for this observation. The fact that most studies (20/22) did not find any significant associations indicates that age and sex are not likely to be primary determinants for acquiring and spreading AMR-bacteria in dogs. However, in humans, a recent study conducted across 29 European countries found that the occurrence of methicillin-resistant Staphylococcus aureus increased with age, while the occurrence of aminopenicillin resistance in E.coli decreased with age (65). The authors also concluded that men generally exhibited a higher risk of antimicrobial resistance compared to women. These findings may suggest that future interventions aimed at reducing the burden of AMR in animals and humans should take into account the significant variations in AMR patterns and prevalence observed across different age groups and between sexes.
Two studies (out of 11) reported breed as a risk factor, indicating a higher prevalence of AMR-E.coli among terriers, herding dogs, and large mixed-breed dogs compared to other breeds (62, 63). The reported high prevalence was attributed to certain lifestyle aspects among large mixed–breed dogs, although no specific aspects were explicitly mentioned. In addition, 20% of these dogs were obtained from a humane society, where animals are in close contact, leading to a higher likelihood of acquiring AMR-bacteria (63). In another study, smaller dogs were also less likely to have resistant isolates of AmpC-producing E. coli compared to heavier ones (13). Dogs from shelters or breeders had also a higher risk of carrying AmpC-producing E. coli, potentially due to frequent antimicrobial use in breeding kennels and common amoxicillin-clavulanate acid use in veterinary medicine (31). Neutering status (i.e., being intact) was significantly associated with the carriage of the mobile colistin resistance gene, mcr-1 (32). They explained hormonal and behavioral changes resulting from ovariectomy/castration were expected to affect the composition and diversity of gut microbiota, potentially impacting the colonization of AMR genes; the opposite of what was observed in this study. However, intact dogs are expected to be more active and more likely to contact other dogs than neutered dogs in estrus, which could have encouraged them to acquire mcr-1-positive bacteria from other dogs and the environment (32).
Environmental factors, such as walking in the countryside, contact with puppies, access to compost, commercial poultry odors detected, living within 5 km of the nearest commercial food animal facilities, direct contact with livestock playing in the river, and having access to ditches/puddles were found to have a positive association with the carriage of AMR-Enterobacterales from the study dogs in seven of the reviewed studies. Environmental contamination through livestock farming practices, such as the use of poultry feces as fertilizers or the contamination of shared water sources, can contribute to the spread of AMR-bacteria among domestic animals (66). Playing in rivers and having access to puddles/ditches was positively associated with the third-generation cephalosporin (3GC)-resistant E.coli and ESBL-E.coli. Previous research has shown that contaminated water sources serve as distinct environments for the spread of AMR genes among pathogens (67). Access to compost was linked with multiclass-resistant E.coli in one study (62). However, it was not specified whether dogs consumed the compost or only had contact with it and the level of exposure was also unknown. Compost may contain animal by-products and the feces of wild animals, which could harbor AMR-bacteria (62).
A positive correlation for the carriage of the colistin resistance gene, mcr-1, was shown between dogs and their caregivers in one study (32), in which, dogs consuming human food (shared with their owners) had a higher prevalence of mcr-1 (24.5%) compared to those eating commercial dog food (14.3%). This finding highlighted the potential role of shared dietary habits and living areas in the transmission of the mcr-1 gene (32). Moreover, previous research has also shown the presence of genetically similar methicillin-resistant Staphylococcus pseudintermedius in patients, contact animals, and environmental samples within the same household (68). However, further studies should focus on the sources and genetic similarities of AMR bacteria between pets, owners, and their environment for a better understanding of the direction of transmission.
Administration of corticosteroids 1 month prior to the hospitalization was found to be significantly associated with ESBL-E.coli carriage in dogs (25). In humans, a significant association between corticosteroid use and carriage of ESBL-K. pneumoniae was reported in patients with rheumatic autoimmune diseases (69); however, the authors did not explain the possible reasons behind this association. Additional studies are required to provide further insights into the association between using corticosteroids and AMR. Moreover, the interval time between admission to the hospital and treatment with NSAIDs demonstrated a protective effect against the development of MDR-E.coli in one study (out of two) (36). While the authors did not provide any reasons for this effect, previous research in humans suggests that certain NSAIDs (acetylsalicylic acid, diclofenac, ibuprofen) have anti-biofilm activities in concentrations found in human pharmacokinetic studies (70–72). However, most of these studies were conducted in vitro and tested non-clinical bacterial isolates, which may not represent the bacterial populations that cause clinically relevant infections. Despite the promising potential of NSAIDs for controlling biofilm-related infections, there is a lack of research, particularly controlled clinical trials to support their use as anti-biofilm agents (73).
Attending dog daycare was associated with the carriage of AmpC producing E. coli in one study (62). The authors explained that while previous research had shown dogs within the same breeding kennel shed bacteria with greater similarity in resistance patterns, no studies had specifically investigated the AMR spread in dog daycare centers.
Coprophagic habits were also positively associated with ESBL-E. coli gut colonization and MDR-E. coli in two studies (33, 74). This common behavior in dogs allows the ingestion of gut microflora, including AMR-bacteria. Feces from animals undergoing antimicrobial treatments may contain drug residues that may further contribute to the selection for and spread of AMR-bacteria. This highlights the important role of veterinarians in hospitals and clinics in educating pet caregivers to prevent and control this behavior.
While this study was designed to transparently and objectively assess the selected studies using the risk-of-bias domains, there were some potential limitations, including small sample sizes, which were frequently reported as a study-level limitation. Many studies either did not conduct or report a sample size calculation. This was a common factor limiting the statistical power of the studies in determining significant risk factors. Another common limitation was the lack of clear information about sampling strategies. Based on the available information, we assumed that a convenient sampling method was applied in these studies, which may substantially limit the internal and external validity (generalizability) of some findings. Some studies also lacked detailed clinical histories, including important factors such as prior antimicrobial use, which could have confounded their results. Our final selection included studies conducted in all continents. However, inevitably, studies from English-speaking countries are relatively overrepresented because of our logistical limitations to include non-English literature. This may somewhat decrease the generalizability of our compiled findings to geographical locations with particular management conditions. The risk factors investigated and their statistical significance could have been affected in the individual studies by including dogs from a wide range of health states. In the diseased dog populations, compared to healthy ones, it is presumed that the likelihood of acquiring and disseminating AMR-bacteria can be higher due to various factors, such as the higher use of antimicrobials and exposure to hospital environments. Lastly, we need to emphasize that our approach of relying on the number of reports (i.e., agreement in findings of different studies) and the significance of reported factors can to some extent simplify the importance of these risk factors. Although we attempted to account for the magnitude of reported odds ratios, along with their corresponding confidence intervals (see Supplementary Table 2), clear inconsistency in reporting and substantial heterogeneity in the study designs and statistical methods precluded conducting a robust meta-analysis on the extracted odds ratios for the commonly investigated risk factors. The readers must keep this limitation in mind when interpreting our findings.
5 Conclusions
Based on our systematic review, a fair amount of evidence has already been published on potential risk factors associated with the carriage of AMR-Enterobacterales in dogs. Our summary has implications for small animal veterinary practitioners, public health authorities, and researchers who are actively involved in addressing the challenge of AMR. Antimicrobial use, feeding raw diets, and hospitalization were the most significant factors reported consistently in the reviewed literature. This highlights the need for implementation and maintaining high standards of antimicrobial stewardship to reduce our reliance on priority classes of antimicrobials and educate pet caregivers on proper diet for their dogs. While several other risk factors have been identified such as age, sex, breed, dog owner's related factors, or the use of medications other than antimicrobials, their potential associations with the carriage of AMR-Enterobacterales are somewhat conflicting or poorly understood. This was either due to the low number of studies specifically designed to address these factors or shortfalls in the sample size and/or study design prohibiting robust statistical analyses. Further studies directed at such potential risk factors are warranted as a comprehensive understanding of the underlying risk factors is crucial for the development of targeted, effective preventive strategies and interventions. Lastly, our quality assessment of the reviewed literature calls for maintaining high standards in study design and peer-review processes by the scientific journals.
Data availability statement
The original contributions presented in the study are included in the article/Supplementary material, further inquiries can be directed to the corresponding authors.
Author contributions
EK: Methodology, Project administration, Software, Writing – original draft, Writing – review & editing, Data curation, Formal analysis, Investigation. KC: Data curation, Formal analysis, Investigation, Writing – original draft, Writing – review & editing. BM: Data curation, Investigation, Writing – original draft, Writing – review & editing. BH: Data curation, Writing – original draft, Writing – review & editing. PB: Writing – original draft, Writing – review & editing, Validation. VB: Validation, Writing – original draft, Writing – review & editing. IE: Validation, Writing – original draft, Writing – review & editing, Conceptualization, Investigation, Resources, Supervision. ON: Conceptualization, Resources, Supervision, Validation, Writing – original draft, Writing – review & editing, Funding acquisition, Methodology, Project administration, Software.
Funding
The author(s) declare financial support was received for the research, authorship, and/or publication of this article. This project was supported by the internal fund provided by City University of Hong Kong (Project Number: 9610511).
Acknowledgments
The authors thank Prof. Dirk U. Pfeiffer for his input in designing the study and Ms. Celia Chow, librarian at City University of Hong Kong, for overseeing the search process.
Conflict of interest
The authors declare that the research was conducted in the absence of any commercial or financial relationships that could be construed as a potential conflict of interest.
Publisher's note
All claims expressed in this article are solely those of the authors and do not necessarily represent those of their affiliated organizations, or those of the publisher, the editors and the reviewers. Any product that may be evaluated in this article, or claim that may be made by its manufacturer, is not guaranteed or endorsed by the publisher.
Supplementary material
The Supplementary Material for this article can be found online at: https://www.frontiersin.org/articles/10.3389/fvets.2024.1447707/full#supplementary-material
References
1. Antimicrobial Resistance. (2023). Available at: https://www.who.int/news-room/fact-sheets/detail/antimicrobial-resistance (accessed May 5, 2024).
2. Murray CJL, Ikuta KS, Sharara F, Swetschinski L, Aguilar GR, Gray A, et al. Global burden of bacterial antimicrobial resistance in 2019: a systematic analysis. Lancet. (2022) 399:629–55.
3. O'Neill J. Tackling Drug-Resistant Infections Globally: Final Report and Recommendations. 2016: Government of the United Kingdom.
4. Velazquez-Meza ME, Galarde-López M, Carrillo-Quiróz B, Alpuche-Aranda CM. Antimicrobial resistance: One Health approach. Vet World. (2022) 15:743–9. doi: 10.14202/vetworld.2022.743-749
5. Marco-Fuertes A, Marin C, Lorenzo-Rebenaque L, Vega S, Montoro-Dasi L. Antimicrobial resistance in companion animals: a new challenge for the One Health approach in the European Union. Vet Sci. (2022) 9:208. doi: 10.3390/vetsci9050208
6. Guardabassi L, Schwarz S, Lloyd DH. Pet animals as reservoirs of antimicrobial-resistant bacteria: review. J Antimicrob Chemother. (2004) 54:321–32. doi: 10.1093/jac/dkh332
7. Wegener HC, Aarestrup FM, Gerner-Smidt P, Bager F. Transfer of antibiotic resistant bacteria from animals to man. Acta Vet Scand Suppl. (1999) 92:51–7.
8. Ljungquist O, Ljungquist D, Myrenås M, Rydén C, Finn M, Bengtsson B. Evidence of household transfer of ESBL-/pAmpC-producing Enterobacteriaceae between humans and dogs – a pilot study. Infect Ecol Epidemiol. (2016) 6:31514. doi: 10.3402/iee.v6.31514
9. Stenske KA, Bemis DA, Gillespie BE, D'Souza DH, Oliver SP, Draughon FA, et al. Comparison of clonal relatedness and antimicrobial susceptibility of fecal Escherichia coli from healthy dogs and their owners. Am J Vet Res. (2009) 70:1108–16. doi: 10.2460/ajvr.70.9.1108
10. Prato-Previde E, Basso Ricci E, Colombo ES. The complexity of the human–animal bond: Empathy, attachment and anthropomorphism in human–animal relationships and animal hoarding. Animals. (2022) 12:2835. doi: 10.3390/ani12202835
11. Walsh F. Human-animal bonds II: the role of pets in family systems and family therapy. Family Proc. (2009) 48:481–99. doi: 10.1111/j.1545-5300.2009.01297.x
12. Reygaert WC. An overview of the antimicrobial resistance mechanisms of bacteria. AIMS Microbiol. (2018) 4:482–501. doi: 10.3934/microbiol.2018.3.482
13. Schmidt VM, Pinchbeck GL, Nuttall T, McEwan N, Dawson S, Williams NJ. Antimicrobial resistance risk factors and characterisation of faecal E coli isolated from healthy Labrador retrievers in the United Kingdom. Prev Vet Med. (2015) 119:31–40. doi: 10.1016/j.prevetmed.2015.01.013
14. Mitman SL, Amato HK, Saraiva-Garcia C, Loayza F, Salinas L, Kurowski K, et al. Risk factors for third-generation cephalosporin-resistant and extended-spectrum β-lactamase-producing Escherichia coli carriage in domestic animals of semirural parishes east of Quito, Ecuador. PLOS Global Public Health. (2022) 2:e0000206. doi: 10.1371/journal.pgph.0000206
15. Ogeer-Gyles J, Mathews KA, Sears W, Prescott JF, Weese JS, Boerlin P. Development of antimicrobial drug resistance in rectal Escherichia coli isolates from dogs hospitalized in an intensive care unit. J Am Vet Med Assoc. (2006) 229:694–9. doi: 10.2460/javma.229.5.694
16. Page MJ, McKenzie JE, Bossuyt PM, Boutron I, Hoffmann TC, Mulrow CD, et al. The PRISMA 2020 statement: an updated guideline for reporting systematic reviews. Int J Surg. (2021) 88:105906.
17. Ouzzani M, Hammady H, Fedorowicz Z, Elmagarmid A. Rayyan—a web and mobile app for systematic reviews. System. Rev. (2016) 5:1–10. doi: 10.1186/s13643-016-0384-4
18. Higgins J, Green S. Cochrane Handbook for Systematic Reviews of Interventions. Wiley (2008). Available at: https://onlinelibrary.wiley.com/doi/book/10.1002/9780470712184
19. Aasmäe B, Volkova J, Häkkinen L, Orro T. In vitro antimicrobial resistance of intestinal Escherichia coli and enterococci in clinically healthy dogs in Estonia. Veterinarija ir Zootechnika (2015) 72:3–8.
20. Lefebvre SL, Reid-Smith R, Boerlin P, Weese JS. Evaluation of the risks of shedding Salmonellae and other potential pathogens by therapy dogs fed raw diets in Ontario and Alberta. Zoonoses Public Health. (2008) 55:470–80. doi: 10.1111/j.1863-2378.2008.01145.x
21. Guri A, Flaks-Manov N, Ghilai A, Hoshen M, Rimon OF, Ciobotaro P, et al. Risk factors for third-generation cephalosporin resistant Enterobacteriaceae in gestational urine cultures: A retrospective cohort study based on centralized electronic health records. PLoS One. (2020) 15:e0226515. doi: 10.1371/journal.pone.0226515
22. Menard J, Goggs R, Mitchell P, Yang Y, Robbins S, Franklin-Guild RJ, et al. Effect of antimicrobial administration on fecal microbiota of critically ill dogs: dynamics of antimicrobial resistance over time. Animal Microbiom. (2022) 4:9. doi: 10.1186/s42523-022-00178-9
23. Saputra S, Jordan D, Mitchell T, Wong HS, Abraham RJ, Kidsley A, et al. Antimicrobial resistance in clinical Escherichia coli isolated from companion animals in Australia. Vet Microbiol. (2017) 211:43–50. doi: 10.1016/j.vetmic.2017.09.014
24. Beining MW, Hartmann M, Luebke-Becker A, Guenther S, Schaufler K, Hille K, et al. Carriage of extended spectrum beta lactamase-producing Escherichia coli: prevalence and factors associated with fecal colonization of dogs from a pet clinic in Lower Saxony, Germany. Animals. (2023) 13:584. doi: 10.3390/ani13040584
25. Ortiz-Díez G, Mengíbar RL, Turrientes MC, Artigao MB, Gallifa RL, Tello AM, et al. Prevalence, incidence and risk factors for acquisition and colonization of extended-spectrum beta-lactamase- and carbapenemase-producing Enterobacteriaceae from dogs attended at a veterinary hospital in Spain. Comp Immunol Microbiol Infect Dis. (2023) 92:101922. doi: 10.1016/j.cimid.2022.101922
26. Dazio V, Nigg A, Schmidt JS, Brilhante M, Mauri N, Kuster SP, et al. Acquisition and carriage of multidrug-resistant organisms in dogs and cats presented to small animal practices and clinics in Switzerland. J Vet Intern Med. (2021) 35:970–9. doi: 10.1111/jvim.16038
27. Groat EF, Williams NJ, Pinchbeck G, Warner B, Simpson A, Schmidt VM. UK dogs eating raw meat diets have higher risk of Salmonella and antimicrobial-resistant Escherichia coli faecal carriage. J Small Anim Pract. (2022) 63:435–41. doi: 10.1111/jsap.13488
28. Salgado-Caxito M, Benavides JA, Munita JM, Rivas L, García P, Listoni FJP, et al. Risk factors associated with faecal carriage of extended-spectrum cephalosporin-resistant Escherichia coli among dogs in Southeast Brazil. Prev Vet Med. (2021) 190:105316. doi: 10.1016/j.prevetmed.2021.105316
29. Gandolfi-Decristophoris P, Petrini O, Ruggeri-Bernardi N, Schelling E. Extended-spectrum β-lactamase-producing Enterobacteriaceae in healthy companion animals living in nursing homes and in the community. Am J Infect Control. (2013) 41:831–5. doi: 10.1016/j.ajic.2012.11.013
30. Chen J, Huang HH, Chang SM, Scaria J, Chiu YL, Chen CM, et al. Antibiotic-resistant Escherichia coli and sequence type 131 in fecal colonization in dogs in Taiwan. Microorganisms. (2020) 8:1439. doi: 10.3390/microorganisms8091439
31. Belas A, Salazar AS, da Gama LT, Couto N, Pomba C. Risk factors for faecal colonisation with Escherichia coli producing extended-spectrum and plasmid-mediated AmpC β-lactamases in dogs. Vet Record. (2014) 175:101978. doi: 10.1136/vr.101978
32. Lei L, Wang Y, He J, Cai C, Liu Q, Yang D, et al. Prevalence and risk analysis of mobile colistin resistance and extended-spectrum β-lactamase genes carriage in pet dogs and their owners: a population based cross-sectional study. Emerg Microb Infect. (2021) 10:242–51. doi: 10.1080/22221751.2021.1882884
33. Leite-Martins LR, Mahú MIM, Costa AL, Mendes A, Lopes E, Mendonça DMV, et al. Prevalence of antimicrobial resistance in enteric Escherichia coli from domestic pets and assessment of associated risk markers using a generalized linear mixed model. Prevent Vet Med. (2014) 117:28–39. doi: 10.1016/j.prevetmed.2014.09.008
34. Cocca G, Piva S, Magno SD, Scarpellini R, Giacometti F, Serraino A, et al. Prevalence and patterns of antimicrobial resistance among Escherichia coli and Staphylococcus spin a veterinary university hospital. Vet Sci. (2021) 8:308. doi: 10.3390/vetsci8120308
35. Schmidt VM, Pinchbeck G, McIntyre KM, Nuttall T, McEwan N, Dawson S, et al. Routine antibiotic therapy in dogs increases the detection of antimicrobial-resistant faecal Escherichia coli. J Antimicrob Chemother. (2018) 73:3305–16. doi: 10.1093/jac/dky352
36. Gibson JS, Morton JM, Cobbold RN, Filippich LJ, Trott DJ. Risk factors for dogs becoming rectal carriers of multidrug-resistant Escherichia coli during hospitalization. Epidemiol Infect. (2011) 139:1511–21. doi: 10.1017/S0950268810002785
37. Hamilton E, Kruger JM, Schall W, Beal M, Manning SD, Kaneene JB. Acquisition and persistence of antimicrobial-resistant bacteria isolated from dogs and cats admitted to a veterinary teaching hospital. J Am Vet Med Assoc. (2013) 243:990–1000. doi: 10.2460/javma.243.7.990
38. Hamilton E, Kruger JM, Schall W, Beal M, Manning SD, Kaneene JB. Risk factors for multidrug-resistant Escherichia coli rectal colonization of dogs on admission to a veterinary hospital. Epidemiol Infect. (2011) 139:197–205. doi: 10.1017/S0950268810000798
39. Shnaiderman-Torban A, Navon-Venezia S, Kelmer E, Cohen A, Paitan Y, Arielly H, et al. Extended-spectrum β-lactamase-producing Enterobacterales Shedding by dogs and cats hospitalized in an emergency and critical care department of a veterinary teaching hospital. Antibiotics. (2020) 9:545. doi: 10.3390/antibiotics9090545
40. Ramos CP, Kamei CYI, Viegas FM, Barbieri Jd, Cunha JLR, Hounmanou YMG, et al. Fecal shedding of multidrug resistant Escherichia coli isolates in dogs fed with raw meat-based diets in Brazil. Antibiotics. (2022) 11:534. doi: 10.3390/antibiotics11040534
41. Sealey JE, Hammond A, Mounsey O, Gould VC, Reyher KK, Avison MB. Molecular ecology and risk factors for third-generation cephalosporin-resistant Escherichia coli carriage by dogs living in urban and nearby rural settings. J Antimicrob Chemother. (2022) 77:2399–405. doi: 10.1093/jac/dkac208
42. Mounsey O, Wareham K, Hammond A, Findlay J, Gould VC, Morley K, et al. Evidence that faecal carriage of resistant Escherichia coli by 16-week-old dogs in the United Kingdom is associated with raw feeding. One Health. (2022) 14:100370. doi: 10.1016/j.onehlt.2022.100370
43. Wedley AL, Dawson S, Maddox TW, Coyne KP, Pinchbeck GL, Clegg P, et al. Carriage of antimicrobial resistant Escherichia coli in dogs: prevalence, associated risk factors and molecular characteristics. Vet Microbiol. (2017) 199:23–30. doi: 10.1016/j.vetmic.2016.11.017
44. Leonard EK, Pearl DL, Janecko N, Finley RL, Reid-Smith RJ, Weese JS, et al. Risk factors for carriage of antimicrobial-resistant Salmonella spp and Escherichia coli in pet dogs from volunteer households in Ontario, Canada, in 2005 and (2006). Am J Vet Res. (2015) 76:959–68. doi: 10.2460/ajvr.76.11.959
45. Caneschi A, Bardhi A, Barbarossa A, Zaghini A. The use of antibiotics and antimicrobial resistance in veterinary medicine, a complex phenomenon: a narrative review. Antibiotics. (2023) 12:487. doi: 10.3390/antibiotics12030487
46. Collignon PC, Conly JM, Andremont A, McEwen SA, Aidara-Kane A, World Health Organization Advisory Group, et al. World Health Organization ranking of antimicrobials according to their importance in human medicine: a critical step for developing risk management strategies to control antimicrobial resistance from food animal production Clin Infect Dis. (2016) 63:1087–93. doi: 10.1093/cid/ciw475
47. Gehring R, Mochel JP, Schmerold I. Understanding the background and clinical significance of the WHO, WOAH, and EMA classifications of antimicrobials to mitigate antimicrobial resistance. Front Vet Sci. (2023) 10:1153048. doi: 10.3389/fvets.2023.1153048
48. Haque M, McKimm J, Sartelli M, Dhingra S, Labricciosa FM, Islam S, et al. Strategies to prevent healthcare-associated infections: a narrative overview. Risk Manag Healthc Policy. (2020) 13:1765–80. doi: 10.2147/RMHP.S269315
49. D'Accolti M, Soffritti I, Mazzacane S, Caselli E. Fighting AMR in the healthcare environment: microbiome-based sanitation approaches and monitoring tools. Int J Mol Sci. (2019) 20:1535. doi: 10.3390/ijms20071535
50. Lavigne SH, Cole SD, Daidone C, Rankin SC. Risk factors for the acquisition of a bla NDM-5 carbapenem-resistant Escherichia coli in a veterinary hospital. J Am Animal Hosp Assoc. (2021) 57:101–5. doi: 10.5326/JAAHA-MS-7105
51. Palacios-Baena ZR, Giannella M, Manissero D, Rodríguez-Baño J, Viale P, Lopes S, et al. Risk factors for carbapenem-resistant gram-negative bacterial infections: a systematic review. Clin Microbiol Infect. (2021) 27:228–35. doi: 10.1016/j.cmi.2020.10.016
52. Buhl M, Peter S, Willmann M. Prevalence and risk factors associated with colonization and infection of extensively drug-resistant Pseudomonas aeruginosa: a systematic review. Expert Rev Anti Infect Ther. (2015) 13:1159–70. doi: 10.1586/14787210.2015.1064310
53. Tuerena I, Williams NJ, Nuttall T, Pinchbeck G. Antimicrobial-resistant Escherichia coli in hospitalised companion animals and their hospital environment. J Small Anim Pract. (2016) 57:339–47. doi: 10.1111/jsap.12525
54. Sukkua K, Manothong S. Sukhumungoon Seroprevalence and molecular epidemiology of EAST1 gene-carrying Escherichia coli from diarrheal patients and raw meats. J Infect Dev Countries. (2017) 11:220–7. doi: 10.3855/jidc.6865
55. Hasib FMY, Magouras I, St-Hilaire S, Paudel S, Kamali M, Lugsomya K, et al. Prevalence and characterization of antimicrobial-resistant Escherichia coli in chicken meat from wet markets in Hong Kong. Front Vet Sci. (2024) 11:1340548. doi: 10.3389/fvets.2024.1340548
56. Gouvêa R, Dos Santos F, De Aquino M. Fluoroquinolones in industrial poultry production, bacterial resistance and food residues: a review. Brazil J Poultry Sci. (2015) 17:1–10. doi: 10.1590/1516-635x17011-10
57. Nekouei O, Checkley S, Waldner C, Smith BA, Invik J, Carson C, et al. Exposure to antimicrobial-resistant Escherichia coli through the consumption of ground beef in Western Canada. Int J Food Microbiol. (2018) 272:41–8. doi: 10.1016/j.ijfoodmicro.2018.02.022
58. Bashir A, Azeem A, Stedman Y, Hilton AC. Pet food factory isolates of Salmonella serotypes do not demonstrate enhanced biofilm formation compared to serotype-matched clinical and veterinary isolates. BioMed Res Int. (2019) 2019:8569459. doi: 10.1155/2019/8569459
59. Vecchiato CG, Schwaiger K, Biagi G, Dobenecker B. From nutritional adequacy to hygiene quality: a detailed assessment of commercial raw pet-food for dogs and cats. Animals. (2022) 12:2395. doi: 10.3390/ani12182395
60. Lumbis R, Chan DL. The raw deal: clarifying the nutritional and public health issues regarding raw meat-based diets. Vet Nurse. (2015) 6:336–41. doi: 10.12968/vetn.2015.6.6.336
61. Raditic DM. Insights into commercial pet foods. Vet Clini. (2021) 51:551–62. doi: 10.1016/j.cvsm.2021.01.013
62. Procter TD, Pearl DL, Finley RL, Leonard EK, Janecko N, Reid-Smith RJ, et al. A cross-sectional study examining the prevalence and risk factors for anti-microbial-resistant generic Escherichia coli in domestic dogs that frequent dog parks in three cities in south-western Ontario, Canada. Zoonoses Public Health. (2014) 61:250–9. doi: 10.1111/zph.12064
63. Ekakoro JE, Hendrix GK, Guptill LF, Ruple A. Antimicrobial susceptibility and risk factors for resistance among Escherichia coli isolated from canine specimens submitted to a diagnostic laboratory in Indiana, 2010-2019. PLoS ONE. (2022) 17:e0263949. doi: 10.1371/journal.pone.0263949
64. Umeda K, Hase A, Matsuo M, Horimoto T, Ogasawara J. Prevalence and genetic characterization of cephalosporin-resistant Enterobacteriaceae among dogs and cats in an animal shelter. J Med Microbiol. (2019) 68:339–45. doi: 10.1099/jmm.0.000933
65. Waterlow NR, Cooper BS, Robotham JV, Knight GM. Antimicrobial resistance prevalence in bloodstream infection in 29 European countries by age and sex: An observational study. PLoS Med. (2024) 21:e1004301. doi: 10.1371/journal.pmed.1004301
66. Salinas L, Loayza F, Cárdenas P, Saraiva C, Johnson TJ, Amato H, et al. Environmental spread of extended spectrum beta-lactamase (ESBL) producing Escherichia coli and ESBL genes among children and domestic animals in Ecuador. Environm Health Perspect. (2021) 129:027007. doi: 10.1289/EHP7729
67. Hayward C, Ross KE, Brown MH, Whiley H. Water as a source of antimicrobial resistance and healthcare-associated infections. Pathogens. (2020) 9:667. doi: 10.3390/pathogens9080667
68. Laarhoven LM, de Heus P, van Luijn J, Duim B, Wagenaar JA, van Duijkeren E. Longitudinal study on methicillin-resistant Staphylococcus pseudintermedius in households. PLoS ONE. (2011) 6:e27788. doi: 10.1371/journal.pone.0027788
69. Liu Y, Liu Y, Dai J, Liu A, Li Y, Xu J, et al. Klebsiella pneumoniae in patients with rheumatic autoimmune diseases: clinical characteristics, antimicrobial resistance and factors associated with extended-spectrum β-lactamase production. BMC Infect Dis. (2021) 21:1–10. doi: 10.1186/s12879-021-06055-1
70. Rosato A, Catalano A, Carocci A, Carrieri A, Carone A, Caggiano G, et al. In vitro interactions between anidulafungin and nonsteroidal anti-inflammatory drugs on biofilms of Candida. Bioorganic Med Chem. (2016) 24:1002–5. doi: 10.1016/j.bmc.2016.01.026
71. Leão C, Borges A, Simões M. NSAIDs as a drug repurposing strategy for biofilm control. Antibiotics. (2020) 9:591. doi: 10.3390/antibiotics9090591
72. Abbas HA, Atallah H, El-Sayed MA, El-Ganiny AM. Diclofenac mitigates virulence of multidrug-resistant Staphylococcus aureus. Arch Microbiol. (2020) 202:2751–60. doi: 10.1007/s00203-020-01992-y
73. Paes Leme RC, da Silva RB. Antimicrobial activity of non-steroidal anti-inflammatory drugs on biofilm: Current evidence and potential for drug repurposing. Front Microbiol. (2021) 12:707629. doi: 10.3389/fmicb.2021.707629
74. Shnaiderman-Torban A, Navon-Venezia S, Baron H, Abu-Ahmad W, Arielly H, Valenci GZ, et al. Prevalence and molecular characterization of extended-spectrum β-lactamase producing Enterobacterales in healthy community dogs in Israel. Antibiotics. (2022) 11:1069. doi: 10.3390/antibiotics11081069
75. Formenti N, et al. Extended-spectrum-β-lactamase-and AmpC-producing Escherichia coli in domestic dogs: spread, characterisation and associated risk factors. Antibiotics. (2021) 10:1251. doi: 10.3390/antibiotics10101251
76. Habib I, Mohteshamuddin K, Mohamed M-YI, Lakshmi GB, Abdalla A, Alkaabi ABA. Domestic pets in the united arab emirates as reservoirs for antibiotic-resistant bacteria: a comprehensive analysis of extended-spectrum beta-lactamase producing Escherichia coli prevalence and risk factors. Animals. (2023) 13:1587. doi: 10.3390/ani13101587
77. Gruel G, Couvin D, Guyomard-Rabenirina S, Arlet G, Bambou J-C, Pot M, et al. High prevalence of blaCTXM−1/IncI1-Iγ/ST3 plasmids in extended-spectrum β-lactamase-producing Escherichia coli isolates collected from domestic animals in guadeloupe (French West Indies). Front Microbiol. (2022) 13:882422. doi: 10.3389/fmicb.2022.882422
Keywords: antimicrobial resistance, risk factor, bacterial infection, dog, Enterobacterales
Citation: Karalliu E, Chung KY, MacKinnon B, Haile B, Beczkowski PM, Barrs VR, Elsohaby I and Nekouei O (2024) Risk factors for antimicrobial-resistant Enterobacterales in dogs: a systematic review. Front. Vet. Sci. 11:1447707. doi: 10.3389/fvets.2024.1447707
Received: 12 June 2024; Accepted: 23 September 2024;
Published: 07 October 2024.
Edited by:
Alda Natale, Experimental Zooprophylactic Institute of the Venezie (IZSVe), ItalyReviewed by:
Raul Alejandro Alegria, University of Chile, ChileJames Wabwire Oguttu, University of South Africa, South Africa
Copyright © 2024 Karalliu, Chung, MacKinnon, Haile, Beczkowski, Barrs, Elsohaby and Nekouei. This is an open-access article distributed under the terms of the Creative Commons Attribution License (CC BY). The use, distribution or reproduction in other forums is permitted, provided the original author(s) and the copyright owner(s) are credited and that the original publication in this journal is cited, in accordance with accepted academic practice. No use, distribution or reproduction is permitted which does not comply with these terms.
*Correspondence: Omid Nekouei, b21pZC5uZWtvdWVpQGNpdHl1LmVkdS5oaw==; Ibrahim Elsohaby, aWVsc29oYWJAY2l0eXUuZWR1Lmhr