- 1College of Life Science and Engineering, Northwest Minzu University, Lanzhou, China
- 2Department of Animal Science and Technology, Gansu Agriculture Technology College, Lanzhou, China
Intestinal microbiota community is an important factor affecting the nutritional and health status of poultry, and its balance is crucial for improving the overall health of poultry. The study aimed to investigate the effect of dietary supplementation with Glycyrrhiza uralensis extract (GUE), Lactobacillus acidophilus (Lac) and their combination (GL) on growth performance and intestinal health in broilers in an 84-day feeding experiment. Supplementary 0.1% GUE and 4.5×107 CFU/g Lac significantly increased average daily gain (ADG), and GL (0.1% GUE and 4.5×107 CFU/g Lac) increased ADG and average daily feed intake (ADFI), and decreased feed conversion rate (FCR) in broilers aged 29 to 84 d and 1 to 84 d. Dietary GUE, Lac and GL increased the superoxide dismutase (SOD) and glutathione peroxidase (GSH-PX) activity and decreased Malondialdehyde (MDA) content in the jejunum mucosa of broilers, and increased secretory IgA (sIgA) content in broilers at 84 d. Moreover, GUE, Lac and GL increased cecal microbial richness and diversity, and modulated microbial community composition. Both GUE and Lac reduced the harmful bacteria Epsilonbacteraeota, Helicobacter, and H. pullorum at 28 d and Proteobacteria, Escherichia, and E. coli at 84 d, while Lac and GL increased beneficial bacteria Lactobacillus and L. gallinarum at 28 d. Compared with individual supplementation, GL markedly increased the SOD activity and the sIgA content, and reduced Helicobacter and Helicobacter pullorum. In conclusion, GUE and Lactobacillus acidophilus as feed additives benefit growth performance and intestinal health, and their combined use shows an even more positive effect in broilers.
1 Introduction
With the continuous improvement of the scale and intensity of poultry farming, the production performance of poultry has been gradually improved. However, broilers are susceptible to external factors such as disease, nutrition, and environment due to the imperfect development of intestinal function and low immunity, which frequently result in poor health, enhanced stress response, and intestinal imbalance (1). In the era of a complete ban on antibiotics as feed additives and a focus on healthy breeding, the development and utilization of feed additives like medicinal plant extracts and probiotics have garnered much attention (2, 3).
Glycyrrhiza uralensis Fisch is a traditional medicinal and edible plant with a long history of dietary and pharmacological applications, and its edible and medicinal parts are the root and rhizome (4, 5). The primary active ingredients in Glycyrrhiza uralensis extract (GUE) include glycyrrhiza polysaccharides, triterpene saponins (glycyrrhizic acid, glycyrrhizinic acid, etc.), and flavonoids (chalcone, isoflavone, etc.) (6). GUE exhibits various pharmacological effects, such as anti-inflammatory (7), antioxidant (8), antiviral (9), immune regulation (10) and improvement intestinal microbiota (11). Lactobacillus acidophilus is a dominant microbiota in the gastrointestinal tract (GIT) of humans and animals, often used as a probiotics due to its health-promoting properties (12). Probiotics Lactobacillus is typically employed as Direct-Fed microbiota to poultry and other livestock to enhance intestinal health (13), enhancing immunity (14, 15) and reducing colonization of pathogens in the GIT (16).
The intestinal tract is a crucial organ for digestion and absorption of feed nutrients in animals, closely linked to a range of physiological and biochemical processes, contributing to the animal’s nutrition and overall health (17). The intestinal microbiota is critical for maintaining host intestinal health, acting as the organism’s “second brain,” preventing pathogen colonization, modulating the intestinal epithelial barrier and inflammatory response (18, 19). The colonization of gut microbiota is relatively stable, and their composition and structure are influenced by factors such as diet, age, and feeding methods in animals (20). Changes in the GIT microbial community impact feed efficiency, productivity, and the health of chickens (21). The cecum, with the highest microbial colonization and the most abundant and diverse microbial community in the broiler gut, is crucial for the overall performance in poultry (22, 23). Generally, the chyme stays in the cecum for an extended period, allowing thorough decomposition of many feed ingredients by microbiota to improve nutrient utilization (24). Therefore, the balance of cecum microbiota is essential for enhancing overall performance of poultry.
Several recent studies have confirmed that GUE (25, 26) and probiotics (27, 28) promote growth and improve intestinal health in broilers. However, the potential effects of the combination of GUE and Lactobacillus on growth performance and intestinal health are not yet fully understood. Thus, the purpose of the present study was to investigate the impact of GUE, Lactobacillus acidophilus and their combination on growth performance and intestinal health in Liangfenghua broiler chickens, a medium-growing broiler strain known for its popularity due to the excellent meat quality. The findings of this study would be beneficial in comprehending the intestinal microecology of chickens and offering novel perspectives on the upkeep of intestinal wellness in broiler chickens that are fed antibiotic-free diets.
2 Materials and methods
2.1 Ethics statement
All procedures involving in animals were performed following the Regulations for the Administration of Affairs Concerning Experimental Animals (Ministry of Science and Technology, China, 2004) and were approved and supervised by the Northwest Minzu University Animal Care and Use Committee (Permit No. xbmu-sm-20210130).
2.2 Experimental design and animal management
The Glycyrrhiza uralensis extract (GUE; prepared from the root of GU using decoction extraction and ethanol precipitation methods; Yalan Pharmaceutical Co, Gansu, China) and the Lactobacillus acidophilus (Lac; Zhongxin Bio-Technology Co., Hebei, China; 3 × 109 CFU/g) used in the present study were commercial products.
A total of 420 healthy one-day-old male Liangfenghua broiler chickens were randomly allocated into 4 dietary treatments, each with 7 replicates and 15 chickens per replicate. The treatment groups included: (1) basal diet (Con group); (2) basal diet supplemented with 0.1% GUE (GUE group); (3) basal diet supplemented with Lac at 4.5 × 107 CFU/g (Lac group); and (4) basal diet supplemented with 0.1% GUE and 4.5 × 107 CFU/g Lac (GL group). Table 1 presented the composition and nutritional levels of the basal diet, formulated in accordance with the “Broilers Feeding Standard in China” (NY/T 33-2004). The trial was conducted at Gansu Agricultural Vocational Farm Co. in Gansu, China.
Before the experiment commenced, the chicken coops and internal equipment were cleaned and fumigated for disinfection. Chicks aged 1–28 days were reared in three-layer ladder cages (1.2 × 0.9 × 1.0 m, length × width × height), with the coop preheated before the chicks entering. During the first week, the coop temperature was maintained at 34°C with a relative humidity (RH) of 50%. Subsequently, the temperature was reduced by 2°C per week until reaching 26°C, with RH at 45%. From 29 to 84 days old, the broilers were raised on the ground, ensuring the coop remained dry, hygienic, and well-ventilated. All the chickens were kept in a single room comprising six floor pens, each measuring 300 × 350 cm. Each pen had solid white plastic walls and was divided by wire mesh into 10 compartments. These compartments were equipped with a round feeder pan (diameter = 30 cm) and one nipple drinker. Cork shavings were used as litter, and the litter was replaced every 3 days. The chickens were exposed to 12-h light/dark cycles daily throughout the test period and had ad libitum access to feed and water. The broilers were vaccinated with Newcastle disease vaccine and the infectious bursal polyvalent vaccine on d 7 and 14 of the experiment, respectively.
2.3 Growth performance determination
All broilers were weighed at 1, 28, and 84 days of age after a 12-h fast. The feed intake per replicate was recorded daily to calculate the average daily gain (ADG), average daily feed intake (ADFI) and feed conversion rate (FCR, feed/gain). Chicken mortality was recorded after which performance parameters were corrected for mortality.
2.4 Sample collection
All birds were fasted for 12 h (overnight) prior to collecting test samples. On d 28 and 84, two broilers with similar body weight per replicate from each group were selected and euthanized by severing the jugular vein. The abdomen was disinfected with 75% ethanol, then immediately dissected. The entire intestine was carefully removed from the abdominal cavity, and the jejunum and cecum were separated with a sterile scalpel. The middle part of jejunum was cut longitudinally, washed with 4% phosphate buffer solution (PBS), and jejunal mucosa was scraped with sterilized slides, put into RNAase-free tubes, snap-frozen in liquid nitrogen, and stored at −80°C for the determination of antioxidant indexes and secretory IgA (sIgA) content. The cecum contents were carefully collected, homogenized with a sterile spatula, transferred to CryoPure Tubes (Sarstedt AG + Co., Nümbrecht, Germany), snap-frozen in liquid nitrogen and stored at −80°C until they were processed for microbial DNA analysis.
2.5 Intestinal antioxidant and sIgA analysis
The activity of superoxide dismutase (SOD), glutathione peroxidase (GSH-PX), and the content of Malondialdehyde (MDA) in the jejunum mucosa were measured by assay kit (Shanghai Gantu Biotechnology Co., China). The level of intestinal sIgA was determined by double antibody one-step sandwich enzyme-linked immunosorbent assay (ELISA; Shanghai Liquid Quality Testing Technology Co., China). All detection methods were performed according to the manufacturer’s instructions.
2.6 Intestinal microbial diversity analysis
Bacterial genomic DNA was isolated from the cecal contents using the TGuide S96 kit (DP812; Tiangen Biotech Co., China) following the manufacturer’s instructions. The purity and quality of the DNA were verified in 0.8% agarose gels. Subsequently, the full-length 16S rRNA gene was amplified using the primers (27F, AGRGTTTGA TYNTGGCTCAG and 1492 R, TASGGHTACCTTGTTASGACTT). The purified PCR products were used to construct the single-read sequencing library on the PacBio platform (Biomarker-Technologies Co., China), following the manufacturer’s specifications. SMRT-Link v8.0 was used to correct the original subreads to obtain Circular Consensus Sequencing (CCS) sequence. The lima v1.7.0 software was used to identify the CCS sequence of different samples through the barcode sequence and remove the chimera (UCHIME v4.2), and obtain effective-CCS sequences. The generated datasets were analyzed using USEARCH v10.0. High-quality sequences were clustered as operational taxonomic units (OTUs) based on 97% similarity. BMK Cloud1 was used for Alpha diversity, Beta diversity, and microbial composition analysis to investigate differences in samples among groups.
2.7 Statistics analysis
All statistical analyses were conducted using SPSS software (version 26.0; IBM Corp., Armonk, NY, United States), with the results reported as mean ± Standard Error of Means (SEM). A one-way ANOVA test for multiple comparisons, followed by Dunnett’s post-hoc test, was employed to assess statistical significance between groups. Histograms were plotted using GraphPad Prism 8.0 (GraphPad, Inc. La Jolla, CA, United States). A probability value of p < 0.05 or p < 0.01 was considered statistically significant. A trend in significance was acknowledged for 0.05 < p < 0.10.
Alpha diversity indices (ACE and Shannon indices) of the samples were evaluated using QIIME2 2020 software, and the significance of differences was confirmed using the Wilcoxon rank sum test. After the alpha diversity analysis, Partialleast squares discriminant analysis (PLS-DA)was conducted with QIIME software based on OTU level.
3 Results
3.1 Growth performance
Throughout the entire experiment, the broiler chickens maintained good health. The effects of supplements on the growth performance of broilers were presented in Table 2. There were no significant differences in body weight (BW) of broilers at 1 and 28 d of age among groups (p > 0.05). Likewise, no significant differences were observed in ADG, ADFI, and F/G among groups from 1 to 28 d of age (p > 0.05). At 84 d of age, the BW in the GUE, Lac and GL groups significantly increased (p < 0.05) compared to the Con group, with the GL group showing the greatest improvement (p < 0.05). From day 29 to 84 and from day 1 to 84, the ADG of broilers in the GUE and Lac groups significantly increased (p < 0.05) compared to that in the Con group, while there was no significant difference in ADFI (p > 0.05). However, the GL group had a significant increase (p < 0.05) in both ADG and ADFI, along with a marked decrease (p < 0.05) in F/G compared to the Con group. Additionally, the F/G from day 1 to 84 in the Lac group was significantly lower (p < 0.05) than in the Con group.
3.2 Intestinal antioxidant and sIgA
The effects of GUE, Lac and their combination on intestinal antioxidant capacity and sIgA content of broilers were presented in Table 3. In comparison to the Con group, the GUE, Lac and GL groups exhibited a significant increased SOD activity and a significant decreased MDA content (p < 0.05) in the jejunum mucosa of broilers at 28 and 84 d of age; the GSH-Px activity in broilers at 28 and 84 d of age in GUE and GL groups, and in broilers at 28 d of age in Lac group, significantly increased (p < 0.01). Additionally, a significantly higher SOD activity in broilers at 28 d of age was observed in the GL group compared to the GUE and Lac groups (p < 0.01). There was no significant difference in the sIgA content in the jejunum mucosa of broilers at 28 d of age among the groups (p > 0.05). However, the GUE, Lac and GL groups exhibited a significant increase of sIgA content at 84 d of age compared to the Con group (p < 0.01). Additionally, the sIgA content in the GL group was significantly higher than in the GUE group or Lac group (p < 0.05).
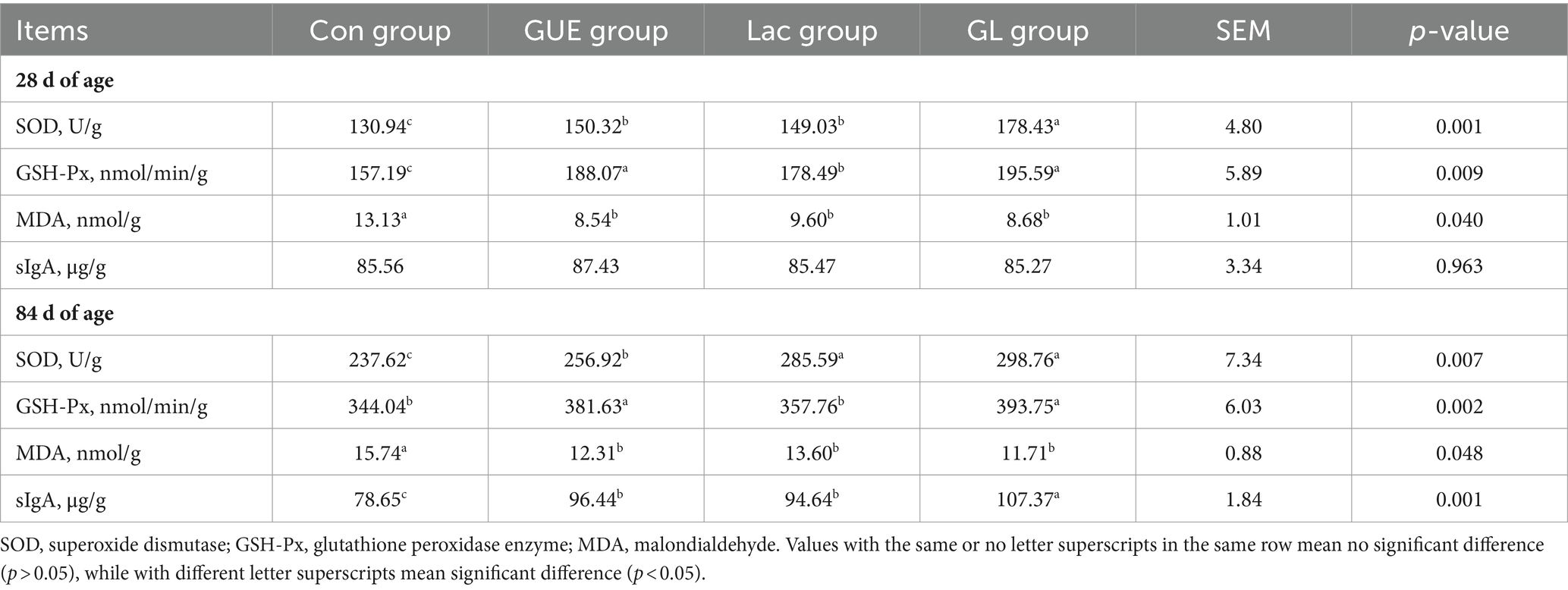
Table 3. Effect of dietary GUE, Lac and their combination on intestinal antioxidant indexes and sIgA content in broilers.
3.3 Variation in cecal microbiota diversity
3.3.1 Variation in alpha diversity
In the microbiome analysis, a total of 256,857 original CCS sequences were obtained through full-length 16S rRNA gene amplification on 40 cecal content samples using the PacBio platform. On average, 6,517 CCS sequences were generated in broilers at 28 d of age, with at least 4,400 CCS sequences for each sample. Similarly, an average of 6,326 CCS sequences were obtained in broilers at 84 d of age, with at least 4,074 CCS sequences for each sample. Following size filtering, quality control and chimera removal, a total of 199,146 high-quality sequences were retained, including 104,911 sequences at 28 d of age and 94,235 at 84 d of age.
At a 97% sequence similarity threshold, 322 OTUs were identified in samples from 28-d-old broilers in Con, GUE, Lac, and GL groups, with 5, 7, 13, and 17 unique OTUs, respectively (Figure 1A). At 84 d of age, 349 OTUs were identified in the four groups, with 13, 5, 5 and 10 unique OTUs, respectively (Figure 1B). The Shannon curves and the Rank abundance curve of cecal samples indicated that the sample size was reasonable, and the sequencing depth was sufficient for all samples based on a saturated trend (Figures 1C–F).
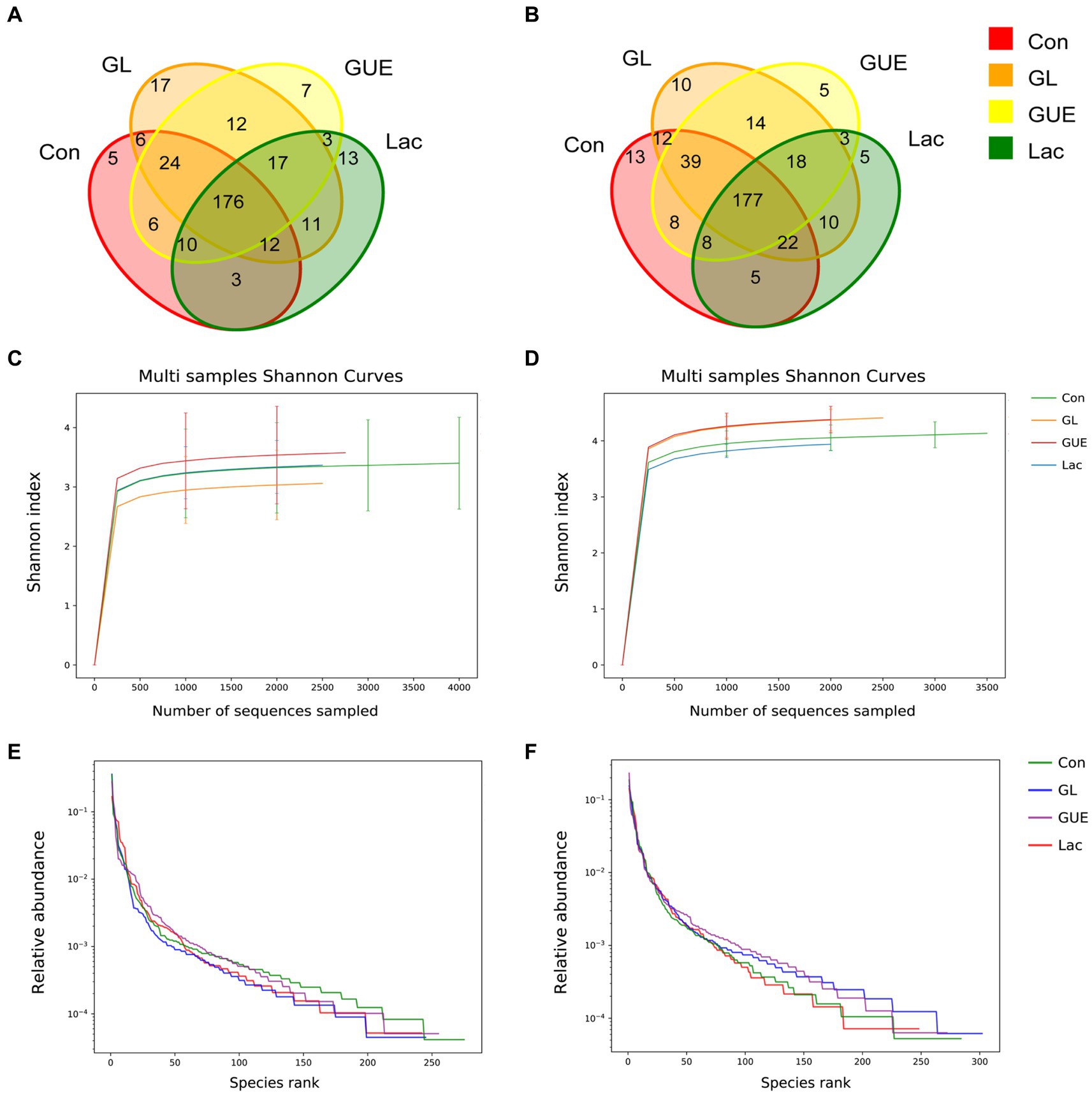
Figure 1. The Venn diagram, Shannon curves and Rank abundance curve of OTUs. (A,B) The Venn diagrams at 28 and 84 d of age. (C,D) The Shannon curves at 28 and 84 d of age. (E,F) The Rank abundance curve at 28 and 84 d of age.
The alpha diversity index serves as an indicator of the species richness and diversity within individual samples (29). To assess the alpha diversity of the samples, the ACE and Shannon indices were determined. At 28 d of age, there was no significant difference in the Shannon index among the groups (p > 0.05; Figure 2B). However, the ACE index in the GL group was significantly higher than in the Con group (p < 0.05; Figure 2A). In comparison with the GUE or Lac groups, there was a tendency to increase in the ACE index in the GL group (p = 0.095; Figure 2A). At 84 d of age, the ACE index (p = 0.095) and Shannon index (p = 0.056) showed a tendency to increase in the GL group compared to the GUE group or Lac group (Figures 2C,D).
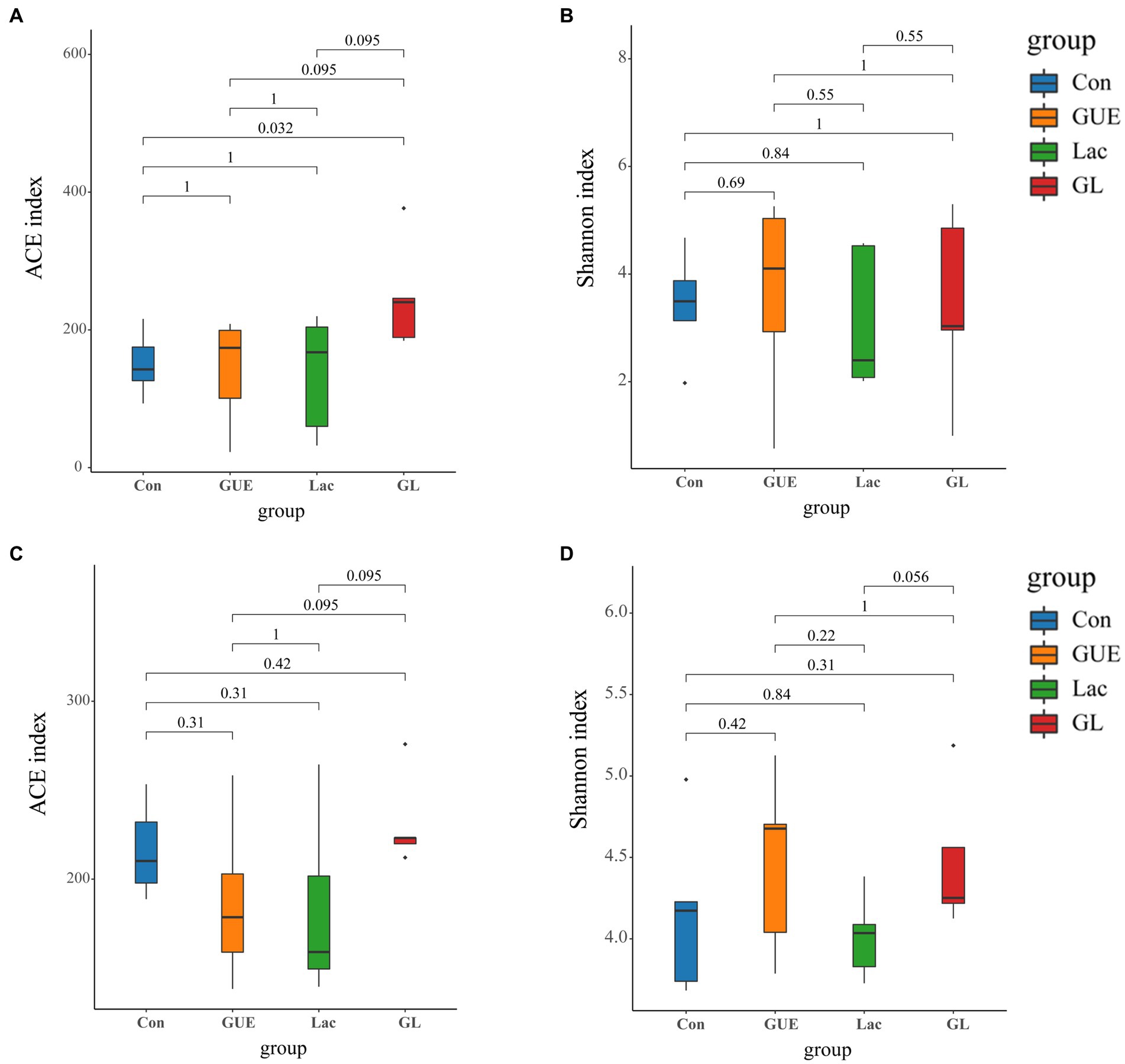
Figure 2. Alpha diversity of the microbiome residing in the cecal contents of broilers. (A,B) ACE and Shannon index in 28-d-old broilers. (C,D) ACE and Shannon index in 84-d-old broilers.
3.3.2 Variation in beta diversity
Beta diversity, a measure of variance in taxa composition between sampling sites (30), was visualized by plotting the distances between samples on Partial Least Squares Discriminant Analysis (PLS-DA) biplot. At 28 d of age, there was no significant separation observed between different treatment groups, and the distribution of samples within each group was discrete (Figure 3A). At 84 d of age, the intestinal microbial community exhibited a distinct separation among the Con, GUE, Lac, and GL groups, with samples clustering within each group (Figure 3B).
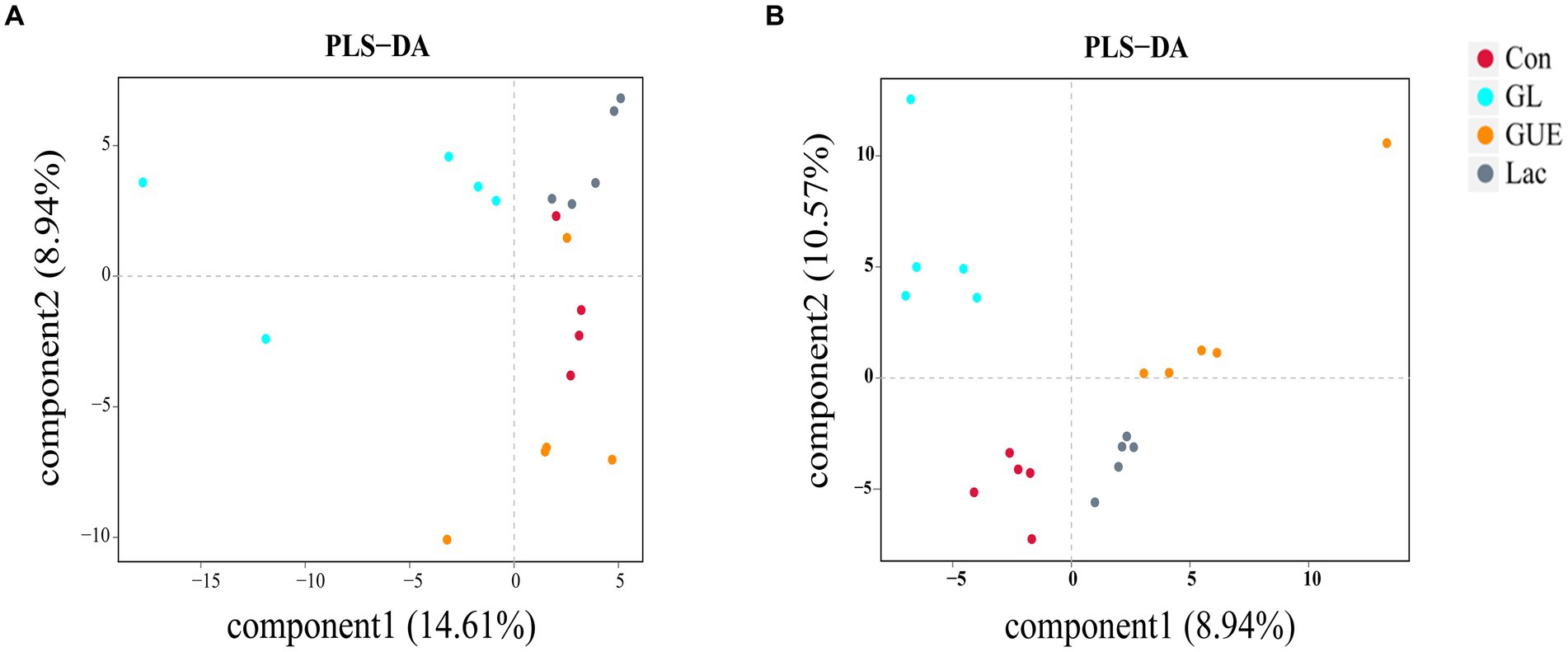
Figure 3. Beta diversity of the microbiome residing in the cecal contents of broilers. (A,B) Partial Least Squares Discriminant Analysis (PLS-DA) biplot at 28- and 84-d-old broilers.
3.4 Variation in cecal microbiota composition
3.4.1 Phylum level
The relative abundance of microbial composition at the phylum level was depicted in Figure 4. At 28 d of age, cecal samples in the Con, GUE, Lac, and GL groups were primarily dominated by five bacterial phyla: Firmicutes (59.24, 63.21, 78.93, and 86.13%, respectively), Bacteroidetes (28.16, 31.37, 16.27, and 8.52%, respectively), Epsilonbacteraeota (10.97, 1.30, 0.98, and 0.46%, respectively), Tenericutes (0.95, 1.98, 3.29, and 1.22%, respectively) and Proteobacteria (0.61, 2.00, 0.13, and 2.93%, respectively) (Figure 4A). Compared to the Con group, the relative abundance of Tenericutes and Proteobacteria significantly increased, while that of Epsilonbacteraeota significantly decreased in the GUE group. Similarly, the relative abundance of Firmicutes and Tenericutes significantly increased, while that of Bacteroidetes and Epsilonbacteraeota significantly decreased in the Lac group. In the GL group, the relative abundance of Firmicutes and Proteobacteria significantly increased, and that of Bacteroidetes and Epsilonbacteraeota significantly decreased (p < 0.05) (Figure 4C). Moreover, the relative abundance of Firmicutes and Proteobacteria significantly increased, while that of Bacteroidetes and Tenericutes significantly decreased in the GL group compared to the GUE or Lac group (p < 0.05) (Figure 4C).
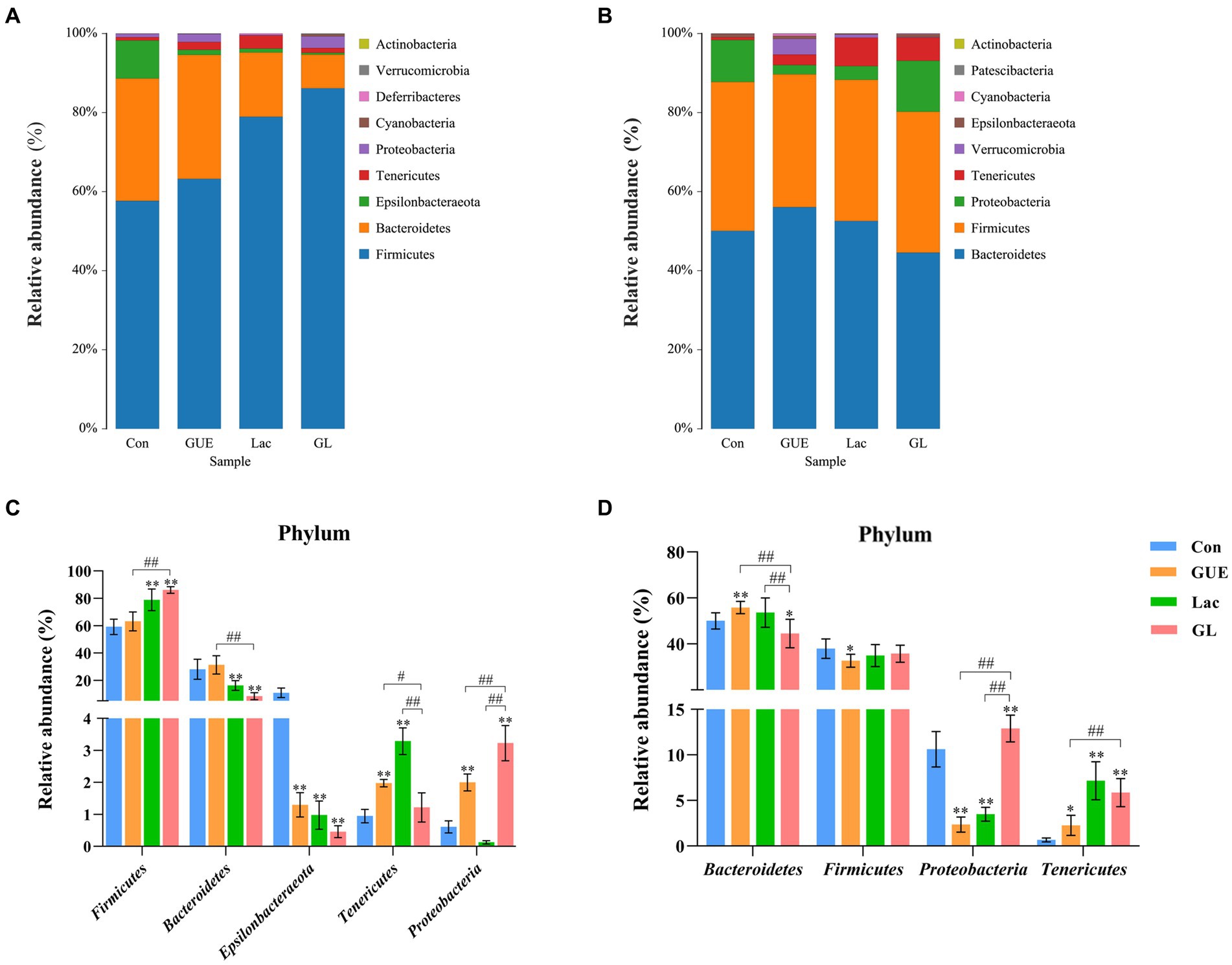
Figure 4. The relative abundance taxa of cecal microbiota in broiler chickens at the phylum level. (A,B) Relative abundance taxa at 28 and 84 d of age. (C,D) Relative abundance difference analysis of cecal bacterial species at the phylum level at 28 and 84 d of age, respectively. “*” indicated statistically significant difference from the Con group (*p < 0.05 and **p < 0.01). “#” indicated a statistical difference between the two groups (#p < 0.05 and ##p < 0.01).
At 84 d of age, cecal samples in the Con, GUE, Lac, and GL groups were dominated by four bacterial phyla: Bacteroidetes (50.06, 56.07, 52.55, and 44.55%, respectively), Firmicutes (37.68, 33.59, 35.73, and 35.65%, respectively), Proteobacteria (10.63, 2.36, 3.48, and 12.90%, respectively) and Tenericutes (0.66, 2.65, 7.17, and 5.87%, respectively) (Figure 4B). Compared to the Con group, the relative abundance of Bacteroidetes and Tenericutes significantly increased, while that of Firmicutes and Proteobacteria significantly decreased in the GUE group. Similarly, the relative abundance of Tenericutes significantly increased, while that of Proteobacteria significantly decreased in the Lac group. In the GL group, the relative abundance of Proteobacteria and Tenericutes significantly increased, while that of Bacteroidetes significantly decreased (p < 0.05) (Figure 4D). Additionally, the relative abundance of Proteobacteria and Tenericutes significantly increased, and that of Bacteroidetes significantly decreased in the GL group compared with the GUE or Lac group (p < 0.05) (Figure 4D). Thus, at the bacterial phylum level, the dietary supplements had a significant impact on the cecal microbial composition of broilers.
3.4.2 Genus level
The relative abundance of the microbial composition at the genus level was showed in Figure 5. At 28 d of age, cecal samples in the Con, GUE, Lac and GL groups were primarily dominated by five bacterial genera: Lactobacillus (33.23, 32.67, 54.19, and 50.98%, respectively), Barnesiella (13.01, 16.47, 7.74, and 0.12%, respectively), Alistipes (5.56, 11.58, 7.03, and 1.88%, respectively), Bacteroides (9.49, 3.22, 0.88, and 6.51%, respectively) and Helicobacter (10.95, 1.30, 0.98, and 0.23%, respectively) (Figure 5A). Among them, the relative abundance of Alistipes in the GUE group and Lactobacillus in Lac and GL groups was higher than in the Con group (p < 0.05), while the relative abundance of Helicobacter, Bacteroides and Barnesiella was lower in the GUE, Lac and GL groups than in the Con group (p < 0.05) (Figure 5C). Additionally, the relative abundance of Lactobacillus and Bacteroides was higher, and that of Barnesiella and Alistipes was lower in the GL group than in the GUE or Lac group (p < 0.05) (Figure 5C). The relative abundance of other genera in the top 20 taxa varied among groups.
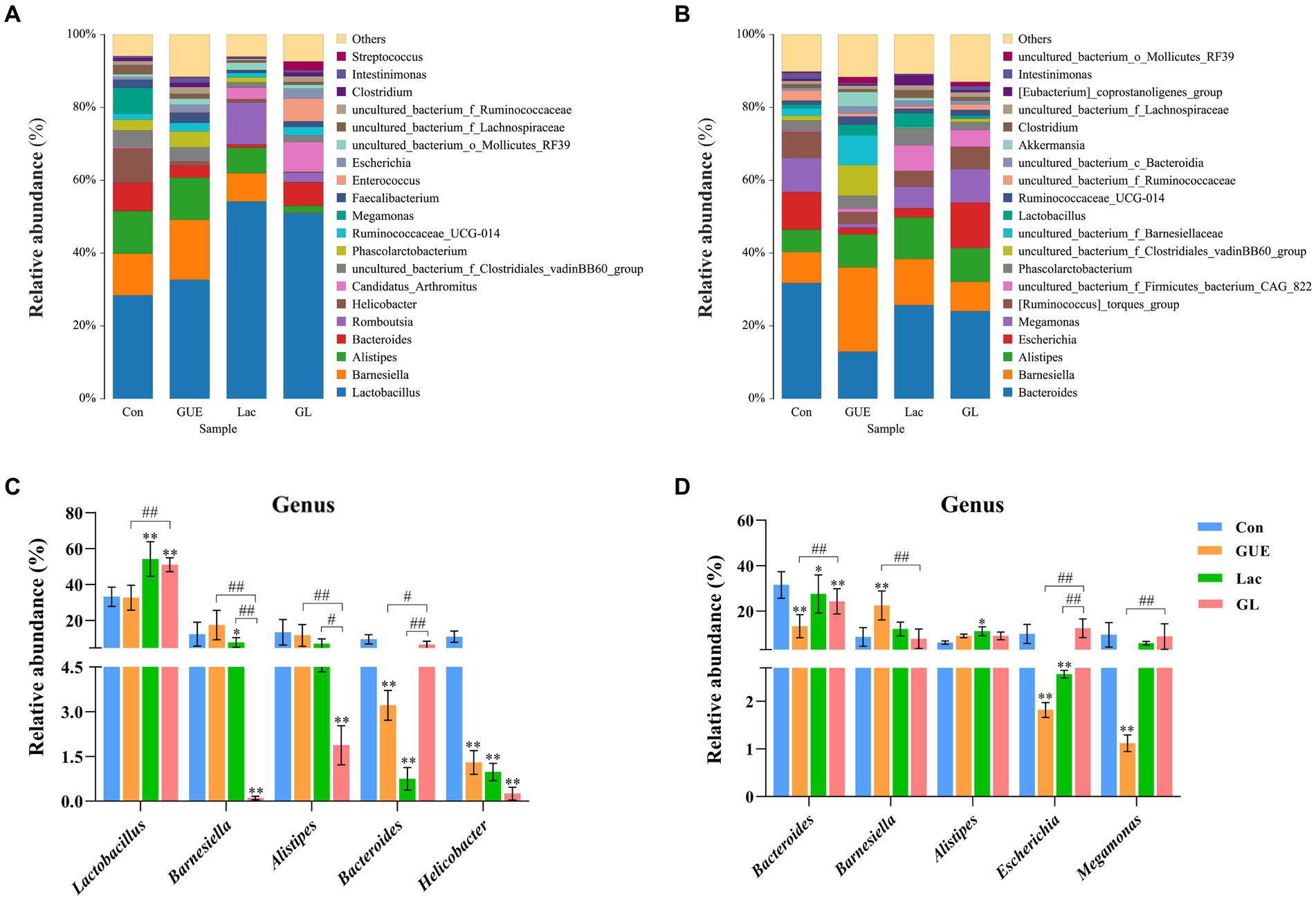
Figure 5. The relative abundance of cecal microbiota at the genus level. (A,B) Relative abundance taxa at 28 and 84 d of age. Relative abundances in the top 20 taxa were shown, and other taxon were combined as “Others.” (C,D) Relative abundance difference analysis of cecal bacterial species at the genus level at 28 and 84 d of age, respectively. “*” indicated statistically significant difference from the Con group (*p < 0.05 and **p < 0.01). “#” indicated a statistical difference between the two groups (#p < 0.05 and ##p < 0.01).
At 84 d of age, the most abundant taxa in the Con, GUE, Lac, and GL groups were Bacteroides (31.75, 12.93, 25.71, and 24.04%, respectively), Barnesiella (8.54, 23.15, 12.67, and 8.10%, respectively), Alistipes (6.15, 9.07, 11.40, and 9.24%, respectively), Escherichia (10.32, 1.82, 2.57, and 12.52%, respectively) and Megamonas (9.33, 1.12, 5.91, and 9.17%, respectively) (Figure 5B). These main bacterial genera showed significant differences among different treatment groups (p < 0.05) (Figure 5D). Additionally, the remaining bacterial genera also exhibited differences in relative abundances, such as [Ruminococcus]_torques_group, Phascolarctobacterium and Lactobacillus. In summary, the difference in supplements also affected the relative abundance of broiler cecal microbiota at the genus level.
3.4.3 Species level
The relative abundance of the microbial composition at the species level was presented in Figure 6. At 28 d of age, cecal samples in the Con, GUE, Lac, and GL groups was dominated by Lactobacillus_gallinarum, Alistipes_sp., Barnesiella_viscericola, Lactobacillus_salivarius, Bacteroides_fragilis, Helicobacter_pullorum, Candidatus_Arthromitus_sp., Barnesiella_intestinihominis, uncultured_bacterium_g_Romboutsia and Lactobacillus_reuteri (Figure 6A). Notably, Lactobacillus_gallinarum was the most dominant bacterium, accounting for more than 19% of the total microbial community detected. Compared to the Con group, the GUE, Lac and GL groups all significantly increased the relative abundance of Lactobacillus_gallinarum and significantly decreased that of Helicobacter_pullorum. Additionally, the GL group exhibited a significant increase in the relative abundance of Bacteroides_fragilis and Candidatus_Arthromitus_sp., and a decrease in Alistipes_sp., Barnesiella_viscericola and Barnesiella_intestinihominis compared to the GUE or Lac groups (Supplementary Table S1).
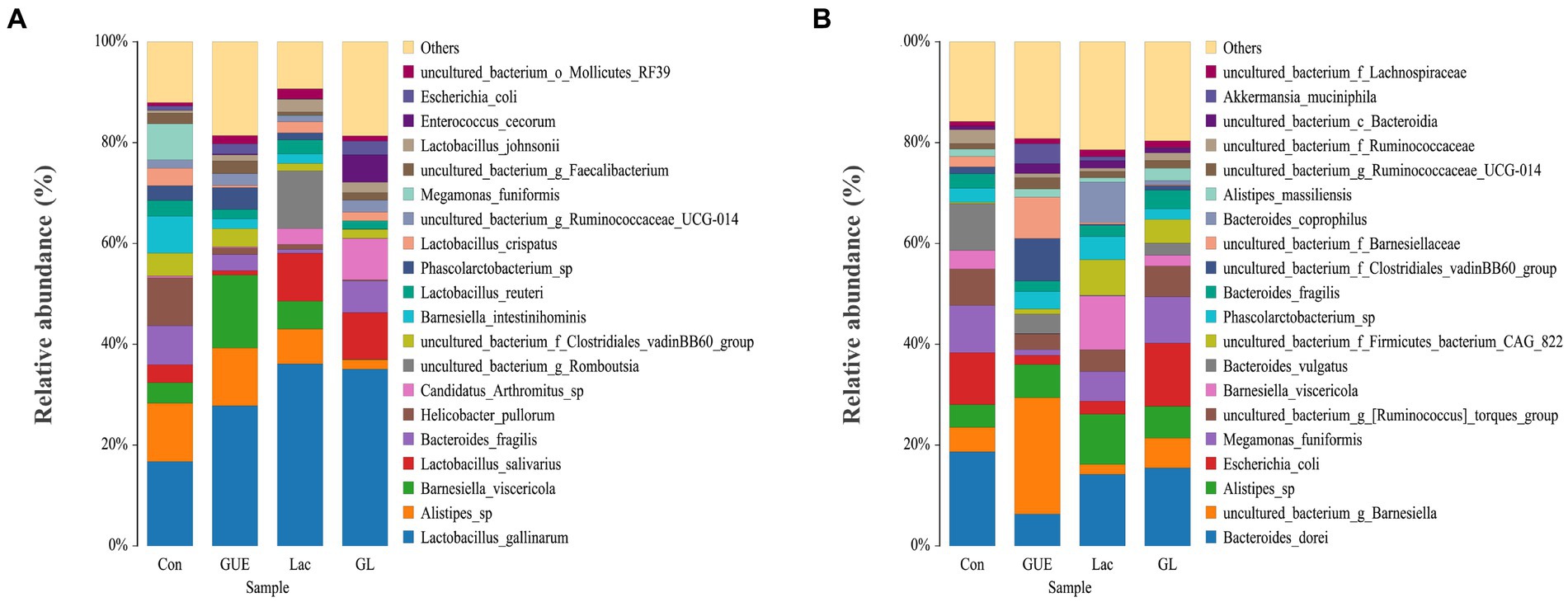
Figure 6. The relative abundance taxa of cecal microbiota in broiler chickens at the species level. (A,B) Relative abundance taxa at 28 and 84 d of age. Relative abundances in the top 20 taxa were shown, and other taxon were combined as “Others”.
At 84 d of age, the most abundant taxa in the Con, GUE, Lac, and GL groups were Bacteroides_dorei, uncultured_bacterium_g_Barnesiella, Alistipes_sp., Escherichia_coli, Megamonas_funiformis, uncultured_bacterium_g_[Ruminococcus]_torques_group, Barnesiella_viscericola, Bacteroides_vulgatus, uncultured_bacterium_f_Firmicutes_bacterium_CAG_822 and Phascolarctobacterium_sp (Figure 6B). Compared with the Con group, the relative abundance of Alistipes_sp was significantly higher, while that of Bacteroides_dorei and Bacteroides_vulgatus was significantly lower in the supplemented groups. Additionally, the GL group displayed a notable increase in the relative abundance of Megamonas_funiformis and uncultured_bacterium_g_[Ruminococcus]_torques_group, along with a decrease in Phascolarctobacterium_sp compared to the GUE or Lac groups (Supplementary Table S2). The relative abundance of other species in the top 20 taxa also exhibited variability across groups.
3.5 Differences between groups in microbial diversity
The Linear discriminant analysis (LDA) combined LDA effect size (LEfSe) method was used to further analyze the differential marker species in the cecal samples in groups. At 28 d of age, 4 significant biomarkers were enriched in the GL group, namely f_Clostridiaceae_1, g_Candidatus_Arthromitus, s_Candidatus_Arthromitus_sp., and f_Moraxellaceae (Figures 7A,B). At 84 d of age, 23 biomarkers were significantly enriched in the GUE, Lac and GL groups. Specifically, there were 8 species in the GUE group, including f_Barnesiellaceae, s_Lactobacillus_oris, f_Clostridiales_vadinBB60_group, 2 uncultured genera, and 3 unnamed species. The Lac group exhibited 5 species, including s_Barnesiella_visceriricola, g_Eubacterium_coprostanoligenes_group, g_Eisenbergiella, and 2 unnamed species. The GL group presented 10 species, including s_Escherichia_coli, g_Escherichia, f_Enterobacteriaceae, o_Enterobacterales, c_Gammaproteobacteria, p_Proteobacteria, s_Parabacteroides_distasonis, s_Butyricimonas_virosa, f_Tannerellaceae, and g_Parabacteroides (Figures 7C,D).
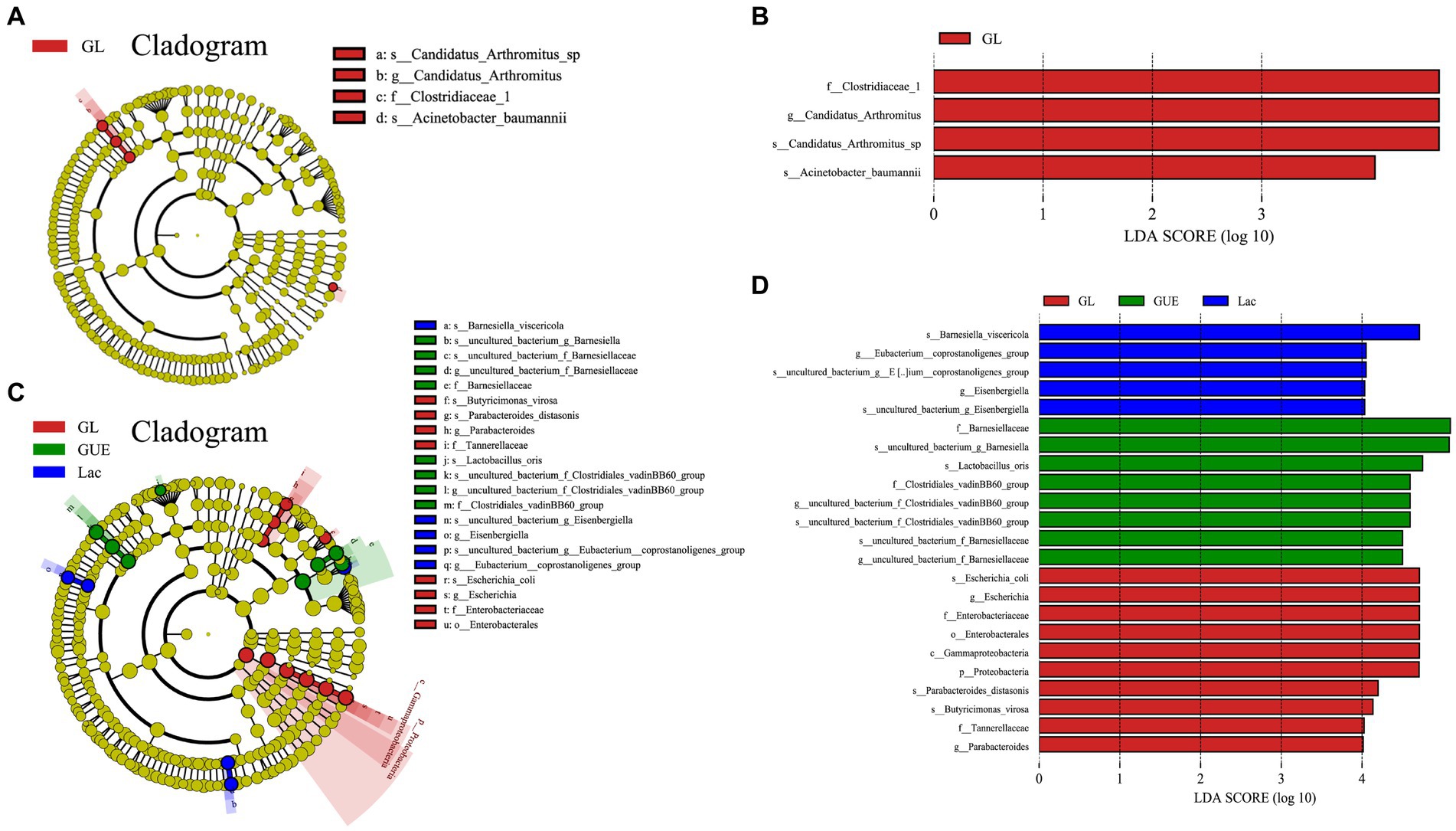
Figure 7. LEfSe taxonomic cladogram analysis and LDA score of cecal microbiota. (A,B) LEfSe taxonomic cladogram and LDA score at 28 d of age. (C,D) LEfSe taxonomic cladogram and LDA score at 84 d of age. The circles radiated from the center to the outer edges of the evolutionary branch map represent the classification level from the phylum to the species. The yellow nodes represent taxonomic units that show no significant differences between groups, and the size of the circle is positively correlated with relative abundance. Different colors indicate different groups. The length of the LDA histogram represents the LDA score, and the difference is significant when LDA > 4.0. p, phylum; c, class; o, order; f, family; g, genus; s, species.
4 Discussion
4.1 Growth performance
Probiotics, certain medicinal plants and their extracts are being viewed as promising alternatives to in-feed antibiotics due to their unique functions, including preventing intestinal diseases, enhancing overall health and performance of poultry. Numerous studies have reported that dietary supplementation with Lactobacillus acidophilus (31) and Lactobacillus plantarum (27) could enhanced the growth performance of broilers by improving intestinal health. Additionally, supplementation with GUE maintained intestinal barrier integrity and improved growth performance of broilers by up-regulating the expression of the intestinal barrier function factors junctional adhesion molecule (JAM) and mucin 2 (MUC-2) (26), and increased weight gain in the late growth and throughout the growth period of broilers reared at high-density (32).
In the present study, it was shown that supplementation with GUE, Lac, and their combination significantly boosted the BW of broilers at 84 d of age, and increased the ADG from d 29 to 84 and throughout the entire experiment, while no significant impact on chickens aged 1 to 28 d were observed. Additionally, the combination GUE and Lac had a higher ADG and a lower F/G in broilers aged 1 to 84 days compared to their individual supplementation. These findings suggested that both GUE and Lac enhanced the growth performance of broilers, and the combination of GUE and Lac had a noticeable synergistic effect.
4.2 Intestinal antioxidant and sIgA
As a channel for animals to communicate with the external environment, the intestine is susceptible to oxidative stress caused by various factors. Zhang et al. (33) found that dietary Glycyrrhiza polysaccharide significantly increased the expression of NF-E2-related factor 2 (Nrf-2), SOD1 and CAT in the jejunum mucosa, and improved the antioxidant capacity of piglets. Probiotics have the effect of resisting oxidative stress in the intestine, and dietary Lactobacillus plantarum could regulate the expression and production of antioxidant enzymes, thereby activating antioxidant defense system (34). Our results indicated that supplementation with GUE and Lac increased the activity of antioxidant enzyme SOD and GSH-Px and reduced MDA content in the jejunum mucosa of 28- and 84-d-old broilers. Furthermore, there was a synergistic effect of their combination. These results suggested that both GUE and Lac could enhance intestinal antioxidant capacity by regulating antioxidant enzyme activity.
SIgA is present in the intestinal mucosa, protects the intestine from pathogens, and regulates the intestinal microbiota throughout animal development (35). The deficiency of sIgA could lead to incomplete intestinal barrier function, resulting in decreased productivity and even death of poultry (36). As the predominant Ig isotype in the intestinal mucosa, sIgA inhibited abnormal epithelial cell translocation and preventing excessive inflammatory responses induced by lipopolysaccharide of Shigella (37). The increase of sIgA secretion contributed to the improvement of intestinal mucosal health, which led to the enhanced nutrient absorption and improved growth performance in broilers (38).
The present study found that GUE increased sIgA levels in the jejunum mucosa, indicating a potential enhancement of intestinal health in broilers, consistent with findings from Wu et al. (39). Probiotics, defined as “living microorganisms,” are known for their immunomodulatory and sIgA-inducing effects (40, 41). Probiotic supplementation enhanced the intestinal sIgA response, leading to changes in the overall intestinal microbiota structure and its interaction with sIgAs, thereby affecting host health (42). In this study, we observed that Lac supplementation notably raised sIgA levels in the jejunum mucosa of broilers, indicating its potential to enhance the intestinal health. Moreover, the combination of GUE and Lac exhibited a stronger effect in enhancing sIgA secretion compared to their individual supplementation.
4.3 Variation in cecal microbiota diversity
In this study, the full-length 16S rRNA gene of the cecal microbiota in broilers was sequenced on the Sequel II platform. Although previous study showed that supplementation of probiotic Lactobacillus increased intestinal microbial diversity under heat stress condition (43), our study found that dietary Lac or GUE had no significant effect on the α diversity based on the OTU level of 28- and 84-d-old broilers. Interestingly, the combination of GUE and Lac exhibited a significant synergistic effect on the ACE index.
Beta diversity analysis primarily describes variations in composition among microbiota (44). We observed that dietary GUE, Lac and their combined supplementation did not affect the structure of the cecal microbiota in broilers at 28 d of age, but had a distinct separation among groups at 84 d of age. Microbiota colonization in the cecum is a dynamic process influenced by various factors such as diet, disease defense, and host interactions. There were differences in microbiota composition at different growth stages of broiler (45). Moreover, microbial colonization in the intestine is affected by genetic background of the host, too. Liangfenghua broiler, a medium-speed growth broiler (46), might require an extended period for the colonization and accumulation of microbiota in the intestine.
In addition, LEfSe analysis indicated that only GL supplementation enriched 4 differential bacteria markers at 28 d of age, which were mainly included in Firmicutes and Proteobacteria. At 84 d of age, GUE enriched 8 specific bacteria, primarily within Bacteroidetes and Firmicutes, Lac enriched 5 differential bacteria, mainly involved in Bacteroidetes and Proteobacteria, and GL enriched 10 differential bacteria, primarily within Proteobacteria, Firmicutes and Bacteroidetes. From the above, each supplement had its unique microbial populations, suggesting that supplementation with GUE and Lac increased the abundance of cecal specific microbiota, with a synergistic effect from their combination. Furthermore, these also reflected that the diversity of intestinal microbiota in broilers increased with age until it stabilized, consistent with the findings of beta diversity.
4.4 Variation in cecal microbiota composition
The species annotation results were analyzed to understand the growth-promoting mechanisms of GUE, Lac, and their combination through intestinal microbiota. In this study, Firmicutes and Bacteroidetes were the most predominant, followed by Proteobacteriau and Tenericutes, with only a few of bacterial sequences in other phyla, which was consistent with Liu et al.’s research on broiler (47). Similar results were found in the study on the intestinal microbiota composition of Muscovy ducks (48), indicating that the intestinal microbiota composition of poultry is relatively stable. This study also observed that Firmicutes and Bacteroidetes were the two most dominant phyla in the cecal microbiota of 28-d-old broilers, while Bacteroidetes and Firmicutes predominated in that of 84-d-old broilers. These suggests that as broilers grow, their intestinal microbiota becomes more diverse, eventually establishing a complex and dynamic microbiome (49, 50). Furthermore, Firmicutes and Bacteroidetes collectively impacted the host’s energy absorption and storage, and the Firmicutes to Bacteroidetes (F / B) ratio in the GIT influenced the host’s ability to obtain energy from feed (51). A higher F / B ratio was often linked to enhanced growth performance (52). In this research, dietary Lac and the combination of GUE and Lac increased the F / B ratio in 28-d-old broilers, suggesting that these supplements could improve the weight gain by influencing cecal microbiota composition in broilers. Qiao et al. (53) found that adding compound polysaccharides derived from Astragalus and Glycyrrhiza to the diet improved broiler weight gain by raising the intestinal F / B ratio. Additionally, GUE, Lac, and their combination increased the relative abundance of Tenericutes and decreased that of Epsilonbacteraeota. In the research by Yang et al. (54), lentinan ameliorated intestinal microbiota dysbiosis in high-fat diet mice by decreasing the abundance of Epsilonbacteraeota.
Based on the analysis of cecal microbial composition at the genus and species levels, we found that Lac and the combination of GUE and Lac significantly increased the abundance of Lactobacillus and Lactobacillus gallinarum in the cecum of broilers at 28 d of age. Gut-residing Lactobacillus not only communicated with each other but also with the intestinal epithelial lining to balance the intestinal barrier integrity and mucosal barrier defense, and ameliorate the host immune responses (55). Under production conditions, Lactobacillus could colonize the GIT of broilers soon after hatching, and their metabolic activity reduced the pH of the chyme, which helped prevent the growth of harmful intestinal bacteria (56). L. gallinarum is beneficial to intestinal health, modulating intestinal microbial composition, secreting protective metabolites (57), improving the intestinal absorption capacity (58), and inhibiting the colonization of Salmonella in GIT (59). Conversely, Helicobacter and Escherichia easily colonize the intestines of humans and animals, causing various diseases by modulating the production of intestinal inflammatory factors and disrupting intestinal mucosal permeability, damaging the intestinal barrier (60–62). In this study, both GUE and Lac reduced the abundance of Helicobacter and Helicobacter pullorum in 28-d-old broilers, and Escherichia and Escherichia coli in 84-d-old broilers. The combination of GUE and Lac had a synergistic effect on reducing the abundance of Helicobacter and Helicobacter pullorum.
In this study, we also observed the GUE, Lac and their combination remarkably decreased the abundance of genus Bacteroides, specifically Bacteroides_fragilis and Barnesiella_intestinihominis in 28-d-old broilers, and Bacteroides_dorei and Bacteroides_vulgatus in 84-d-old broilers. Bacteroides was positively correlated with serum inflammatory cytokines TNF-a, IL-1β, and IL-6, and dietary supplementation with Glycyrrhiza polysaccharides suppressed the proliferation of Bacteroides (1). Bacteroides, a Gram-negative anaerobic bacterium, primarily achieved mutualism with the host through utilizing polysaccharides (63). The composition of Bacteroides is diverse and complex, playing a crucial role in various metabolic activities in animals (64). Certain Bacteroides species have pathogenic potential, promoting intestinal bacterial penetration and causing diarrhea by producing enterotoxins on the surfaces of intestinal epithelial cells (65). While some members of the phylum are part of the normal GIT microbiota, they may cause opportunistic infections if the intestinal mucosal barrier integrity is disrupted (66). This infection is typically triggered by various microorganisms, with B. fragilis being the most prevalent, found in the GIT of healthy individuals and associated with anaerobic bacteremia (67). B. dorei can cause intestinal inflammation and is recognized as a bacterial pathogen. Lan et al. (68) reported that α-glycerol monolaurate regulated the cecal microbiota of broilers at late growth stage by reducing the relative abundance of opportunistic pathogens such as B. dorei. Additionally, Bamba et al. (69) proposed a potential association between B. vulgatus and ulcerative colitis. The results mentioned above indicated that GUE and Lac could regulate the balance of intestinal microecology by increasing beneficial bacteria and reducing harmful bacteria in the cecum of broilers.
5 Conclusion
In summary, dietary GUE and L. acidophilus improved the growth performance of Liangfenghua broiler chickens, especially during the growing-finishing period, and enhanced intestinal health. Moreover, they increased the richness and diversity of cecal microbiota, and modulated the balance of intestinal microecology by increasing beneficial bacteria and reducing harmful bacteria in the cecum. The combined use of GUE and Lac had synergistic effects on growth performance, intestinal health, and microbiota composition. These findings suggest that the combination of GUE and L. acidophilus as feed additives has better application prospects in the poultry industry. However, further study is needed to understand the mechanism by which the combined supplementation of GUE and Lac affects the intestinal microbiota.
Data availability statement
The datasets presented in this study can be found in online repositories. The names of the repository/repositories and accession number(s) can be found in the article/Supplementary material.
Ethics statement
The animal study was approved by Northwest Minzu University Animal Care and Use Committee. The study was conducted in accordance with the local legislation and institutional requirements.
Author contributions
XL: Conceptualization, Data curation, Formal analysis, Investigation, Software, Validation, Writing – original draft, Writing – review & editing. JLi: Methodology, Resources, Validation, Writing – original draft. HY: Data curation, Methodology, Validation, Writing – original draft. YC: Software, Visualization, Writing – original draft. SL: Data curation, Methodology, Writing – original draft. SJ: Resources, Software, Writing – original draft. YZ: Formal analysis, Visualization, Writing – original draft. GZ: Conceptualization, Funding acquisition, Investigation, Supervision, Writing – review & editing. JLu: Conceptualization, Project administration, Supervision, Writing – review & editing.
Funding
The author(s) declare that financial support was received for the research, authorship, and/or publication of this article. This work was funded by the Fundamental Research Funds for the Central Universities of China (No. 31920240104).
Acknowledgments
We are grateful to Beijing Biomarker Technologies Corporation (Beijing, China) for assisting in sequencing.
Conflict of interest
The authors declare that the research was conducted in the absence of any commercial or financial relationships that could be construed as a potential conflict of interest.
Publisher’s note
All claims expressed in this article are solely those of the authors and do not necessarily represent those of their affiliated organizations, or those of the publisher, the editors and the reviewers. Any product that may be evaluated in this article, or claim that may be made by its manufacturer, is not guaranteed or endorsed by the publisher.
Supplementary material
The Supplementary material for this article can be found online at: https://www.frontiersin.org/articles/10.3389/fvets.2024.1436807/full#supplementary-material
Footnotes
References
1. Qiao, Y, Liu, C, Guo, Y, Zhang, W, Guo, W, Oleksandr, K, et al. Polysaccharides derived from Astragalus membranaceus and Glycyrrhiza uralensis improve growth performance of broilers by enhancing intestinal health and modulating gut microbiota. Poult Sci. (2022) 101:101905. doi: 10.1016/j.psj.2022.101905
2. Buntyn, JO, Schmidt, TB, Nisbet, DJ, and Callaway, TR. The role of direct-fed Microbials in conventional livestock production. Annu Rev Anim Biosci. (2016) 4:335–55. doi: 10.1146/annurev-animal-022114-111123
3. Park, JH, and Kim, IH. Supplemental effect of probioticBacillus subtilis B2A on productivity, organ weight, intestinalSalmonella microflora, and breast meat quality of growing broiler chicks. Poult Sci. (2014) 93:2054–9. doi: 10.3382/ps.2013-03818
4. Chen, C, Zhong, C, Gao, X, Tan, C, Bai, H, Ning, K, et al. Root-associated microbiota: the multifaceted hubs associated with environmental factors, growth status and accumulation of secondary metabolites. Environ Microbiome. (2022) 17:23. doi: 10.1186/s40793-022-00418-0
5. Zhong, C, Chen, C, Gao, X, Tan, C, Bai, H, and Ning, K. Multi-omics profiling reveals comprehensive microbe-plant-metabolite regulation patterns for medicinal plant Glycyrrhiza uralensis Fisch. Plant Biotechnol J. (2022) 20:1874–87. doi: 10.1111/pbi.13868
6. Li, T, Hua, S, Ma, J, Dong, L, Xu, F, and Fu, X. Spectrum-effect relationships of flavonoids in Glycyrrhiza uralensis Fisch. J Anal Methods Chem. (2020) 2020:8838290–11. doi: 10.1155/2020/8838290
7. Yang, R, Yuan, B-C, Ma, Y-S, Zhou, S, and Liu, Y. The anti-inflammatory activity of licorice, a widely used Chinese herb. Pharm Biol. (2016) 55:5–18. doi: 10.1080/13880209.2016.1225775
8. Zhang, C, Li, CX, Shao, Q, Chen, WB, Ma, L, Xu, WH, et al. Effects of Glycyrrhiza polysaccharide in diet on growth performance, serum antioxidant capacity, and biochemistry of broilers. Poult Sci. (2021) 100:100927. doi: 10.1016/j.psj.2020.12.025
9. Wang, J, Chen, X, Wang, W, Zhang, Y, Yang, Z, Jin, Y, et al. Glycyrrhizic acid as the antiviral component of Glycyrrhiza uralensis Fisch. Against coxsackievirus A16 and enterovirus 71 of hand foot and mouth disease. J Ethnopharmacol. (2013) 147:114–21. doi: 10.1016/j.jep.2013.02.017
10. Ayeka, PA, Bian, Y, Githaiga, PM, and Zhao, Y. The immunomodulatory activities of licorice polysaccharides (Glycyrrhiza uralensis Fisch.) in CT 26 tumor-bearing mice. BMC Complement Altern Med. (2017) 17:536. doi: 10.1186/s12906-017-2030-7
11. Yue, SJ, Qin, YF, Kang, A, Tao, HJ, Zhou, GS, Chen, YY, et al. Total flavonoids of Glycyrrhiza uralensis alleviates irinotecan-induced colitis via modification of gut microbiota and fecal metabolism. Front Immunol. (2021) 12:628358. doi: 10.3389/fimmu.2021.628358
12. Klaenhammer, TR, Altermann, E, Pfeiler, E, Buck, BL, Goh, YJ, O'Flaherty, S, et al. Functional genomics of probiotic lactobacilli. J Clin Gastroenterol. (2008) 42:S160–2. doi: 10.1097/MCG.0b013e31817da140
13. De Cesare, A, Sirri, F, Manfreda, G, Moniaci, P, Giardini, A, Zampiga, M, et al. Effect of dietary supplementation with Lactobacillus acidophilus D2/CSL (CECT 4529) on caecum microbioma and productive performance in broiler chickens. PLoS One. (2017) 12:e0176309. doi: 10.1371/journal.pone.0176309
14. Dalloul, RA, Lillehoj, HS, Shellem, TA, and Doerr, JA. Enhanced mucosal immunity against Eimeria acervulina in broilers fed a Lactobacillus-based probiotic. Poult Sci. (2003) 82:62–6. doi: 10.1093/ps/82.1.62
15. Brisbin, JT, Gong, J, Orouji, S, Esufali, J, Mallick, AI, Parvizi, P, et al. Oral treatment of chickens with lactobacilli influences elicitation of immune responses. Clin Vaccine Immunol. (2011) 18:1447–55. doi: 10.1128/CVI.05100-11
16. Ghareeb, K, Awad, WA, Mohnl, M, Porta, R, Biarnés, M, Böhm, J, et al. Evaluating the efficacy of an avian-specific probiotic to reduce the colonization of Campylobacter jejuni in broiler chickens. Poult Sci. (2012) 91:1825–32. doi: 10.3382/ps.2012-02168
17. Zhang, L, Wu, W, Lee, YK, Xie, J, and Zhang, H. Spatial heterogeneity and co-occurrence of mucosal and luminal microbiome across swine intestinal tract. Front Microbiol. (2018) 9:48. doi: 10.3389/fmicb.2018.00048
18. Sochocka, M, Donskow-Lysoniewska, K, Diniz, BS, Kurpas, D, Brzozowska, E, and Leszek, J. The gut microbiome alterations and inflammation-driven pathogenesis of Alzheimer's disease-a critical review. Mol Neurobiol. (2019) 56:1841–51. doi: 10.1007/s12035-018-1188-4
19. Roberts, T, Wilson, J, Guthrie, A, Cookson, K, Vancraeynest, D, Schaeffer, J, et al. New issues and science in broiler chicken intestinal health: intestinal microbial composition, shifts, and impacts. Worlds Poult Sci J. (2015) 71:259–70. doi: 10.1017/s0043933915000276
20. Dore, J, and Blottiere, H. The influence of diet on the gut microbiota and its consequences for health. Curr Opin Biotechnol. (2015) 32:195–9. doi: 10.1016/j.copbio.2015.01.002
21. Shang, Y, Kumar, S, Oakley, B, and Kim, WK. Chicken gut microbiota: importance and detection technology. Front Vet Sci. (2018) 5:254. doi: 10.3389/fvets.2018.00254
22. Glendinning, L, Watson, KA, and Watson, M. Development of the duodenal, ileal, jejunal and caecal microbiota in chickens. Anim Microbiome. (2019) 1:17. doi: 10.1186/s42523-019-0017-z
23. Mohd Shaufi, MA, Sieo, CC, Chong, CW, Gan, HM, and Ho, YW. Deciphering chicken gut microbial dynamics based on high-throughput 16S rRNA metagenomics analyses. Gut Pathogens. (2015) 7:4. doi: 10.1186/s13099-015-0051-7
24. Ricke, SC, Lee, SI, Kim, SA, Park, SH, and Shi, Z. Prebiotics and the poultry gastrointestinal tract microbiome. Poult Sci. (2020) 99:670–7. doi: 10.1016/j.psj.2019.12.018
25. Abo-Samaha, MI, Alghamdi, YS, El-Shobokshy, SA, Albogami, S, El-Maksoud, EMA, Farrag, F, et al. Licorice extract supplementation affects antioxidant activity, growth-related genes, lipid metabolism, and immune markers in broiler chickens. Life (Basel, Switzerland). (2022) 12:914. doi: 10.3390/life12060914
26. Ibrahim, D, Sewid, AH, Arisha, AH, Abd El-Fattah, AH, Abdelaziz, AM, Al-Jabr, OA, et al. Influence of Glycyrrhiza glabra extract on growth, gene expression of gut integrity, and Campylobacter jejuni colonization in broiler chickens. Front Vet Sci. (2020) 7:612063. doi: 10.3389/fvets.2020.612063
27. Wang, B, Gong, L, Zhou, Y, Tang, L, Zeng, Z, Wang, Q, et al. Probiotic Paenibacillus polymyxa 10 and Lactobacillus plantarum 16 enhance growth performance of broilers by improving the intestinal health. Anim Nutr (Zhongguo xu mu shou yi xue hui). (2021) 7:829–40. doi: 10.1016/j.aninu.2021.03.008
28. Qiu, K, Wang, X, Zhang, H, Wang, J, Qi, G, and Wu, S. Dietary supplementation of a new probiotic compound improves the growth performance and health of broilers by altering the composition of Cecal microflora. Biology. (2022) 11:633. doi: 10.3390/biology11050633
29. Shi, X, Huang, M, Song, J, Zeng, L, Liang, Q, Qu, Y, et al. Effects of different duck rearing systems on egg flavor and quality and microbial diversity. Poult Sci. (2022) 101:102110. doi: 10.1016/j.psj.2022.102110
30. Wagner, BD, Grunwald, GK, Zerbe, GO, Mikulich-Gilbertson, SK, Robertson, CE, Zemanick, ET, et al. On the use of diversity measures in longitudinal sequencing studies of microbial communities. Front Microbiol. (2018) 9:1037. doi: 10.3389/fmicb.2018.01037
31. Wu, Z, Yang, K, Zhang, A, Chang, W, Zheng, A, Chen, Z, et al. Effects of Lactobacillus acidophilus on the growth performance, immune response, and intestinal barrier function of broiler chickens challenged with Escherichia coli O157. Poult Sci. (2021) 100:101323. doi: 10.1016/j.psj.2021.101323
32. Rashidi, N, Ghorbani, MR, Tatar, A, and Salari, S. Response of broiler chickens reared at high density to dietary supplementation with licorice extract and probiotic. J Anim Physiol Anim Nutr. (2019) 103:100–7. doi: 10.1111/jpn.13007
33. Zhang, C, Li, C, Zhao, P, Shao, Q, Ma, Y, Bai, D, et al. Effects of dietary Glycyrrhiza polysaccharide supplementation on growth performance, intestinal antioxidants, immunity and microbiota in weaned piglets. Anim Biotechnol. (2023) 34:2273–84. doi: 10.1080/10495398.2022.2086878
34. Wang, J, Zhang, W, Wang, S, Wang, Y, Chu, X, and Ji, H. Lactobacillus plantarum exhibits antioxidant and Cytoprotective activities in porcine intestinal epithelial cells exposed to hydrogen peroxide. Oxidative Med Cell Longev. (2021) 2021:8936907–13. doi: 10.1155/2021/8936907
35. Hendrickx, AP, Top, J, Bayjanov, JR, Kemperman, H, Rogers, MR, Paganelli, FL, et al. Antibiotic-driven Dysbiosis mediates intraluminal agglutination and alternative segregation of Enterococcus faecium from the intestinal epithelium. MBio. (2015) 6:e01346–15. doi: 10.1128/mBio.01346-15
36. Bemark, M, Boysen, P, and Lycke, NY. Induction of gut IgA production through T cell-dependent and T cell-independent pathways. Ann N Y Acad Sci. (2012) 1247:97–116. doi: 10.1111/j.1749-6632.2011.06378.x
37. Boullier, S, Tanguy, M, Kadaoui, KA, Caubet, C, Sansonetti, P, Corthésy, B, et al. Secretory IgA-mediated neutralization of Shigella flexneri prevents intestinal tissue destruction by down-regulating inflammatory circuits. J Immunol. (2009) 183:5879–85. doi: 10.4049/jimmunol.0901838
38. Peng, Q, Zeng, XF, Zhu, JL, Wang, S, Liu, XT, Hou, CL, et al. Effects of dietary Lactobacillus plantarum B1 on growth performance, intestinal microbiota, and short chain fatty acid profiles in broiler chickens. Poult Sci. (2016) 95:893–900. doi: 10.3382/ps/pev435
39. Wu, Y, Wu, C, Che, Y, Zhang, T, Dai, C, Nguyễn, AD, et al. Effects of Glycyrrhiza polysaccharides on Chickens' intestinal health and homeostasis. Front Vet Sci. (2022) 9:891429. doi: 10.3389/fvets.2022.891429
40. Wan, LY, Chen, ZJ, Shah, NP, and El-Nezami, H. Modulation of intestinal epithelial defense responses by probiotic bacteria. Crit Rev Food Sci Nutr. (2016) 56:2628–41. doi: 10.1080/10408398.2014.905450
41. Wang, Y, Liu, L, Moore, DJ, Shen, X, Peek, RM, Acra, SA, et al. An LGG-derived protein promotes IgA production through upregulation of APRIL expression in intestinal epithelial cells. Mucosal Immunol. (2017) 10:373–84. doi: 10.1038/mi.2016.57
42. Han, X, Guo, J, Qin, Y, Huang, W, You, Y, and Zhan, J. Dietary regulation of the SIgA-gut microbiota interaction. Crit Rev Food Sci Nutr. (2023) 63:6379–92. doi: 10.1080/10408398.2022.2031097
43. Lan, PT, Sakamoto, M, and Benno, Y. Effects of two probiotic Lactobacillus strains on jejunal and cecal microbiota of broiler chicken under acute heat stress condition as revealed by molecular analysis of 16S rRNA genes. Microbiol Immunol. (2004) 48:917–29. doi: 10.1111/j.1348-0421.2004.tb03620.x
44. Cuccato, M, Rubiola, S, Giannuzzi, D, Grego, E, Pregel, P, Divari, S, et al. 16S rRNA sequencing analysis of the gut microbiota in broiler chickens prophylactically administered with antimicrobial agents. Antibiotics (Basel, Switzerland). (2021) 10:146. doi: 10.3390/antibiotics10020146
45. Zhou, Q, Lan, F, Li, X, Yan, W, Sun, C, Li, J, et al. The spatial and temporal characterization of gut microbiota in broilers. Front Vet Sci. (2021) 8:712226. doi: 10.3389/fvets.2021.712226
46. Sarica, M, Yamak, US, Boz, MA, Erensoy, K, Cilavdaroglu, E, and Noubandiguim, M. Performance of fast, medium and slow growing broilers in indoor and free-range production systems. South Afr J Anim Sci. (2020) 49:1127–38. doi: 10.4314/sajas.v49i6.16
47. Liu, YS, Li, S, Wang, XF, Xing, T, Li, JL, Zhu, XD, et al. Microbiota populations and short-chain fatty acids production in cecum of immunosuppressed broilers consuming diets containing γ-irradiated Astragalus polysaccharides. Poult Sci. (2021) 100:273–82. doi: 10.1016/j.psj.2020.09.089
48. Chen, X, Zheng, M, Lin, F, Cheng, X, Xiao, S, Chen, S, et al. Impacts of novel duck reovirus infection on the composition of intestinal microbiota of Muscovy ducklings. Microb Pathog. (2019) 137:103764. doi: 10.1016/j.micpath.2019.103764
49. Pan, D, and Yu, Z. Intestinal microbiome of poultry and its interaction with host and diet. Gut Microbes. (2013) 5:108–19. doi: 10.4161/gmic.26945
50. Brisbin, JT, Gong, J, and Sharif, S. Interactions between commensal bacteria and the gut-associated immune system of the chicken. Anim Health Res Rev. (2008) 9:101–10. doi: 10.1017/s146625230800145x
51. Han, GG, Lee, JY, Jin, GD, Park, J, Choi, YH, Chae, BJ, et al. Evaluating the association between body weight and the intestinal microbiota of weaned piglets via 16S rRNA sequencing. Appl Microbiol Biotechnol. (2017) 101:5903–11. doi: 10.1007/s00253-017-8304-7
52. Salaheen, S, Kim, SW, Haley, BJ, Van Kessel, JAS, and Biswas, D. Alternative growth promoters modulate broiler gut microbiome and enhance body weight gain. Front Microbiol. (2017) 8:2088. doi: 10.3389/fmicb.2017.02088
53. Qiao, Y, Guo, Y, Zhang, W, Guo, W, Oleksandr, K, Bozhko, N, et al. Effects of compound polysaccharides derived from Astragalus and Glycyrrhiza on growth performance, meat quality and antioxidant function of broilers based on serum metabolomics and Cecal microbiota. Antioxidants (Basel, Switzerland). (2022) 11:1872. doi: 10.3390/antiox11101872
54. Yang, X, Zheng, M, Zhou, M, Zhou, L, Ge, X, Pang, N, et al. Lentinan supplementation protects the gut-liver Axis and prevents steatohepatitis: the role of gut microbiota involved. Front Nutr. (2021) 8:803691. doi: 10.3389/fnut.2021.803691
55. Martín, R, Chamignon, C, Mhedbi-Hajri, N, Chain, F, Derrien, M, Escribano-Vázquez, U, et al. The potential probiotic Lactobacillus rhamnosus CNCM I-3690 strain protects the intestinal barrier by stimulating both mucus production and cytoprotective response. Sci Rep. (2019) 9:5398. doi: 10.1038/s41598-019-41738-5
56. Tannock, GW . A special fondness for lactobacilli. Appl Environ Microbiol. (2004) 70:3189–94. doi: 10.1128/aem.70.6.3189-3194.2004
57. Sugimura, N, Li, Q, Chu, ESH, Lau, HCH, Fong, W, Liu, W, et al. Lactobacillus gallinarum modulates the gut microbiota and produces anti-cancer metabolites to protect against colorectal tumourigenesis. Gut. (2021) 71:2011–21. doi: 10.1136/gutjnl-2020-323951
58. Madiha, K . Effect of Lactobacillus gallinarum PL 53 supplementation on xylose absorption and intestinal morphology in broilers challenged with Campylobacter jejuni. Pak Vet J. (2019) 40:163–8. doi: 10.29261/pakvetj/2019.011
59. Neveling, DP, van Emmenes, L, Ahire, JJ, Pieterse, E, Smith, C, and Dicks, LMT. Effect of a multi-species probiotic on the colonisation of Salmonella in broilers. Probiot Antimicrob Proteins. (2020) 12:896–905. doi: 10.1007/s12602-019-09593-y
60. Waite, DW, Vanwonterghem, I, Rinke, C, Parks, DH, Zhang, Y, Takai, K, et al. Comparative genomic analysis of the class Epsilonproteobacteria and proposed reclassification to Epsilonbacteraeota (phyl. Nov.). Front Microbiol. (2017) 8:682. doi: 10.3389/fmicb.2017.00682
61. Javed, S, Gul, F, Javed, K, and Bokhari, H. Helicobacter pullorum: an emerging zoonotic pathogen. Front Microbiol. (2017) 8:604. doi: 10.3389/fmicb.2017.00604
62. Shin, NR, Whon, TW, and Bae, JW. Proteobacteria: microbial signature of dysbiosis in gut microbiota. Trends Biotechnol. (2015) 33:496–503. doi: 10.1016/j.tibtech.2015.06.011
63. Comstock, LE . Importance of glycans to the host-bacteroides mutualism in the mammalian intestine. Cell Host Microbe. (2009) 5:522–6. doi: 10.1016/j.chom.2009.05.010
64. Rubio, LA, Peinado, MJ, Ruiz, R, Suárez-Pereira, E, Ortiz Mellet, C, and García Fernández, JM. Correlations between changes in intestinal microbiota composition and performance parameters in broiler chickens. J Anim Physiol Anim Nutr. (2015) 99:418–23. doi: 10.1111/jpn.12256
65. Wells, C, Evd, W, Jechorek, R, Feltis, B, Wilkins, T, and Erlandsen, S. Bacteroides fragilis enterotoxin modulates epithelial permeability and bacterial internalization by HT-29 enterocytes. Gastroenterology. (1996) 110:1429–37. doi: 10.1053/gast.1996.v110.pm8613048
66. Smith, CJ, Rocha, ER, and Paster, BJ. The medically important Bacteroides spp. in health and disease. Prokaryotes. (2006) 7:381–427. doi: 10.1007/0-387-30747-8_14
67. BG, SHW, Andrew, M, Lorraine, M, and Sheila, P. Binding and degradation of fibrinogen by Bacteroides fragilis and characterization of a 54 kDa fibrinogen-binding protein. Microbiology. (2010) 156:2516–26. doi: 10.1099/mic.0.038588-0
68. Lan, J, Chen, G, Cao, G, Tang, J, Li, Q, Zhang, B, et al. Effects of α-glyceryl monolaurate on growth, immune function, volatile fatty acids, and gut microbiota in broiler chickens. Poult Sci. (2021) 100:100875. doi: 10.1016/j.psj.2020.11.052
Keywords: Glycyrrhiza uralensis extract, Lactobacillus acidophilus, growth performance, intestine health, broiler chickens
Citation: Li X, Li J, Yuan H, Chen Y, Li S, Jiang S, Zha Xi Y, Zhang G and Lu J (2024) Effect of supplementation with Glycyrrhiza uralensis extract and Lactobacillus acidophilus on growth performance and intestinal health in broiler chickens. Front. Vet. Sci. 11:1436807. doi: 10.3389/fvets.2024.1436807
Edited by:
Yanting Chen, Nanjing Agricultural University, ChinaReviewed by:
Jesús Adonai Maguey-González, University of Arkansas, United StatesGuiling Ma, Nanjing Agricultural University, China
Copyright © 2024 Li, Li, Yuan, Chen, Li, Jiang, Zha Xi, Zhang and Lu. This is an open-access article distributed under the terms of the Creative Commons Attribution License (CC BY). The use, distribution or reproduction in other forums is permitted, provided the original author(s) and the copyright owner(s) are credited and that the original publication in this journal is cited, in accordance with accepted academic practice. No use, distribution or reproduction is permitted which does not comply with these terms.
*Correspondence: Guohua Zhang, MjgwMTEyMTAzQHhibXUuZWR1LmNu; Jianxiong Lu, MTY1ODYwNjE2QHhibXUuZWR1LmNu