- 1College of Animal Science and Technology, Hunan Agricultural University, Changsha, China
- 2Hunan Institute of Microbiology, Changsha, China
Oxidative damage resulting from weaning stress significantly impacts the growth performance and health status of piglets. Taurine, a dietary antioxidant with diverse functions, was investigated in this study for its protective role against weaning stress-induced oxidative damage and its underlying mechanism. Forty 28-day-old male castrated weaned piglets were randomly assigned to four groups. The control group received the basal diet, while the experimental groups were fed the basal diet supplemented with 0.1, 0.2%, or 0.3% taurine over a 28-day period. In vitro, H2O2 was utilized to induce oxidative damage to the jejunal mucosa of piglets via IPEC-J2 cells. The results demonstrated that taurine supplementation reduced the incidence of diarrhea in piglets compared to that in the control group (p < 0.05); the addition of 0.2 and 0.3% taurine led to increased average daily gain and improved feed conversion efficiency in weaned piglets, showing a linear dose-response correlation (p < 0.05). Taurine supplementation at 0.2 and 0.3% enhanced the activities of serum CAT and GSH-Px while decreasing the levels of serum NO, XOD, GSSG, and MDA (p < 0.05). Moreover, it significantly elevated the levels of GSS, Trx, POD, complex I, mt-nd5, and mt-nd6, enhancing superoxide anion scavenging capacity and the hydroxyl-free scavenging rate in the livers of weaned piglets while reducing NO levels in the liver (p < 0.05). Additionally, 0.2 and 0.3% taurine supplementation decreased serum IL-6 levels and elevated the concentrations of IgA, IgG, and IL-10 in weaned piglets (p < 0.05). The levels of occludin, claudin, and ZO-1 in the jejunum mucosa of weaned piglets increased with 0.2 and 0.3% taurine supplementation (p < 0.05). In IPEC-J2 cells, pretreatment with 25 mM taurine for 24 h enhanced the activities of SOD and CAT; reduced the MDA content; upregulated the mRNA expression of various genes, including ZO-1, occludin, claudin-1, Nrf2, and HO-1; and reversed the oxidative damage induced by H2O2 exposure (p < 0.05). Overall, the findings suggest that the inclusion of 2 and 3% taurine in the diet can enhance growth performance, reduce diarrhea rates, ameliorate oxidative stress and inflammation, and promote intestinal barrier function in weaned piglets.
1 Introduction
Overall, piglet breeding plays a vital role in the process of pig breeding. The proper feeding and management of piglets directly impact key metrics such as slaughter time, rate, and weight, thus influencing the production efficiency of pig breeding (1, 2). Weaning is a critical phase in the pig growth cycle and is highly susceptible to environmental stimuli, feed quality, mycotoxins, and pathogenic microorganisms. These factors discussed may result in an overproduction of intestinal reactive oxygen species (ROS) in piglets, leading to oxidative stress. This oxidative stress can subsequently trigger inflammatory damage to the intestinal mucosa and compromise barrier function. As a consequence, piglets may experience reduced growth performance, diarrhea, and potentially fatal outcomes, thereby imposing substantial economic burdens on the swine industry (3, 4).
Oxidative stress occurs when animals release oxidative free radicals that surpass the body’s antioxidant defenses, giving rise to various physiological or pathological manifestations (5, 6). Research indicates that the weaning process disrupts the redox balance in piglets, markedly reducing the activity of antioxidant enzymes while elevating free radicals and peroxide products, essentially inducing oxidative stress (7, 8). Cells respond to oxidative stress by activating various transcription factors, including nuclear factor E2-related factor 2 (Nrf2). Nrf2 plays a crucial role in regulating cellular redox balance, facilitating the expression of protective antioxidants, and initiating phase I detoxification reactions in mammals. It stands as a key target for the body in the management of oxidative stress (9). Dietary antioxidants such as anthocyanins and Vitamin E are potent natural antioxidants capable of efficiently scavenging free radicals, thereby safeguarding cells against oxidative damage and immune stress (10, 11). Consequently, enhancing the redox status of piglets is a crucial strategy for fostering healthy development.
Supplementing piglet diets with functional components has emerged as a feasible and effective approach for mitigating oxidative stress postweaning (7, 12). Taurine, a sulfur-containing nonprotein amino acid, plays pivotal roles in cellular processes such as volume regulation, osmotic pressure adjustment, protein phosphorylation, membrane stability, bile acid metabolism, neural modulation, calcium homeostasis, and detoxification and exhibits antioxidant and anti-inflammatory properties that are crucial for maintaining animal homeostasis (13, 14). Recent research underscores the ability of taurine to alleviate various oxidative damage-related ailments and its involvement in immune system modulation, offering significant benefits to animal production (15–17). Therefore, this study aimed to assess how taurine impacts growth performance, oxidative stress levels, immune function, and intestinal barrier integrity in weaned piglets.
2 Materials and methods
This animal study was reviewed and approved by the Hunan Agricultural University Institutional Animal Care and Use Committee (202105). Written informed consent was obtained from the owners for the participation of their animals in this study.
2.1 Materials
Taurine (purity ≥99%) and hydrogen peroxide (purity 30%) were purchased from Shanghai Aladdin Biochemical Technology Co., Ltd.
2.2 Experimental design and diets
Forty 28-day-old healthy castrated Duroc × Landrace × Large weaned piglets were randomly divided into 4 groups with 10 replicates in each group and 1 pig in each replicate. The plants were fed in a single pen and fed for 3 days. The control group was fed a basal diet, and the experimental group was fed a diet supplemented with 0.1, 0.2% or 0.3% taurine. The test period was 28 days. The basic diet was prepared according to the NRC 2012, and the feed composition and nutritional level are shown in Table 1. The piglet house was cleaned and disinfected before the experiment. During the experiment, the animals were fed twice a day, provided food freely and allowed to drink freely. The piglets were subjected to routine immunization procedures, and their health status was carefully observed and recorded every day.
2.3 Sample collection
After 12 h of fasting, the anterior vena cava of the piglets was collected on the morning of the 29th day of the experimental period. The whole blood was placed in an ordinary vacuum blood collection vessel. After standing at room temperature for 30 min, the mixture was centrifuged at 845 g and 4°C for 10 min, after which the supernatant was separated in a 1.5 mL EP tube. After quick freezing with liquid nitrogen, the samples were stored at −80°C. The patients were randomly anesthetized with pentobarbital sodium (40 mg/kg) and killed by bloodletting. The thoracic cavity and abdominal cavity were opened, and the liver and jejunum were separated. An appropriate amount of liver was removed from the frozen tube at the fixed position and stored at −80°C after quick freezing with liquid nitrogen. Approximately 20 cm of middle jejunum tissue was removed, and the tissue was rinsed with normal saline. The mucosal layer was scraped with a slide, collected in a cryopreservation tube for quick freezing with liquid nitrogen, and then stored at −80°C.
2.4 Growth performance
Piglets were weighed on an empty stomach on the mornings of day 1 and day 29 of the experiment. During the experiment, daily feed intake and residual feed weight were recorded to calculate the average daily gain (ADG), average daily feed intake (ADFI) and feed-to-gain ratio (F/G). The diarrhea of the piglets was observed and recorded at 5 PM every day, and the diarrhea rate (%) = number of piglets with diarrhea × 100/28.
2.5 Serum parameters
Serum catalase (CAT), glutathione peroxidase (GSH-Px), superoxide dismutase (SOD), total antioxidant capacity (T-AOC), superoxide anion scavenging capacity, hydroxyl radical scavenging rate, nitric oxide (NO), xanthine oxidase activity (XOD), glutathione (GSH), oxidized glutathione (GSSG), malondialdehyde (MDA) and peroxidase (POD) contents were detected by biochemical kits produced by Nanjing Jianchen Bioengineering Institute, China. Serum immunoglobulin A (IgA), immunoglobulin G (IgG), immunoglobulin M (IgM), interleukin-4 (IL-4), interleukin-10 (IL-10), interleukin-1β (IL-1β), interleukin-6 (IL-6), and tumor necrosis factor-α (TNF-α) were detected by ELISA kits, which were purchased from Jiangsu Enzyme Immunoassay Industry Co., Ltd., China. All procedures were performed in strict accordance with the kit instructions.
2.6 Analysis of hepatic oxidative status
The levels of glutathione synthase (GSS), thiredoxin (Trx), NO, superoxide anion scavenging capacity, hydroxyl radical scavenging rate, POD, complex I, mitochondrially encoded NADH dehydrogenase 5 (mt-ND5) and mitochondrially encoded NADH dehydrogenase 6 (mt-ND6) were measured. All operations were carried out in strict accordance with the instructions of the kit produced by Nanjing Jianchen Biotechnology Institute, China.
2.7 Analysis of jejunal mucosal barrier proteins
The levels of claudin-1, tight junction protein 1 (ZO-1) and Occludin in the jejunal mucosa were detected by ELISA kits purchased from Jiangsu Enzyme Immunoassay Industry Co., Ltd., China. All operations were carried out strictly according to the instructions of the kit.
2.8 Cell culture
Porcine jejunal epithelial cells (IPEC-J2) were cultured in complete DMEM/F12 supplemented with 10% FBS (OPCEL, Inner Mongolia Opcel Biotechnology Co., Ltd., BS1101) and 1% penicillin–streptomycin (Gibco, 15140-122) in a 5% CO2 cell incubator at 37°C. When the cells reached 80% confluence, they were washed twice with sterile PBS and then subcultured or treated with drugs. The cell seeding ratios for culture vessels of various sizes were as follows: 5 × 106 cells/cm2 for 100 mm cell culture dishes; 9 × 105 cells/cm2 for 6-well cell culture plates; and 3 × 104 cells/cm2 for 96-well cell culture plates.
2.9 Selection of the daidzein concentration
To determine the optimal taurine concentration, IPEC-J2 cells were seeded in 96-well cell culture plates with six replicates per treatment. After reaching approximately 80% confluency, the cells were subjected to two washes with sterile PBS. Subsequently, 100 μL of DMEM/F12 medium with various concentrations of taurosulfonate (0, 10, 25, 50, and 100 mM, supplemented with 100 μg/mL streptomycin and 100 U/mL penicillin) was added to each well for a 24 h incubation period. After another round of PBS washes, the model group received 100 μL of the treatment medium containing 0.6 mM H2O2 according to the methods of Li et al. (18), while the blank group was treated with the same volume of the treatment medium without H2O2. Cell viability assessment was conducted using the CCK8 assay after 1 h of incubation.
2.10 Measurements of T-AOC, SOD, and CAT activity and MDA content
The cells were cultured and treated in 6-well plates. Cells cultured to 80% confluence were preincubated with the treatment medium, either with or without 25 mM taurine, for 24 h. Subsequently, the cells were exposed to 0.6 mM H2O2 for 1 h, harvested and analyzed following the operational guidelines of the biochemical kits for T-AOC (G0115W), SOD (G0101W), CAT (G0105W), and MDA (G0109W) provided by Suzhou Grandis Biotechnology Co., Ltd., China.
2.11 RT-qPCR
Cells were categorized into four groups based on the presence or absence of 25 mM taurine and 0.6 mM H2O2 as per the culture method outlined in section 2.10. Upon completion of cell culture, the cells were harvested using a cell scraper, followed by total RNA extraction using the TRIzol method. Subsequently, the mRNA expression levels of the Nrf2, HO-1, CAT, SOD, ZO-1, occludin, and claudin-1 genes were analyzed through quantitative real-time PCR. The porcine-specific primers utilized in this study were custom-designed (Table 2). The PCR cycles and relative expression assays adhered to the methodology outlined in our prior research study conducted by Yin et al. (19).
2.12 Statistical analysis
In animal experiments, a single-factor random design is utilized, with each pig serving as a designated statistical unit. Normally distributed data were subjected to single-factor ANOVA, along with pairwise group comparisons via the Duncan method. Polynomial contrast analysis is implemented to evaluate the linear and quadratic impacts of the additives. A nonparametric Kruskal–Wallis test was performed if the data deviated from a normal distribution, followed by a post hoc Dunn test for intergroup comparisons. The graphs were generated using GraphPad Prism 9.0.0 software. Statistical significance was determined at the p < 0.05 level. The results are presented as the means and standard errors of the means (SEMs).
3 Results
3.1 Growth performance
The impact of taurine on the growth performance of weanling piglets is detailed in Table 3. Compared with those in the control group, the inclusion of 0.2 and 0.3% taurine in the diet significantly enhanced the average final weight and ADG of the piglets while markedly reducing the F/G. The final weight and ADG of the piglets increased proportionally to the taurine concentration, while the F/G ratio decreased linearly. Moreover, supplementing the diets of weaned piglets with 0.1, 0.2%, or 0.3% taurine led to a significant reduction in diarrhea incidence, with both linear and quadratic effects.
3.2 Serum antioxidant parameters
The analysis presented in Table 4 demonstrates that supplementing the diets of weaned piglets with 0.1, 0.2, and 0.3% taurine resulted in an increase in the serum GSH levels in comparison to those in the control group. The inclusion of 0.2 and 0.3% taurine led to elevated serum CAT activity and reduced levels of serum NO, XOD, GSSG, and MDA in the weaned piglets. Moreover, the introduction of 0.3% taurine enhanced the activities of GSH-Px and SOD in piglet serum and increased the T-AOC and POD, superoxide anion scavenging capacity, and hydroxyl radical scavenging rate. The study also revealed a linear increase in CAT, GSH-Px, SOD, T-AOC, superoxide anion scavenging capacity, the hydroxyl radical scavenging rate, GSH, and POD with increasing taurine concentration. Conversely, there were linear decreases in the levels of NO, XOD, GSSG, and MDA.
3.3 Liver antioxidant parameters
The findings presented in Table 5 illustrate the impact of taurine on the antioxidant capacity of the liver of weanling piglets. Compared with those in the control group, dietary supplementation with 0.1, 0.2, and 0.3% taurine resulted in increased levels of liver GSS, Trx, POD, and mt-ND5; enhanced superoxide anion clearance capacity; and an elevated hydroxyl-free clearance rate while reducing the liver NO levels in the weaned piglets. Moreover, administering 0.2 and 0.3% taurine led to increased levels of liver complex I and mt-ND6 in weanling piglets. The present study also revealed a linear increase in GSS, Trx, superoxide anion scavenging capacity, the hydroxyl radical scavenging rate, POD, complex I, mt-ND5, and mt-ND6 with increasing taurine concentrations and a linear decrease in NO levels.
3.4 Serum immune parameters
Table 6 shows that the supplementation of 0.1, 0.2, and 0.3% taurine in the diet of weaned piglets led to a significant reduction in the serum IL-6 levels and a notable increase in the IgA and IgG levels compared to those in the control group. Moreover, the addition of 0.2 and 0.3% taurine significantly elevated the serum IL-10 level, whereas the inclusion of 0.3% taurine notably increased the serum IL-4 and IgM levels. Notably, the levels of IL-4, IL-10, IgA, IgG, and IgM exhibited both linear and quadratic growth patterns in correlation with increases in the taurine concentration, while the level of IL-6 decreased linearly.
3.5 Jejunal mucosal barrier proteins
Table 7 shows that, in comparison to those in the control group, supplementation of the diets of weaned piglets with 0.1, 0.2, and 0.3% taurine led to a notable increase in occludin levels in the jejunal mucosa. Moreover, the inclusion of 0.2 and 0.3% taurine markedly elevated the levels of claudin and ZO-1. Concurrently, occludin, claudin, and ZO-1 levels exhibited both linear and quadratic increases proportional to the increase in taurine concentration.
3.6 The concentration of taurine in IPEC-J2 cells
To determine the optimal treatment parameters for taurine. The cells were pretreated with various taurine concentrations for 24 h and subsequently either exposed or not exposed to 0.6 mM H2O2 for 1 h. Cell viability assessments revealed that the highest viability rate occurred in the 25 mM taurine treatment group (Figure 1). Consequently, we selected 25 mM as the optimal concentration for all subsequent experiments.
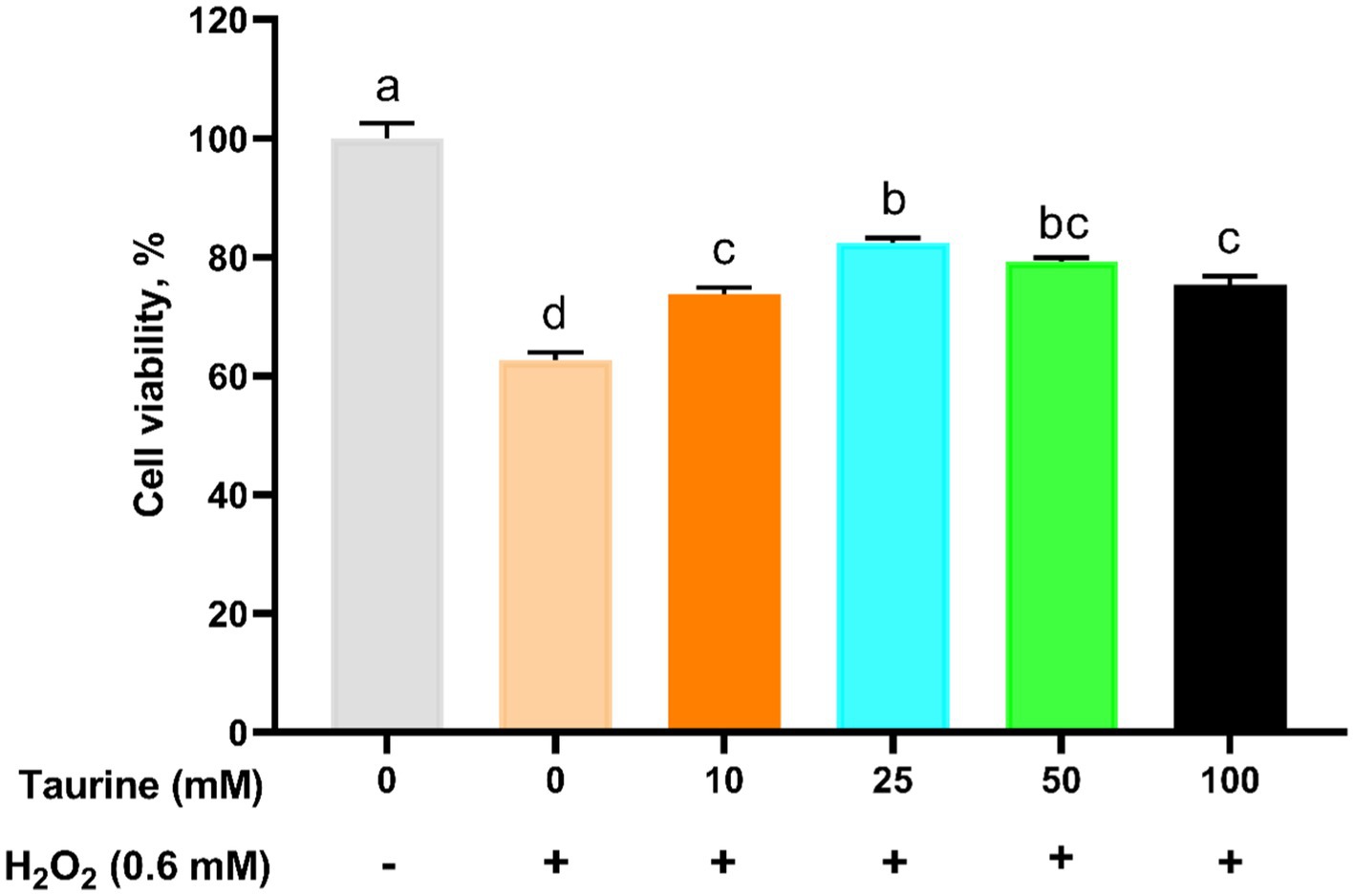
Figure 1. Effect of taurine on IPEC-J2 cell viability. IPEC-J2 cells were cultured in 96-well plates and preincubated with various concentrations of taurine (ranging from 0 to 100 mM) for 24 h, followed by exposure to 0.6 mM H2O2 for 1 h. The results, presented as the mean ± standard error of the mean (SEM), n = 6, indicate the values as a percentage relative to the untreated group with neither H2O2 nor taurine. Significant differences (p < 0.05) are denoted by the distinct letters a, b, c, and d. The following abbreviations were used: IPEC-J2, porcine intestinal epithelial cells; H2O2, hydrogen peroxide.
3.7 SOD activity, CAT activity, T-AOC and MDA content in H2O2-treated IPEC-J2 cells
This study focused on investigating the impact of taurine on antioxidant enzyme activity and MDA content in IPEC-J2 cells exposed to catalase. Biochemical kits (Figure 2) were utilized for analysis. Exposure to H2O2 significantly decreased the activities of CAT and SOD, as well as the T-AOC, while notably increasing the MDA content in the cells compared to those in the control group. As expected, 25 mM pretreatment for 24 h not only significantly boosted the antioxidant capacity of the cells but also ameliorated the oxidative stress injury caused by H2O2 exposure. This pretreatment led to a considerable increase in CAT and SOD activities and T-AOC, coupled with a reduction in MDA content.
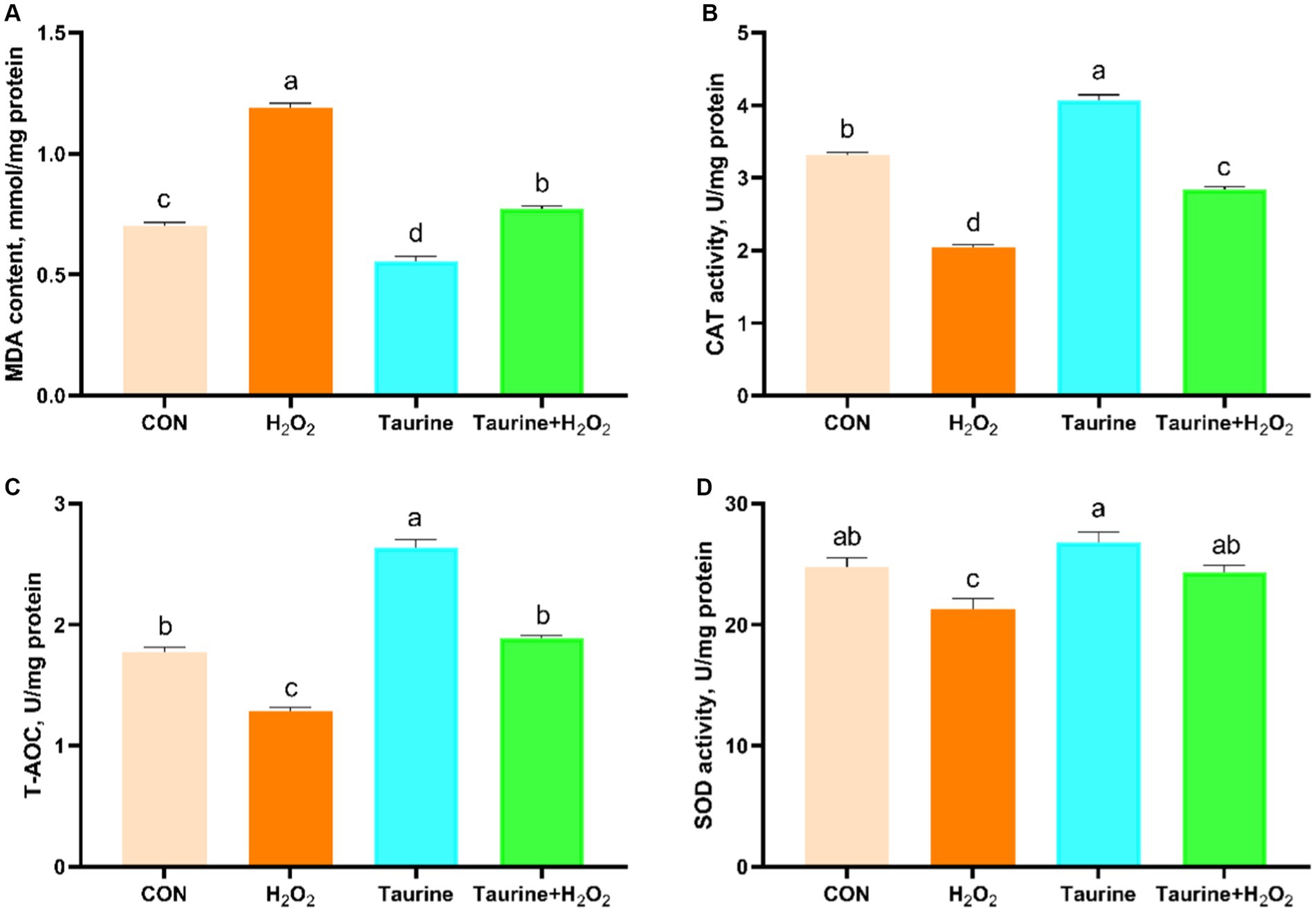
Figure 2. Effect of taurine on antioxidant enzymes and MDA in IPEC-J2 cells. (A) MDA content. (B) CAT activity. (C) Total antioxidant capacity. (D) SOD activity. IPEC-J2 cells were cultured in 6-well plates and pretreated with either 25 mM taurine or no taurine for 24 h, followed by exposure to 0.6 mM H2O2 or no H2O2 for 1 h. The results are presented as the mean ± standard error of the mean (SEM), n = 6. Statistical significance, denoted by p < 0.05, is indicated by values assigned unique alphabetical labels (a, b, c). IPEC-J2, porcine intestinal epithelial cells; H2O2, hydrogen peroxide; MDA, malondialdehyde; CAT, catalase; T-AOC, total antioxidant capacity; SOD, superoxide dismutase.
3.8 Effect of taurine on the expression of genes involved in the Nrf2 signaling pathway in IPEC-J2 cells
The Nrf2 signaling pathway serves as a crucial regulatory mechanism in combating oxidative stress. Our study explored the impact of pretreatment with taurine on the expression levels of Nrf2 pathway genes in H2O2-exposed IPEC-J2 cells (Figure 3). Compared to those in the control group, the expression of the Nrf2, HO-1 and CAT genes in the H2O2-treated group was notably lower. Conversely, pretreatment with 25 mM taurine for 24 h substantially upregulated the expression of the Nrf2, HO-1 and CAT genes, leading to a significant amelioration of oxidative damage caused by H2O2 exposure.
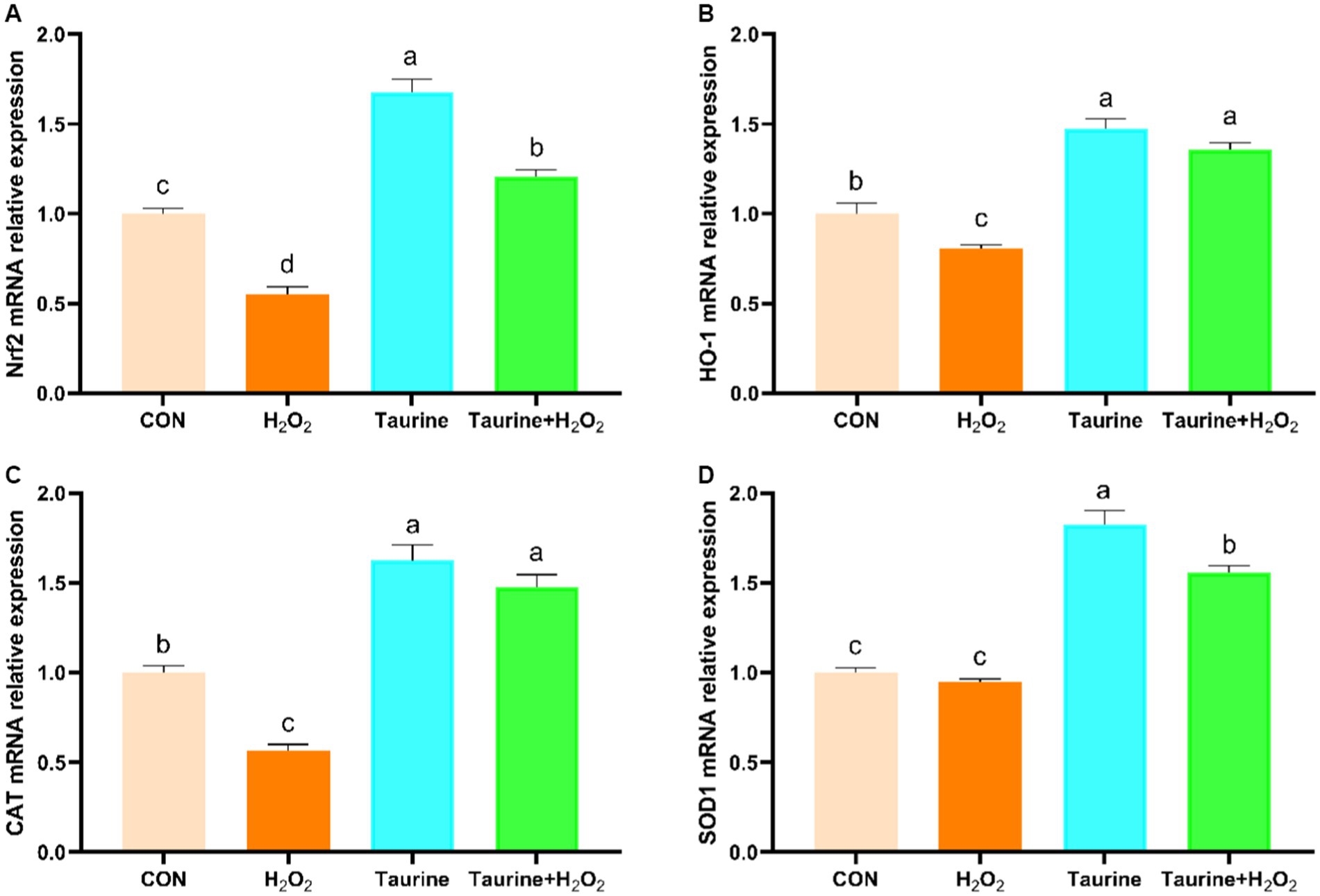
Figure 3. Effect of taurine on the expression of genes involved in the Nrf2 signaling pathway in IPEC-J2 cells. (A) Nrf2 mRNA expression relative to that of β-actin. (B) HO-1 mRNA expression relative to that of β-actin. (C) CAT mRNA expression relative to that of β-actin. (D) SOD1 mRNA expression relative to that of β-actin. IPEC-J2 cells were cultured in 6-well plates and pretreated with either 25 mM taurine or no taurine for 24 h, followed by exposure to 0.6 mM H2O2 or no H2O2 for 1 h. The results are presented as the mean ± standard error of the mean (SEM), n = 6. Statistical significance, denoted by p < 0.05, is indicated by values assigned unique alphabetical labels (a, b, c). IPEC-J2, porcine intestinal epithelial cells; H2O2, hydrogen peroxide; MDA, malondialdehyde; CAT, catalase; T-AOC, total antioxidant capacity; SOD, superoxide dismutase.
3.9 Effect of taurine on barrier function-related gene expression in IPEC-J2 cells exposed to H2O2
The impact of taurine pretreatment on the expression levels of tight junction-related genes in IPEC-J2 cells exposed to H2O2 is illustrated in Figure 4. Compared to those in the control group, H2O2 exposure markedly decreased the expression of the ZO-1, occludin, and claudin-1 genes. Conversely, pretreatment with 25 mM taurine for 24 h significantly upregulated the expression of the ZO-1, occludin, and claudin-1 genes, effectively reversing the downregulation induced by H2O2.
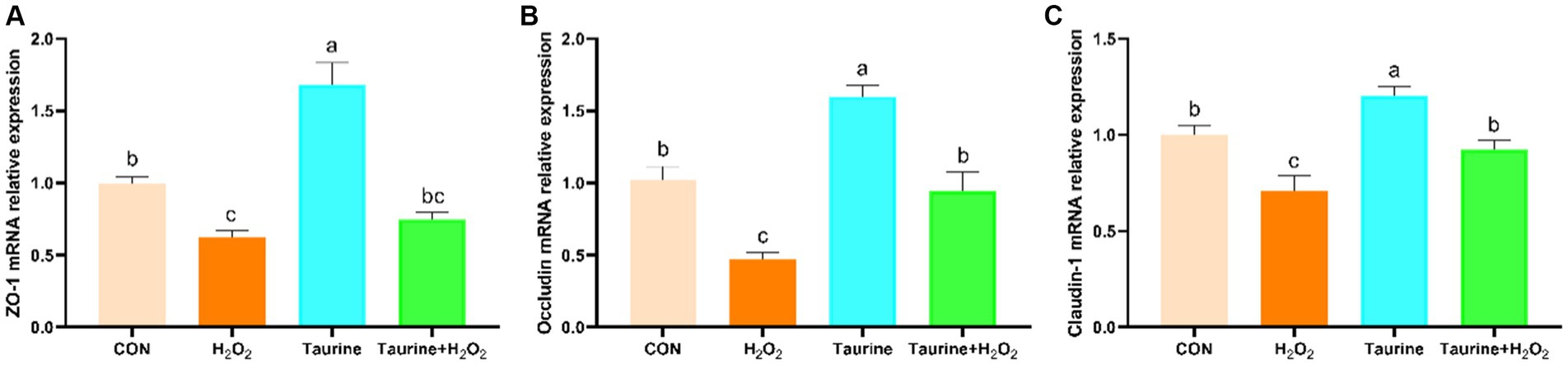
Figure 4. Effect of taurine on barrier function-related gene expression in IPEC-J2 cells. (A) ZO-1 mRNA expression relative to that of β-actin. (B) Occludin mRNA expression relative to that of β-actin. (C) Claudin-1 mRNA expression relative to that of β-actin. IPEC-J2 cells were cultured in 6-well plates and pretreated with either 25 mM taurine or no taurine for 24 h, followed by exposure to 0.6 mM H2O2 or no H2O2 for 1 h. The results are presented as the mean ± standard error of the mean (SEM), n = 6. Statistical significance, denoted by p < 0.05, is indicated by values assigned unique alphabetical labels (a, b, c). IPEC-J2, porcine intestinal epithelial cells; H2O2, hydrogen peroxide; ZO-1, zonula occludens 1.
4 Discussion
Weanling stress leads to diarrhea, reduced growth performance, compromised intestinal function, and other harmful effects, resulting in notable economic losses for the pig breeding industry (20, 21). This study aimed to investigate the potential benefits of taurine on the growth performance, oxidative stress, and intestinal barrier of weaned piglets. Currently, supplementing diets with antioxidants is an effective strategy for mitigating oxidative stress and enhancing the growth performance and health status of weaned piglets (7). Furthermore, past research has indicated that antioxidants can serve as alternatives to antibiotics in facilitating smooth weaning for piglets (22). Our findings revealed that the addition of taurine to the diets of weaned piglets, particularly at the 0.2 and 0.3% levels, significantly increased both the average final weight and daily gain of the piglets while reducing the feed-to-meat ratio. Consistent with the findings of a recent study by Wang et al. (23), taurine supplementation at 0.2 and 0.4% notably enhanced the growth performance of weaned piglets. Moreover, previous studies have suggested that taurine could mitigate the adverse impacts of LPS on the growth performance of piglets and broilers (16, 24). During the weaning process, piglets often experience diarrhea due to diverse external stressors and incomplete development. Each year, a considerable number of weaned piglets succumb to or are culled because of weaning stress-related diarrhea, resulting in significant losses in the breeding sector (3, 25). Our research showed that taurine effectively decreased the rate of diarrhea in weaned piglets compared to that in the control group. Subsequent studies revealed that taurine improved the oxidative stress levels and intestinal barrier function of piglets, further corroborating the potential of taurine as an antioxidant substitute for feed antibiotics to ameliorate the growth performance and diarrhea of weaned piglets.
Weanling stress is not only closely related to potential changes in the immune system and intestinal barrier function but also results in severe oxidative stress (26). The body’s redox reactions underlie various biochemical pathways and cellular functions, primarily reliant on the delicate equilibrium between the oxidative and antioxidant systems. An imbalance due to excessive ROS production or inadequate ROS scavenging by antioxidants induces oxidative stress, leading to cell apoptosis, tissue damage, metabolic disorders, inflammation, diarrhea, and reduced production performance in piglets (27, 28). Studies by Wen et al. (29) highlighted a notable increase in malondialdehyde (MDA) levels in the blood of weaned piglets, along with decreased SOD and GSH-Px activities. Taurine can scavenge reactive oxygen species and mitigate lipid peroxidation. The levels of SOD, GSH-Px, and MDA in blood and tissues are common biomarkers of oxidative stress. MDA is a metabolite of autolipid peroxidation, and an increase in GSH and SOD levels reflects an increase in host antioxidant capacity. Our research demonstrated that taurine supplementation enhances serum GSH-Px activity and GSH levels and improves superoxide anion and hydroxyl radical scavenging capacities while reducing NO, XOD, and GSSG serum levels. Moreover, oxidative stress is intricately linked to ferroptosis, where both GSH-Px and GSH play crucial roles as regulators by facilitating the reduction of ROS (30, 31). In conclusion, our findings align with previous research suggesting that taurine enhances the serum antioxidant capacity of weaned piglets.
The liver, an essential organ, performs various vital functions, including detoxification, metabolism, bile secretion, and immune defense (32). It exhibits high sensitivity to exogenous substances, rendering it susceptible to oxidative stress induced by drugs, viruses, or toxins. Weanling stress can trigger oxidative stress in the liver of piglets (33, 34). Previous research has demonstrated that weaning decreases the activities of SOD, CAT, and GSH-PX in the liver of piglets (35). Numerous studies support the protective role of taurine in liver injury. In our study, taurine increased the levels of GSS, Trx, POD, complex I, mt-nd5, and mt-nd6 in the livers of weaned piglets. It enhanced superoxide anion scavenging capacity and the hydroxyl free radical scavenging rate and notably reduced NO levels in the liver. Wu et al. (36) reported that taurine inhibits the increase in MDA and decrease in antioxidant enzyme activity in rat livers induced by aflatoxin B1. Shi et al. (37) reported the efficacy of taurine in alleviating liver oxidative damage from oxidized fish oil in young catfish. Our findings align with these studies, suggesting the potential of taurine to enhance the antioxidant capacity of the livers of weaned piglets.
Weanling stress commonly results in compromised immune function and an inflammatory response (38). During weaning, piglets are exposed to bacteria, toxins, and antigens from the intestinal cavity, which can infiltrate tissues, organs, and bloodstream through the submucosa, leading to disruptions in the intestinal immune system and triggering inflammation (39, 40). Excessive proinflammatory cytokines can exacerbate damage to intestinal integrity and epithelial function. Researchers studied the gene expression of inflammatory cytokines in weanling piglets and noted a significant increase in IL-6 levels in the intestinal tract, as did the levels of IL-1β and TNF-α (41, 42). Taurine exhibits anti-inflammatory properties and contributes significantly to managing cardiovascular diseases and metabolic inflammatory conditions such as diabetes mellitus and nonalcoholic fatty liver disease (15, 43, 44). Neutrophils are the primary contributors to tissue damage in mastitis. Studies have shown that taurine can mitigate neutrophil aggregation, hinder cxcl2 expression, and alleviate mastitis caused by Streptococcus uberis in dairy cows (45). Additionally, research has demonstrated that taurine supplementation can ameliorate liver cell swelling and inflammatory infiltration in piglets afflicted with mycotoxins, reducing the serum levels of the proinflammatory factors IL-1β, IL-6, IL-8, and TNF-α (46). Our study corroborates these findings, showing that incorporating taurine into the diets of weaned piglets increases the serum levels of IgA, IgG, IgM, IL-4, and IL-10 while reducing IL-6 levels. This finding suggested that taurine plays a crucial role in immune regulation, counteracting immune function impairment in weaned piglets due to weanling stress.
The jejunum serves as a vital organ for nutrient absorption from the external environment and plays a crucial role in communication with both the internal and external environments (47, 48). Due to its sensitivity to internal and external factors, the jejunum can experience oxidative stress, leading to abnormalities in its morphology and structure, damage to barrier functions, and compromised digestion and absorption capabilities (49). Consequently, oxidative damage to the jejunal mucosa is closely linked to the development of conditions such as growth retardation and diarrhea in livestock and poultry (12, 50). In this study, the supplementation of weanling piglets with taurine significantly elevated the levels of claudin, ZO-1, and occludin in the jejunal mucosa. Previous research by Shi et al. (37) demonstrated that incorporating taurine into a diet containing oxidized fish oil markedly upregulated intestinal ZO-1 and ZO-2 mRNA expression in catfish seedlings. Additionally, Zhao et al. (24) reported that taurine could increase the protein expression of ZO-1, occludin, and claudin-1 in the colons of piglets challenged with LPS. These findings align with those of the present study, reinforcing the notion that taurine has the potential to alleviate Weanling stress-induced impairment of intestinal barrier function.
The intestinal structure is primarily composed of villi and crypts, which are enveloped by a single layer of columnar epithelial cells (51, 52). These absorptive cells, constituting 90 to 95% of epithelial cells, feature apical microvilli and house various enzymes responsible for the breakdown and absorption of sugars and proteins (53–55). Furthermore, intestinal epithelial cells act as a crucial interface between immune cells and environmental agents, assisting in the detection and response to food, symbiotic bacteria, and pathogens to protect against pathogen invasion (56, 57). Thus, intestinal epithelial cells play a pivotal role in intestinal function. Oxidative stress has the potential to harm the intestinal mucosa. In this investigation, IPEC-J2 were utilized to replicate the oxidative damage observed in the intestinal mucosa due to weanling stress in piglets through exposure to H2O2. The presence of H2O2 triggers the generation of ROS and results in lipid damage, leading to the production of lipid degradation biomarkers (MDA). The present study revealed that pretreatment of IPEC-J2 cells with 25 mM taurine for 24 h significantly enhanced the activities of antioxidant enzymes and reduced the MDA content. Additionally, taurine pretreatment for 24 h substantially mitigated the oxidative damage induced by H2O2 exposure in IPEC-J2 cells, reversing the decreases in SOD and CAT activities and the increase in MDA content caused by H2O2 exposure. SOD is widely distributed in the mitochondrial matrix of animal cells and plays a crucial role in scavenging oxygen free radicals to protect intestinal mucosal cells from oxidative damage, making it a vital antioxidant in the body (58). Furthermore, taurine pretreatment for 24 h reversed the downregulation of genes encoding barrier proteins induced by H2O2, underscoring the role of taurine in ameliorating H2O2-induced mucosal injury.
The Nrf2 signaling pathway, which is crucial for the oxidative stress response, plays a vital role in maintaining the body’s redox homeostasis. Excessive ROS production can impede Nrf2 expression, leading to a redox imbalance (59, 60). Our study investigated the expression of Nrf2 pathway-related genes in IPEC-J2 cells using qPCR. We observed that taurine significantly enhanced Nrf2 and HO-1 gene expression, reversing the downregulation induced by H2O2 exposure. HO-1, a target of Nrf2, directly regulates HO-1 promoter activity through Nrf2, exhibiting potent antioxidant effects by scavenging ROS and defending against harmful substances such as peroxides and free radicals (61, 62). Taurine pretreatment also upregulated SOD and CAT gene expression in IPEC-J2 cells. Consistent with previous research, taurine shows promise for alleviating oxidative stress. Researchers have demonstrated that taurine, through Nrf2 activation, protects pig mammary gland epithelial cells from oxidative stress induced by H2O2 (63). Furthermore, studies by Wang et al. (64) revealed that taurine could mitigate the downregulation of Nrf2 and HO-1 induced by 5-fluorouracil, ameliorating mucosal inflammation in mouse jejunum and colon tissues. Our findings support the potential of taurine as a dietary supplement for combating oxidative stress.
5 Conclusion
This study demonstrated that taurine enhances the growth performance of weaned piglets, reduces the incidence of diarrhea, alleviates oxidative stress, and mitigates inflammatory responses associated with weaning. Taurine supplementation can upregulate the expression of genes and proteins related to tight junctions in the jejunal intestinal epithelium. Additionally, taurine has the potential to ameliorate oxidative damage in intestinal epithelial cells through the Nrf2/HO-1 pathway. In conclusion, taurine has emerged as a promising dietary supplement for mitigating oxidative damage in weanling piglets.
Data availability statement
The raw data supporting the conclusions of this article will be made available by the authors, without undue reservation.
Ethics statement
The animal studies were approved by Hunan Agricultural University Institutional Animal Care and Use Committee (202105). The studies were conducted in accordance with the local legislation and institutional requirements. Written informed consent was obtained from the owners for the participation of their animals in this study.
Author contributions
MZ: Data curation, Formal analysis, Investigation, Methodology, Software, Writing – original draft, Writing – review & editing. ZW: Formal analysis, Investigation, Methodology, Software, Writing – original draft, Writing – review & editing. DD: Formal analysis, Investigation, Software, Writing – original draft, Writing – review & editing. BW: Investigation, Software, Writing – original draft, Writing – review & editing. XZ: Investigation, Software, Writing – original draft, Writing – review & editing. BZ: Investigation, Software, Writing – original draft, Writing – review & editing. CW: Investigation, Software, Writing – original draft, Writing – review & editing. YZ: Conceptualization, Investigation, Project administration, Resources, Writing – original draft, Writing – review & editing.
Funding
The author(s) declare that no financial support was received for the research, authorship, and/or publication of this article.
Conflict of interest
The authors declare that the research was conducted in the absence of any commercial or financial relationships that could be construed as a potential conflict of interest.
Publisher’s note
All claims expressed in this article are solely those of the authors and do not necessarily represent those of their affiliated organizations, or those of the publisher, the editors and the reviewers. Any product that may be evaluated in this article, or claim that may be made by its manufacturer, is not guaranteed or endorsed by the publisher.
References
1. Ko, H-L, López-Vergé, S, Chong, Q, Gasa, J, Manteca, X, and Llonch, P. Short communication: preweaning socialization and environmental enrichment affect short-term performance after regrouping in commercially reared pigs. Animal. (2021) 15:100115. doi: 10.1016/j.animal.2020.100115
2. Douglas, SL, Edwards, SA, and Kyriazakis, I. Management strategies to improve the performance of low birth weight pigs to weaning and their long-term consequences. J Anim Sci. (2014) 92:2280–8. doi: 10.2527/jas.2013-7388
3. Canibe, N, Højberg, O, Kongsted, H, Vodolazska, D, Lauridsen, C, Nielsen, TS, et al. Review on preventive measures to reduce post-weaning diarrhoea in piglets. Animals. (2022) 12:2585. doi: 10.3390/ani12192585
4. Li, M, Chen, L, Zhao, Y, Sun, H, and Zhao, L. Research on the mechanism of HRP relieving IPEC-J2 cells immunological stress based on transcriptome sequencing analysis. Front Nutr. (2022) 9:944390. doi: 10.3389/fnut.2022.944390
5. Wu, K, El Zowalaty, AE, Sayin, VI, and Papagiannakopoulos, T. The pleiotropic functions of reactive oxygen species in cancer. Nat Cancer. (2024) 5:384–99. doi: 10.1038/s43018-024-00738-9
6. Rauf, A, Khalil, AA, Awadallah, S, Khan, SA, Abu-Izneid, T, Kamran, M, et al. Reactive oxygen species in biological systems: pathways, associated diseases, and potential inhibitors-a review. Food Sci Nutr. (2024) 12:675–93. doi: 10.1002/fsn3.3784
7. Qiao, L, Dou, X, Song, X, Chang, J, Yi, H, and Xu, C. Targeting mitochondria with antioxidant nutrients for the prevention and treatment of postweaning diarrhea in piglets. Anim Nutr. (2023) 15:275–87. doi: 10.1016/j.aninu.2023.09.002
8. Xu, X, Wei, Y, Hua, H, Jing, X, Zhu, H, Xiao, K, et al. Polyphenols sourced from Ilex latifolia Thunb. relieve intestinal injury via modulating ferroptosis in weanling piglets under oxidative stress. Antioxidants. (2022) 11:966. doi: 10.3390/antiox11050966
9. Yu, Z, Zhao, L, Zhao, J-L, Xu, W, Guo, Z, Zhang, A-Z, et al. Dietary Taraxacum mongolicum polysaccharide ameliorates the growth, immune response, and antioxidant status in association with NF-κB, Nrf2 and TOR in Jian carp (Cyprinus carpio var. Jian). Aquaculture. (2022) 547:737522. doi: 10.1016/j.aquaculture.2021.737522
10. Niu, X-T, Sun, C, Zhao, L, Chen, X-M, Wang, G-Q, and Li, M-Y. The major role of glucocorticoid receptor (GR) in astaxanthin alleviates immune stress in Channa argus lymphocyte. Aquaculture. (2024) 584:740637. doi: 10.1016/j.aquaculture.2024.740637
11. Nishida, Y, Nawaz, A, Hecht, K, and Tobe, K. Astaxanthin as a novel mitochondrial regulator: a new aspect of carotenoids, beyond antioxidants. Nutrients. (2021) 14:107. doi: 10.3390/nu14010107
12. Wang, L, Wang, C, Peng, Y, Zhang, Y, Liu, Y, Liu, Y, et al. Research progress on anti-stress nutrition strategies in swine. Anim Nutr. (2023) 13:342–60. doi: 10.1016/j.aninu.2023.03.006
13. Surai, PF, Earle-Payne, K, and Kidd, MT. Taurine as a natural antioxidant: from direct antioxidant effects to protective action in various toxicological models. Antioxidants. (2021) 10:1876. doi: 10.3390/antiox10121876
14. García-Ayuso, D, Di Pierdomenico, J, Martínez-Vacas, A, Vidal-Sanz, M, Picaud, S, and Villegas-Pérez, MP. Taurine: a promising nutraceutic in the prevention of retinal degeneration. Neural Regen Res. (2024) 19:606–10. doi: 10.4103/1673-5374.380820
15. Bae, M, Ahmed, K, and Yim, J-E. Beneficial effects of taurine on metabolic parameters in animals and humans. J Obes Metab Syndr. (2022) 31:134–46. doi: 10.7570/jomes21088
16. Han, H, Zhang, J, Chen, Y, Shen, M, Yan, E, Wei, C, et al. Dietary taurine supplementation attenuates lipopolysaccharide-induced inflammatory responses and oxidative stress of broiler chickens at an early age. J Anim Sci. (2020) 98:98. doi: 10.1093/jas/skaa311
17. Wu, S, Li, J, Deng, Y, Fang, P, Lei, W, Luo, A, et al. Effects of apple polyphenols and taurine on growth performance, tissue morphology, and lipid and glucose metabolism in rice field eel (Monopterus albus) fed high oxidized fish oil. Aquac Nutr. (2023) 2023:4912141. doi: 10.1155/2023/4912141
18. Li, Y, Jiang, X, Cai, L, Zhang, Y, Ding, H, Yin, J, et al. Effects of daidzein on antioxidant capacity in weaned pigs and IPEC-J2 cells. Anim Nutr. (2022) 11:48–59. doi: 10.1016/j.aninu.2022.06.014
19. Yin, J, Li, Y, Tian, Y, Zhou, F, Ma, J, Xia, S, et al. Obese Ningxiang pig-derived microbiota rewires carnitine metabolism to promote muscle fatty acid deposition in lean DLY pigs. Innovation. (2023) 4:100486. doi: 10.1016/j.xinn.2023.100486
20. Li, Y, Shi, P, Yao, K, Lin, Q, Wang, M, Hou, Z, et al. Diarrhea induced by insufficient fat absorption in weaned piglets: causes and nutrition regulation. Anim Nutr. (2024) 16:299–305. doi: 10.1016/j.aninu.2023.12.004
21. Zhao, X, Pang, J, Zhang, W, Peng, X, Yang, Z, Bai, G, et al. Tryptophan metabolism and piglet diarrhea: where we stand and the challenges ahead. Anim Nutr. (2024) 17:123–33. doi: 10.1016/j.aninu.2024.03.005
22. Li, Q, Zheng, T, Ding, H, Chen, J, Li, B, Zhang, Q, et al. Exploring the benefits of probiotics in gut inflammation and diarrhea-from an antioxidant perspective. Antioxidants. (2023) 12:1342. doi: 10.3390/antiox12071342
23. Wang, L, Jiang, L, Chu, Y, Feng, F, Tang, W, Chen, C, et al. Dietary taurine improves growth performance and intestine health via the GSH/GSSG antioxidant system and Nrf2/ARE signaling pathway in weaned piglets. Antioxidants. (2023) 12:1852. doi: 10.3390/antiox12101852
24. Zhao, D-D, Gai, Y-D, Li, C, Fu, Z-Z, Yin, D-Q, Xie, M, et al. Dietary taurine effect on intestinal barrier function, colonic microbiota and metabolites in weanling piglets induced by LPS. Front Microbiol. (2023) 14:1259133. doi: 10.3389/fmicb.2023.1259133
25. Yin, Z, Wang, K, Liu, Y, Li, Y, He, F, Yin, J, et al. Lactobacillus johnsonii improves intestinal barrier function and reduces post-weaning diarrhea in piglets: involvement of the endocannabinoid system. Animals. (2024) 14:493. doi: 10.3390/ani14030493
26. Correa, F, Luise, D, Palladino, G, Scicchitano, D, Brigidi, P, Martelli, PL, et al. Influence of body lesion severity on oxidative status and gut microbiota of weaned pigs. Animal. (2023) 17:100818. doi: 10.1016/j.animal.2023.100818
27. Yu, J, Zheng, C, Zheng, J, Duan, G, Guo, Q, Zhang, P, et al. Development of intestinal injury and restoration of weaned piglets under chronic immune stress. Antioxidants. (2022) 11:2215. doi: 10.3390/antiox11112215
28. Wang, M, Huang, H, Wang, L, Yin, L, Yang, H, Chen, C, et al. Tannic acid attenuates intestinal oxidative damage by improving antioxidant capacity and intestinal barrier in weaned piglets and IPEC-J2 cells. Front Nutr. (2022) 9:1012207. doi: 10.3389/fnut.2022.1012207
29. Wen, C, Li, F, Guo, Q, Zhang, L, Duan, Y, Wang, W, et al. Protective effects of taurine against muscle damage induced by diquat in 35 days weaned piglets. J Anim Sci Biotechnol. (2020) 11:56. doi: 10.1186/s40104-020-00463-0
30. Li, M-Y, Shi, Y-C, Xu, W-X, Zhao, L, and Zhang, A-Z. Exploring Cr(VI)-induced blood-brain barrier injury and neurotoxicity in zebrafish and snakehead fish, and inhibiting toxic effects of astaxanthin. Environ Pollut. (2024) 355:124280. doi: 10.1016/j.envpol.2024.124280
31. Zhao, L, Duan, H, Liu, Y, Wang, Y, Li, M, and Li, M. Long-term exposure of zebrafish (Danio rerio) to Cr(VI): reproductive toxicity and neurotoxicity. Reg Stud Mar Sci. (2024) 74:103559. doi: 10.1016/j.rsma.2024.103559
32. Tan, Y, An, K, and Su, J. Review: mechanism of herbivores synergistically metabolizing toxic plants through liver and intestinal microbiota. Comp Biochem Physiol C. (2024) 281:109925. doi: 10.1016/j.cbpc.2024.109925
33. Yu, C, Wang, D, Shen, C, Luo, Z, Zhang, H, Zhang, J, et al. Remodeling of hepatic glucose metabolism in response to early weaning in piglets. Animals. (2024) 14:190. doi: 10.3390/ani14020190
34. Cai, L, Ming, D, Chen, W, Zhao, Y, Li, Y, Sun, W, et al. Silybin alleviated hepatic injury by regulating redox balance, inflammatory response, and mitochondrial function in weaned piglets under paraquat-induced oxidative stress. Antioxidants. (2024) 13:324. doi: 10.3390/antiox13030324
35. Yu, L, Li, H, Peng, Z, Ge, Y, Liu, J, Wang, T, et al. Early weaning affects liver antioxidant function in piglets. Animals. (2021) 11:2679. doi: 10.3390/ani11092679
36. Wu, G, San, J, Pang, H, Du, Y, Li, W, Zhou, X, et al. Taurine attenuates AFB1-induced liver injury by alleviating oxidative stress and regulating mitochondria-mediated apoptosis. Toxicon. (2022) 215:17–27. doi: 10.1016/j.toxicon.2022.06.003
37. Shi, Y, Hu, Y, Wang, Z, Zhou, J, Zhang, J, Zhong, H, et al. The protective effect of taurine on oxidized fish-oil-induced liver oxidative stress and intestinal barrier-function impairment in juvenile Ictalurus punctatus. Antioxidants. (2021) 10:1690. doi: 10.3390/antiox10111690
38. Liu, B, Jiang, X, Cai, L, Zhao, X, Dai, Z, Wu, G, et al. Putrescine mitigates intestinal atrophy through suppressing inflammatory response in weanling piglets. J Anim Sci Biotechnol. (2019) 10:69. doi: 10.1186/s40104-019-0379-9
39. Tang, X, Xiong, K, Fang, R, and Li, M. Weaning stress and intestinal health of piglets: a review. Front Immunol. (2022) 13:1042778. doi: 10.3389/fimmu.2022.1042778
40. Huting, AM, Middelkoop, A, Guan, X, and Molist, F. Using nutritional strategies to shape the gastro-intestinal tracts of suckling and weaned piglets. Animals. (2021) 11:402. doi: 10.3390/ani11020402
41. Tang, W, Wei, Y, Ni, Z, Hou, K, Luo, XM, and Wang, H. IgA-mediated control of host-microbial interaction during weaning reaction influences gut inflammation. Gut Microbes. (2024) 16:2323220. doi: 10.1080/19490976.2024.2323220
42. Yan, H, Xing, Q, Xiao, X, Yu, B, He, J, Mao, X, et al. Effect of Saccharomyces cerevisiae postbiotics and essential oil on growth performance and intestinal health of weanling pigs during K88 ETEC infection. J Anim Sci. (2024) 102:skae007. doi: 10.1093/jas/skae007
43. Qaradakhi, T, Gadanec, LK, McSweeney, KR, Abraham, JR, Apostolopoulos, V, and Zulli, A. The anti-inflammatory effect of taurine on cardiovascular disease. Nutrients. (2020) 12:2847. doi: 10.3390/nu12092847
44. Ouyang, G, Wang, N, Tong, J, Sun, W, Yang, J, and Wu, G. Alleviation of taurine on liver injury of type 2 diabetic rats by improving antioxidant and anti-inflammatory capacity. Heliyon. (2024) 10:e28400. doi: 10.1016/j.heliyon.2024.e28400
45. Li, M, Wang, Z, Fu, S, Sun, N, Li, W, Xu, Y, et al. Taurine reduction of injury from neutrophil infiltration ameliorates Streptococcus uberis-induced mastitis. Int Immunopharmacol. (2023) 124:111028. doi: 10.1016/j.intimp.2023.111028
46. Ji, X, Tang, Z, Zhang, F, Zhou, F, Wu, Y, and Wu, D. Dietary taurine supplementation counteracts deoxynivalenol-induced liver injury via alleviating oxidative stress, mitochondrial dysfunction, apoptosis, and inflammation in piglets. Ecotoxicol Environ Saf. (2023) 253:114705. doi: 10.1016/j.ecoenv.2023.114705
47. Rao, MC. Physiology of electrolyte transport in the gut: implications for disease. Compr Physiol. (2019) 9:947–1023. doi: 10.1002/cphy.c180011
48. Cheng, Y, Ding, S, Azad, MA, Song, B, and Kong, X. Comparison of the pig breeds in the small intestinal morphology and digestive functions at different ages. Metabolites. (2023) 13:132. doi: 10.3390/metabo13010132
49. Hu, W, Du, L, Shao, J, Qu, Y, Zhang, L, Zhang, D, et al. Molecular and metabolic responses to immune stress in the jejunum of broiler chickens: transcriptomic and metabolomic analysis. Poult Sci. (2024) 103:103621. doi: 10.1016/j.psj.2024.103621
50. Jin, S, Xu, H, Yang, C, and Karmin, O. Regulation of oxidative stress in the intestine of piglets after enterotoxigenic Escherichia coli (ETEC) infection. Biochim Biophys Acta. (2024) 1871:119711. doi: 10.1016/j.bbamcr.2024.119711
51. Lema, I, Araújo, JR, Rolhion, N, and Demignot, S. Jejunum: the understudied meeting place of dietary lipids and the microbiota. Biochimie. (2020) 178:124–36. doi: 10.1016/j.biochi.2020.09.007
52. Steinbach, E, Masi, D, Ribeiro, A, Serradas, P, Le Roy, T, and Clément, K. Upper small intestine microbiome in obesity and related metabolic disorders: a new field of investigation. Metabolism. (2024) 150:155712. doi: 10.1016/j.metabol.2023.155712
53. Cui, C, Wang, X, Li, L, Wei, H, and Peng, J. Multifaceted involvements of Paneth cells in various diseases within intestine and systemically. Front Immunol. (2023) 14:1115552. doi: 10.3389/fimmu.2023.1115552
54. Atanga, R, Singh, V, and In, JG. Intestinal enteroendocrine cells: present and future druggable targets. Int J Mol Sci. (2023) 24:8836. doi: 10.3390/ijms24108836
55. Wiarda, JE, Becker, SR, Sivasankaran, SK, and Loving, CL. Regional epithelial cell diversity in the small intestine of pigs. J Anim Sci. (2023) 101:101. doi: 10.1093/jas/skac318
56. Wallaeys, C, Garcia-Gonzalez, N, and Libert, C. Paneth cells as the cornerstones of intestinal and organismal health: a primer. EMBO Mol Med. (2023) 15:e16427. doi: 10.15252/emmm.202216427
57. Seo, K, Seo, J, Yeun, J, Choi, H, Kim, Y-I, and Chang, S-Y. The role of mucosal barriers in human gut health. Arch Pharm Res. (2021) 44:325–41. doi: 10.1007/s12272-021-01327-5
58. Chen, Y, Li, B, Li, K, and Lin, Y. Superoxide dismutase nanozymes: current status and future perspectives on brain disease treatment and diagnosis. Chem Commun. (2024) 60:4140–7. doi: 10.1039/d3cc06288k
59. Morgenstern, C, Lastres-Becker, I, Demirdöğen, BC, Costa, VM, Daiber, A, Foresti, R, et al. Biomarkers of NRF2 signalling: current status and future challenges. Redox Biol. (2024) 72:103134. doi: 10.1016/j.redox.2024.103134
60. Egbujor, MC, Olaniyan, OT, Emeruwa, CN, Saha, S, Saso, L, and Tucci, P. An insight into role of amino acids as antioxidants via NRF2 activation. Amino Acids. (2024) 56:23. doi: 10.1007/s00726-024-03384-8
61. Yang, W, Huang, J, Xiao, B, Liu, Y, Zhu, Y, Wang, F, et al. Taurine protects mouse spermatocytes from ionizing radiation-induced damage through activation of Nrf2/HO-1 signaling. Cell Physiol Biochem. (2017) 44:1629–39. doi: 10.1159/000485762
62. Wang, H, Cheng, Q, Bao, L, Li, M, Chang, K, and Yi, X. Cytoprotective role of heme oxygenase-1 in cancer chemoresistance: focus on antioxidant, antiapoptotic, and pro-autophagy properties. Antioxidants (Basel). (2023) 12:1217. doi: 10.3390/antiox12061217
63. Xu, M, Che, L, Gao, K, Wang, L, Yang, X, Wen, X, et al. Taurine alleviates oxidative stress in porcine mammary epithelial cells by stimulating the Nrf2-MAPK signaling pathway. Food Sci Nutr. (2023) 11:1736–46. doi: 10.1002/fsn3.3203
Keywords: weanling piglets, taurine, oxidative stress, intestinal health, Nrf2/HO-1 signaling pathway
Citation: Zhou M, Wu Z, Deng D, Wang B, Zhou X, Zhou B, Wang C and Zeng Y (2024) Effects of taurine on the growth performance, diarrhea, oxidative stress and intestinal barrier function of weanling piglets. Front. Vet. Sci. 11:1436282. doi: 10.3389/fvets.2024.1436282
Edited by:
Moyosore Joseph Adegbeye, University of Africa, Bayelsa State, NigeriaReviewed by:
Lei Zhao, Heilongjiang Bayi Agricultural University, ChinaHongbin Si, Guangxi University, China
Copyright © 2024 Zhou, Wu, Deng, Wang, Zhou, Zhou, Wang and Zeng. This is an open-access article distributed under the terms of the Creative Commons Attribution License (CC BY). The use, distribution or reproduction in other forums is permitted, provided the original author(s) and the copyright owner(s) are credited and that the original publication in this journal is cited, in accordance with accepted academic practice. No use, distribution or reproduction is permitted which does not comply with these terms.
*Correspondence: Yan Zeng, zengyan@hnwyy.cn