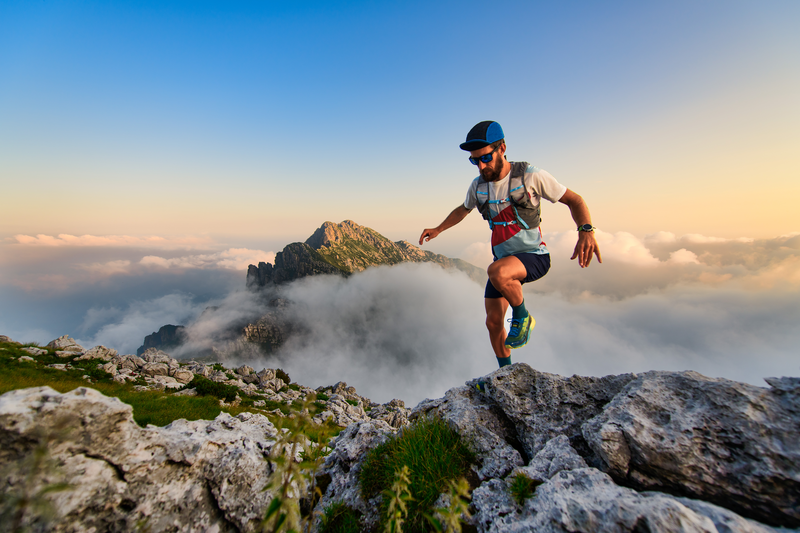
94% of researchers rate our articles as excellent or good
Learn more about the work of our research integrity team to safeguard the quality of each article we publish.
Find out more
ORIGINAL RESEARCH article
Front. Vet. Sci. , 29 August 2024
Sec. Veterinary Epidemiology and Economics
Volume 11 - 2024 | https://doi.org/10.3389/fvets.2024.1435111
Antimicrobial resistance has emerged as a significant health problem worldwide, including in Bangladesh, where chickens are an important protein source for human nutrition. One of the factors accelerating the development of antimicrobial resistance is the inappropriate use of antimicrobials on commercial chicken farms. A cross-sectional study was conducted in 2019 on 140 commercial chicken farms in the Chattogram district of Bangladesh to investigate the association between antimicrobial use and resistance in Escherichia coli and Salmonella spp. cultured from cloacal swabs of chickens and from the poultry shed environment. All E. coli and Salmonella spp. isolates were resistant to multiple antimicrobial classes, including those categorized as “Highest Priority Critically Important Antimicrobials” for human medicine. Notably, resistance was observed in E. coli isolates from farms that did not use these antimicrobial classes in the current production cycle. For example, although quinolones were not used on 43.9% of E. coli positive farms, 95.7% of these farms had quinolone-resistant E. coli isolates. The results of the path analysis revealed that there was a “direct effect” of the frequency of antimicrobial usage on “high” resistance, with resistance increasing when antimicrobials were administered more frequently (β = 0.28, p = 0.002). There was a “direct effect” of the purpose of antimicrobial use on “low” resistance, with resistance marginally decreasing when antimicrobials were administered solely for therapeutic use (β = −0.17, p = 0.062), but increasing when they were used prophylactically. Overall, the study results could be used to educate farmers on better practices for antimicrobial administration, and to guide government agencies to update policies on antimicrobial use and resistance surveillance in the poultry sector of Bangladesh.
Antimicrobial resistance in humans has been associated with the administration of antimicrobials in livestock (1). Antimicrobial resistant bacteria or their resistance genes can be transmitted to humans through direct contact with animals, consumption of animal products, or through environmental exposure (2).
In Bangladesh, two poultry production systems are established: backyard and commercial farming (3). Backyard farmers typically raise an average of seven birds (4), and commercial producers up to 20,000 birds (5). Commercial poultry farmers in Bangladesh predominately use antimicrobials to prevent and treat poultry diseases, but they are often misused (6). The lack of proper monitoring of antimicrobial usage and inadequate awareness regarding the impact of antimicrobial use on antimicrobial resistance emergence (7), the influence of feed and chick traders on husbandry practices (8), low biosecurity (9), financial constraints (10), and limited access to veterinary services (11) promote the misuse of antimicrobials, which may lead to the development of resistance (1). In Bangladesh, beta-lactams, tetracyclines, sulphonamides, fluoroquinolones, polymyxins, and aminoglycosides are frequently used in poultry to treat and prevent disease (12–14). For example, in Bangladeshi broiler farms, polymyxin (30.14%) was the most used antimicrobial, followed by sulphonamides (26%), tetracyclines (20.6%), quinolones (19.2%) (12). On layer farms, quinolones (1.66–22.5%), beta lactams (16.66%), tetracyclines (10.83%), and sulphonamides (3.33%) were commonly used antimicrobials (13). Resistance to these antimicrobials have been found in Escherichia coli and Salmonella spp. cultured from commercial chickens (12–14). However, these studies have either evaluated antimicrobial use or resistance, but not both together.
Under the “One Health” paradigm, the World Organization for Animal Health (WOAH), the Food and Agriculture Organization (FAO) and the World Health Organization (WHO) have provided recommendations to reduce the global use of antimicrobials and limit the risk of global spread of resistant bacteria (15). A database monitoring the type and quantities of antimicrobial agents used in animals was launched in October 2015 (15). The FAO has also launched an Action Plan to minimize the impact of antimicrobial resistance (16). This plan aims to develop the capacity for monitoring the use of antimicrobials in food animals (16), while WHO has developed a Global Action Plan to reduce the non-judicious use of antimicrobials in animals and humans (17).
The Bangladesh government formulated a National Action Plan (2017–2022) to tackle antimicrobial resistance in humans, livestock and fisheries and strengthen infectious disease prevention and control (18). However, for implementation, reliable data on antimicrobial use and resistance in the livestock sector is required. Our previous research assessed antimicrobial usage on commercial chicken farms, identifying that “Medically Important Antimicrobials” were frequently purchased from feed and chick traders, and often used in the absence of clinical signs and without adherence to withholding periods (6).
Escherichia coli is a commensal bacterium in food producing animals and is commonly used to monitor the development of antimicrobial resistance and the risk of antimicrobial resistant bacteria transmission from animals to human (19). Colibacillosis, caused by avian pathogenic E. coli (APEC), is among the most prevalent bacterial infections in chickens and other poultry in Bangladesh (20). Chickens are continually exposed to E. coli through feces, water, dust, and their environment (20). Since E. coli are always present in the gastrointestinal tract of birds, they easily spread via feces (20). In a study conducted on a Bangladeshi chicken farm, 36 of 99 samples, comprising internal organs, feces, and air from inside poultry sheds, tested positive for the APEC-associated virulence genes fimC, iucD, and papC (21). Salmonella is a zoonotic foodborne bacterium and one of the major causes of human food borne disease (22). Both bacteria, which are often cultured from healthy animals at slaughter, are used worldwide as bioindicators for antimicrobial susceptibility.
In this study, we aimed to quantify the association between antimicrobial usage and susceptibility of E. coli and Salmonella spp. cultured from samples collected on commercial chicken farms in Bangladesh. In addition, we aimed to identify farm management factors associated with the increased antimicrobial resistance.
A cross-sectional study was conducted from February to May 2019 on 140 commercial layer and broiler farms from eight upazilas (subdistricts) in the Chattogram district of Bangladesh (Figure 1).
Figure 1. Commercial layer and broiler farms in the Chattogram district of Bangladesh. The eight upazilas sampled are highlighted in purple. Pie charts display the number of farms by farm type (broiler farm, layer farm), with the size of the pie charts representing the number of farms sampled per upazila.
Data collection included sampling chickens and their environment and the administration of a structured questionnaire to obtain data on antimicrobial usage, and farm management practices implemented on these farms, as described previously (6). Briefly, commercial chicken farms were chosen from a sampling frame of 1748 commercial chicken farms using simple random sampling by applying the syntax RANDBETWEEN in Microsoft Excel (Microsoft Corporation, 2010). The number of farms per upazila was maintained using probability proportional to size sampling.
On each farm, the following strategy was followed to select the chicken shed of interest:
i. If the same number of antimicrobials was used in all sheds, the shed with the oldest chickens was selected.
ii. If the number of antimicrobials used across sheds differed, then the shed with the highest number of antimicrobials used was selected.
The structured questionnaire was validated by piloting it on five layer and five broiler farms. Additionally, photographs of antimicrobial packages were taken to cross-check the information provided. If available, photographs of the drug registration book kept on the farms were also taken to verify the data further.
Both cloacal and environmental swab samples were collected from the selected shed using the following strategy:
i. Cloacal swab samples were collected from five randomly selected healthy chickens and pooled into a 15 mL sterile falcon tube.
ii. Environmental swab samples were collected from the four corners and the middle of the chicken shed and pooled into a falcon tube containing buffered peptone water (BPW) (Neogen Corporation, 620 Lesher Place, Lansing MI 48912, United States).
Samples were kept in an insulated box containing ice packs and transported to the Poultry Research and Training Center (PRTC) laboratory in Chattogram, Bangladesh within 4–6 h. The samples were stored at −20°C until analysis. Bacterial culture and antimicrobial susceptibility testing were conducted between June and September 2019.
Only farmers actively engaged in the management of poultry farming were interviewed. One farmer from each farm was interviewed. Verbal consent was obtained from the farmers for the interview and collection of cloacal and environmental samples. Interviews were conducted by trained researchers from the Chattogram Veterinary and Animal Sciences University (CVASU).
Salmonella and E. coli were isolated by standard microbiological methods according to ISO 6579-1: 2017 and ISO 7251: 2005, respectively (Neogen, Lansing MI) (23).
All positive isolates were stored at −80°C in brain heart infusion broth with 50% glycerol (Neogen Corporation) (24). Identification was confirmed by Vitek (VITEK IVP, Inc., United States) at the Bangladesh Livestock Research Institute (BLRI). Details of the bacterial culture are summarized in the Supplementary material.
All E. coli and Salmonella spp. underwent Kirby-Bauer disk diffusion antimicrobial susceptibility testing against 12 antimicrobials following the Clinical and Laboratory Standard Institute (CLSI) guidelines (25). Details of antimicrobial susceptibility testing are summarized in the Supplementary material.
These antimicrobials were selected because they were identified as being commonly used on chicken farms in Bangladesh (12, 26). As resistance for one antimicrobial in a class often selects for resistance for other antimicrobials in that class (27), we categorized the antimicrobials into classes for data analysis. If any antimicrobial in the class was resistant, the bacteria was classified as resistant to that antimicrobial class.
A farm was classified as positive for E. coli or Salmonella spp. if either a cloacal or environmental sample tested positive for the bacteria.
E. coli and Salmonella spp. isolates identified as “resistant” or “intermediate” in the susceptibility testing were categorized as “resistant” for further data analysis. When a farm tested positive for E. coli or Salmonella spp., the cloacal or environmental sample with resistance to the largest number of antimicrobial classes determined the farms’ level of resistance. The association between usage and non-usage of antimicrobial classes and, resistance and susceptibility to these antimicrobial classes was explored using scatter plots and the Fisher’s exact test (28).
Multiclass resistance was defined as “resistance to antimicrobials of three or more different classes” (29).
Path analysis was used to examine potential relationships either in a “direct” or “indirect” way among a set of observed variables (30). A “direct” relationship exists when an exogenous variable (in regression, known as an independent variable) directly affects an endogenous variable (known as an outcome variable), while an “indirect” relationship occurs when an exogenous variable affects an endogenous variable through a mediator variable (30). The “indirect” relationship can involve one or more mediator variables between the exogenous variable and the endogenous variable (31). Path coefficients (β) are then estimated to quantify the relationships between variables along a hypothesized pathway (30).
We utilized path analysis to explore the relationship between published management factors related to antimicrobial usage that could directly or indirectly increase antimicrobial resistance in E. coli. Details on the management factors that could potentially increase antimicrobial usage in E. coli on commercial chicken farms are shown in Table 1. Path analysis was not conducted for Salmonella spp. because this bacterium was cultured from only a limited number of farms.
A diagram showing the hypothesized direct and indirect farm management pathways that could impact antimicrobial resistance on these farms is shown in Figure 2. For the path analysis, we classified resistance into two categories: “high” resistance to more than 5 antimicrobial classes (coded as “1”) and “low” resistance to up to 5 antimicrobial classes (coded as “0”).
Figure 2. Hypothesized pathway model of commercial chicken farm management practices that potentially could have an effect on increasing resistance to antimicrobial classes used on these farms.
Table 1. Management factors related to antimicrobial usage that potentially could have an effect on increasing resistance in E. coli on commercial chicken farms.
Variables with no significant “direct” and/or “indirect” effect at p < 0.05 on increasing the level of antimicrobial resistance were not included in the final path model. The fit of the path model was assessed using the Chi-square (χ)2 statistic, with a p-value >0.05 indicating a good fit (35), the Root Mean Square Error of Approximations (RMSEA), with a value <0.05 indicating a good fit and a value up to 0.08 indicating an acceptable fit (36), the Comparative Fit Index (CFI), with a value >0.95 indicating very good fit and ≥ 0.90 an acceptable fit (35), and the Standard Root Mean Square Residuals (SRMR) with a value ≤0.05 indicating a close-fitting model and a value between 0.05 up to 0.10 an acceptable fit (36).
Descriptive analysis was conducted using STATA 16 (StataCorp®, 2019). The Stata 16.0 SEM Builder was used for the path analysis (STATA 16, Statacorp®, 2019). R 4.0.3 was used to create the scatterplot (“ggplot2,” R Core Team®, 2020). The map was created using ArcGIS version 10.8.1 (Redlands, CA: Environmental Systems Research Institute, Inc., 2011).
From the 137 commercial farms where antimicrobials were used during the current production cycle, E. coli were cultured from 107 (78.1%) farms, and Salmonella spp. from 11 (8.0%) farms. From 107 farms, 79 (73.8%) had E. coli cultured exclusively from cloacal swabs, 81 (75.7%) from environmental swabs, and 53 (49.5%) from both swabs. From 11 farms, 3 (27.3%) had Salmonella spp. cultured exclusively from cloacal swabs, 10 (90.9%) from environmental swabs, and 2 (18.2%) from both swabs.
On E. coli positive farms, the most used antimicrobial classes (either alone or in combination with other antimicrobials) in the current production cycle were quinolones (56.1%, 60/107), tetracyclines (50.5%, 54/107), and polymyxins (43.0%, 46/107). On Salmonella spp. positive farms, tetracyclines (63.6%, 7/11), polymyxins (63.6%, 7/11), and beta lactams (54.5%, 5/11) were most used. On both E. coli and Salmonella positive farms, polymyxins and quinolones were mostly used (55.6%, 5/9), followed by beta lactams (44.4%, 4/9), macrolides (33.3%, 3/9) and sulphonamides (33.3%, 3/9).
On an isolate level, all E. coli isolates collected from cloacal samples were resistant to beta lactams and tetracyclines, followed by macrolides (98.7%, 78/79), sulphonamides (97.5%, 77/79), quinolones (92.4%, 73/79), aminoglycosides (82.3%, 65/79) and polymyxins (3.8%, 3/79). All E. coli isolates collected from environmental samples were resistant to beta lactams and tetracyclines, followed by macrolides (98.8%, 80/81), sulphonamides (95.1%, 77/81), quinolones (86.4%, 70/81), aminoglycosides (74.0%, 60/81) and polymyxins (3.7%, 3/81) (Table 2). All E. coli isolates resistant to antimicrobials are presented in Supplementary Table S1.
Table 2. Results of antimicrobial susceptibility testing of E. coli and Salmonella spp. isolates cultured from cloacal and environmental samples collected on commercial chicken farms in the Chattogram district of Bangladesh.
All Salmonella spp. isolates collected from cloacal samples were resistant to beta lactams, tetracyclines, quinolones, and sulphonamides followed by aminoglycosides (33.3%, 1/3). All Salmonella spp. isolates collected from environmental samples were resistant to beta lactams and quinolones, followed by tetracyclines (90.0%, 9/10), aminoglycosides (80.0%, 8/10), sulphonamides (30.0%, 3/10) and polymyxins (20.0%, 2/10) (Table 2). All Salmonella spp. isolates resistant to antimicrobials are presented in Supplementary Table S1.
On a farm level, all E. coli positive farms (N = 107) were resistant to beta lactams, tetracyclines, macrolides and sulphonamides, followed by quinolones (95.3%, 102/107), and aminoglycosides (86.0%, 92/107). A relatively low resistance was found for polymyxins (5.6%, 6/107). All Salmonella positive farms (N = 11) were resistant to beta lactams and quinolones, followed by tetracyclines (90.9%, 10/11), aminoglycosides (81.8%, 9/11) and sulphonamides (54.5%, 6/11); while low resistance was identified for polymyxins (18.2%, 2/11) (Table 2).
Only 3.7% (4/107) of the E. coli positive farms were resistant to four antimicrobial classes, 15.9% (17/107) were resistant to five, 74.8% (80/107) to six and, 5.6% (6/107) were resistant to all seven antimicrobial classes. Alternatively, 18.2% (2/11) of the Salmonella spp. positive farms were resistant to three antimicrobial classes, 27.3% (3/11) were resistant to four, 36.4% (4/11) to five, and 18.2% (2/11) were resistant to six antimicrobial classes. All E. coli and Salmonella spp. isolated were multiclass resistant.
Supplementary Table S2 shows the frequencies of farms using antimicrobial classes on E. coli-positive and Salmonella spp.-positive commercial poultry farms, while the frequency of E. coli-positive and resistant farms where antimicrobials classes were not used in the current production cycle are presented in Supplementary Table S3. The relationship of usage and resistance pattern of quinolones, macrolides, polymyxins, aminoglycosides, beta lactams, sulphonamides, and tetracyclines is presented in Figure 3.
Figure 3. Usage and resistance pattern of antimicrobial classes on commercial chicken farms in the Chattogram district of Bangladesh where E. coli was cultured. Each data point represents a E. coli positive farm. The data points are jittered to avoid overlapping.
Our results highlight that E. coli-positive farms were frequently resistant to antimicrobial classes even when these classes were not reported to have been used during the studied production cycle. Indeed, quinolones, classified among the “Highest Priority Critically Important Antimicrobials” (HPCIAs) (37) were not reported being used on 43.9% (47/107) of the farms where E. coli was cultured, but 95.7% (45/47) of these non-user farms had quinolone-resistant E. coli isolates. Similarly, all isolates on E. coli-positive farms (100%, 74/74) were resistant to macrolides, beta lactams, sulphonamides and tetracyclines, despite the reported usage of these antimicrobial classes ranging between 29.0–50.5%. In contrast, polymyxins (colistin) were not used on 57.0% (61/107) of E. coli-positive farms, and only 8.2% (5/61) of these farms had polymyxin-resistant E. coli cultured. The frequency of polymyxin-resistance on farms that used polymyxins did not differ significantly from those farms that did not use polymyxins (p = 0.234). However, aminoglycosides were not used on 70.1% (75/107) of E. coli positive farms, but 81.3% (61/75) of these non-user farms had aminoglycosides-resistant E. coli isolates (p = 0.036).
Descriptive statistics of management procedures used on commercial chicken farms that were considered as predictors in the path analysis are summarized in Table 3.
Table 3. Descriptive statistics of the management factors related to antimicrobial usage which potentially have an effect on increasing resistance in E. coli on commercial chicken farms.
Out of the 107 E. coli positive farms, 72.3% (60/83) of farmers rearing small flocks (≤2,500 birds) used antimicrobials three times per day, compared to 45.8% (11/24) of farmers with larger flocks (>2,500 birds) (p = 0.003). The proportion of farmers using antimicrobials therapeutically only was higher among those rearing large flocks (29.2%, 7/24) than those rearing small flocks (9.6%, 8/83) (p = 0.023).
The results of the path analysis (Figure 4) indicated that there was a “direct effect” of frequency of antimicrobial usage on higher resistance, with resistance increasing with more frequent administration of antimicrobials (β = 0.28, p = 0.002). Conversely, there was a “direct effect” of purpose of antimicrobial usage on lower resistance, with resistance marginally declining when antimicrobials were only administered therapeutically (β = −0.17, p = 0.062; or increasing when antimicrobials were administered prophylactically). There was an “indirect effect” of flock size on resistance, which decreased in larger flocks (β = −0.12, p = 0.005). Having larger flock sizes was associated with antimicrobial usage for therapeutic purposes only (β = 0.23, p = 0.009), while in smaller flocks antimicrobial usage for prophylactic purposes was more common (i.e. less antimicrobial usage for therapeutic purposes only β = −0.32, p < 0.001).
Figure 4. Final path analysis results for farm management factors that had an effect on increasing resistance on commercial chicken farms where E. coli was cultured.
The other management factors were not significant at p < 0.05 in the final model. The model fitted the data well, as indicated by χ2 = 2.347 (p = 0.309), RMSEA = 0.040, CFI = 0.987 and SRMR = 0.042.
The present study provides unique insights into the relationship between antimicrobial usage and resistance in E. coli and Salmonella spp. isolates cultured from commercial chicken farms in Bangladesh. It should be noted that isolates were cultured from cloacal swabs of apparently healthy birds and from their environment.
Commensal E. coli is present in chickens’ intestinal tracts, with a 100% recovery rate expected according to FAO guidelines (38). However, studies have reported differing recovery rates of E. coli from cloacal swabs from poultry, such as in Bangladesh (100–88%), Timor-Leste (85.5%), China (53.4%), Qatar (52.0%) and Pakistan (51.3%) (37, 39–43). In our study, we achieved a recovery rate of 73.8%. Varying recovery rates of E. coli between studies are likely influenced by transport and storage of samples, laboratory techniques used, and husbandry and management conditions on poultry farms (37).
In our study, Salmonella was detected in 27.3% of cloacal swabs. In other studies in Bangladesh, Salmonella spp. have been detected in 27–48% of cloacal swabs in poultry. Prevalence may differ due to differences in isolation and identification procedures, but also may be influenced by type of poultry, flock size and factors such as biosecurity, and hygiene (37, 40).
Some antimicrobials that were administered to chickens are classified as HPCIAs for human health, such as quinolones and polymyxins (33). Resistance to these antimicrobials was found on the studied farms. These antimicrobials are not recommended for use in animals without culture and antimicrobial susceptibility testing (34). Establishing a direct link between antimicrobial usage and resistance is challenging as multiple pathways exist and resistance might develop over many years. Nevertheless, this study identified that antimicrobial resistance patterns do not only depend on antimicrobial usage, as high levels of resistance were found on farms not reporting the use of these antimicrobials in the current production cycle. Furthermore, repeated administration of antimicrobials within a single day might have contributed to increased resistance. The impact of antimicrobial overuse on the development of antimicrobial resistance has been previously described (44) and selection pressure, cross-resistance and/or co-resistance might have contributed to the occurrence of antimicrobial resistance (32).
The presence of resistant bacteria in environmental samples is concerning as it represents a risk of contamination for areas surrounding poultry sheds. This contamination can occur through run off from poultry sheds, which may, in turn, contaminate water sources and the environment (45). Resistant bacteria can transfer resistance genes between different strains and across species (46). Such resistant commensal or pathogenic bacteria transfer can then result in resistant infections in humans, leading to treatment failure, higher medical costs, prolonged hospital stays, and increased mortality (47).
Antimicrobial agents may remain in the environment for years (48). Poor poultry husbandry practices, including inadequate disinfection, waste disposal into the environment, and poor infection control practices may contribute to the development of an environmental reservoir of resistant bacteria over time (49). The presence of resistant bacteria cultured from cloacal samples is also concerning because they may contaminate food products, resulting in foodborne illness (50).
Resistance was higher on small scale commercial chicken farms. This could be attributed to lower biosecurity standards and management practices, including inadequate housing facilities, or inadequate disinfection of water sources as reported by FAO (51). Our previous investigations into the biosecurity practices and antimicrobial usage on these farms revealed that inadequate biosecurity was more prevalent on farms with smaller flock sizes (<2,500 birds) compared to those with larger flocks (≥2,500 birds); and usage of antimicrobials was more common on small scale farms (6, 9). Small-scale farmers often lack formal training in biosecurity (13) and may be advised by representatives of pharmaceutical companies (8), and unqualified veterinary care givers who offer more affordable animal care (52). Although it is commonly reported that farmers are influenced by the advice of feed and chick traders (8), our study did not find evidence supporting this. Farmers rearing smaller flocks may more frequently administer antimicrobials prophylactically compared to farmers with larger flocks, to reduce the potential disease risk associated with poorer biosecurity.
Farms where antimicrobials were used prophylactically had higher levels of resistance in E. coli. Mass prophylactic administration of antimicrobials might result in lower adaptive immunity in chicken flocks (53), and their effectiveness in preventing poultry diseases may diminish over time (54), Thus, the misuse of antimicrobials in broiler (12) and layer (13) farms in Bangladesh may select for antimicrobial resistance in E. coli and potentially increase resistance.
We have used path analysis to investigate the factors contributing to high antimicrobial resistance. This approach enabled us to clarify complex interrelationships among variables, highlighting the most important pathways that were associated with the outcome (55). It allowed to display the direction and magnitude of both “direct” and “indirect” relationships between farm management factors and their impact on antimicrobial resistance in E. coli. However, although path analysis was used to analyze the hypothesized pathways, it is primarily based on correlations and cannot be used to prove causality (55).
The data collection in this study had several limitations. Firstly, while we could identify if an antimicrobial class was used during the current production cycle, we could not determine the frequency, dose or the duration of administration. Therefore, actual antimicrobial usage may have been underestimated. Secondly, some farmers might not have accurately remembered which antimicrobials they had administered. However, by focusing on antimicrobial use in the current production cycle, we aimed to minimize potential recall bias. Thirdly, antimicrobial susceptibility testing was performed using the disk diffusion method, which might not be optimal for detecting polymyxins (i.e. colistin in this study) resistance (25, 56). Colistin is a cationic, multicomponent, lipopeptide antimicrobial agent that diffuses slowly in antimicrobial susceptibility testing media, therefore the resulting zone of inhibitions tends to be inaccurate; and may bias the interpretation (57). Minimum inhibitory concentration (MIC) methods are preferred for colistin (25, 56), however, were not feasible to conduct in the laboratory in Bangladesh. In future studies, the use of MIC methods would be recommended for all antimicrobials, especially for colistin. However, MIC methods for colistin may also be affected by its cationic properties (58). Finally, the lack of veterinary breakpoints for most of the antimicrobials tested (25, 56) is a general limitation in veterinary studies, as breakpoints need to be extrapolated from humans or other species.
Overall, this study provides important baseline data that could be used to develop recommendations aimed at reducing antimicrobial usage in chicken production. Such recommendations may include initiatives for training farmers and raising awareness regarding the appropriate use of antimicrobials. Basic training in poultry pathology and necropsy would allow farmers to make informed decisions when selecting antimicrobials based on gross pathology. Routine availability of culture and antimicrobial susceptibility testing, and molecular techniques to identify and characterize commensal and pathogenic organisms, along with residue testing performed by governmental authorities, could also form integral components of an effective antimicrobial stewardship program for Bangladesh.
The raw data supporting the conclusions of this article will be made available by the authors, without undue reservation.
The studies involving humans were approved by University of Queensland Institutional Human Ethics Committee (Approval no. 2018002266). The studies were conducted in accordance with the local legislation and institutional requirements. Written informed consent for participation was not required from the participants or the participants’ legal guardians/next of kin because “Verbal” consent was obtained from the farmers for the interview and for the collection of cloacal and environmental samples. The animal study was approved by Chattogram Veterinary and Animal Sciences University [Approval no: CVASU/Dir(R&E) EC/2020/241(3)]. The study was conducted in accordance with the local legislation and institutional requirements.
MF: Data curation, Formal analysis, Investigation, Methodology, Writing – review & editing. TI: Conceptualization, Data curation, Formal analysis, Investigation, Methodology, Software, Writing – original draft. SD: Data curation, Formal analysis, Investigation, Methodology, Writing – review & editing. JG: Conceptualization, Supervision, Writing – review & editing. RM: Data curation, Writing – review & editing. SG: Formal analysis, Writing – review & editing. GF: Conceptualization, Funding acquisition, Resources, Supervision, Writing – review & editing. MH: Conceptualization, Funding acquisition, Resources, Supervision, Writing – review & editing. JH: Conceptualization, Formal analysis, Funding acquisition, Resources, Supervision, Writing – review & editing.
The author(s) declare that financial support was received for the research, authorship, and/or publication of this article. This field data collection was supported by the BALZAC research program ‘Behavioral adaptations in live poultry trading and farming systems and zoonoses control in Bangladesh’ (BB/L018993/1), a joint research initiative between the Biotechnology and Biological Sciences Research Council, the Defense Science and Technology Laboratory, the Department for International Development, the Economic and Social Sciences Research Council, the Medical Research Council and the Natural Environment Research Council. The corresponding author of this publication was supported by the Australia Awards Scholarship.
We are grateful to the farmers for participating in the study. Also, we thank the CVASU research team for helping with the data collection.
The authors declare that the research was conducted in the absence of any commercial or financial relationships that could be construed as a potential conflict of interest.
All claims expressed in this article are solely those of the authors and do not necessarily represent those of their affiliated organizations, or those of the publisher, the editors and the reviewers. Any product that may be evaluated in this article, or claim that may be made by its manufacturer, is not guaranteed or endorsed by the publisher.
The Supplementary material for this article can be found online at: https://www.frontiersin.org/articles/10.3389/fvets.2024.1435111/full#supplementary-material
1. Economou, V, and Gousia, P. Agriculture and food animals as a source of antimicrobial-resistant bacteria. Infect Drug Resist. (2015) 8:49–61. doi: 10.2147/IDR.S55778
2. Regecová, I, Výrostková, J, Zigo, F, Gregová, G, and Kováčová, M. Detection of antimicrobial resistance of bacteria Staphylococcus chromogenes isolated from sheep’s milk and cheese. Antibiotics. (2021) 10:570. doi: 10.3390/antibiotics10050570
3. Hamid, M, Rahman, M, Ahmed, S, and Hossain, K. Status of poultry industry in Bangladesh and the role of private sector for its development. Asian J Poult Sci. (2017) 11:1–13. doi: 10.3923/ajpsaj.2017.1.13
4. Chowdhury, S. Family poultry production in Bangladesh: is it meaningful or an aimless journey? Worlds Poult Sci J. (2013) 69:649–65. doi: 10.1017/S0043933913000652
5. Hamid, M. Livestock production systems and their distribution pattern in Bangladesh: a review. Cent J Bangladesh Open Univ. (2017) 4:1–11.
6. Imam, T, Gibson, J, Foysal, M, Das, SB, Gupta, SD, Fournié, G, et al. A cross-sectional study of antimicrobial usage on commercial broiler and layer chicken farms in Bangladesh. Front Vet Sci. (2020) 7:576113. doi: 10.3389/fvets.2020.576113
7. Hoque, R, Ahmed, SM, Naher, N, Islam, MA, Rousham, EK, Islam, BZ, et al. Tackling antimicrobial resistance in Bangladesh: a scoping review of policy and practice in human, animal and environment sectors. PLoS One. (2020) 15:e0227947. doi: 10.1371/journal.pone.0227947
8. Masud, AA, Rousham, EK, Islam, MA, Alam, M-U, Rahman, M, Mamun, AA, et al. Drivers of antibiotic use in poultry production in Bangladesh: dependencies and dynamics of a patron-client relationship. Frontiers in veterinary. Science. (2020) 7:78. doi: 10.3389/fvets.2020.00078
9. Imam, T, Gibson, JS, Gupta, SD, Hoque, MA, Fournié, G, and Henning, J. Association between farm biosecurity practices and antimicrobial usage on commercial chicken farms in Chattogram, Bangladesh. Prev Vet Med. (2021) 196:105500. doi: 10.1016/j.prevetmed.2021.105500
10. Okeke, IN, Laxminarayan, R, Bhutta, ZA, Duse, AG, Jenkins, P, O'Brien, TF, et al. Antimicrobial resistance in developing countries. Part I: recent trends and current status. Lancet Infect Dis. (2005) 5:481–93. doi: 10.1016/S1473-3099(05)70189-4
11. Hedman, HD, Vasco, KA, and Zhang, L. A review of antimicrobial resistance in poultry farming within low-resource settings. Animals. (2020) 10:1264. doi: 10.3390/ani10081264
12. Islam, KBMS, Shiraj-Um-Mahmuda, S, and Hazzaz-Bin-Kabir, M. Antibiotic usage patterns in selected broiler farms of Bangladesh and their public health implications. J Public Health Dev Ctries. (2016) 2:276–84.
13. Ferdous, J, Sachi, S, Zakaria Al Noman, S, Hussani, YAS, and Sikder, MH. Assessing farmers’ perspective on antibiotic usage and management practices in small-scale layer farms of Mymensingh district, Bangladesh. Veterinary. WORLD. (2019) 12:1441–7. doi: 10.14202/vetworld.2019.1441-1447
14. Shahjada, Z, Hussain, K, Islam, MM, Majumder, S, Hasan, I, Rahman, M, et al. Bacteria causing omphalitis in newly hatched chicks from broiler and layer flocks and their antibiotic profiles. Int J Nat Soc Sci. (2017) 4:73–81.
15. OIE. OIE Strategy Tackles the threat of antimicrobial resistance in animals. (2018). Available at: https://search-proquest-com.ezproxy.library.uq.edu.au/docview/1864046717?rfr_id=info%3Axri%2Fsid%3Aprimo (Accessed November 25, 2023).
16. FAO. The FAO action plan on antimicrobial resistance 2016–2020. (2016). Available at: http://www.fao.org/3/a-i5996e.pdf (Accessed November 25, 2023).
17. WHO. Global action plan on antimicrobial resistance. (2015). Available at: https://www.who.int/antimicrobial-resistance/global-action-plan/en/ [Accessed November 25, 2023].
18. Ministry of Health and Family Welfare, (2017). National action plan: antimicrobial resistance containment in Bangladesh 2017–2022. Available at: https://www.flemingfund.org/wp-content/uploads/d3379eafad36f597500cb07c21771ae3.pdf (Accessed November 25, 2023).
19. Ramos, S, Silva, V, Enes Dapkevicius, ML, Canica, M, Tejedor-Junco, MT, Igrejas, G, et al. Escherichia coli as commensal and pathogenic bacteria among food-producing animals: health implications of extended spectrum beta-lactamase (ESBL) production. Animals. (2020) 10:2239. doi: 10.3390/ani10122239
20. Islam, MS, Hossain, MJ, Sobur, MA, Punom, SA, Rahman, A, and Rahman, MT. A systematic review on the occurrence of antimicrobial-resistant Escherichia coli in poultry and poultry environments in Bangladesh between 2010 and 2021. Biomed Res Int. (2023) 2023:2425564. doi: 10.1155/2023/2425564
21. Ievy, S, Islam, MS, Sobur, MA, Talukder, M, Rahman, MB, Khan, MFR, et al. Molecular detection of avian pathogenic Escherichia coli (APEC) for the first time in layer farms in Bangladesh and their antibiotic resistance patterns. Microorganisms. (2020) 8:1021. doi: 10.3390/microorganisms8071021
22. Abebe, E, Gugsa, G, and Ahmed, M. Review on major food-borne zoonotic bacterial pathogens. J Trop Med. (2020) 2020:1–19. doi: 10.1155/2020/4674235
23. Quinn, PJ, Markey, BK, Leonard, FC, Hartigan, P, Fanning, S, and Fitzpatrick, ES. Veterinary microbiology and microbial disease. United Kingdom: Wiley-Blackwell (2011).
24. Kakkar, M, Chatterjee, P, Chauhan, AS, Grace, D, Lindahl, J, Beeche, A, et al. Antimicrobial resistance in South East Asia: time to ask the right questions. Glob Health Action. (2018) 11:1483637. doi: 10.1080/16549716.2018.1483637
25. CLSI. Performance standards for antimicrobial suceptibility testing. Wayne: Clinical and Laboratory Standards Institute (2020).
26. Hasan, B, Faruque, R, Drobni, M, Waldenström, J, Sadique, A, Ahmed, KU, et al. High prevalence of antibiotic resistance in pathogenic Escherichia coli from large- and small-scale poultry farms in Bangladesh. Avian Dis. (2011) 55:689–92. doi: 10.1637/9686-021411-Reg.1
27. Raymond, B. Five rules for resistance management in the antibiotic apocalypse, a road map for integrated microbial management. Evol Appl. (2019) 12:1079–91. doi: 10.1111/eva.12808
28. Fisher, RA. On the interpretation of χ 2 from contingency tables, and the calculation of P. J R Stat Soc. (1922) 85:87–94. doi: 10.2307/2340521
29. JIACRA-II. ECDC, EFSA and EMA Joint Scientific Opinion on a list of outcome indicators as regards surveillance of antimicrobial resistance and antimicrobial consumption in humans and food-producing animals, European Medicines Agency. (2017). Available at: https://www.ema.europa.eu/en/documents/report/ecdc-efsa-ema-joint-scientific-opinion-list-outcome-indicators-regards-surveillance-antimicrobial_en.pdf (Accessed November 25, 2023).
30. Valenzuela, S, and Bachmann, I. Path analysis. The international encyclopedia of communication research methods. New York: John Wiley and Sons, Inc (2017).
31. Greenland, MS, Pearl, MJ, and Robins, MJ. Causal diagrams for epidemiologic research. Epidemiology. (1999) 10:37–48. doi: 10.1097/00001648-199901000-00008
32. Asai, T, Kojima, A, Harada, K, Ishihara, K, Takahashi, T, and Tamura, Y. Correlation between the usage volume of veterinary therapeutic antimicrobials and resistance in Escherichia coli isolated from the feces of food-producing animals in Japan. Jpn J Infect Dis. (2005) 58:369–72. doi: 10.7883/yoken.JJID.2005.369
33. WHO. WHO list of medically important antimicrobials. (2024). Available at: https://cdn.who.int/media/docs/default-source/gcp/who-mia-list-2024-lv.pdf (Accessed November 25, 2023).
34. OIE. OIE List of Antimicrobial Agents of Veterinary Importance. (2019). Available at: https://rr-africa.oie.int/wp-content/uploads/2019/09/eng_oie_list_antimicrobials_may2015.pdf (Accessed November 25, 2021).
35. Hu, L-t, and Bentler, PM. Cutoff criteria for fit indexes in covariance structure analysis: conventional criteria versus new alternatives. Struct Equ Model. (1999) 6:1–55. doi: 10.1080/10705519909540118
36. Pituch, KA, and Stevens, JP. Applied multivariate statistics for the social sciences: Analyses with SAS and IBM’s SPSS. 6th ed. London, UK: Routledge (2015).
37. Pereira, A, Sidjabat, HE, Davis, S, Vong da Silva, PG, Alves, A, Dos Santos, C, et al. Prevalence of antimicrobial resistance in Escherichia coli and Salmonella species isolates from chickens in live bird markets and boot swabs from layer farms in Timor-Leste. Antibiotics (Basel). (2024) 13:120. doi: 10.3390/antibiotics13020120
38. FAO. Monitoring and surveillance of antimicrobial resistance in bacteria from healthy food animals intended for consumption. (2023). Available at: https://openknowledge.fao.org/items/20f51889-bedc-4c14-8598-323a87a9a896 (Accessed November 25, 2023).
39. Azad, M, Rahman, MM, Amin, R, Begum, MIA, Fries, R, Husna, A, et al. Susceptibility and multidrug resistance patterns of Escherichia coli isolated from cloacal swabs of live broiler chickens in Bangladesh. Pathogens. (2019) 8:118. doi: 10.3390/pathogens8030118
40. Parvin, MS, Ali, MY, Mandal, AK, Talukder, S, and Islam, MT. Sink survey to investigate multidrug resistance pattern of common foodborne bacteria from wholesale chicken markets in Dhaka city of Bangladesh. Sci Rep. (2022) 12:10818. doi: 10.1038/s41598-022-14883-7
41. Liu, C, Wang, P, Dai, Y, Liu, Y, Song, Y, Yu, L, et al. Longitudinal monitoring of multidrug resistance in Escherichia coli on broiler chicken fattening farms in Shandong, China. Poult Sci. (2021) 100:100887. doi: 10.1016/j.psj.2020.11.064
42. Eltai, NO, Abdfarag, EA, Al-Romaihi, H, Wehedy, E, Mahmoud, MH, Alawad, OK, et al. Antibiotic resistance profile of commensal Escherichia coli isolated from broiler chickens in Qatar. J Food Prot. (2018) 81:302–7. doi: 10.4315/0362-028x.Jfp-17-191
43. Saeed, MA, Saqlain, M, Waheed, U, Ehtisham-Ul-Haque, S, Khan, AU, Rehman, AU, et al. Cross-sectional study for detection and risk factor analysis of ESBL-producing avian pathogenic Escherichia coli associated with backyard chickens in Pakistan. Antibiotics (Basel). (2023) 12:934. doi: 10.3390/antibiotics12050934
44. Read, AF, and Woods, RJ. Antibiotic resistance management. Evol Med Public Health. (2014) 2014:147. doi: 10.1093/emph/eou024
45. Rothrock, MJ Jr, Gibson, KE, Micciche, AC, and Ricke, SC. Pastured poultry production in the United States: strategies to balance system sustainability and environmental impact. Front Sustain Food Syst. (2019) 3:74. doi: 10.3389/fsufs.2019.00074
46. Chang, Q, Wang, W, Regev-Yochay, G, Lipsitch, M, and Hanage, WP. Antibiotics in agriculture and the risk to human health: how worried should we be? Evol Appl. (2015) 8:240–7. doi: 10.1111/eva.12185
47. WHO. Antimicrobial resistance. (2020). Available at: https://www.who.int/news-room/fact-sheets/detail/antimicrobial-resistance (Accessed November 25, 2023).
48. Singer, AC, Shaw, H, Rhodes, V, and Hart, A. Review of antimicrobial resistance in the environment and its relevance to environmental regulators. Front Microbiol. (2016) 7:1728. doi: 10.3389/fmicb.2016.01728
49. Alam, M-U, Rahman, M, Abdullah Al, M, Islam, MA, Asaduzzaman, M, Sarker, S, et al. Human exposure to antimicrobial resistance from poultry production: assessing hygiene and waste-disposal practices in Bangladesh. Int J Hyg Environ Health. (2019) 222:1068–76. doi: 10.1016/j.ijheh.2019.07.007
50. Racewicz, P, Majewski, M, Biesiada, H, Nowaczewski, S, Wilczyński, J, Wystalska, D, et al. Prevalence and characterisation of antimicrobial resistance genes and class 1 and 2 integrons in multiresistant Escherichia coli isolated from poultry production. Sci Rep. (2022) 12:6062. doi: 10.1038/s41598-022-09996-y
51. FAO. Biosecurity for highly pathogenic avian influenza. (2008). Available at: http://www.fao.org/docs/eims/upload/248381/i0359e00.pdf (Accessed November 25, 2023).
52. Roess, AA, Winch, PJ, Akhter, A, Afroz, D, Ali, NA, Shah, R, et al. Household animal and human medicine use and animal husbandry practices in rural Bangladesh: risk factors for emerging zoonotic disease and antibiotic resistance. Zoonoses Public Health. (2015) 62:569–78. doi: 10.1111/zph.12186
53. Simon, K, Verwoolde, M, Zhang, J, Smidt, H, de Vries, RG, Kemp, B, et al. Long-term effects of early life microbiota disturbance on adaptive immunity in laying hens, poultry science (2016) 95:1543–54. doi: 10.3382/ps/pew088,
54. Nguyen, VC, Bach, TK, Doan, HP, Van Nguyen, TB, Vo, BH, Thwaites, G, et al. Effects of prophylactic and therapeutic antimicrobial uses in small-scale chicken flocks. Zoonoses Public Health. (2020) 68:483–92. doi: 10.1111/zph.12839
56. EUCAST. Available breakpoints of antimicrobials for veterinary use. (2015). Available at: https://www.eucast.org/fileadmin/src/media/PDFs/EUCAST_files/VetCAST/VetDocuments/Closed_session_VetCAST_breakpoints_27-04-15_def_KV.PDF (Accessed November 25, 2023).
57. Catry, B, Cavaleri, M, Baptiste, K, Grave, K, Grein, K, Holm, A, et al. Use of colistin-containing products within the European Union and European economic area (EU/EEA): development of resistance in animals and possible impact on human and animal health. Int J Antimicrob Agents. (2015) 46:297–306. doi: 10.1016/j.ijantimicag.2015.06.005
Keywords: Escherichia coli , Salmonella spp., broiler, layer, commercial farm, Chattogram
Citation: Foysal M, Imam T, Das SB, Gibson JS, Mahmud R, Gupta SD, Fournié G, Hoque MA and Henning J (2024) Association between antimicrobial usage and resistance on commercial broiler and layer farms in Bangladesh. Front. Vet. Sci. 11:1435111. doi: 10.3389/fvets.2024.1435111
Received: 19 May 2024; Accepted: 06 August 2024;
Published: 29 August 2024.
Edited by:
Roswitha Merle, Free University of Berlin, GermanyReviewed by:
František Zigo, University of Veterinary Medicine and Pharmacy in Košice, SlovakiaCopyright © 2024 Foysal, Imam, Das, Gibson, Mahmud, Gupta, Fournié, Hoque and Henning. This is an open-access article distributed under the terms of the Creative Commons Attribution License (CC BY). The use, distribution or reproduction in other forums is permitted, provided the original author(s) and the copyright owner(s) are credited and that the original publication in this journal is cited, in accordance with accepted academic practice. No use, distribution or reproduction is permitted which does not comply with these terms.
*Correspondence: Tasneem Imam, dC5pbWFtQHVxY29ubmVjdC5lZHUuYXU=
†These authors have contributed equally to this work and share first authorship
Disclaimer: All claims expressed in this article are solely those of the authors and do not necessarily represent those of their affiliated organizations, or those of the publisher, the editors and the reviewers. Any product that may be evaluated in this article or claim that may be made by its manufacturer is not guaranteed or endorsed by the publisher.
Research integrity at Frontiers
Learn more about the work of our research integrity team to safeguard the quality of each article we publish.