- College of Veterinary Medicine, Gyeongsang National University, Jinju, Republic of Korea
Background: The effect of acidemia on blood coagulation remains inadequately understood in veterinary medicine. Therefore, we assessed the effect of in vitro acidification of canine whole blood on coagulation and investigated whether acidemia-induced coagulopathy could be reversed by reversing acidemia.
Methods: Citrated whole blood samples were taken from six healthy Beagle dogs and categorized, based on pH adjustment, into neutral, weak acidemia (WA), strong acidemia (SA), and reversal from SA. Then, prothrombin time (PT), activated partial thromboplastin time (aPTT), fibrinogen concentration, conventional thromboelastography (TEG) parameters, and velocity curve (V-curve) variables of TEG were assessed.
Results: The PT, aPTT, and most TEG parameters showed significant coagulopathy in the SA group compared to the neutral group, with additional significant changes in reaction time (R), clot kinetic (K), maximum amplitude (MA), split point (SP), elasticity (E), thrombodynamic potential index (TPI), and coagulation index (CI) between the SA and WA groups. Among V-curve variables, the maximum rate of thrombus generation (MRTG) and total thrombus generation were significantly inhibited in the SA group compared to the neutral group, with significant differences in the time to maximum rate of thrombus generation (TMRTG) between the WA and SA groups. In the reverse group, aPTT, R, K, α-angle, MRTG, TMRTG, SP, TPI, and CI exhibited significant recovery compared to the SA group.
Conclusion: The in vitro induction of acidemia in canine whole blood leads to impairment of coagulation profiles, and pH correction can reverse most acidemia-induced coagulopathy.
1 Introduction
Blood pH is tightly regulated within a narrow range (pH: 7.35–7.45) and deviations from this range can have serious health implications. Acidemia is commonly observed in critically ill patients with metabolic derangements or trauma, and it also plays a significant role in veterinary medicine, influencing various animal diseases and pathophysiological conditions such as respiratory diseases (e.g., pneumonia, asthma), metabolic disorders like diabetic ketoacidosis and renal failure, hypoxia-related conditions such as septic shock and heart failure, poisoning from substances like ethylene glycol or salicylates, liver failure, and pregnancy toxemia in ruminants. Acidemia has several adverse effects including decreased cardiac contractility, ventricular arrhythmia, arterial vasodilation, and catecholamine resistance (1–4). Ultimately, acidemia can influence the body’s ability to form and maintain blood clots, resulting in coagulopathy. This association is evident in the well-known “lethal triad” of hypothermia, acidosis, and coagulopathy, which has been linked to increased mortality after major trauma (5–8).
Previous in vitro human studies demonstrated a strong correlation between pH levels and coagulopathy in blood samples infused with acid, typically hydrochloric acid (HCl), from healthy volunteers (9, 10). In vivo studies of swine models found that HCl-induced acidemia leads to coagulopathy, as measured by prothrombin time (PT), activated partial thromboplastin time (aPTT), fibrinogen concentration, conventional thromboelastography (TEG) parameters, and thrombin generation tests (11, 12). However, research on the effects of acidemia on coagulation is limited in veterinary medicine, and the results are often inconsistent (13–15).
Considering the lack of research in this field on dogs, the primary objective of this study was to investigate the effects of in vitro acidification of canine whole blood on coagulation to identify the correlation between acidemia and coagulopathy. Furthermore, this study aimed to investigate whether acidemia-induced coagulopathy could be reversed by reversing acidemia.
2 Materials and methods
2.1 Study design
This study used an in vitro assay to evaluate the effects of acidification of canine whole blood on coagulation and investigate the potential recovery of these effects upon reversing acidemia. Blood samples were categorized into four groups: weak acidemia (WA), strong acidemia (SA), neutral, and reverse. The acidification group was further divided into WA and SA groups to assess progressive coagulation impairment with worsening acidemia (15, 16). The research protocol was reviewed and approved by the Institutional Animal Care and Use Committee (IACUC) GNU-231017-D0193 of Gyeongsang National University (GNU).
2.1.1 Blood sample collection
Six Beagle dogs consisting of four castrated males and two spayed females with median body weight and age of 10.8 ± 1.3 kg and 7.0 ± 1.4 years, respectively, were included in this study. The dogs were deemed healthy based on physical examination, complete blood count (Procyte Dx Hematology Analyzer; IDEXX, Westbrook, ME, United States), serum biochemistry analysis (Catalyst Dx® Chemistry Analyzer; IDEXX, Westbrook, ME, United States), acid–base balance and electrolyte concentration analysis, and coagulation profiles.
Blood samples were collected from each dog using the two-syringe method with a 21 G butterfly needle, following the recommended guideline to minimize stasis for TEG analysis (17). The procedure involved discarding the initial 3 mL of blood drawn from the jugular vein and collecting 10 mL of blood from each dog. The 10 mL blood samples were transferred into five sodium citrate 2-mL tubes, each containing 0.2 mL of buffered 3.2% sodium citrate, resulting in a volume ratio of 1:9 (Vacuette, 3.2% sodium citrate, 2-mL tubes; Greiner Bio-One, Kremsmünster, Austria). Subsequently, the citrated blood tubes were gently inverted 5–7 times to ensure that the ratio of sodium citrate to whole blood was maintained at 1:9. Four tubes were used for the neutral, WA, SA, and reverse groups, and one tube was used for pH adjustment.
2.1.2 Blood sample acidification and reversal
The blood samples in the acidification group were all acidified using the same 1 M HCl (Sigma-Aldrich, St. Louis, MO, United States) solution. The pH change was measured after adding 10 μL of 1 M HCl to the blood sample for pH adjustment. Subsequently, 1 M HCl was added to the other two citrate tubes to obtain the WA and SA samples (target pH: 6.8–7.0 and 6.5–6.6, respectively). The median volume of HCl administered for acidemia induction was 30 μL (range, 25.0–32.5 μL) and 59.5 μL (range, 51–60 μL) in the WA and SA groups, respectively. The fourth blood sample was used as the neutral group, and an amount of distilled water similar to that of the HCl administered to induce SA was added to eliminate the dilutional effect. Strong acidemia was induced and then reversed by adding 45 μL of Tris (hydroxymethyl) aminomethane buffer (1.5 M Tris–HCl, pH, 8.8, Bio-Solution co., LTD., Suwon, Korea) for the reverse group. Tris buffer was used instead of sodium bicarbonate for acidemia reversal because CO2 is generated when bicarbonate neutralizes protons (H+), which is unsuitable for in vitro experiments conducted in closed systems.
The pH of the blood samples was confirmed using the blood gas analyzer (Stat Profile® pHOX Ultra Analyzer; Nova Biochemical, Waltham, MA, United States) immediately after manipulation.
2.2 Analysis of coagulation profiles
Point-of-care PT, aPTT, fibrinogen concentration, and TEG were analyzed within 2 h of blood collection using the citrated whole blood.
2.2.1 TEG
The samples were analyzed using a single TEG® 5,000 Thromboelastograph Hemostasis Analyzer (Haemonetics Corp, Braintree, Massachusetts, United States). TEG is a non-invasive test that quantitatively measures the ability of whole blood to form a clot. The test detects and quantifies dynamic changes in the viscoelastic properties of blood during clotting under low shear stress. Citrated whole blood samples were analyzed after a 30-min resting period at room temperature, following a previously recommended protocol (17). All the samples were activated using kaolin, in accordance with the manufacturer’s recommendations.
Regarding TEG conventional parameters, the reaction time (R) represents initial clot latency, the clot kinetic (K) is the duration from the initial to maximum clot formation, the α-angle measures the rapidity of fibrin buildup and cross-linking, and the maximum amplitude (MA) represents the maximum strength of the clot (Figure 1A).
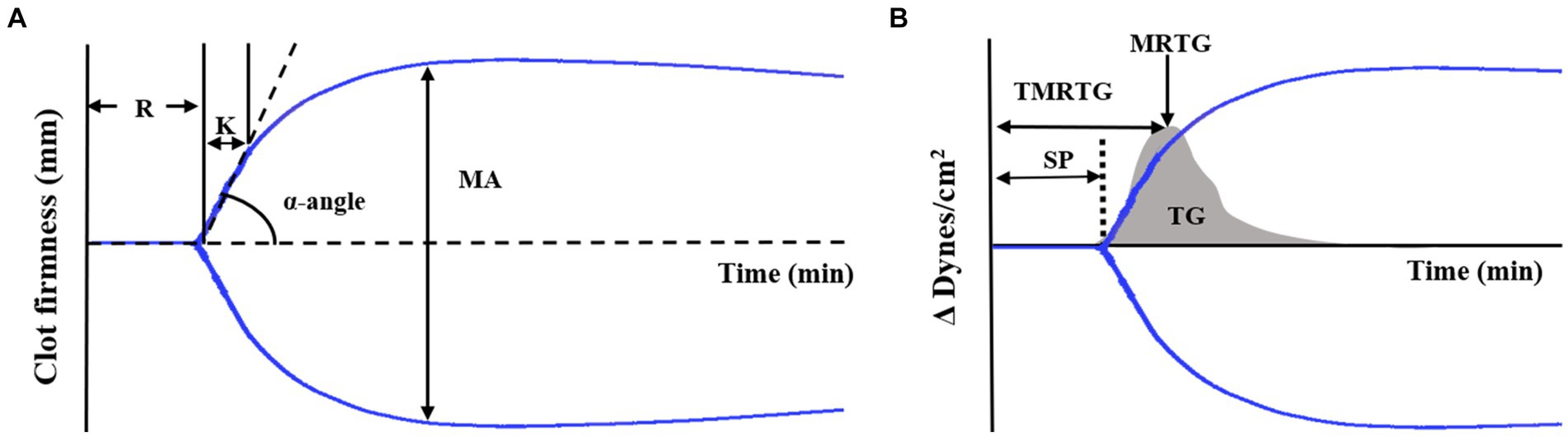
Figure 1. TEG tracing and velocity (first derivative) curve (A) Standard TEG tracing. The four conventional coagulation parameters (R, K, α-angle, MA) can be combined to yield indices of coagulability. (B) TEG showing the standard tracing (black line) with the superimposed velocity curve (gray solid curve). Velocity curve parameters are generated from the mathematical first derivative of the standard TEG tracing and describe the formation of the thrombus. Thromboelastography (TEG), reaction time (R), clot kinetic (K), α-angle, maximum amplitude (MA), split point (SP), maximum rate of thrombus generation (MRTG), time to maximum rate of thrombus generation (TMRTG), and total thrombus generation (TG).
Additionally, the TEG system software calculated the first-degree derivative velocity curve (V-curve), providing information about thrombin generation (Figure 1B). The MRTG corresponds to the peak of the clot formation curve and the time to maximum rate of thrombus generation (TMRTG) is determined by measuring the time it takes for the clot to reach its peak formation rate. Moreover, TG is calculated by assessing the area under the thrombus formation rate curve (18).
Furthermore, several non-conventional TEG parameters were analyzed. These parameters included split point (SP, the first point at which the arms of the thromboelastogram diverge) (Figure 1B), amplitude (A, equal to MA until MA is determined), shear elastic modulus strength (G, clot elasticity at the specific time of reaching the maximal amplitude, calculated from A as follows: G = 5,000A/[100-A]), elasticity (E, a normalized G parameter, calculated as E = 100A/[100-A]), thrombodynamic potential index (TPI, describing the global coagulation, calculated as SP/R + K), coagulation index (CI, describing overall coagulation, calculated as 0.1227 × R + 0.0092 × K + 0.1654 × MA − 0.0241 × Angle−5.0220), and time to maximum amplitude (TMA, the time needed to form a stable clot).
2.2.2 Point-of-care PT and aPTT
After the TEG analysis, PT and aPTT were measured using a point-of-care device and cartridges (Coag Dx™ Analyzer, Citrate PT, and Citrate aPTT cartridges; IDEXX, Westbrook, ME, United States). The measurements were conducted using the same citrate tube used for the TEG analysis, following the manufacturer’s guidelines.
2.2.3 Fibrinogen concentration
The remaining citrated blood was centrifuged at 2000 × g for 15 min, and fibrinogen analysis was conducted using the supernatant of the citrated plasma. Fibrinogen concentration was determined using the STA-Compact-Max® analyzer (Diagnostica Stago, Asnières sur Seine, France), following the manufacturer’s instructions.
2.3 Statistical methods
All statistical analyses were performed using SPSS for Windows (version 27.0; SPSS Inc., Chicago, IL, United States) with nonparametric statistical tests. Statistical analysis was performed using the initial Kruskal–Wallis test, followed by the post-hoc Mann–Whitney U test with Bonferroni correction to compare the neutral, WA, and SA variables. The Mann–Whitney U test was used to compare the two groups (SA and reverse). The Spearman rank order test was used for correlation analysis between pH and coagulation impairment. The results are provided as median (range), represented using box plots, with boxes representing the 25–75th percentiles and whiskers representing the minimum and maximum values. Statistical significance was set at p < 0.05. All graphical analyses were performed using the GraphPad Prism 5 software (GraphPad Software, La Jolla, CA, United States).
3 Results
The median pH was 7.25 (range, 7.22–7.28), 6.87 (range, 6.82–6.91), and 6.57 (range, 6.51–6.58) for the neutral, WA, and SA groups, respectively. Severe acidemia was successfully corrected with Tris buffer for the reverse group, resulting in a median pH of 7.26 (range, 7.14–7.28) (Table 1).

Table 1. Effect of acidification and reversal on pH level, PT, aPTT, and fibrinogen concentration for each group.
3.1 Effect of acidification and reversal on PT, aPTT, and fibrinogen concentration
The PT (p = 0.001) and aPTT (p < 0.001) tests showed significant prolongation in the SA group compared to the neutral group. Additionally, a significant prolongation was observed in aPTT in the SA group compared to the WA group (p < 0.001) (Table 1). A negative correlation between pH value and PT (r = −0.663, p < 0.001) as well as aPTT (r = −0.866, p < 0.001) were observed within the pH range 7.3–6.5 (Figure 2). A significant recovery of aPTT was observed in the reverse group compared to the SA group (p = 0.002) upon acidemia reversal. Furthermore, PT showed a tendency to recover in response to acidemia reversal compared to the SA group; however, statistical significance was not achieved (Table 1). No significant change was observed in fibrinogen concentration (Table 1).
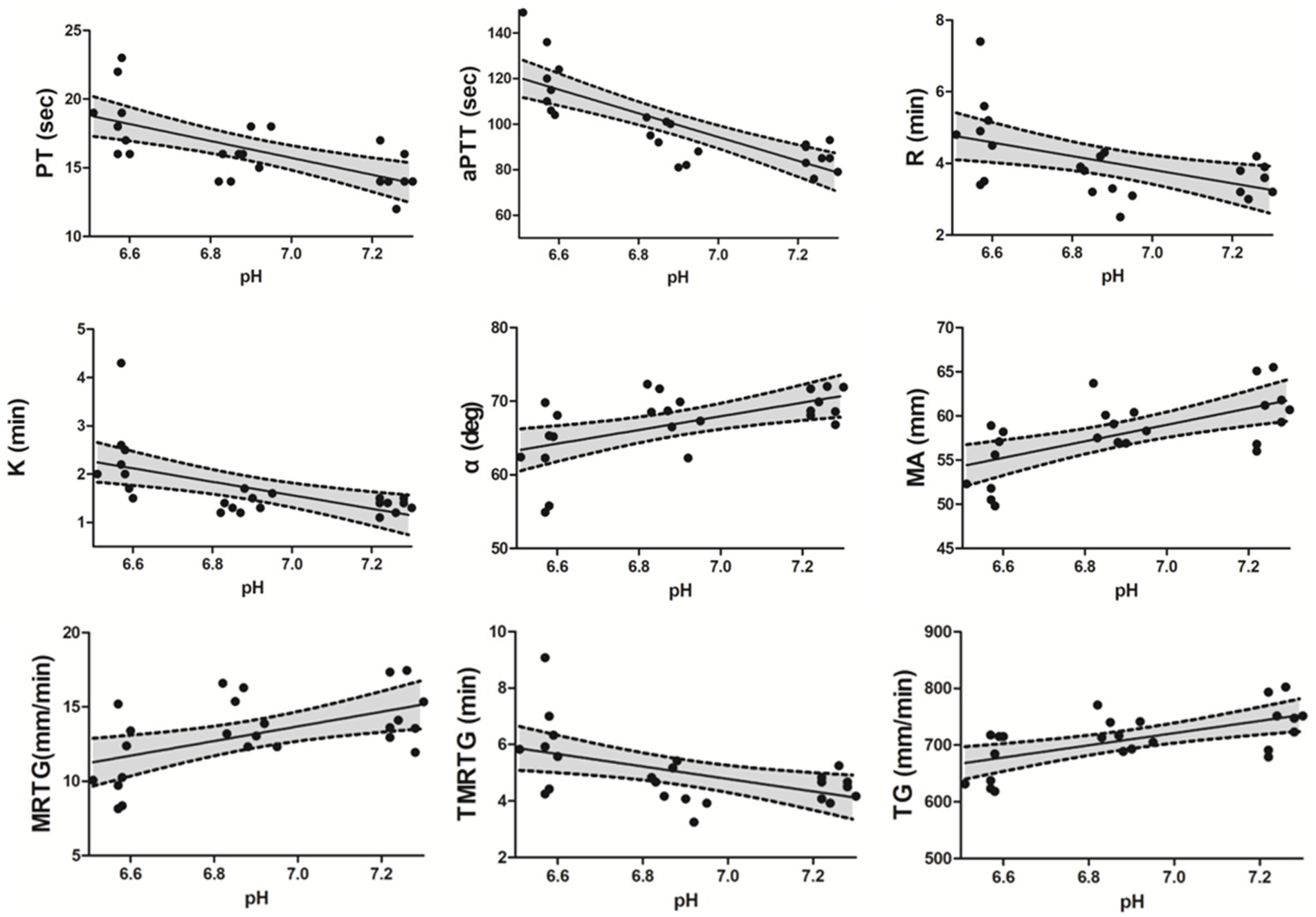
Figure 2. Spearman correlations between PT, aPTT, conventional TEG parameters (R, K, α-angle, and MA), and pH level, as well as between TEG velocity curve parameters and pH level. A negative correlation was observed between pH level and PT (r = −0.663, p < 0.001), aPTT (r = −0.866, p < 0.001), R (r = −0.533, p = 0.007), K (r = −0.645, p < 0.001), and TMRTG (r = −0.530, p = 0.008) within the pH range 6.5–7.3, whereas a positive correlation was observed between pH level and α-angle (r = 0.514, p = 0.01), MA (r = 0.638, p = 0.001), MRTG (r = 0.472, p = 0.02) as well as TG (r = 0.603, p = 0.002). A 95% confidence interval (CI) is reported in each scatter plot. Prothrombin time (PT), activated partial thromboplastin time (aPTT), Thromboelastography (TEG), reaction time (R), clot kinetic (K), α-angle, maximum amplitude (MA), maximum rate of thrombus generation (MRTG), time to maximum rate of thrombus generation (TMRTG), and total thrombus generation (TG).
3.2 Effect of acidification and reversal on TEG parameters
Significant differences were identified in conventional TEG parameters, including K (p < 0.001), α-angle (p = 0.003), and MA (p = 0.005) between the neutral and SA groups (Table 2). Additionally, significant changes were observed in R (p = 0.01), K (p = 0.001), and MA (p = 0.015) in the SA group compared to the WA group (Table 2). A negative correlation was observed between pH value and R (r = −0.533, p = 0.007) as well as K (r = −0.645, p < 0.001) within the pH range 7.3–6.5. A positive correlation was observed between pH value and α-angle (r = 0.514, p = 0.01) as well as MA (r = 0.638, p < 0.001) (Figure 2). Significant recovery of R (p = 0.001), K (p < 0.001), and α-angle (p < 0.001) was observed in the reverse group compared to the SA group. Moreover, in the reverse group compared with the SA group, MA increased without significant difference (Table 2).
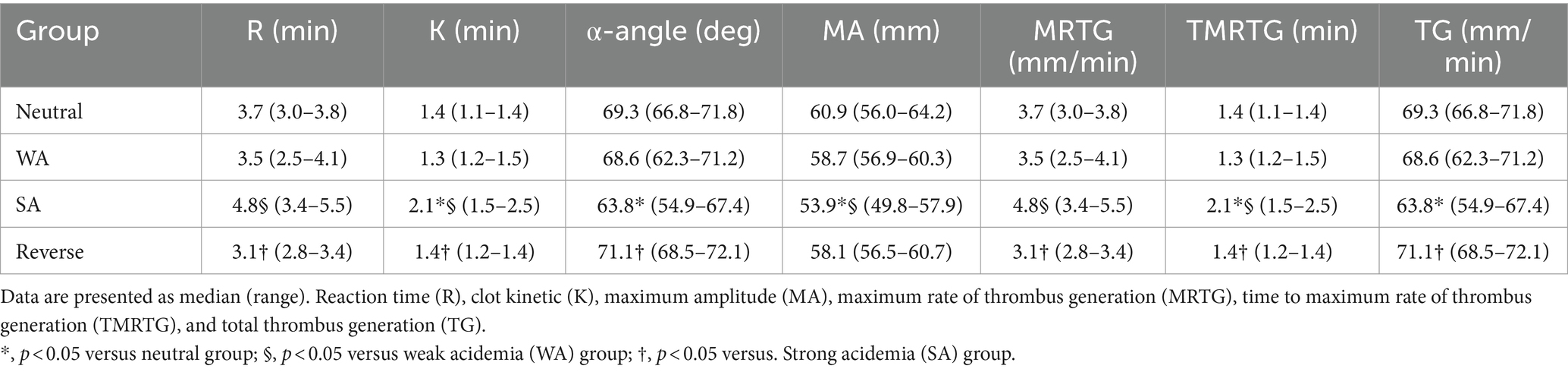
Table 2. Effect of acidification and reversal on TEG conventional parameters and TEG velocity curve parameters for each group.
In the SA group compared with the neutral group, MRTG (p = 0.01) and TG (p = 0.007) were significantly inhibited (Table 2). Moreover, a significant difference in the TMRTG (p = 0.01) was identified between the WA and SA groups (Table 2). A positive correlation was observed between pH value and MRTG (r = 0.472, p = 0.02) as well as TG (r = 0.603, p = 0.002), and a negative correlation was observed between pH and TMRTG (r = −0.530, p = 0.008) (Figure 2). Furthermore, MRTG (p = 0.01) and TMRTG (p = 0.001) significantly recovered in the reverse group compared to the SA group, whereas TG increased with pH correction, although non-significantly (Table 2).
Nonconventional TEG parameters showed significant differences in SP, G, E, TPI, A, and CI, except TMA, between the neutral and SA groups (Table 3). Additionally, SP (p = 0.015), E (p = 0.015), TPI (p = 0.01), and CI (p = 0.01) showed significant differences between the WA and SA groups (Table 3). A negative correlation was observed between the pH value and SP (r = −0.4534, p = 0.007) within the pH range 7.3–6.5, whereas a positive correlation was observed between the pH value and G (r = 0.636, p < 0.001), E (r = 0.638, p < 0.001), TPI (r = 0.60, p = 0.002), A (r = 0.602, p = 0.002) and CI (r = 0.744, p < 0.001) (Figure 3). Additionally, SP (p = 0.003), TPI (p = 0.01), and CI (p < 0.001) showed significant recovery between the reverse and SA groups. Similarly, G, E, and A values increased compared to those in the SA group, although non-significantly (Table 3).
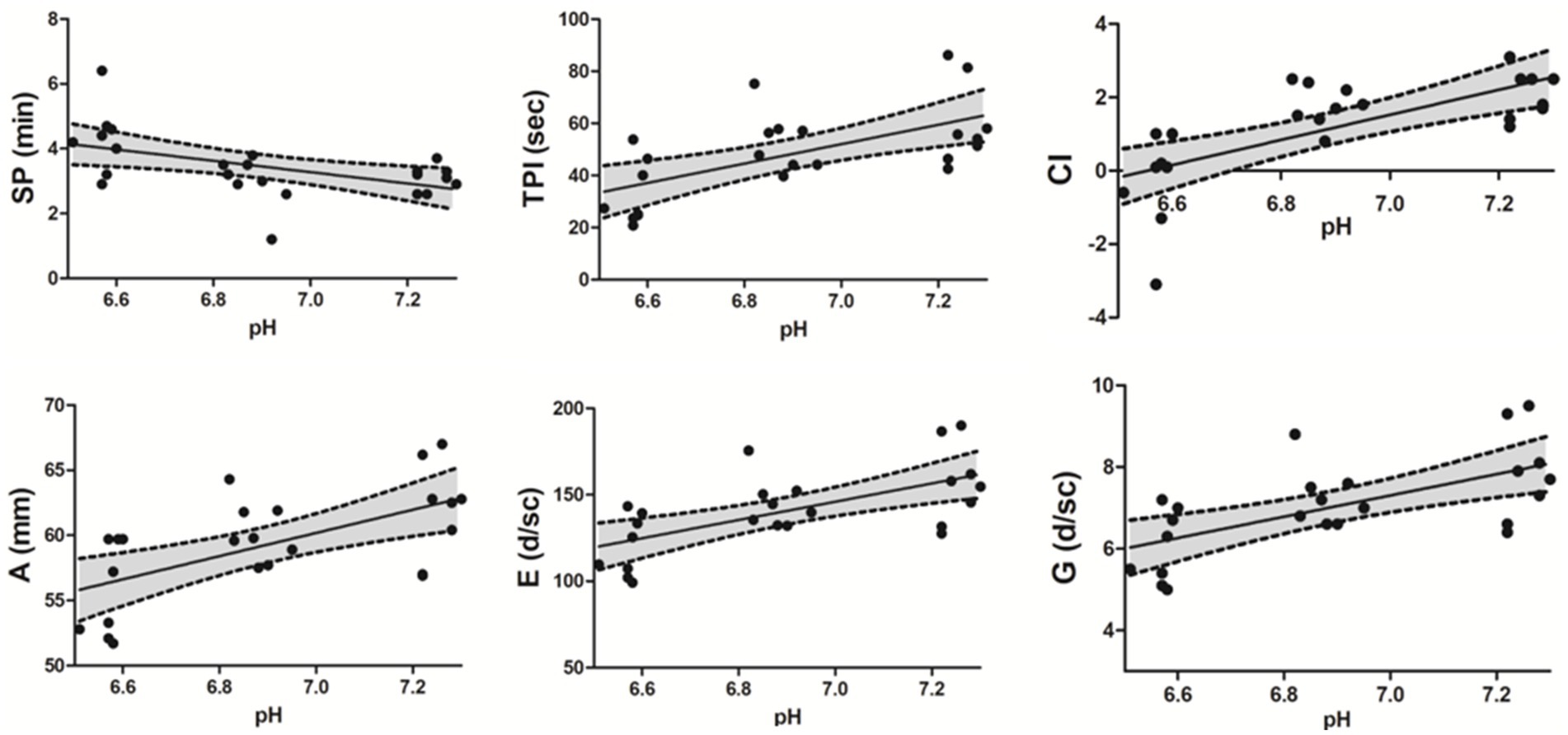
Figure 3. Spearman correlations between nonconventional TEG parameters and pH level. A negative correlation was observed between pH level and SP (r = −0.534, p = 0.007) within the pH range 6.5–7.3, whereas a positive correlation was observed between pH level and G (r = 0.636, p < 0.001), E (r = 0.638, p < 0.001), TPI (r = 0.60, p = 0.002), A (r = 0.602, p = 0.002), and CI (r = 0.744, p < 0.001). A 95% confidence interval (CI) is reported in each scatter plot. Thromboelastography (TEG), split point (SP), thrombodynamic potential index (TPI), coagulation index (CI), amplitude (A), elasticity or elastic modulus (E), Shear Elastic Modulus (G).
4 Discussion
This study investigated the effects of acidemia on coagulation in dogs using several coagulation profiles and the recovery from acidemia-induced coagulopathy after reversing acidemia. The in vitro induction of acidemia from pH 7.28 to 6.51 in canine whole blood significantly impaired most coagulation parameters, and a correlation was observed between pH and these coagulation impairments, except for fibrinogen and TMA levels. Most coagulation profiles exhibited significant recovery upon acidemia reversal.
Acidemia significantly and progressively prolonged PT and aPTT as the pH decreased. In general, PT and aPTT are used to evaluate clotting ability, and significant changes in them indicate the inhibition of overall coagulation factor activity (19–23). Lowering the pH value of whole blood to 7.0 decreased FVIIa activity by >90% and FVIIa/TF activity by >60%, and the rate of prothrombin activation by FXa/Fva complexes by approximately 70% (24). Previous in vivo swine studies demonstrated that PT and aPTT were prolonged when acidemia was induced (25, 26). Consequently, acidemia significantly affects the coagulation process by interfering with the activation of coagulation factors.
Thrombin generation is a crucial step in blood clot formation (27, 28). This process involves a series of complex biochemical reactions that ultimately lead to the conversion of fibrinogen into fibrin strands (29, 30). The thrombus velocity curve derived from conventional TEG parameters was first described by Sorensen et al. and showed a “profile similarity” to thrombin generation curves (31). Rivard et al. revealed a strong correlation between TG—derived from the first derivative of the TEG waveform—and thrombin generation—assessed using the thrombin/antithrombin (TAT) complex—indicating the potential utility of the V-curve as a surrogate marker for thrombin generation (32). In this study, MRTG, TMRTG, and TG were inhibited by severe acidemia, and coagulation impairment progressively worsened with decreasing pH. A study examining changes in thrombin generation kinetics under acidic conditions using swine found that initial thrombin generation at pH 7.1 was moderately delayed. After initial thrombin formation, acidemia drastically inhibited the thrombin generation rate during the propagation phase (33). Therefore, the inhibition of TEG V-curve variables observed in this experiment indicates that acidemia suppresses thrombin generation.
Severe acidemia affected other TEG parameters. Prolonged R time indicated delayed fibrin clot formation due to acidemia, reflecting the inhibition of coagulation factor activation. Moreover, K time and α-angle are primarily influenced by fibrinogen levels and, to a lesser extent, platelet function. Fibrinogen is a crucial protein in the blood clotting process and acts as a precursor to fibrin. Previous in vivo swine experiments demonstrated a significant decrease in fibrinogen concentration during acidemia (11, 12) and recent research has revealed that acidemia increases fibrinogen degradation by 1.8-fold (25). However, unlike the results of previous studies, this study did not confirm any change in fibrinogen concentration with acidemia. The reason for the lack of significant changes in fibrinogen concentration is unclear but may be attributed to the in vitro design, which cannot reproduce the fibrinogen degradation process caused by the complex and diverse factors in living organisms. Nevertheless, K and α-angle, which are significantly influenced by fibrinogen, were notably impaired in this experiment, leading to the assumption that this may have resulted from the acidemia-induced fibrinogen dysfunction.
The MA and G values significantly decreased with acidemia, indicating that acidemia weakens blood clot strength. The inhibition of platelet function may have played a significant role in these changes since no significant changes in fibrinogen levels were observed; this is supported by previous studies showing that platelet function is suppressed in acidic environments (34). Another study measuring platelet aggregation in canine blood found that increasing acidemia led to a mild but significant decrease in platelet aggregation (35). Although TEG parameters do not independently assess platelet function, the collective findings of these studies support the conclusion that acidemia affects platelet function. However, this may have been partially influenced by fibrinogen dysfunction, as previously described.
Furthermore, significant impairment was observed in nonconventional TEG parameters in severe acidemia. This impairment progressively increased as the pH decreased. The significant impairment in these parameters was likely caused by the inhibition of coagulation factor activation, thrombin generation, and platelet function since these parameters are calculated from conventional TEG (R, K, Angle, and MA) and G parameters.
This experiment demonstrated impairment in most coagulation parameters in severe acidemia; however, the results of some previous in vitro studies of humans and dogs showed that acidemia alone resulted in mild or no coagulation impairment (15, 36–38). A study assessing the effects of an acidic environment on coagulation dynamics using synthetic plasma reported that the rate constants for antithrombin (AT) inhibition were reduced by approximately 25–30% at pH 7.0 compared to pH 7.4. This suggests that the decreased reactivity of AT, which is suppressed in acidic environments, toward coagulation proteases may counteract the impairment of procoagulant catalysis caused by acidification. Accordingly, the severity of acidemia and features of in vitro experiments may have influenced the results. Acidemia was induced at levels similar to those in our WA group in previous in vitro experiments in which acidemia alone could not confirm apparent coagulation failure (15, 36–38). Similar to previous results, no statistically significant coagulation failure was confirmed in the WA group in this experiment compared to the neutral group. Therefore, the lack of apparent coagulation failure can be explained by the AT inhibition effect at pH levels similar to those in our WA group. However, the hypocoagulable tendency is presumed to have a more significant effect than the AT inhibition in severe acidemia (< pH 6.6), as confirmed in a preliminary study (data not shown) and the results of this study.
However, in vivo studies using a swine model (11, 12) confirmed the impairment of most coagulation parameters, even at pH 7.1. In vitro studies in which blood is acidified outside the patient are difficult to compare with studies in which acidemia is present in the patient. In vitro acidification does not consider the possible effects of acidemia on tissues or the in vivo interplay with the endothelium. Significant coagulopathy can only be induced when unrealistic levels of acidemia are induced in vitro, which are difficult to achieve in vivo.
Therefore, this study induced more severe acidemia than that induced in previous in vitro studies to discern the distinct impact of in vitro acidemia in dogs, considering the limited research and inconsistent results in assessing the effect of acidemia on coagulation in dogs. Additionally, the significant PT and aPTT prolongation in this experiment can be attributed to testing equipment differences. The coagulation analyzer employed in this investigation possessed a broader measurement range, which may have contributed to the observed significant statistical differences, unlike what was observed when the STA-compact analyzer was utilized in a previous study (15).
Ultimately, reversing acidemia reversed the coagulation impairment in this study. Significant recovery was confirmed for many coagulation parameters, and a trend towards recovery was observed for all parameters, except fibrinogen and TMA, without significant differences. These findings are consistent with previous in vitro experiments using human whole blood (16). However, an in vivo study using a swine model showed that Tris buffer infusion successfully corrects pH but does not significantly ameliorate coagulopathy (26). The experimental results appear conflicting, and to the best of our knowledge, no studies investigating whether acidemia-induced coagulopathy can be recovered by reversing acidemia in dogs have been conducted. Further investigations are necessary to determine whether pH correction can improve acidemia-induced coagulopathy in vivo.
A potential limitation of this study is the in vitro design. In vitro experiments are minimally invasive and offer high control over experimental conditions. However, they may lack physiological relevance because they do not fully reproduce the complex interplay of physiological systems in living organisms, limiting their application in clinical settings. Another limitation is the small number of enrolled dogs. Additional research involving a larger number of dogs is required to ascertain whether coagulation failure also occurs in dogs with clinical acidosis and whether it can be reversed by correcting acidemia in vivo.
In conclusion, the in vitro induction of acidemia in canine whole blood resulted in the progressive impairment of various coagulation parameters, including alterations in PT, aPTT, TEG parameters, and velocity curves. Reversing acidemia has the potential to recover acidemia-induced coagulation impairments in vitro. Therefore, coagulation tests should be conducted on dogs with acidemia in clinical practice to confirm whether coagulation failure occurs owing to acidemia, as demonstrated by in vitro experiments. Further research is required to determine whether reversing acidemia can improve coagulation dysfunction in vivo.
Data availability statement
The raw data supporting the conclusions of this article will be made available by the authors, without undue reservation.
Ethics statement
The animal study was approved by Institutional Animal Care and Use Committee (IACUC) GNU-231017-D0193 of Gyeongsang National University (GNU). The study was conducted in accordance with the local legislation and institutional requirements.
Author contributions
YK: Conceptualization, Formal analysis, Methodology, Project administration, Resources, Validation, Writing – original draft, Writing – review & editing. HB: Methodology, Project administration, Resources, Supervision, Writing – original draft, Writing – review & editing. DY: Conceptualization, Formal analysis, Funding acquisition, Methodology, Resources, Supervision, Validation, Writing – original draft, Writing – review & editing.
Funding
The author(s) declare financial support was received for the research, authorship, and/or publication of this article. This research was supported by the Basic Science Research Program through the National Research Foundation of Korea (NRF), funded by the Ministry of Science and ICT & Future Planning (2020R1C1C1008675).
Conflict of interest
The authors declare that the research was conducted in the absence of any commercial or financial relationships that could be construed as a potential conflict of interest.
The author(s) declared that they were an editorial board member of Frontiers, at the time of submission. This had no impact on the peer review process and the final decision.
Publisher’s note
All claims expressed in this article are solely those of the authors and do not necessarily represent those of their affiliated organizations, or those of the publisher, the editors and the reviewers. Any product that may be evaluated in this article, or claim that may be made by its manufacturer, is not guaranteed or endorsed by the publisher.
References
1. Kraut, JA, and Madias, NE. Metabolic acidosis: pathophysiology, diagnosis and management. Nat Rev Nephrol. (2010) 6:274–85. doi: 10.1038/nrneph.2010.33
2. Mitchell, JH, Wildenthal, K, and Johnson, RL Jr. The effects of acid-base disturbances on cardiovascular and pulmonary function. Kidney Int. (1972) 1:375–89. doi: 10.1038/ki.1972.48
3. Davies, AO . Rapid desensitization and uncoupling of human β-adrenergic receptors in an in vitro model of lactic acidosis. J Clin Endocrinol Metab. (1984) 59:398–405. doi: 10.1210/jcem-59-3-398
4. Orchard, CH, and Cingolani, HE. Acidosis and arrhythmias in cardiac muscle. Cardiovasc Res. (1994) 28:1312–9. doi: 10.1093/cvr/28.9.1312
5. Eddy, VA, Morris, JA Jr, and Cullinane, DC. Hypothermia, coagulopathy, and acidosis. Surg Clin North Am. (2000) 80:845–54. doi: 10.1016/s0039-6109(05)70099-2
6. Lynn, M, Jeroukhimov, I, Klein, Y, and Martinowitz, U. Updates in the management of severe coagulopathy in trauma patients. Intensive Care Med. (2002) 28:s241–7. doi: 10.1007/s00134-002-1471-7
7. Thorsen, K, Ringdal, K, Strand, K, Søreide, E, Hagemo, J, and Søreide, K. Clinical and cellular effects of hypothermia, acidosis and coagulopathy in major injury. Br J Surg. (2011) 98:894–907. doi: 10.1002/bjs.7497
8. Cosgriff, N, Moore, EE, Sauaia, A, Kenny-Moynihan, M, Burch, JM, and Galloway, B. Predicting life-threatening coagulopathy in the massively transfused trauma patient: hypothermia and acidoses revisited. J Trauma. (1997) 42:857–62. doi: 10.1097/00005373-199705000-00016
9. Ramaker, AJ, Meyer, P, van der Meer, J, Struys, MM, Lisman, T, and van Oeveren, W. Effects of acidosis, alkalosis, hyperthermia and hypothermia on haemostasis: results of point-of-care testing with the thromboelastography analyser. Blood Coagul Fibrinolysis. (2009) 20:436–9. doi: 10.1097/MBC.0b013e32832dc327
10. Lv, X, Mao, Y, and Qin, Z. Evaluation for effects of severe acidosis on hemostasis in trauma patients using thrombelastography analyzer. Am J Emerg Med. (2018) 36:1332–40. doi: 10.1016/j.ajem.2017.12.037
11. Darlington, DN, Kheirabadi, BS, Delgado, AV, Scherer, MR, Martini, WZ, and Dubick, MA. Coagulation changes to systemic acidosis and bicarbonate correction in swine. J Trauma. (2011) 71:1271–7. doi: 10.1097/TA.0b013e318214f522
12. Martini, WZ, Dubick, MA, Pusateri, AE, Park, MS, Ryan, KL, and Holcomb, JB. Does bicarbonate correct coagulation function impaired by acidosis in swine? J Trauma. (2006) 61:99–106. doi: 10.1097/01.ta.0000215574.99093.22
13. Crowell, JW, and Houston, B. Effect of acidity on blood coagulation. Am J Phys. (1961) 201:379–82. doi: 10.1152/ajplegacy.1961.201.2.379
14. Hardaway, RM, Elovitz, MJ, Brewster, WR, and Houchin, DN. Clotting time of heparinized blood: influence of acidosis. Arch Surg. (1964) 89:701–5. doi: 10.1001/archsurg.1964.01320040117020
15. Burton, AG, Burges, J, Borchers, A, and Hopper, K. In vitro assessment of the effect of acidemia on coagulation in dogs. J Vet Emerg Crit Care. (2018) 28:168–72. doi: 10.1111/vec.12697
16. Engstrom, M, Schott, U, Romner, B, and Reinstrup, P. Acidosis impairs the coagulation: a thromboelastographic study. J Trauma. (2006) 61:624–8. doi: 10.1097/01.ta.0000226739.30655.75
17. Flatland, B, Koenigshof, AM, Rozanski, EA, Goggs, R, and Wiinberg, B. Systematic evaluation of evidence on veterinary viscoelastic testing part 2: sample acquisition and handling. J Vet Emerg Crit Care. (2014) 24:30–6. doi: 10.1111/vec.12142
18. Pommerening, MJ, Goodman, MD, Farley, DL, Cardenas, JC, Podbielski, J, Matijevic, N, et al. Early diagnosis of clinically significant hyperfibrinolysis using thrombelastography velocity curves. J Am Coll Surg. (2014) 219:1157–66. doi: 10.1016/j.jamcollsurg.2014.07.943
19. Brohi, K, Cohen, MJ, and Davenport, RA. Acute coagulopathy of trauma: mechanism, identification and effect. Curr Opin Crit Care. (2007) 13:680–5. doi: 10.1097/MCC.0b013e3282f1e78f
20. MacLeod, JB, Lynn, M, McKenney, MG, Cohn, SM, and Murtha, M. Early coagulopathy predicts mortality in trauma. J Trauma. (2003) 55:39–44. doi: 10.1097/01.TA.0000075338.21177.EF
21. Maegele, M, Lefering, R, Yucel, N, Tjardes, T, Rixen, D, Paffrath, T, et al. Early coagulopathy in multiple injury: an analysis from the German trauma registry on 8724 patients. Injury. (2007) 38:298–304. doi: 10.1016/j.injury.2006.10.003
22. Schreiber, MA, Perkins, J, Kiraly, L, Underwood, S, Wade, C, and Holcomb, JB. Early predictors of massive transfusion in combat casualties. J Am Coll Surg. (2007) 205:541–5. doi: 10.1016/j.jamcollsurg.2007.05.007
23. Martini, WZ, Cortez, DS, Dubick, MA, Park, MS, and Holcomb, JB. Thrombelastography is better than PT, aPTT, and activated clotting time in detecting clinically relevant clotting abnormalities after hypothermia, hemorrhagic shock and resuscitation in pigs. J Trauma. (2008) 65:535–43. doi: 10.1097/TA.0b013e31818379a6
24. Meng, ZH, Wolberg, AS, Monroe, DM 3rd, and Hoffman, M. The effect of temperature and pH on the activity of factor VIIa: implications for the efficacy of high-dose factor VIIa in hypothermic and acidotic patients. J Trauma. (2003) 55:886–91. doi: 10.1097/01.TA.0000066184.20808.A5
25. Martini, WZ, and Holcomb, JB. Acidosis and coagulopathy: the differential effects on fibrinogen synthesis and breakdown in pigs. Ann Surg. (2007) 246:831–5. doi: 10.1097/SLA.0b013e3180cc2e94
26. Martini, WZ, Dubick, MA, Wade, CE, and Holcomb, JB. Evaluation of tris-hydroxymethylaminomethane on reversing coagulation abnormalities caused by acidosis in pigs. Crit Care Med. (2007) 35:1568–74. doi: 10.1097/01.CCM.0000266682.74602.6A
27. Butenas, S, van’t, C, and Mann, K. “Normal” thrombin generation. Blood. (1999) 94:2169–78. doi: 10.1182/blood.V94.7.2169.419k22_2169_2178
28. Hemker, CH, Giesen, PL, Ramjee, M, Wagenvoord, R, and Béguin, S. The thrombogram: monitoring thrombin generation in platelet rich plasma. Thromb Haemost. (2000) 83:589–91. doi: 10.1055/s-0037-1613868
29. Mann, K, Brummel, K, and Butenas, S. What is all that thrombin for? J Thromb Haemost. (2003) 1:1504–14. doi: 10.1046/j.1538-7836.2003.00298.x
30. Furie, B, and Furie, BC. Mechanisms of thrombus formation. N Engl J Med. (2008) 359:938–49. doi: 10.1056/NEJMra0801082
31. Sorensen, B, Johansen, P, Christiansen, K, Woelke, M, and Ingerslev, J. Whole blood coagulation thrombelastographic profiles employing minimal tissue factor activation. J Thromb Haemost. (2003) 1:551–8. doi: 10.1046/j.1538-7836.2003.00075.x
32. Rivard, GE, Brummel-Ziedins, K, Mann, KG, Fan, L, Hofer, A, and Cohen, E. Evaluation of the profile of thrombin generation during the process of whole blood clotting as assessed by thrombelastography. J Thromb Haemost. (2005) 3:2039–43. doi: 10.1111/j.1538-7836.2005.01513.x
33. Martini, WZ, Pusateri, AE, Uscilowicz, JM, Delgado, AV, and Holcomb, JB. Independent contributions of hypothermia and acidosis to coagulopathy in swine. J Trauma. (2005) 58:1002–10; discussion 1009-10. doi: 10.1097/01.ta.0000156246.53383.9f
34. Etulain, J, Negrotto, S, Carestia, A, Pozner, RG, Romaniuk, MA, D'Atri, LP, et al. Acidosis downregulates platelet haemostatic functions and promotes neutrophil proinflammatory responses mediated by platelets. Thromb Haemost. (2012) 107:99–110. doi: 10.1160/TH11-06-0443
35. Lawson, CA, and Spangler, EA. The effect of adding lactic acid to canine whole blood on platelet aggregation as measured by impedance aggregometry. Vet Clin Pathol. (2020) 49:217–21. doi: 10.1111/vcp.12862
36. Gissel, M, Brummel-Ziedins, KE, Butenas, S, Pusateri, AE, Mann, KG, and Orfeo, T. Effects of an acidic environment on coagulation dynamics. J Thromb Haemost. (2016) 14:2001–10. doi: 10.1111/jth.13418
37. Mitrophanov, AY, Szlam, F, Sniecinski, RM, Levy, JH, and Reifman, J. Controlled multifactorial coagulopathy: effects of dilution, hypothermia, and acidosis on thrombin generation in vitro. Anesth Analg. (2020) 130:1063–76. doi: 10.1213/ANE.0000000000004479
Keywords: acidosis, dog, hemostasis, blood coagulation factors, thromboelastography
Citation: Kim Y, Bae H and Yu D (2024) The in vitro effects of acidemia and acidemia reversal on coagulation in dogs. Front. Vet. Sci. 11:1427237. doi: 10.3389/fvets.2024.1427237
Edited by:
Ursula Windberger, Medical University of Vienna, AustriaReviewed by:
Adam Deak, University of Debrecen, HungaryAnika Alexandrova-Watanabe, Institute of Mechanics (BAS), Bulgaria
Copyright © 2024 Kim, Bae and Yu. This is an open-access article distributed under the terms of the Creative Commons Attribution License (CC BY). The use, distribution or reproduction in other forums is permitted, provided the original author(s) and the copyright owner(s) are credited and that the original publication in this journal is cited, in accordance with accepted academic practice. No use, distribution or reproduction is permitted which does not comply with these terms.
*Correspondence: DoHyeon Yu, eXVkaEBnbnUuYWMua3I=