- 1Ophthalmology Unit, Department of Companion Animals and Horses, University of Veterinary Medicine Vienna, Vienna, Austria
- 2Centre for Animal Reproduction, Department of Companion Animals and Horses, University of Veterinary Medicine Vienna, Vienna, Austria
- 3Department of Biomedical Science and Pathobiology, University of Veterinary Medicine Vienna, Vienna, Austria
- 4VetCore Facility for Research, University of Veterinary Medicine Vienna, Vienna, Austria
- 5Research Unit Internal Medicine, Department of Companion Animals and Horses, University of Veterinary Medicine Vienna, Vienna, Austria
Angiogenesis plays a crucial role in various physiological and pathological conditions. However, research in equine angiogenesis is relative limited, necessitating the development of suitable in-vitro models. To effectively analyze angiogenesis in-vitro, it is essential to target the specific cells responsible for this process, namely endothelial cells. Human umbilical vein endothelial cells (HUVECs) are one of the most used in vitro models for studying angiogenesis in humans. Serving as an equivalent to HUVECs, we present a comprehensive isolation protocol for equine umbilical vein endothelial cells (EqUVECs) with relatively minimal requirements, thereby enhancing accessibility for researchers. Umbilical cords obtained from five foals were used to isolate endothelial cells, followed by morphological and immunohistochemical identification. Performance of the cells in various assays commonly used in angiogenesis research was studied. Additionally, EqUVEC expression of vascular endothelial growth factor (VEGF) was assessed using ELISA. EqUVECs exhibited endothelial characteristics, forming a homogeneous monolayer with distinctive morphology. Immunohistochemical staining confirmed positive expression of key endothelial markers including von Willebrand factor (vWF), CD31, and vascular endothelial growth factor receptor-2 (VEGFR-2). Furthermore, performance assessments in in-vitro assays demonstrated the viability, proliferation, migration, tube formation and VEGF-expression capabilities of EqUVECs. The findings suggest that EqUVECs are a promising in-vitro model for studying equine angiogenesis, offering a foundation for further investigations into equine-specific vascular processes and therapeutic interventions.
1 Introduction
Angiogenesis, the formation of new blood vessels from pre-existing ones, plays a pivotal role in various physiological and pathological conditions (1). The regulation through a balance of pro-and anti-angiogenic factors is essential for maintaining a healthy and well-functioning tissue (2–4). Dysregulation of angiogenesis can lead to numerous diseases in humans, including ocular neovascularization and wound healing disorders, eventually causing tissue and organ dysfunctions (5–7). Pathological vascularization is one of the factors considered responsible for metastasis and cancer development, making it a key area within recent human cancer research (6, 8).
In horses, dysregulated angiogenesis is associated with various conditions, such as laminitis and ocular pathologies, including equine recurrent uveitis (ERU) (9–16). ERU is characterized by recurrent episodes of inflammation within the eye, leading to vision impairment and blindness (17). Abnormal growth of blood vessels within the uveal tract contributes to the progression of this condition, exacerbating tissue damage and inflammation (17). Furthermore, tumors like sarcoids and squamous cell carcinomas in horses May also exhibit aberrant angiogenic processes (12, 18, 19). Additionally, impaired wound healing is linked to dysregulated angiogenesis (9, 15, 16). Inadequate blood supply to the wound site hinders the delivery of essential nutrients and oxygen, delaying the healing process.
Despite the clinical relevance, research about the topic of angiogenesis as well as pro-and anti-angiogenic treatments for horses is relatively limited (7). Considering the various functions of the vascular system, it is vital to understand the molecular mechanisms of angiogenesis to develop therapeutic approaches. In-vitro models provide a valuable tool for investigating angiogenesis by offering controlled environments for studying molecular mechanisms and assessing potential therapeutic interventions and species-specific responses. These models bridge the gap between in-vivo studies and clinical applications (20, 21).
Endothelial cells (ECs) are primarily responsible for physiological and pathological angiogenesis and are thus extensively used in angiogenesis research. Various EC models have been studied, using sources such as pulmonary arteries, digital or jugular veins (22–25). However, the endothelium is highly heterogeneous, with arterial ECs significantly differing from venous ECs in morphological and functional properties (26–28). Notably, results obtained from human umbilical vein endothelial cells (HUVECs) can be extended to other EC types and seem to reflect the behavior of arterial ECs as well (29). Furthermore, umbilical cords are considered medical waste and are therefore easily accessible (29). The HUVEC model, specifically, has proven beneficial in studying both physiological and pathological effects in response to various stimuli and pathways, either in isolation or in co-culture with other cell types (30). In-vitro HUVEC models have found to be useful for studying various aspects of endothelial function, including monocyte adhesion, endothelial damage, and repair (31). The HUVEC model allows for exposure of the cells to shear stress under controlled flow conditions, mimicking in-vivo blood flow (32). Furthermore, this model is used for assessing the impact of novel drugs on human endothelium, contributing to the understanding of diverse biological processes and diseases such as inflammation, cancer, and diabetes mellitus (33–37).
Considering this, EqUVECs, serving as an equivalent to HUVECs, could enhance our understanding of equine angiogenesis. This research May help develop effective therapeutic strategies for pro-and anti-angiogenic treatments in horses. Currently, there is no equine-umbilical-vein-endothelial-cell-line commercially available; thus, we aimed to describe a method for isolating EqUVECs with relatively minimal equipment in order to increase accessibility of EqUVECs for researchers.
2 Materials and equipment
The materials and equipment required for umbilical cord sampling, cell isolation and processing, and immunolabeling are listed in Tables 1–4.
2.1 Animals
Umbilical cords of foals containing two umbilical arteries (UA) and one vein (UV) were harvested and included in the study after delivery (Table 5). Informed owner consent was obtained from all owners at the time of hospitalization of the horses (Mare 1, 2, 4) whose umbilical veins were harvested after placental shedding and involved in the study. The collection of umbilical veins from research animals (Mare 3 and 5) was approved by the competent authority for animal experimentation in Austria (Federal Ministry for Science and Research, license number 2020–0.547.889). The mares and foals included in the study were healthy, the deliveries unassisted or required minor assistance.
3 Methods
3.1 Sampling
After harvesting, umbilical cords were carefully cleaned and rinsed with isotonic saline solution 0.9% (B. Braun, Melsungen, Germany) to eliminate any remaining blood residues. Samples were then transferred to a transport flask containing 500 mL PBS supplemented with antibiotics (10 μg/mL colistin, 10 μg/mL vancomycin, 100 units/mL of penicillin and 100 μg/mL of streptomycin) and stored at 4° until further processing (Table 1). Endothelial cells were isolated within 12 h after harvesting.
3.2 Isolation and cell culture of endothelial cells
The protocol was adapted from a human endothelial vein cell (HUVEC) isolation protocol by Baudin et al. (30). Isolated cells were used in a drug-efficiency experiment (38). All samples were processed in a laminar flow system under sterile conditions. After removal of the connective tissue and umbilical arteries, the umbilical vein was again rinsed with 50 mL PBS to remove the red blood cells until transparent buffer was effluent. One end of the vein was tightly clamped using a sterile surgical clamp and filled with prewarmed (37°C) 0.2% collagenase solution to dissociate ECs. The umbilical vein was incubated in a sterile metal dish with prewarmed PBS in a water bath at 37°C. After incubation for 10 min, the cord was gently squeezed to promote further ECs detachment. Dissociated cells were collected by flushing the vein with 40 mL of PBS. The cell solution was decanted into a 50 mL tube containing 10 mL of culture medium. High glucose basis culture medium (DMEM + GlutaMAX ™, Life Technologies, Thermofisher Science, Carlsbad, California, United States) was supplemented with 20% fetal calf serum (FCS), 15 mM HEPES, 100 units/mL of penicillin, 100 μg/mL of streptomycin, and 0.25 μg/mL of Gibco Amphotericin B (Life Technologies, Thermofisher Science, Carlsbad, California, United States) (Table 2). The cell suspension was centrifuged at 450 G for 10 min at room temperature (RT). The supernatant was discarded, and the pellet was resuspended in the complete culture medium. In order to separate cell aggregates, the solution was repeatedly aspirated and repulsed through a 23 G needle (Sterican, 0.6 × 30 mm, Braun, Melsungen, Germany). Cells were seeded at a density of 4 × 104 cells/cm2 and incubated at 37°C with 5% CO2 concentration and 98% relative humidity. The medium was changed the following day (<24 h) to remove non-endothelial cells with less adhesion capacity. After reaching confluence the monolayer was washed with PBS and trypsinized (1X, Trypsin–EDTA for primary cells, ATCC®, USA). After 2–3 min, complete culture medium was added for inactivation of trypsin and the cells were centrifuged. The pellet was resuspended in the complete culture medium, and subcultures were expanded by passaging before being used in further experiments. Cells of passage three were then cryopreserved until further processing and stored at −150°C. EqUVECs up to passage five were used in the assays. To detect cell culture changes cells up to passage 8 were cultured in 6-well plates (Biologix, Delhi, India) and monitored. Each passage was photographed using a 10x phase contrast magnification on a Leica DMi8 inverted microscope (Leica Microsystems GmbH, Wetzlar, Germany). A list of necessary equipment is shown in Table 3; Step-by-Step isolation procedure and critical steps are outlined in Figure 1.
To compare morphological characteristics of equine and human ECs, HUVECs were purchased from American Type Culture Collection (ATCC®, USA). Cells were grown in Vascular Basal Medium (ATCC®, USA) supplemented with 2% FBS and rhEGF, rhFGF, rhVEGF, rhIGF-1, ascorbic acid, hydrocortisone, heparin and L-glutamine (VEGF Growth kit, ATCC®, USA) in a 37°C and 5% CO2 humidified incubator. Cells were seeded at 5000 cells/cm2 in T-75 flasks. After reaching confluence the monolayer was washed with PBS and trypsinized (1X, Trypsin–EDTA for primary cells, ATCC®, USA) before being used in experiments. HUVECs of passage three to five were used in all experiments.
3.3 EqUVEC identification and characterization
3.3.1 Morphological characterization of EqUVECs
Transmission electron microscopy (TEM) was used to examine the ultrastructure of EqUVECs. Furthermore, morphological characteristics of EqUVECs were compared to HUVECs. After ECs formed a monolayer, all samples were fixed in 3% buffered glutaraldehyde (pH 7.4, Merck) for 10 min and scraped from the cell culture flask afterwards. Cell culture specimens were pre-embedded in Histogel (Epredia Inc., New Hampshire, USA). After being washed in 0.1 M Soerensen buffer (pH 7.4), the cells were postfixed with 1% osmium tetroxide (Electron Microscopy Sciences, Hatfield, PA, USA) for 2 h at RT. Subsequently, the cells were dehydrated and incubated in increasing ratios of epoxy resin-propylene oxide (1,1, 3:1) and finally pure resin before embedding and polymerization in epoxy resin (Serva, Mannheim, Germany) for 48 h at 60°C. Ultrathin sections of 70 nm were cut, contrasted in methanolic uranyl acetate (Fluka Chemie AG, Buchs, Switzerland) and alkaline lead citrate (Merck KG, Darmstadt, Germany) and examined using an EM 900 electron microscope (Zeiss, Oberkochen, Germany). Digital images were captured using an ImageSP Professional software (SYSPROG, TRS, Moorenweis, Germany).
3.3.2 Identification of EqUVECs using immunohistochemical staining
To confirm the collection of endothelial cells, samples from a representative vessel (positive control) as well as cultured cells were prepared for antibody staining with endothelial markers von Willebrand Factor (vWF), CD31 and vascular endothelial growth factor receptor-2 (VEGFR-2). Endothelial cells were fixed within an 8-well chamber slide (Ibidi, Munich, Germany) in 4% formaldehyde for 10 min. After this the cells were washed twice with PBS for 2 min and dried at room temperature for 15 min and stored at 4°C until further processing. Endogenous peroxidase activity was blocked by incubating the slides in 3% H2O2 in methanol for 15 min. After rinsing, antigen retrieval was performed by heating tissue sections in Tris EDTA buffer at pH 9.0 in a steamer for 45 min. After cooling down for 15 min, a protein block was conducted to prevent non-specific protein binding by incubation with 1.5% goat serum (DAKO, Glostrup, Denmark) in PBS for 30 min. Slides were then incubated with primary antibodies (anti-VEGFR-2, anti-CD31 and anti-vWF) overnight at 4°C in a humidity chamber. To test the specificity of the secondary antibody, a negative control with PBS instead of the primary antibody was used for each staining. Following overnight incubation, sections were rinsed with PBS solution (pH 7.4) and incubated with secondary antibodies (Poly-HRP anti-mouse for VEGFR-2 and Poly-HRP anti-rabbit for CD31 and vWF, all from ImmunoLogic, Duiven, The Netherlands) for 30 min at room temperature in a humidity chamber. After a PBS wash step, immunostaining was visualized using 3,3’ Diaminobenzidine (DAB, Quanto, Richard Allan Scientific, TA-125-QHDX) for 5 min at RT, followed by washing in distilled water and counterstaining with Mayer’s Haemalaun for 3 min before a final rinse in tap water for 10 min. Chambers were assessed independently under light microscopy using a Polyvar microscope (Reichert-Jung, Vienna, Austria). Digital images were captured using a Nikon Ds-Fi1 camera and NIS-Element software (Nikon, Melville, NY, United States). Supplements are listed in Table 4.
3.4 Examples of application: performance in different angiogenesis assays
3.4.1 EqUVEC cell viability
The viability of isolated EqUVECs was assessed by measuring their capacity to reduce a substrate, indicative of cellular metabolism. Cell viability was determined using the RealTime-Glo™ MT Cell Viability Assay (Promega Corporation, Fitchburg, WI, United States) according to the manufacturer’s protocol at a 24 h-interval for 96 h. Cells were inoculated at 1.6 × 104 cells/well onto an opaque-walled 96-well plate (Falcon; Becton Dickinson Labware, Plymouth, England). Upon reaching confluence, RealTime-Glo™, MT Cell Viability Substrate and NanoLuc® Enzyme were added. Following a 1-h incubation at 37°C in a 5% CO2 humidified chamber, luminescence was quantified using the GloMax® Explorer Multimode Microplate Reader (Promega Corporation, Fitchburg, WI, United States). Each experiment was conducted in triplicate.
3.4.2 EqUVEC cell proliferation
To assess viable cells in proliferation an MTS-assay (3-(4,5-dimethylthiazol-2-yl)-5-(3-carboxymethoxyphenyl)-2-(4-sulfophenyl)-2H-tetrazolium) was performed using the CellTiter 96® AQueous One Solution Cell Proliferation Assay (Promega Corporation, Fitchburg, WI, United States). Briefly, cells were plated (1.6 × 104 cells/well) on 96-well plates in 100 μL/well culture medium with 20 μL/well of CellTiter 96AQueous One Solution (MTS) solution (Promega Corporation, Fitchburg, WI, USA). After incubation at 37°C in a 5% CO2 humidified chamber for 1 h, absorbance was measured at 490 nm using the GloMax® Explorer Multimode Microplate Reader (Promega Corporation, Fitchburg, WI, USA). Experiments were carried out in triplicates.
3.4.3 EqUVEC cell migration
EqUVECs (1 × 105 cells/well) were cultured onto a 12-well plate. Upon confluency, a scratch was introduced with a sterile 1,000 μL tip. After removing cell debris by washing with PBS, the scratch filling was documented using a Leica DMi8 inverted microscope (Leica Microsystems GmbH, Wetzlar, Germany) with fixed X and Y positions (4x magnification) for at least three positions/well at defined intervals (0 h, 24 h, 36 h, 48 h, 56 h, and 72 h). Wounded area was analyzed using the Wound Healing Size Tool plugin for ImageJ/Fiji® (39). Experiments were carried out in triplicates.
3.4.4 EqUVEC tube formation
In order to investigate the ability of the cells to form capillary-like structures, tube formation assays were carried out. Ibidi μ-angiogenesis slide (Ibidi, Munich, Germany) were coated with 10 μL Matrigel (Thermofisher Science, Carlsbad, California, United States). After polymerization at 37°C for 30 min, the cells were harvested and 1 × 104, 1.5 × 104 and 2 × 104 EqUVECs were seeded. Angiogenesis slides were allowed to incubate at 37°C in 5% CO2 humidified ambient oxygen conditions for 30 min. Digital images of the whole wells were captured using a 10x phase contrast magnification on a Leica DMi8 inverted microscope (Leica Microsystems GmbH, Wetzlar, Germany) at 1–3 h-intervals for up to 14 h. Using the Angiogenesis Analyzer plugin for ImageJ/Fiji® number of tubes, number of junctions, and total length of branches and segments were quantified (40).
3.4.5 ELISA for EqUVEC VEGF expression
EqUVECs (3 × 104/well) were cultured onto a 48-well plate. Cell culture supernatants were collected at 24 h-intervals (0 h, 24 h, 48 h, and 72 h) and analyzed for VEGF concentrations using a commercially available, species-specific ELISA kit (Equine VEGF-A ELISA Kit, Invitrogen, Waltham, Massachusetts, United States) according to the manufacturer’s protocol. Standards and samples were added to wells and incubated for 2.5 h at room temperature, followed by an incubation with biotinylated equine VEGF-A for 1 h at room temperature whilst being gently shaken. Streptavidin HRP (1:200) was added to each well and peroxidase activity was determined by incubation with 100 μL peroxidase substrate solution 3,3′,5,5′-tetramethylbenzidine (TMB). Color development was stopped after 30 min and absorbance at 450 nm was quantified using a microplate reader (Promega Corporation, Fitchburg, WI, United States). The standard curve was established by serial dilutions of VEGF-A with a linear range between 1.95 pg./mL and 62.5 pg./mL. Experiments were carried out in triplicates.
4 Results
4.1 Isolation procedure and culture
The cells were successfully isolated and cultured in all specimens. Umbilical cords could be sampled quickly and inexpensively and without the need for horses to be euthanized as this is a by-product of natural deliveries. Furthermore, sampling of the cords could be roughly planned, as the mares were hospitalized for birth monitoring. Isolation was performed in one vein per horse with approx. Ten–20 cm length without pooling. Per vein 2.78–7.25 × 106 cells with a viability of 70–82.3% could be isolated (NucleoCounter®NC-250, ChemoMetec, Allerod, Denmark). EqUVECs formed a homogeneous monolayer in approximately 1 week, whereas HUVECs showed confluency within 3–5 days. As the primary culture grew and expanded, human and equine cells acquired their typical endothelial shape with long and polygonal cells with a prominent oval nucleus in the cell center. Over passages 3 to 8, EqUVECs exhibited slight morphological changes. Initially, at passage 3, the cells displayed a uniform cobblestone-like appearance typical of endothelial cells. However, with each subsequent passage, cells became increasingly elongated and heterogeneous in shape. By passage 8, the cells showed a more spindle-shaped appearance, indicating potential senescence or phenotypic shift due to extended passaging (Figure 2).
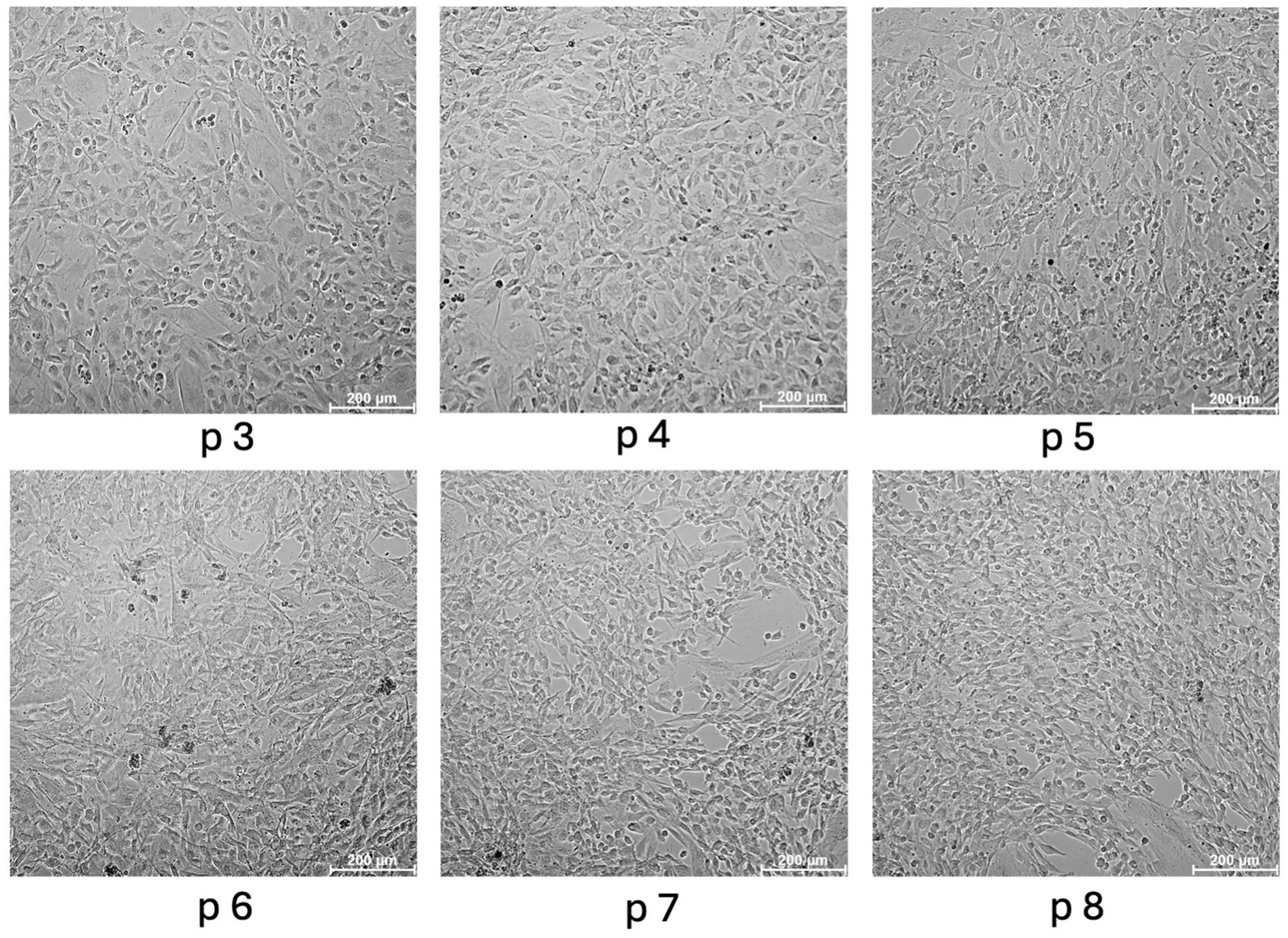
Figure 2. Morphology of equine umbilical vein endothelial cells (EqUVECs). EqUVECs of passage (p) 3 were cultured and after reaching confluency expanded by passaging. Cells were monitored up to passage 8 and photographed by phase contrast microscopy. Scale bars represent 200 μm.
Care must be taken to discard umbilical cords from diseased horses as well as damaged cords. In preliminary experiments one sample was obtained from a foal of a 5-year-old Noriker mare which suffered from inflammatory processes prior to foaling (placentitis). The isolated cells demonstrated the poorest proliferation and survival rate compared to primary cultures of healthy foals, taking 2–3 times longer for monolayer formation. In this period, a significant number of cells perished, especially within the initial days. However, once a monolayer was successfully established and after the subsequent expansion by passaging, their performance in diverse assays (viability, proliferation and migration assay) closely resembled that of cell lines derived from healthy animals (data not shown).
4.2 EqUVEC identification and characterization
4.2.1 Morphological characterization of EqUVECs using TEM
EqUVECs observed by TEM were homogeneous cells with prominent nuclei rich of euchromatin (Figure 3). EqUVECs rarely contained oval rod-shaped bodies, described previously by Weibel and Palade (WPB) (41, 42), whereas they could be found regularly in the cytoplasm of HUVECs (Figure 4A). In EqUVECs a basement membrane could only be found in some areas, but the cells showed apical-basal polarity with caveolae appearing mainly on the apical cell pole and adherence structures at the basal domain (Figure 4B). The perinuclear region was found to be rich in rough endoplasmic reticulum, mitochondria with tubular shapes, Golgi complexes, pinocytic vesicles and clusters of free ribosomes in human and equine cells (Figures 3, 4C). Furthermore, the oval nuclei presented with a fine granular pattern abundant of euchromatin with prominent nucleoli and enveloped with clearly visible bilayer membranes (nuclear membrane) (Figure 3). TEM observations revealed that cell–cell contacts were created by spot-like adhesions of desmosomes present in overlapping areas of neighboring cells in both cell types. Equine cells formed adhesion “buds” at the basal domain (most likely hemidesmosomes) supported by cytoskeletal elements, which could not be found in HUVECs (Figure 4D). Bundles of cytoskeletal filaments were located throughout the cytoplasm and within the complex interdigitation (EqUVEC) in the peripheral cytoplasm (Figure 3).
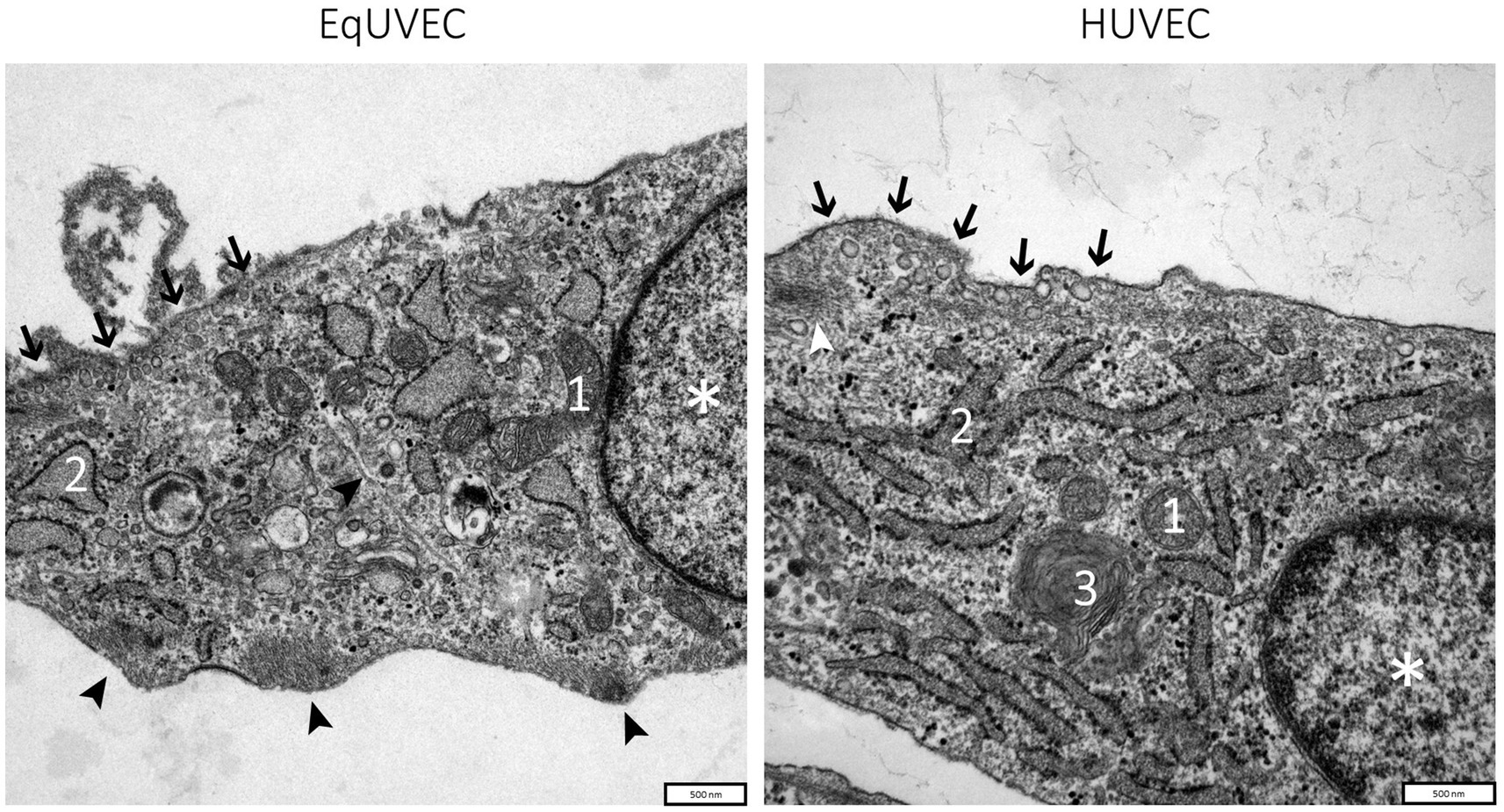
Figure 3. TEM images of equine and human umbilical vein endothelial cells. Overview on cell morphology with nuclei indicated by (*), caveolae on the apical cell domain (➔), cytoplasm with cell organelles like mitochondria (1), rough endoplasmic reticulum with numerous ribosomes on cisternae surface (2), Golgi complexes (3), and agglomerations of the cytoskeletal filaments at adhesion “buds” (➤). Scale bar is 500 nm.
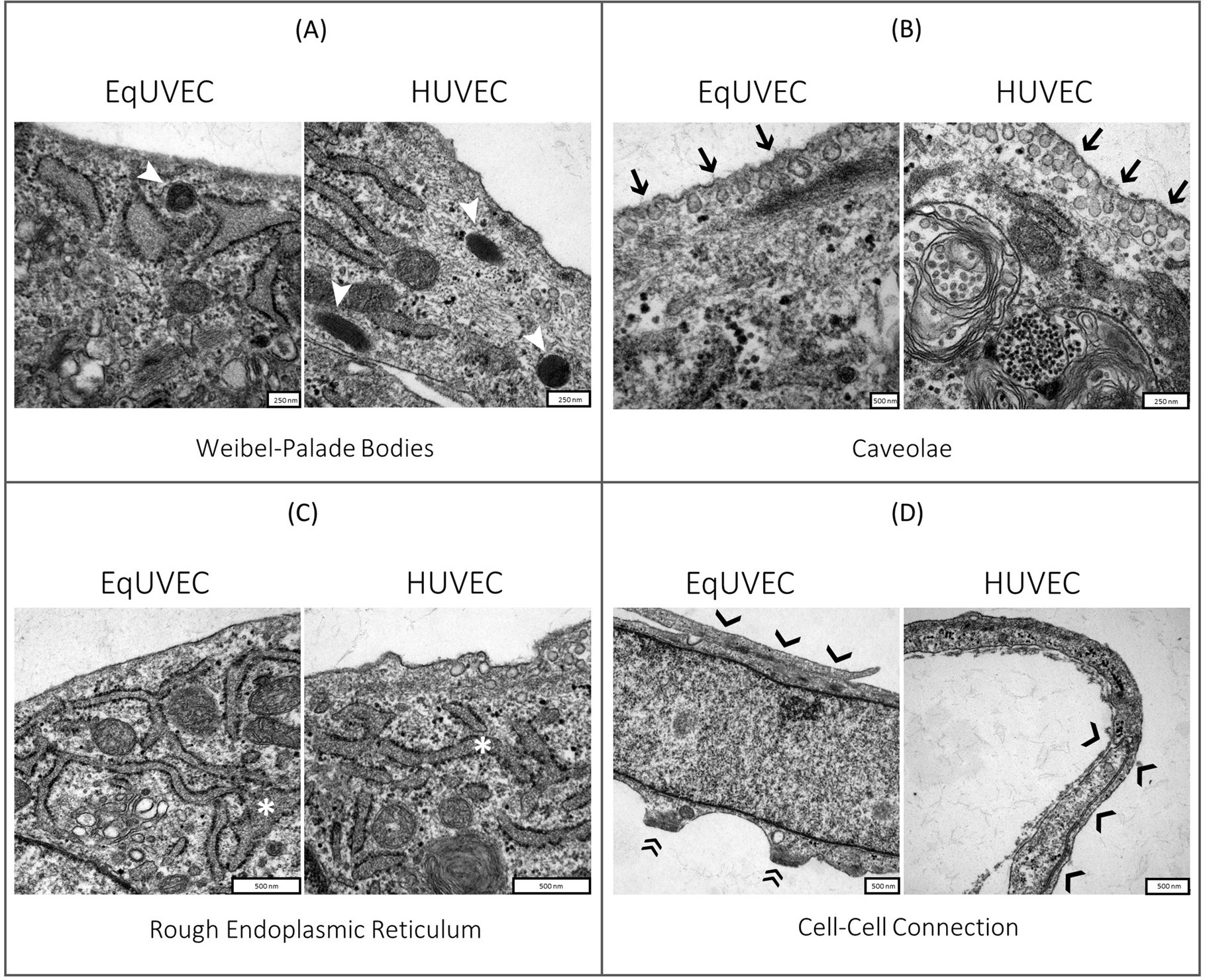
Figure 4. TEM images of equine and human umbilical vein endothelial cells. Close-up of cell morphology with focus on (A) Weibel-Palade bodies (➤), (B) caveolae on the apical cell domain (➔), (C) rough endoplasmic reticulum (*) and (D) cell–cell connections formed by desmosomes (❯) and adhesion “buds” (EqUVEC) (»). Scale bar is between 250 and 500 nm.
4.2.2 Identification of EqUVECs using Immunohistochemical staining
Endothelial cells were identified by immunohistochemical staining of vWF, CD-31 and VEGFR-2. Cells showed an intense staining for vWF predominantly localized within the cytoplasm. A heterogeneous staining pattern, with areas showing more concentrated staining, likely reflecting the intracellular processing of vWF, was detected. Cultivated cells stained moderately intense for CD31 and were primarily localized on the cell membrane. The VEGFR2 staining displayed moderate intensity, with varying degrees of staining intensity across different cells mainly localized on the cell membrane but also showing presence within the cytoplasm (Figure 5). Negative controls are shown in Supplementary Figure S1.
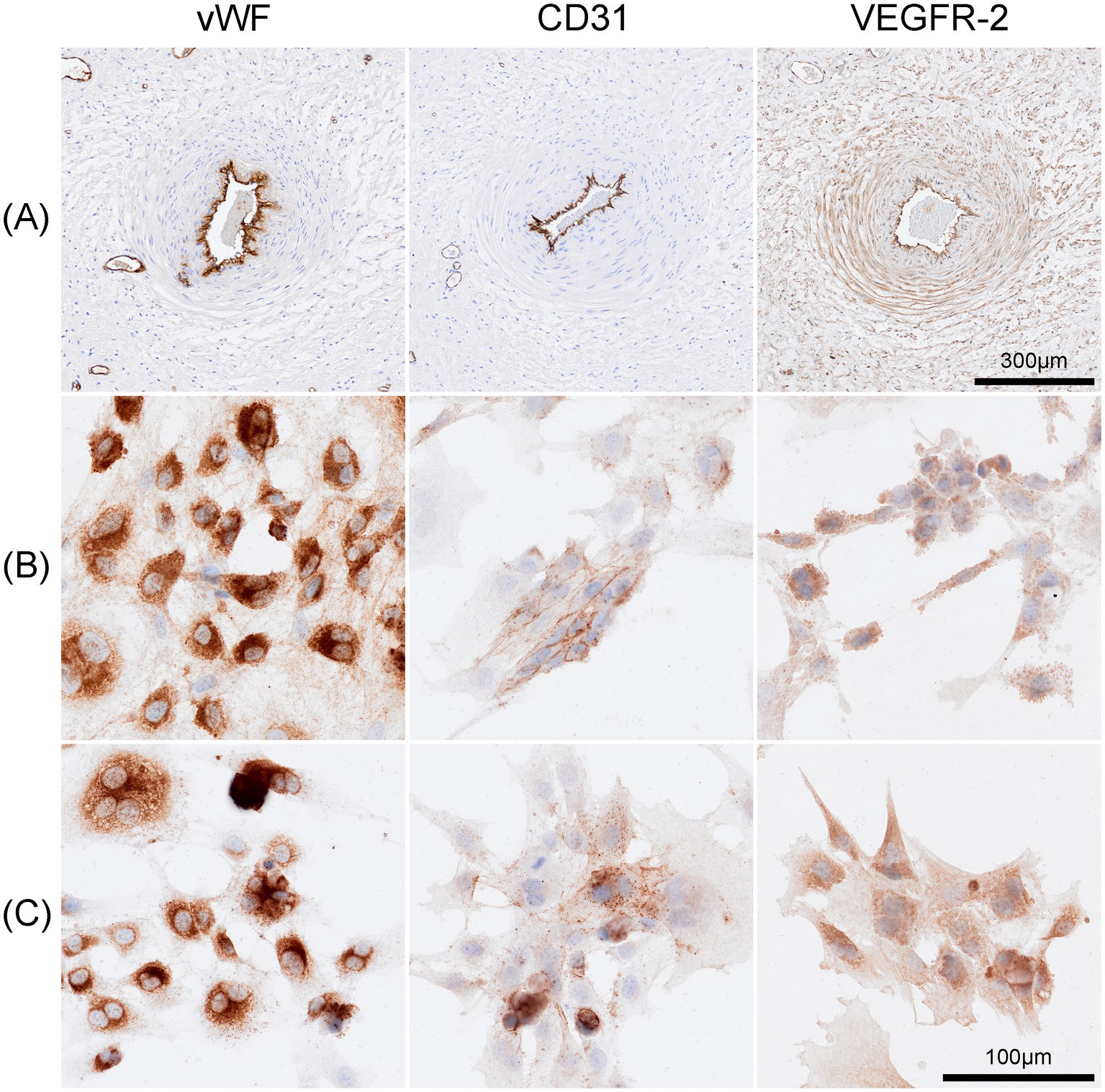
Figure 5. Immunohistochemical staining of Equine umbilical veins and cultured cells. (A) Endothelial cells lining the umbilical vein stained strongly positive for anti-von Willebrand factor (vWF), anti-CD-31 and anti-VEGFR-2. Similarly, cells of passage 4 (B), passage7 (C) exhibited strong positive staining for these markers. The vWF staining was intense and predominantly cytoplasmic, CD31 expression was primarily localized to the cell membrane, and VEGFR2 showed moderate staining with a heterogeneous pattern observed on both the cell membrane and within the cytoplasm. Scale bar is 100 μm.
4.3 Examples of application: performance in different angiogenesis assays
The cell lines were subjected to various assays associated with angiogenesis and their performance was investigated.
4.3.1 EqUVEC cell viability and proliferation
When analyzed over a 72 h culture period no decrease in relative cell viability was observed throughout the time course (n = 20, 24 h = 1.62, 48 h = 2.20, 72 h = 2.05). However, studied cell lines showed an increase in dispersion over the time course with standard deviations of 0.28 (CI: 1.50–1.74) at 24 h, 0.63 (CI: 1.92–2.47) at 48 h and 0.82 (CI: 2.19–3.35) at 72 h (Figure 6A). To determine viable cells in proliferation an end-point assay was carried out. Measured absorbance of the cell lines was 0.92 ± 0.16 (CI: 0.77–0.99) after 72 h.
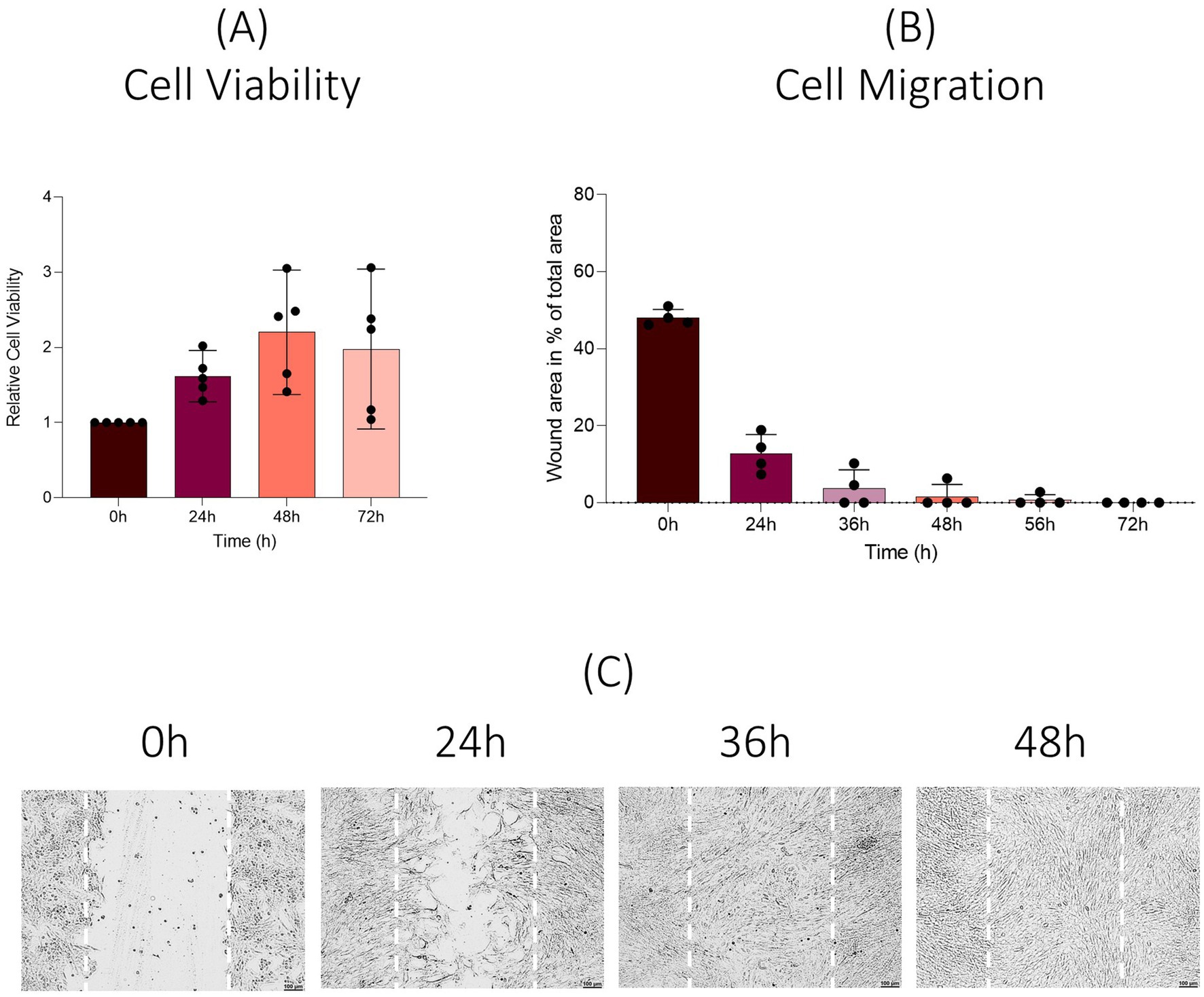
Figure 6. Viability and migration assay. Results are shown as means (n = 20). (A) Change in cell viability compared to baseline values over a 72 h period (real-time assay). (B) Results are shown as means (n = 12). Percentage of wounded area at 0 h, 24 h, 36 h, 48 h, 56 h, and 72 h post scratch is shown. (C) Representative images after scratch wound.
4.3.2 EqUVEC cell migration
EqUVEC cell migration was assessed by observing their capacity of scratch wound closure. Cells were monitored over 72 h (0 h, 24 h, 36 h, 48 h, 56 h, and 72 h). The migrated cells reduced the wounded area from 48.33% ± 2.89 (n = 12; CI: 46.69–49.96) to 12.40% ± 5.27 (n = 12, CI: 9.42–15.38) after 24 h and 3.69% ± 4.56 (n = 12, CI: 1.11–6.27) after 36 h. After 48 h and 56 h the cell lines showed a further decrease to 1.57% ± 3.02 (n = 12, CI: 0–3.28) and 0.69% ± 1.64 (n = 12, CI: 0–1.62), respectively. The gap was refilled within 72 h in all cell lines. Cell migration resulted in a confluent monolayer in 50% of the cells within 36 h, in 75% within 48 h and in 83% within 56 h after wound initiation (Figure 6B). Representative images are shown in Figure 6C; images of all time points and cell lines are shown in Supplementary Figure S2.
4.3.3 EqUVEC tube formation
Descriptive tube formation assays were carried out in order to assess optimal seeding densities for μ-slide angiogenesis assays. Observing a 14 h culture period, EqUVECs formed capillary-like structures when seeded in densities as high as 1 × 104 cells and 1.5 × 104 cells per well, although more pronounced when seeded with 1.5 × 104 cells/well. Higher seeding densities (2 × 104 cells/well) resulted in clumping and cell death. When analyzed (1.5 × 104 cells/well), this resulted in an increase of tubes from 28 (0 h) to 102 (14 h) tubes (+7.63 tubes/h). Similarly, junctions increased from 170 (0 h) to 452 (14 h) junctions (+25.68 junctions/h) and total length of segments and branches from 39,537 (0 h) to 49,888 (14 h) (+855.11 increase in total length/h). Representative images after 2 h, 7 h and 14 h of incubation are shown in Figure 7.
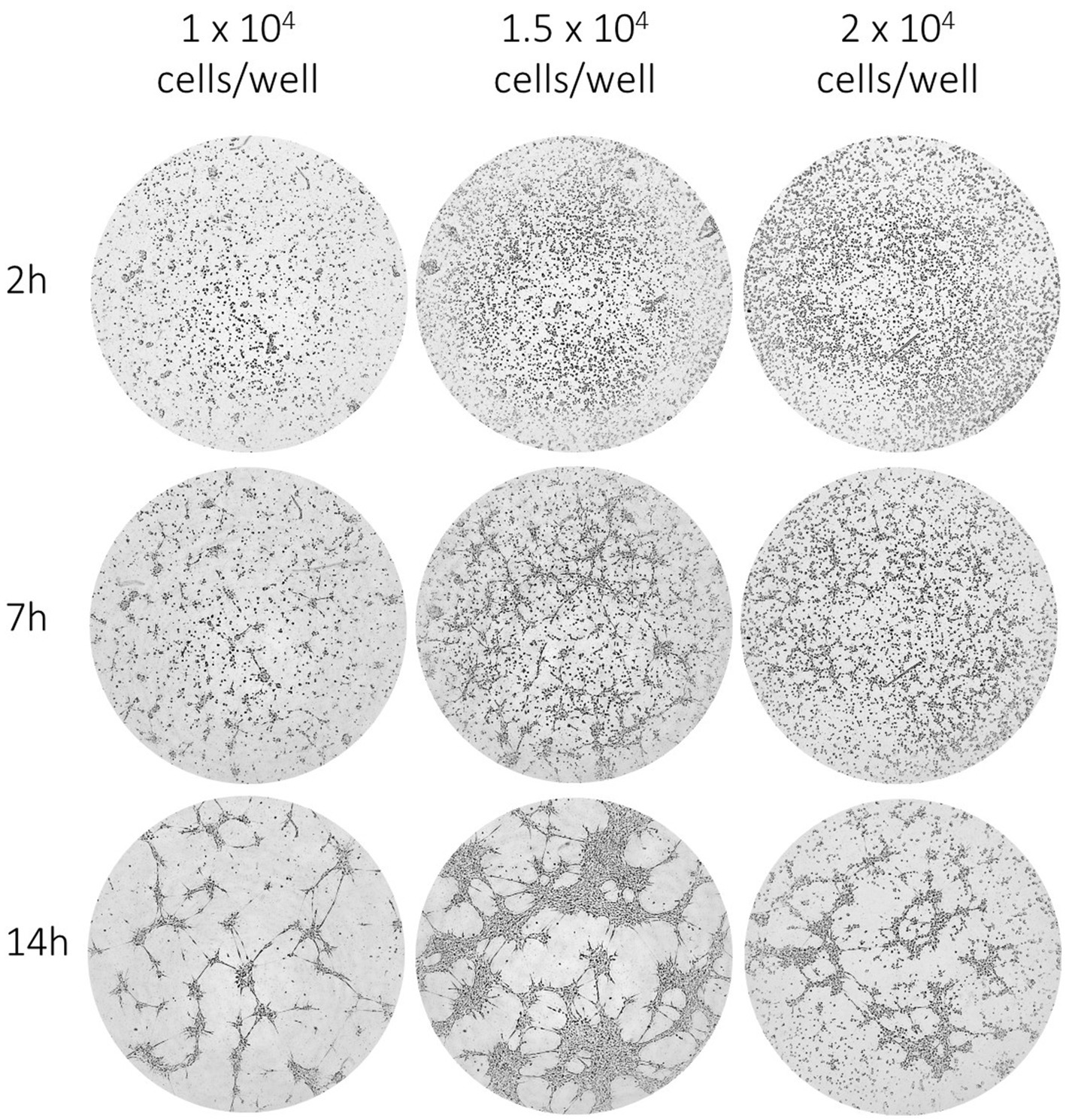
Figure 7. EqUVEC tubular network formation in in vitro angiogenesis assay. EqUVEC cells seeded with 1 × 104, 1.5 × 104, and 2 × 104 cells/well growing on Matrigel overnight. Representative images of EqUVECs after 0 h, 7 h, and 14 h of incubation.
4.3.4 ELISA for EqUVEC VEGF expression
The VEGF-levels in cell culture supernatants were investigated. A standard curve was established using VEGF-A concentrations between 1.95 pg./mL and 62.5 pg./mL. The detected range resulted in a linear relationship (Figure 8A). An increase of VEGF expression was observed over 72 h at all time points. EqUVECs showed VEGF levels of 7.00 pg./mL ± 0.13 (n = 3, CI: 6.86–7.15) when seeded. After 24 h and 48 h levels increased to 7.81 pg./mL ± 0.09 (n = 3, CI: 7.71–7.91) and 8.34 pg./mL ± 0.11 (n = 3, CI: 8.22–8.46). Highest VEGF-level was measured with 9.32 pg./mL ± 0.26 (n = 3, CI: 9.03–9.61) after 72 h (Figure 8B).
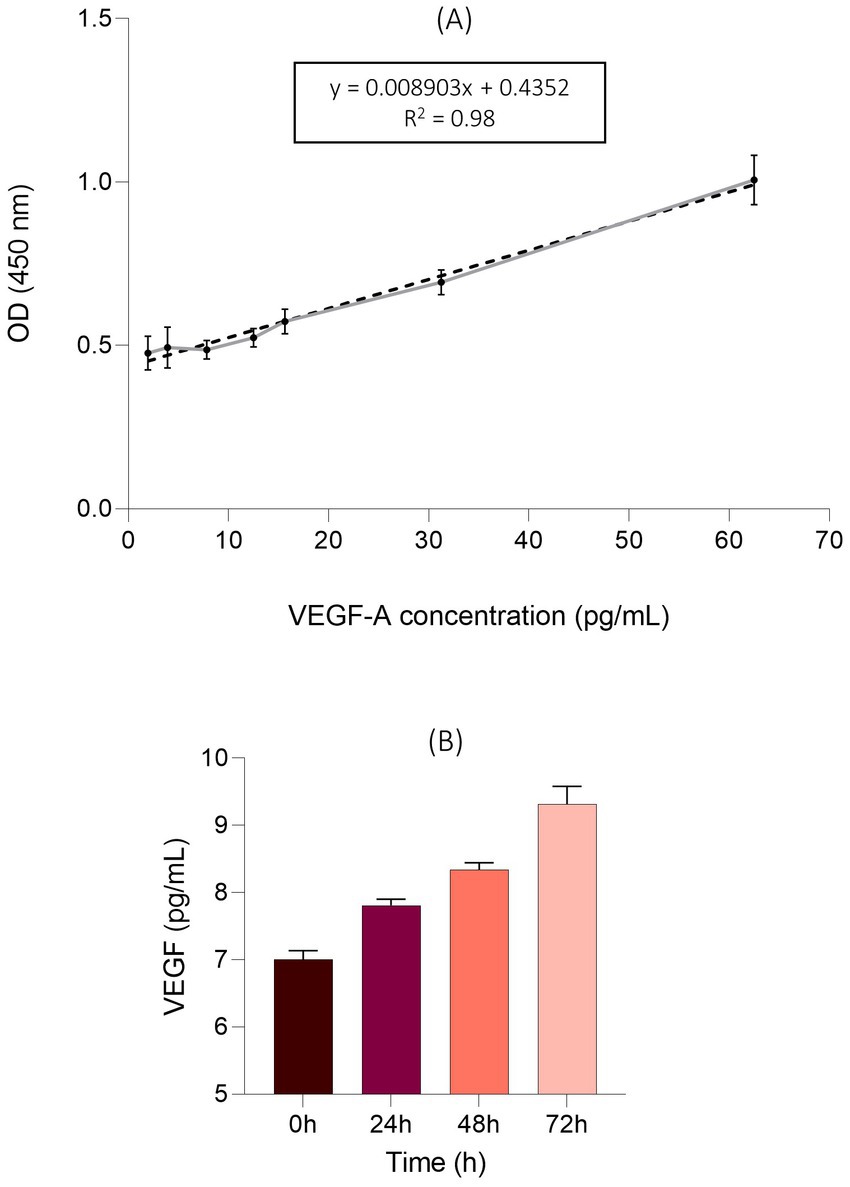
Figure 8. VEGF levels in the supernatants of the EqUVEC cell culture. (A) An ELISA standard curve was established to detect 1.95 pg./mL to 62.5 pg./mL of VEGF-A as a reference. The standard curve was generated (n = 3) and data points are presented as means with 95% CI. The dotted line rep-resents the best fit determined by linear curve fitting (r2 = 0.98). OD = Optical Density. (B) Absolute VEGF expression after 0 h, 24 h, 48 h, and 72 h of incubation. Results are shown as means with 95% CI (n = 3).
5 Discussion
This study presents a simple protocol for the isolation and culture of equine umbilical vein endothelial cells (EqUVECs), providing a method suitable for application in laboratories with standard equipment. EqUVECs were isolated from the umbilical vein, cultured in-vitro, characterized morphologically and immunohistochemically, and evaluated for their performance in various in-vitro assays. Documentation on the isolation procedure and utilization of equine ECs from different sources is scarce, with sporadic reports lacking detailed descriptions of the isolation procedures on ECs derived from equine umbilical veins (22–25, 43–51). This protocol was extrapolated from the original description by Baudin et al. (30) for HUVECs.
While developing an isolation protocol it is important to impede culture contamination with foreign cells such as fibrocytes or muscle cells. To promote the selective growth of ECs, non-adherent cells are regularly flushed away after seeding. To further accelerate adhesion of ECs to the plate compared to non-ECs, pre-coated plates could be used (52). While EqUVECs in this study were investigated on uncoated plates without notable contamination, the possible benefits of using matrices like gelatine or fibronectin demand further investigation (52–55).
Choosing an optimal medium is another critical aspect of establishing an endothelial cell culture. Fetal calf serum (FCS) was used as the primary medium supplement due to its high content of adhesion proteins, immunoglobulins, transcription factors, nutrients, and growth factors, among other vital components (56). Fetal horse serum (FHS) is also suitable for equine endothelial cell cultures and specific applications May benefit from species-specific serums, as evidenced in the cultivation of porcine intestinal cells (48, 57). However, data provided by Dietze et al. (22) indicate that FHS did not increase equine EC proliferation. The possible superiority of FHS for EqUVEC culture was not assessed and needs further investigations (22).
When culturing ECs, it is recommended to use specific culture media containing not only amino acids, vitamins, carbohydrates and salts, but also a variety of growth factors in addition to those found in fetal bovine sera (35). This approach is crucial for the optimal growth and maintenance of ECs derived from various species, including humans, rhesus macaques, and ovines (58–60). Commonly used growth factors known for their high mitogenic, and selective properties include fibroblast growth factors (FGFs), platelet-derived endothelial growth factors (PD-EGF) and vascular endothelial growth factors (VEGF) (16, 61, 62). However, this study opted to not use supplementary growth factors, considering their potential influence on protein synthesis, intracellular exchange and impact as a compensatory factor (63–66). Despite not supplementing the media, we were able to successfully isolate and cultivate EqUVECs demonstrating the adaptability of the cells to grow and proliferate in basic culture conditions. Several published protocols described the successful isolation and characterization of endothelial cells from umbilical cords of species such as bovines and swine using non-supplemented media (67, 68). These studies highlight that endothelial cells from these species can be effectively cultured and maintained even without additional growth factors.
Cultures needed to be checked for contaminations, such as bacteria, fungi and foreign cells, regularly. Most bacterial and fungal contaminations can be identified by light microscopy; however, mycoplasma remains usually undetected. Therefore, all cell cultures underwent mycoplasma DNA detection using PCR prior to conducting further experiments. To prevent contamination of foreign cells, non-adherent cells were regularly flushed away after seeding. Most markers used to identify foreign cells, such as fibroblasts and smooth muscle cells, are also expressed in endothelial cells, making them insufficient for foreign cell identification (69). However, these cells display characteristic features, such as fibroblasts often exhibiting a spindle-shaped morphology and forming a characteristic mesh-like pattern, while smooth muscle cells are typically elongated and exhibit a “hill and valley” growth pattern. These distinct features aid in the identification of foreign cells in cultures (70, 71).
Identification of endothelial cells depends on various factors, including cell morphology, the presence of marker organelles and immunolabeling with protein markers. In this study, EqUVECs exhibited characteristics similar to those described for HUVECs, although Weibel-Palade bodies (WPBs) were rarely observed. Immunohistochemical staining confirmed the presence of cell markers, including vWF, CD31, and VEGFR-2, supporting the endothelial character of the cells. The cellular identity of ECs can be ascertained through cell morphology, the presence of endothelial marker organelles and immunolabeling with specific markers (21). In this study, EqUVECs exhibited characteristics similar to those of HUVECs initially characterized by Jaffe in 1973, although Weibel-Palade bodies (WPBs) were rarely observed (72). Weibel-Palade bodies are oval-or rod-shaped secretory organelles specifically found in ECs of numerous species and found to release von Willebrand factor (vWF) (11, 21, 73–77). The discrepancy in observing Weibel-Palade bodies could be explained by an overall difficulty in locating these organelles, as well as a low observation frequency described by Jaffe (72).
While ECs exhibit numerous morphological characteristics, relying solely on microscopic examination is insufficient for their identification. Endothelial cells can be identified by immunohistochemical staining; however, due to their diversity, there is no universal marker (21). Despite forming a single-layered lining in blood vessels and the heart, ECs display notable variations in function, morphology, and antigen expression (78). Different cell markers can be identified in the cell’s cytoplasm and membrane. In horses, among other species, the von-Willebrand-factor is seen as a specific endothelial marker (79, 80). In this study, positive vWF labelling affirmed the endothelial-specific character of the cells. To ensure consistency, cells up to passage 5 were used in the experiments, since fading or loss of vWF labelling has been reported in advanced cell culture passages of other endothelial cultures, including equine ECs (81). This decline is attributed to an aging mechanism coupled with spontaneous apoptosis, as evidenced by decreased angiotensin I-converting enzyme (ACE) and prostacyclin synthesis in advanced cell culture passages (82–85).
Furthermore, EqUVEC stained positive for CD31 and VEGFR-2. CD31, a glycoprotein belonging to the immunoglobulin (Ig) superfamily, serving as a universal endothelial marker situated in the EC membrane near the intercellular junction (86). While VEGFR-2 is being highly expressed by endothelial cells, it is also present in various other cell types such as luminal and glandular epithelium of the endometrium, trophoblasts and the fetal epithelium (87, 88). Despite not being a specific endothelial marker, investigating VEGFR-2 expression in EqUVECs is crucial given its potential as an in-vitro model for angiogenesis in horses. Interactions between VEGF-A and its receptors (VEGFR 1, 2, and 3) are pivotal in angiogenesis. In horses, numerous physiological and pathological conditions are influenced by the VEGF-VEGFR axis, including embryonic vascular development, wound healing, chronic inflammatory diseases and ocular neovascular diseases (9, 11, 89, 90). Additionally, the VEGF–VEGFR-2 signaling pathway is extensively studied and holds significant importance as a promoter of pathological vascularization in tumor development and progression (91). To assess VEGF-A expression in EqUVECs, an ELISA approach was chosen to measure VEGF levels in the cell culture supernatants. An increase in VEGF levels by approximately 33% was observed, with the highest VEGF concentration reaching 9.32 pg./mL ± 0.26 after 72 h. Sadick et al. (33) reported a 64% increase in VEGF expression over 72 h, with the highest VEGF level reaching 41.54 mg/mL in humans. This disparity suggests limited comparability of human and equine VEGF expression in endothelial cells, underscoring the necessity for further investigations. In a previous study, we demonstrated, that vascular endothelial growth factor inhibitors efficiently inhibit VEGF-associated cellular processes and lead to a decrease in VEGF expression (38). Future investigations should focus on evaluating the specific effects of VEGF on these cells. Stimulating cell cultures with growth factors could provide deeper insights into signal pathways and cellular mechanisms involved in these processes.
In-vitro models, while being unable to replicate all aspects of physiological angiogenesis, can successfully simulate in-vivo conditions, although certain limitations, like cellular heterogeneity and disparities in growth rates between in vitro and in vivo settings have to be considered (39). Standardized preparations, media, and cell culture passages are essential to address cellular heterogeneity. Gender differences, including variations in cell proliferation, migration and protein expression, as observed in HUVECs, should also be taken into account (92). The current study investigated umbilical cords of three male and two female foals, which showed generally homogeneous performance. However, due to the small sample size, robust conclusion cannot be drawn, and future research should investigate potential sex-based differences.
Additionally, conducting a set of assays targeting distinct stages of angiogenesis is crucial for obtaining comprehensive insights into the process (3). This study assessed the performance of EqUVECs in viability, proliferation, migration and tube formation assays. Metabolic assays, readily accessible and requiring minimal handling and equipment, were used to investigate cell proliferation. The most well-known assay is the MTT assay (3-(4,5-dimethylthiazol-2-yl)-2,5-diphenyltetrazolium bromide), which induces a color change upon reduction of the tetrazolium compound by metabolically active cells. Furthermore, proliferation and viability assays serve as valuable tools for chemo-sensitivity and cytotoxicity testing of drugs (93, 94). EC migration is a crucial aspect of angiogenesis, representing an early stage in the angiogenic cascade (95–97). It is characterized by autonomous cell motility and collective migration with groups of cells coordinating movements towards a chemotactic gradient, thus establishing a hierarchical structure with leader and follower cells (98). Investigating the molecular mechanisms behind EC migration is essential for a comprehensive understanding and future therapeutic intervention, like inhibition of angiogenesis in tumors or stimulation of vessel formation during wound healing (3). One of the basic tools for cell migration assessment is the cell culture wound closure assay. Various closure times have been observed in equine ECs derived from different vessels, spanning from 15 h to 40 h (78). This emphasizes the importance of determining the migration rate in ECs derived from umbilical veins and cultured in different settings. EqUVECs reformed a monolayer within an average of 47 h with consistently similar closure times observed across different cell lines. Interestingly, achieving a uniform scratch proved to be considerably more challenging in equine cells compared to HUVECs. This finding was also noted by Rieger et al. (78) in equine ECs obtained from jugular veins, despite being cultured under varied conditions. The tube-formation-assay is another powerful tool reflecting the coordination of various cellular processes such as proliferation, migration and apoptosis (69). Care must be taken when interpreting the tube formation assay, due to the use of a single replicate. However, when seeded at a density of 1.5 × 104 cells/well EqUVECs formed capillary-like structures within 14 h.
Viability-, proliferation-, migration-and tube-formation-assays were found to be applicable in EqUVECs. Future studies should include more advanced angiogenesis assays, such as 3D spheres, to evaluate processes like VEGF-dependent sprout and lumen formation in extracellular matrices. These approaches would provide deeper insights into equine angiogenesis.
6 Conclusion
In conclusion, this study aimed to address the need for suitable in-vitro models for angiogenesis in equine research by isolating and characterizing equine umbilical vein endothelial cells (EqUVECs). The results demonstrate that EqUVECs can serve as a valuable model for studying endothelial function and angiogenesis, similar to the well-established human umbilical vein endothelial cell (HUVEC) model. This achievement highlights the potential of EqUVECs for investigating angiogenesis-related processes and therapeutic interventions in horses. Nevertheless, future studies should aim to investigate specific properties and behaviors of EqUVECs in experimental setups.
Data availability statement
The original contributions presented in the study are included in the article/Supplementary material, further inquiries can be directed to the corresponding author.
Ethics statement
Ethical approval was not required for the studies on humans in accordance with the local legislation and institutional requirements because only commercially available established cell lines were used. The animal studies were approved by Federal Ministry for Science and Research, license number 2020–92 0.547.889. The studies were conducted in accordance with the local legislation and institutional requirements. Written informed consent was obtained from the owners for the participation of their animals in this study.
Author contributions
UL: Conceptualization, Data curation, Formal analysis, Investigation, Methodology, Project administration, Validation, Visualization, Writing – original draft, Writing – review & editing. MM: Data curation, Resources, Writing – review & editing. IW: Data curation, Formal analysis, Resources, Validation, Visualization, Writing – review & editing. SK: Data curation, Formal analysis, Visualization, Writing – review & editing. BN: Resources, Supervision, Writing – review & editing. WT: Data curation, Formal analysis, Visualization, Writing – review & editing. BP: Conceptualization, Data curation, Methodology, Resources, Software, Supervision, Validation, Writing – review & editing.
Funding
The author(s) declare that no financial support was received for the research, authorship, and/or publication of this article.
Acknowledgments
The authors want to thank Georg Csukovich and Katrin Spirk of the Department for Small Animals and Horses, University of Veterinary Medicine Vienna for their generously provided knowledge and for their technical support. Additionally, many thanks to Prof. Christine Aurich (gynecology) and Prof. Iwan Burgener (internal medicine) of the Department for Small Animals and Horses, and University of Veterinary Medicine Vienna for providing necessary resources to carry out this study. Additionally, many thanks to Pia Semp and Lee MacDougall for patiently revising the manuscript and language editing.
Conflict of interest
The authors declare that the research was conducted in the absence of any commercial or financial relationships that could be construed as a potential conflict of interest.
Publisher’s note
All claims expressed in this article are solely those of the authors and do not necessarily represent those of their affiliated organizations, or those of the publisher, the editors and the reviewers. Any product that may be evaluated in this article, or claim that may be made by its manufacturer, is not guaranteed or endorsed by the publisher.
Supplementary material
The Supplementary material for this article can be found online at: https://www.frontiersin.org/articles/10.3389/fvets.2024.1421946/full#supplementary-material
SUPPLEMENTARY FIGURE 1 | Negative controls of immunohistochemical staining for anti-rabbit secondary antibody (VEGFR2) and anti-mouse secondary antibody (CD31 and vWF). Scale bar is 100 µm.
References
1. Cao, Y, Gong, Y, Liu, L, Zhou, Y, Fang, X, Zhang, C, et al. The use of human umbilical vein endothelial cells (HUVECs) as an in vitro model to assess the toxicity of nanoparticles to endothelium: a review. J Appl Toxicol. (2017) 37:1359–69. doi: 10.1002/jat.3470
2. Shi, H, Zhu, Y, Xing, C, Li, S, Bao, Z, Lei, L, et al. An injectable thermosensitive hydrogel for dual delivery of diclofenac and Avastin® to effectively suppress inflammatory corneal neovascularization. Int J Pharm. (2022) 625:122081. doi: 10.1016/j.ijpharm.2022.122081
3. Nowak-Sliwinska, P, Alitalo, K, Allen, E, Anisimov, A, Aplin, AC, Auerbach, R, et al. Consensus guidelines for the use and interpretation of angiogenesis assays. Angiogenesis. (2018) 21:425–532. doi: 10.1007/s10456-018-9613-x
4. Zhao, Z, Sun, W, Guo, Z, Zhang, J, Yu, H, and Liu, B. Mechanisms of lncRNA/microRNA interactions in angiogenesis. Life Sci. (2020) 254:116900. doi: 10.1016/j.lfs.2019.116900
5. Folkman, J. Angiogenesis. Annu Rev Med. (2006) 57:1–18. doi: 10.1146/annurev.med.57.121304.131306
6. Rajabi, M, and Mousa, SA. The role of angiogenesis in Cancer treatment. Biomedicines. (2017) 5:34. doi: 10.3390/biomedicines5020034
7. Rieger, J, Kaessmeyer, S, Al Masri, S, Hünigen, H, and Plendl, J. Endothelial cells and angiogenesis in the horse in health and disease-a review. Anat Histol Embryol. (2020) 49:656–78. doi: 10.1111/ahe.12588
8. Ozel, I, Duerig, I, Domnich, M, Lang, S, Pylaeva, E, and Jablonska, J. The good, the bad, and the ugly: neutrophils, angiogenesis, and Cancer. Cancers. (2022) 14:536. doi: 10.3390/cancers14030536
9. Bodaan, CJ, Wise, LM, Wakelin, KA, Stuart, GS, Real, NC, Mercer, AA, et al. Short-term treatment of equine wounds with orf virus IL-10 and VEGF-E dampens inflammation and promotes repair processes without accelerating closure. Wound Repair Regen. (2016) 24:966–80. doi: 10.1111/wrr.12488
10. Brooks, AC, Menzies-Gow, N, Bailey, SR, Cunningham, FM, and Elliott, J. Endotoxin-induced HIF-1alpha stabilisation in equine endothelial cells: synergistic action with hypoxia. Inflamm Res. (2010) 59:689–98. doi: 10.1007/s00011-010-0180-x
11. Kovac, M, Litvin, YA, Aliev, RO, Zakirova, EY, Rutland, CS, Kiyasov, AP, et al. Gene therapy using plasmid DNA encoding VEGF164 and FGF2 genes: a novel treatment of naturally occurring tendinitis and Desmitis in horses. Front Pharmacol. (2018) 9:978. doi: 10.3389/fphar.2018.00978
12. Martano, M, Power, K, Restucci, B, Pagano, I, Altamura, G, Borzacchiello, G, et al. Expression of vascular endothelial growth factor (VEGF) in equine sarcoid. BMC Vet Res. (2018) 14:266. doi: 10.1186/s12917-018-1576-z
13. Moore, CP, Halenda, RM, Grevan, VL, and Collins, BK. Post traumatic keratouveitis in horses. Equine Vet J. (1998) 30:366–72. doi: 10.1111/j.2042-3306.1998.tb04503.x
14. Müller, K, Ellenberger, C, Hoppen, H-O, and Schoon, H-A. Immunohistochemical study of angiogenesis and angiogenic factors in equine granulosa cell tumours. Res Vet Sci. (2012) 92:471–7. doi: 10.1016/j.rvsc.2011.02.016
15. Wakelin, KA. Viral proteins as novel therapeutics in chronic horse wounds. Otago: University of Otago (2015).
16. Wise, LM, Bodaan, CJ, Stuart, GS, Real, NC, Lateef, Z, Mercer, AA, et al. Treatment of limb wounds of horses with orf virus IL-10 and VEGF-E accelerates resolution of exuberant granulation tissue, but does not prevent its development. PLoS One. (2018) 13:e0197223. doi: 10.1371/journal.pone.0197223
17. Gilger, B, and Hollingsworth, SR. Diseases of the uvea, uveitis, and recurrent uveitis In: BC Gilger, editor. Equine ophthalmology. 3rd ed. Iowa: Wiley Blackwell Ames (2017). 380–406.
18. Knottenbelt, DC. The equine sarcoid: why are there so many treatment options? Vet Clin North Am Equine Pract. (2019) 35:243–62. doi: 10.1016/j.cveq.2019.03.006
19. Tornago, R, Sabattini, S, de Simoi, A, Bettini, G, Furlanello, T, Baldassarre, V, et al. Treatment of oral squamous cell carcinoma in a horse by surgical debulking followed by metronomic chemotherapy. Equine Vet Educ. (2015) 29:208–12. doi: 10.1111/eve.12427
20. Irvin, MW, Zijlstra, A, Wikswo, JP, and Pozzi, A. Techniques and assays for the study of angiogenesis. Exp Biol Med (Maywood). (2014) 239:1476–88. doi: 10.1177/1535370214529386
21. Medina-Leyte, DJ, Domínguez-Pérez, M, Mercado, I, Villarreal-Molina, MT, and Jacobo-Albavera, L. Use of human umbilical vein endothelial cells (HUVEC) as a model to study cardiovascular disease: a review. Appl Sci. (2020) 10:938. doi: 10.3390/app10030938
22. Dietze, K, Slosarek, I, Fuhrmann-Selter, T, Hopperdietzel, C, Plendl, J, and Kaessmeyer, S. Isolation of equine endothelial cells and life cell angiogenesis assay. Clin Hemorheol Microcirc. (2014) 58:127–46. doi: 10.3233/CH-141877
23. Huang, H, Lavoie-Lamoureux, A, and Lavoie, JP. Cholinergic stimulation attenuates the IL-4 induced expression of E-selectin and vascular endothelial growth factor by equine pulmonary artery endothelial cells. Vet Immunol Immunopathol. (2009) 132:116–21. doi: 10.1016/j.vetimm.2009.05.003
24. Laval, K, Favoreel, HW, Poelaert, KCK, van Cleemput, J, and Nauwynck, HJ. Equine herpesvirus type 1 enhances viral replication in CD172a+ Monocytic cells upon adhesion to endothelial cells. J Virol. (2015) 89:10912–23. doi: 10.1128/JVI.01589-15
25. Menzies-Gow, NJ, Bailey, SR, Berhane, Y, Brooks, AC, and Elliott, J. Evaluation of the induction of vasoactive mediators from equine digital vein endothelial cells by endotoxin. Am J Vet Res. (2008) 69:349–55. doi: 10.2460/ajvr.69.3.349
26. Wagner, WH, Henderson, RM, Hicks, HE, Banes, AJ, and Johnson, G. Differences in morphology, growth rate, and protein synthesis between cultured arterial and venous endothelial cells. J Vasc Surg. (1988) 8:509–19. doi: 10.1016/0741-5214(88)90119-X
27. Dela Paz, NG, and D’Amore, PA. Arterial versus venous endothelial cells. Cell Tissue Res. (2009) 335:5–16. doi: 10.1007/s00441-008-0706-5
28. Aird, WC. Phenotypic heterogeneity of the endothelium: I. Structure, function, andmechanisms. Circ Res. (2007) 100:158–73. doi: 10.1161/01.RES.0000255691.76142.4a
29. Lau, S, Gossen, M, Lendlein, A, and Jung, F. Venous and arterial endothelial cells from human umbilical cords: potential cell sources for cardiovascular research. Int J Mol Sci. (2021) 22:978. doi: 10.3390/ijms22020978
30. Baudin, B, Bruneel, A, Bosselut, N, and Vaubourdolle, M. A protocol for isolation and culture of human umbilical vein endothelial cells. Nat Protoc. (2007) 2:481–5. doi: 10.1038/nprot.2007.54
31. Fearon, IM, Gaça, MD, and Nordskog, BK. In vitro models for assessing the potential cardiovascular disease risk associated with cigarette smoking. Toxicol In Vitro. (2013) 27:513–22. doi: 10.1016/j.tiv.2012.08.018
32. Vion, A-C, Ramkhelawon, B, Loyer, X, Chironi, G, Devue, C, Loirand, G, et al. Shear stress regulates endothelial microparticle release. Circ Res. (2013) 112:1323–33. doi: 10.1161/CIRCRESAHA.112.300818
33. Sadick, H, Schäfer, E, Weiss, C, Rotter, N, Müller, CE, Birk, R, et al. An in vitro study on the effect of bevacizumab on endothelial cell proliferation and VEGF concentration level in patients with hereditary hemorrhagic telangiectasia. Exp Ther Med. (2022) 24:555. doi: 10.3892/etm.2022.11493
34. Wang, Y, Fei, D, Vanderlaan, M, and Song, A. Biological activity of bevacizumab, a humanized anti-VEGF antibody in vitro. Angiogenesis. (2004) 7:335–45. doi: 10.1007/s10456-004-8272-2
35. Bachetti, T, and Morbidelli, L. Endothelial cells in culture: a model for studying vascular functions. Pharmacol Res. (2000) 42:9–19. doi: 10.1006/phrs.1999.0655
36. Rhim, JS, Tsai, WP, Chen, ZQ, Chen, Z, van Waes, C, Burger, AM, et al. A human vascular endothelial cell model to study angiogenesis and tumorigenesis. Carcinogenesis. (1998) 19:673–81. doi: 10.1093/carcin/19.4.673
37. Onat, D, Brillon, D, Colombo, PC, and Schmidt, AM. Human vascular endothelial cells: a model system for studying vascular inflammation in diabetes and atherosclerosis. Curr Diab Rep. (2011) 11:193–202. doi: 10.1007/s11892-011-0182-2
38. Lessiak, U, Pratscher, B, Tichy, A, and Nell, B. Bevacizumab efficiently inhibits VEGF-associated cellular processes in equine umbilical vein endothelial cells: an in vitro characterization. Vet Sci. (2023) 10:632. doi: 10.3390/vetsci10110632
39. Suarez-Arnedo, A, Torres Figueroa, F, Clavijo, C, Arbeláez, P, Cruz, JC, and Muñoz-Camargo, C. An image J plugin for the high throughput image analysis of in vitro scratch wound healing assays. PLoS One. (2020) 15:e0232565. doi: 10.1371/journal.pone.0232565
40. Carpentier, G, Berndt, S, Ferratge, S, Rasband, W, Cuendet, M, Uzan, G, et al. Angiogenesis analyzer for image J-A comparative morphometric analysis of "endothelial tube formation assay" and "fibrin bead assay". Sci Rep. (2020) 10:11568. doi: 10.1038/s41598-020-67289-8
41. Weibel, ER, and Palade, GE. New cytoplasmic components in arterial endothelia. J Cell Biol. (1964) 23:101–12. doi: 10.1083/jcb.23.1.101
42. Weibel, ER. Fifty years of Weibel-Palade bodies: the discovery and early history of an enigmatic organelle of endothelial cells. J Throm Haemost. (2012) 10:979–84. doi: 10.1111/j.1538-7836.2012.04718.x
43. Benbarek, H, Grülke, S, Deby-Dupont, G, Deby, C, Mathy-Hartert, M, Caudron, I, et al. Cytotoxicity of stimulated equine neutrophils on equine endothelial cells in culture. Equine Vet J. (2000) 32:327–33. doi: 10.2746/042516400777032273
44. Hedges, JF, Demaula, CD, Moore, BD, McLaughlin, BE, Simon, SI, and MacLachlan, NJ. Characterization of equine E-selectin. Immunology. (2001) 103:498–504. doi: 10.1046/j.1365-2567.2001.01262.x
45. Lamar, CH, Turek, JJ, Bottoms, GD, and Fessler, JF. Equine endothelial cells in vitro. Am J Vet Res. (1986) 47:956–8.
46. MacEachern, KE, Smith, GL, and Nolan, AM. Methods for the isolation, culture and characterisation of equine pulmonary artery endothelial cells. Res Vet Sci. (1997) 62:147–52. doi: 10.1016/s0034-5288(97)90137-5
47. Rebière Pouyade, G, Salciccia, A, Ceusters, J, Deby-Dupont, G, Serteyn, D, and Mouithys-Mickalad, A. Production of free radicals and oxygen consumption by primary equine endothelial cells during anoxia-reoxygenation. Open Biochem J. (2011) 5:52–9. doi: 10.2174/1874091X01105010052
48. Smith, D, Hamblin, A, and Edington, N. Equid herpesvirus 1 infection of endothelial cells requires activation of putative adhesion molecules: an in vitro model. Clin Exp Immunol. (2002) 129:281–7. doi: 10.1046/j.1365-2249.2002.01463.x
49. Chiam, R, Smid, L, Kydd, JH, Smith, KC, Platt, A, and Davis-Poynter, NJ. Use of polarised equineendothelial cell cultures and an in vitro thrombosis model for potential characterisation of EHV-1 strain variation. Vet Microbiol. (2006) 113:243–9. doi: 10.1016/j.vetmic.2005.11.005
50. Tearle, JP, Smith, KC, Platt, AJ, Hannant, D, Davis-Poynter, NJ, and Mumford, JA. In vitro characterisation of high and low virulence isolates of equine herpesvirus-1 and -4. Res Vet Sci. (2003) 75:83–6. doi: 10.1016/s0034-5288(03)00031-6
51. Brindley, MA, and Maury, W. Equine infectious anemia virus entry occurs through clathrin-mediated endocytosis. J Virol. (2008) 82:1628–37. doi: 10.1128/JVI.01754-07
52. Relou, IA, Damen, CA, van der Schaft, DW, Groenewegen, G, and Griffioen, AW. Effect of culture conditions on endothelial cell growth and responsiveness. Tissue Cell. (1998) 30:525–30. doi: 10.1016/s0040-8166(98)80032-3
53. Bailey, SR, and Cunningham, FM. Adherence of eosinophils from allergic and normal ponies to cultured equine endothelial cells. Inflamm Res. (2001) 50:32–8. doi: 10.1007/s000110050721
54. Johnstone, S, Barsova, J, Campos, I, and Frampton, AR. Equine herpesvirus type 1 modulates inflammatory host immune response genes in equine endothelial cells. Vet Microbiol. (2016) 192:52–9. doi: 10.1016/j.vetmic.2016.06.012
55. Spiesschaert, B, Goldenbogen, B, Taferner, S, Schade, M, Mahmoud, M, Klipp, E, et al. Role of gB and pUS3 in equine herpesvirus 1 transfer between peripheral blood mononuclear cells and endothelial cells: a dynamic in vitro model. J Virol. (2015) 89:11899–908. doi: 10.1128/JVI.01809-15
56. Bauman, E, Granja, PL, and Barrias, CC. Fetal bovine serum-free culture of endothelial progenitor cells-progress and challenges. J Tissue Eng Regen Med. (2018) 12:1567–78. doi: 10.1002/term.2678
57. Zakrzewski, SS, Richter, JF, Krug, SM, Jebautzke, B, Lee, I-FM, Rieger, J, et al. Improved cell line IPEC-J2, characterized as a model for porcine jejunal epithelium. PLoS One. (2013) 8:e79643. doi: 10.1371/journal.pone.0079643
58. Grooby, WL, Krishnan, R, and Russ, GR. Characterization of ovine umbilical vein endothelial cells and their expression of cell adhesion molecules: comparative study with human endothelial cells. Immunol Cell Biol. (1997) 75:21–8. doi: 10.1038/icb.1997.4
59. MacLean, AG, Orandle, MS, Alvarez, X, Williams, KC, and Lackner, AA. Rhesus macaque brain microvessel endothelial cells behave in a manner phenotypically distinct from umbilical vein endothelial cells. J Neuroimmunol. (2001) 118:223–32. doi: 10.1016/s0165-5728(01)00348-4
60. Marin, V, Kaplanski, G, Gres, S, Farnarier, C, and Bongrand, P. Endothelial cell culture: protocol to obtain and cultivate human umbilical endothelial cells. J Immunol Methods. (2001) 254:183–90. doi: 10.1016/S0022-1759(01)00408-2
61. Gospodarowicz, D, and Cheng, J. Heparin protects basic and acidic FGF from inactivation. J Cell Physiol. (1986) 128:475–84. doi: 10.1002/jcp.1041280317
62. Montesano, R, Vassalli, JD, Baird, A, Guillemin, R, and Orci, L. Basic fibroblast growth factor induces angiogenesis in vitro. Proc Natl Acad Sci USA. (1986) 83:7297–301. doi: 10.1073/pnas.83.19.7297
63. Sawada, S, Toyoda, T, Takamatsu, H, Niwa, I, Maebo, N, Tsuji, H, et al. Prostacyclin generation by cultured human vascular endothelial cells with reference to angiotensin I-converting enzyme. Jpn Circ J. (1986) 50:242–7. doi: 10.1253/jcj.50.242
64. Okabe, T, Yamagata, K, Fujisawa, M, Takaku, F, Hidaka, H, and Umezawa, Y. Induction by fibroblast growth factor of angiotensin converting enzyme in vascular endothelial cells in vitro. Biochem Biophys Res Commun. (1987) 145:1211–6. doi: 10.1016/0006-291x(87)91566-x
65. Reinders, JH, Vervoorn, RC, Verweij, CL, and van Mourik, JA. Perturbation of cultured human vascular endothelial cells by phorbol ester or thrombin alters the cellular von Willebrand factor distribution. J Cell Physiol. (1987) 133:79–87. doi: 10.1002/jcp.1041330110
66. Liu, Y, Tian, H, Blobe, GC, Theuer, CP, Hurwitz, HI, and Nixon, AB. Effects of the combination of TRC105 and bevacizumab on endothelial cell biology. Investig New Drugs. (2014) 32:851–9. doi: 10.1007/s10637-014-0129-y
67. Cajero-Juárez, M, Avila, B, Ochoa, A, Garrido-Guerrero, E, Varela-Echavarría, A, Martínez de la Escalera, G, et al. Immortalization of bovine umbilical vein endothelial cells: a model for the study of vascular endothelium. Eur J Cell Biol. (2002) 81:1–8. doi: 10.1078/0171-9335-00213
68. Hong, HX, Zhang, YM, Xu, H, Su, ZY, and Sun, P. Immortalization of swine umbilical vein endothelial cells with human telomerase reverse transcriptase. Mol Cells. (2007) 24:358–63. doi: 10.1016/S1016-8478(23)07351-X
69. Bruneel, A, Labas, V, Mailloux, A, Sharma, S, Vinh, J, Vaubourdolle, M, et al. Proteomic study of human umbilical vein endothelial cells in culture. Proteomics. (2003) 3:714–23. doi: 10.1002/pmic.200300409
70. Hua, S, Song, Z, Zhenga, Q, and Nieb, J. Differences in the primary culture, purification and biological characteristics between endothelial cells and smooth muscle cells from rat aorta. J Nanjing Med Univ. (2009) 23:241–6. doi: 10.1016/S1007-4376(09)60063-0
71. Plikus, MV, Wang, X, Sinha, S, Forte, E, Thompson, SM, Herzog, EL, et al. Fibroblasts: origins, definitions, and functions in health and disease. Cell. (2021) 184:3852–72. doi: 10.1016/j.cell.2021.06.024
72. Jaffe, EA, Nachman, RL, Becker, CG, and Minick, CR. Culture of human endothelial cells derived from umbilical veins. Identification by morphologic and immunologic criteria. J Clin Invest. (1973) 52:2745–56. doi: 10.1172/JCI107470
73. Wu, Y, Wang, Z, Hu, G, and Zhang, T. Isolation and culture of rat intestinal mucosal microvascular endothelial cells using immunomagnetic beads. J Immunol Methods. (2022) 507:113296. doi: 10.1016/j.jim.2022.113296
74. Rhodin, JA. Ultrastructure of mammalian venous capillaries, venules, and small collecting veins. J Ultrastruct Res. (1968) 25:452–500. doi: 10.1016/s0022-5320(68)80098-x
75. Raviola, E, and Raviola, G. A light and electron microscopic study of the pecten of the pigeon eye. Am J Anat. (1967) 120:427–61. doi: 10.1002/aja.1001200304
76. Yano, K, Gale, D, Massberg, S, Cheruvu, PK, Monahan-Earley, R, Morgan, ES, et al. Phenotypic heterogeneity is an evolutionarily conserved feature of the endothelium. Blood. (2007) 109:613–5. doi: 10.1182/blood-2006-05-026401
77. Valentijn, KM, Sadler, JE, Valentijn, JA, Voorberg, J, and Eikenboom, J. Functional architecture of Weibel-Palade bodies. Blood. (2011) 117:5033–43. doi: 10.1182/blood-2010-09-267492
78. Rieger, J, Hopperdietzel, C, Kaessmeyer, S, Slosarek, I, Diecke, S, Richardson, K, et al. Human and equine endothelial cells in a live cell imaging scratch assay in vitro. Clin Hemorheol Microcirc. (2018) 70:495–509. doi: 10.3233/CH-189316
79. Bosch, G, Moleman, M, Barneveld, A, van Weeren, PR, and van Schie, HTM. The effect of platelet-rich plasma on the neovascularization of surgically created equine superficial digital flexor tendon lesions. Scand J Med Sci Sports. (2011) 21:554–61. doi: 10.1111/j.1600-0838.2009.01070.x
80. Doan, CC, Le, TL, Hoang, NS, Doan, NT, van Le, D, and Do, MS. Differentiation of umbilical cord lining membrane-derived mesenchymal stem cells into endothelial-like cells. Iran Biomed J. (2014) 18:67–75. doi: 10.6091/ibj.1261.2013
81. Müller, AM, Hermanns, MI, Skrzynski, C, Nesslinger, M, Müller, K-M, and Kirkpatrick, CJ. Expression of the endothelial markers PECAM-1, vWf, and CD34 in vivo and in vitro. Exp Mol Pathol. (2002) 72:221–9. doi: 10.1006/exmp.2002.2424
82. Ryan, JW, and Ryan, US. Endothelial surface enzymes and the dynamic processing of plasma substrates. Int Rev Exp Pathol. (1984) 26:1–43.
83. Goldsmith, JC, McCormick, JJ, and Yen, A. Endothelial cell cycle kinetics. Changes in culture and correlation with endothelial properties. Lab Investig. (1984) 51:643–7.
84. Noveral, JP, Mueller, SN, and Levine, EM. Release of angiotensin I-converting enzyme by endothelial cells in vitro. J Cell Physiol. (1987) 131:1–5. doi: 10.1002/jcp.1041310102
85. Dimmeler, S, and Zeiher, AM. Endothelial cell apoptosis in angiogenesis and vessel regression. Circ Res. (2000) 87:434–9. doi: 10.1161/01.res.87.6.434
86. Merkl, M, Ulbrich, SE, Otzdorff, C, Herbach, N, Wanke, R, Wolf, E, et al. Microarray analysis of equine endometrium at days 8 and 12 of pregnancy. Biol Reprod. (2010) 83:874–86. doi: 10.1095/biolreprod.110.085233
87. Melincovici, CS, Boşca, AB, Şuşman, S, Mărginean, M, Mihu, C, Istrate, M, et al. Vascular endothelial growth factor (VEGF) - key factor in normal and pathological angiogenesis. Romanian J Morphol Embryol. (2018) 59:455–67.
88. Allen, WR, Gower, S, and Wilsher, S. Immunohistochemical localization of vascular endothelial growth factor (VEGF) and its two receptors (Flt-I and KDR) in the endometrium and placenta of the mare during the oestrous cycle and pregnancy. Reprod Domest Anim. (2007) 42:516–26. doi: 10.1111/j.1439-0531.2006.00815.x
89. Shalaby, F, Rossant, J, Yamaguchi, TP, Gertsenstein, M, Wu, XF, Breitman, ML, et al. Failure of blood-island formation and vasculogenesis in Flk-1-deficient mice. Nature. (1995) 376:62–6. doi: 10.1038/376062a0
90. Salmon, J.H., and Gilger, B.C. Serum and Aqueous humor inflammatory cytokine profiles in recurrent uveitis. IOVS: ARVO Annual Meeting Abstract (2007).
91. Eelen, G, Treps, L, Li, X, and Carmeliet, P. Basic and therapeutic aspects of angiogenesis updated. Circ Res. (2020) 127:310–29. doi: 10.1161/CIRCRESAHA.120.316851
92. Addis, R, Campesi, I, Fois, M, Capobianco, G, Dessole, S, Fenu, G, et al. Human umbilical endothelial cells (HUVECs) have a sex: characterisation of the phenotype of male and female cells. Biol Sex Differ. (2014) 5:18. doi: 10.1186/s13293-014-0018-2
93. Kurbacher, CM, and Cree, IA. Chemosensitivity testing using microplate adenosine triphosphate-based luminescence measurements. Methods Mol Med. (2005) 110:101–20. doi: 10.1385/1-59259-869-2:101
94. Crouch, SP, Kozlowski, R, Slater, KJ, and Fletcher, J. The use of ATP bioluminescence as a measure of cell proliferation and cytotoxicity. J Immunol Methods. (1993) 160:81–8. doi: 10.1016/0022-1759(93)90011-u
95. Costa, G, Harrington, KI, Lovegrove, HE, Page, DJ, Chakravartula, S, Bentley, K, et al. Asymmetric division coordinates collective cell migration in angiogenesis. Nat Cell Biol. (2016) 18:1292–301. doi: 10.1038/ncb3443
96. Hamm, MJ, Kirchmaier, BC, and Herzog, W. Sema 3d controls collective endothelial cell migration by distinct mechanisms via Nrp1 and PlxnD1. J Cell Biol. (2016) 215:415–30. doi: 10.1083/jcb.201603100
97. Vitorino, P, and Meyer, T. Modular control of endothelial sheet migration. Genes Dev. (2008) 22:3268–81. doi: 10.1101/gad.1725808
Keywords: EqUVEC, angiogenesis, equine endothelial cells, immunohistology, TEM, cell culture
Citation: Lessiak U, Melchert M, Walter I, Kummer S, Nell B, Tschulenk W and Pratscher B (2024) Isolation-protocol, characterization, and in-vitro performance of equine umbilical vein endothelial cells. Front. Vet. Sci. 11:1421946. doi: 10.3389/fvets.2024.1421946
Edited by:
Fausto Cremonesi, University of Milan, ItalyReviewed by:
Gustavo Rosa, São Paulo State University (UNESP), BrazilNicola Antonio Martino, University of Bari Aldo Moro, Italy
Copyright © 2024 Lessiak, Melchert, Walter, Kummer, Nell, Tschulenk and Pratscher. This is an open-access article distributed under the terms of the Creative Commons Attribution License (CC BY). The use, distribution or reproduction in other forums is permitted, provided the original author(s) and the copyright owner(s) are credited and that the original publication in this journal is cited, in accordance with accepted academic practice. No use, distribution or reproduction is permitted which does not comply with these terms.
*Correspondence: Ulrike Lessiak, dWxyaWtlLmxlc3NpYWtAdmV0bWVkdW5pLmFjLmF0