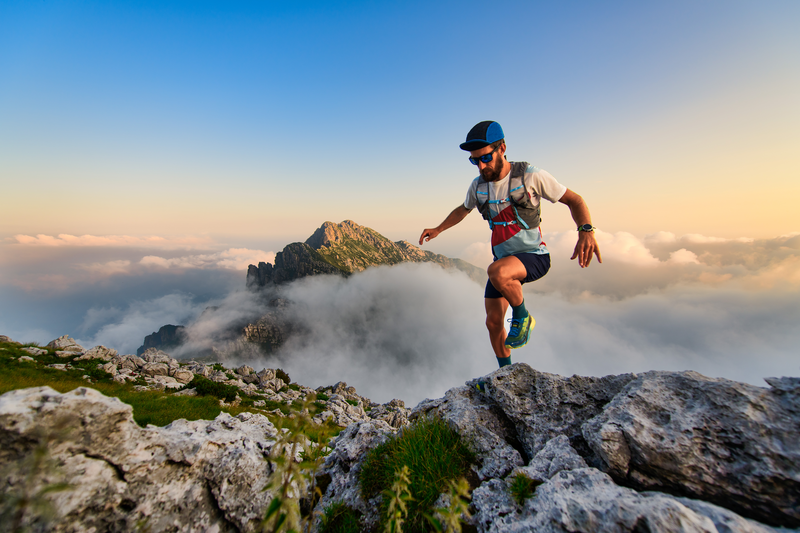
95% of researchers rate our articles as excellent or good
Learn more about the work of our research integrity team to safeguard the quality of each article we publish.
Find out more
ORIGINAL RESEARCH article
Front. Vet. Sci. , 08 August 2024
Sec. Animal Reproduction - Theriogenology
Volume 11 - 2024 | https://doi.org/10.3389/fvets.2024.1418091
This article is part of the Research Topic Understanding the Female Reproductive Microbiome in Livestock View all 9 articles
Introduction: Postpartum endometritis is a prevalent reproductive disorder in bovines, leading to a prolonged open period, infertility, and other complications. While Lactobacillus strains can mitigate these conditions by reducing uterine inflammation, their effectiveness is limited due to a lack of direct anti microbial action and extended treatment duration. This study aimed to construct a recombinant Lactobacillus johnsonii strain expressing bovine Granulocyte-macrophage colony-stimulating factor (GM-CSF) to evaluate its potential in reducing postpartum uterine inflammation.
Methods: The recombinant Lactobacillus johnsonii strain was engineered to express bovine GM-CSF and administered to pregnant mice via vaginal perfusion. Postpartum endometritis was induced using E. coli infection, and the protective effects of the engineered strain were assessed. Inflammatory markers (IL-6, IL-1β, TNF-α), myeloperoxidase (MPO) activity, and nitric oxide (NO) concentration were measured. Histological examination was performed to evaluate uterine morphology and pathological damage.
Results: The recombinant L. johnsonii strain expressing GM-CSF significantly reduced inflammation levels induced by E. coli infection in the uterus. This reduction was evidenced by decreased expression of IL-6, IL-1β, TNF-α, as well as reduced MPO activity and NO concentration. Histological examination revealed improved uterine morphology and reduced pathological damage in mice treated with the recombinant GM-CSF strain. Crucially, the recombinant strain also exerts beneficial effects on bovine endometritis by reducing levels of inflammatory cytokines, suggesting a beneficial effect on clinical bovine endometritis.
Conclusion: The recombinant Lactobacillus johnsonii expressing GM-CSF demonstrated protective effects against postpartum endometritis in bovines by reducing inflammatory cytokines. The findings indicate the potential clinical application of this engineered strain in preventing postpartum uterine inflammation, offering a novel and effective protective option for related disorders and improving bovine reproductive efficiency.
Endometritis is one of the main diseases of bovine reproductive disorders, which can damage the reproductive ability of cows and accelerate their elimination. At the same time, it can bring huge economic losses to the livestock industry (1). Typically, 20 to 40% of cattle develop acute clinical uterine disease within a week from parturition (metritis), while 20% have a persistent clinical disease 3 weeks after calving (endometritis). Additionally, approximately 30% suffer from a chronic subclinical inflammation of the uterus (subclinical endometritis) (2). Currently, antibiotic therapy represents the prevailing approach for treating endometritis. However, the side effects of long-term antibiotic treatment, such as antibiotic resistance and antibiotic residue, have yielded unsatisfactory treatment effects. Consequently, addressing the side effects of antibiotics has prompted an increased emphasis on endometritis prevention. The most common preventive measures include enhancing dietary management or incorporating vitamins and minerals into the diet (3, 4). Unfortunately, the efficacy of these methods remains limited. Hence, there is an urgent need to identify an effective protective strategy against endometritis that can reduce its incidence rate and circumvent the adverse effects of antibiotics.
Neutrophil function is vital for the innate immunity in the reproductive tract of dairy cows. Following parturition, there is an immediate decline in neutrophil counts within the circulatory system, likely attributed to the migration of these cells towards sites of inflammation, including the postpartum uterus and mammary gland (5). Additionally, a transient state of immunosuppression occurs postpartum, characterized by impaired neutrophil phagocytosis and attenuated oxidative burst activity (6, 7). This reduction in both neutrophil abundance and functional capacity is considered a primary factor contributing to the heightened risk of retained placenta, metritis, subclinical endometritis, and mastitis (8–10). Granulocyte-macrophage colony-stimulating factor (GM-CSF) can be produced by various cell types, such as macrophages, fibroblasts, activated T-lymphocytes, natural killer cells, mast cells and so on (11). It serves as a potent hematopoietic growth factor, stimulating the expansion and maturation of monocyte-macrophages, dendritic cells, and granulocytes derived from hematopoietic progenitor cells (12). GM-CSF is widely distributed within the female reproductive tract and regulates a multitude of myeloid leukocytes within the uterus and decidual tissue. Moreover, it acts as a local mediator involved in the migration and activation of endometrial macrophages, granulocytes, and dendritic cells (13). GM-CSF also plays a role in tissue remodeling during acute structural changes associated with embryo implantation, placental development, and postpartum endometrial regeneration (14). However, the expensive price of GM-CSF has limited its application in veterinary clinical practice.
As a substitute for antibiotics, probiotics offer a broad spectrum of applications in the treatment of animal diseases resulting from bacterial infections. Lactic acid bacteria (LAB) represent the predominant microbial population within the healthy reproductive tract of cows (15). Notably, Lactobacillus johnsonii, isolated from the bovine vagina, has demonstrated specific adherence to the epithelium and the production of inhibitory substances (16). Our prior investigations have revealed that the Lactobacillus johnsonii strain obtained from bovine uterine secretions exhibits remarkable capabilities in preventing endometritis and exerts potent anti-inflammatory effects (17). However, using probiotics as a substitute for antibiotics may suffer from unstable effectiveness, limited applicability, lack of direct antimicrobial action, and longer treatment durations. Recombinant lactic acid bacteria have been successful in expressing numerous bacterial and viral antigens, thereby contributing to the enhancement of microbial homeostasis within the uterine environment (18, 19). Therefore, in the present study, we constructed a recombinant Lactobacillus johnsonii expressing bovine GM-CSF. Subsequently, this strain was administered to pregnant mice via vaginal perfusion, then induced postpartum endometritis mouse model, finally evaluated the effects of recombinant GM-CSF Lactobacillus johnsonii on the protective effects of postpartum endometritis mouse model. And also the GM-CSF strain demonstrated a favorable therapeutic efficacy against postpartum endometritis in bovines.
A total of 122 healthy BALB/c mice (8 weeks old, weighing 25–30 g) was employed in the present study. All animal experimental procedures were performed in accordance with the Regulations for Animal Experimentation of Jilin Agriculture University (JLAU08201409), and the animal facility was based on the National Institutes of Health Guide for the Care and Use of Laboratory Animals (NIH Publications No. 8023).
Lactobacillus johnsonii (stored in the China General Microbiological Culture Collection Center: GU428184.1) isolated from the uterine secretion of healthy dairy cows by our laboratory. The Lactobacillus johnsonii strain was incubated anaerobically at 37°C for 8 h to recover. Then 100 μL of the bacterial suspension was inoculated onto an MRS agar plate and cultured anaerobically at 37°C for 20 h. A single colony was picked and inoculated into 400 mL of MRS broth, which was then cultured to an OD of 0.5. The culture was chilled in an ice bath for 25 min and centrifuged at 4°C at 7,000 rpm for 8 min, resuspended in 20 mL of EPWB, followed by centrifugation at 4°C at 7,000 rpm for 8 min. This process was repeated twice. The pellet was resuspended in 15 mL of EPB, centrifuged again at 4°C at 7,000 rpm for 8 min, and the supernatant was discarded once more. The pellet was then resuspended in 2 mL of EPB, aliquoted into 200 μL per tube, and stored at −80°C for future use.
The Escherichia coli-Lactobacillus shuttle vector pPG612, is a type of cell-surface expression plasmid that contains an anchoring matrix-encoding pgsA gene, and it was derived from Bacillus subtilis behind the target gene. The pPG includes the ssUSP secretion signal before the target gene to ensure target protein secretion.
The bovine GM-CSF gene (NM_174027.2) was artificially synthesized and inserted into the vector PUC57 (Shanghai Sangon Biological Engineering and Technology Service Co., Ltd.). The length of the GM-CSF gene is 456 bp and includes GM-CSF gene coding sequence (sequences bp 10–441), two stop codon (TAATAG), restriction site EcoR I (gaattc), EcoR V (ggatcc), and a pair of protective bases (GCC and CGC) (Figure 1C).
Figure 1. Construction of the L. johnsonii expressing bovine GM-CSF (Lc-pPG-GM-CSF). (A) Schematic diagram of the recombinant plasmid pPG612-GM-CSF. (B) GM-CSF gene amplification products, M: DL2000 marker; 1, 2: GM-CSF gene amplification products. (C) Restriction enzyme assay of recombinant plasmid pPG612-GM-CSF. M: DL5000 marker; 1: pPG-GM-CSF restriction enzyme digestion product. (D) PCR test results of recombinant Lactobacillus, M: DL5000 marker; 1, 2: Lc-pPG-GM-CSF plasmid PCR product. (E) Genetic stability test results of recombinant Lactobacillus, M: DL5000 marker, 1–5: PCR amplification products of recombinant bacteria 1st, 10th, 20th, 30th and 40th generations. (F) Detection of protein expression of recombinant Lactobacillus; M: protein marker; 1: Lc-pPG-GM-CSF recombinant lactobacillus protein extract; 2: Lactobacillus johnsonii protein extract control.
To generate recombinant Lactobacillus johnsonii expressing the GM-CSF (L-GM-CSF), the DNA sequence for bovine GM-CSF was inserted into pPG-612 by restriction site EcoR I and EcoR V, followed by the formation of pPG-612-GM-CSF recombinant plasmids. The constitutive expression plasmid pPG-612-GM-CSF was transformed into Lactobacillus johnsonii competent cells by electroporation (Electroporator 2510, Eppendorf) under 2.1 kV 3 ms. The Lactobacillus johnsonii competent cells were thawed in an ice-water slurry for 10 min. Then, 5 μL of the pPG-GM-CSF recombinant plasmid was mixed gently with 100 μL of the Lactobacillus johnsonii competent cells. After incubating on ice for 5 min, transferred to a pre-chilled electroporation cuvette and allowed to rest for 2 min before applying a single electric pulse of 2.1 kV for 3 ms. Immediately after electroporation, the cells were chilled on ice for 10 min. The transformed cells were then transferred into 1,000 mL of MRS broth containing 15% sucrose and incubated anaerobically at 37°C for 2 h. The culture was centrifuged at 3,000 rpm 10 min, resuspended in 200 μL of MRS broth and spread onto an MRS agar plate containing 10 μg/mL of chloramphenicol. The plate was incubated anaerobically at 37°C overnight. Then, the treated Lactobacillus johnsonii was cultured in MRS broth medium contain 0.3 M sucrose, and the recombinant strain Lc-pPG-GM-CSF was obtained on MRS agar medium containing 10 μg/mL chloromycetin. Lactobacillus johnsonii containing wild-type pPG612 (Lc-pPG) was used as a vector control, and non-transformed wild-type Lactobacillus johnsonii was used as a negative control in all subsequent assays.
Expression of the recombinant plasmid Lc-pPG-GM-CSF in Lactobacillus johnsonii was detected using western blotting analysis. Briefly, Lc-pPG-GM-CSF and Lc-pPG were grown in basal MRS medium supplemented with 10 μg/mL chloramphenicol. Xylose was added to the culture medium to a final concentration of 10 g/L to induce GM-CSF expression. After induction at 37°C for 20 h, approximately 1 × 108 cell pellets were examined using sodium dodecyl sulphate-polyacrylamide gel electrophoresis (SDS-PAGE) and transferred to a nitrocellulose membrane. The membrane was blocked with 3% BSA and incubated with bovine GM-CSF antibody (Bioss, Beijing, China) diluted 1:1,000 with phosphate-buffered saline (PBS; Bioss, Beijing, China) overnight at 4°C. Affinity-purified horseradish peroxidase (HRP)-conjugated mouse anti-bovine immunoglobulin G (IgG; Bioss, Beijing, China) was used as the secondary antibody. The blot was visualized using chemiluminescence detection with a western ECL substrate (Thermo Scientific) using an Amersham Imager 600 (GE Healthcare, Boston, United Kingdom).
One hundred and twenty-two female BALB/c mice (8 weeks old, weighing 25–30 g) were sourced from Liaoning Changsheng Biotechnology Co., Ltd. The mice were housed in specific pathogen-free enclosures within the laboratory animal facility at Jilin Agricultural University, where they were maintained under standard conditions (humidity: 51–13%, temperature: 23 ± 3°C, light–dark cycle: 12/12). Following a period of adaptive feeding lasting 7 days, the mice were categorically assigned to one of four groups: (i) Control group (C, n = 28), which received standard feeding without additional interventions. (ii) Inflammatory control group (E, n = 28), where vaginal perfusion with normal saline occurred from gestational days 17.5 to 19.5, followed by uterine infusion with bovine pathogenic E. coli on the first and second days postpartum. E. coli was isolated from cows with endometritis, and the E. coli-induced endometritis mouse model was established following the protocol outlined in our previous studies (20, 21). Briefly, 25 μL of a mixed E. coli suspension (1 × 1010 CFU/mL) was inoculated into the uterus of anesthetized mice using a 19 mm soft needle (outer diameter 0.7 mm) to induce endometritis. (iii) L. johnsonii group (Lc, n = 34), subjected to vaginal perfusion with bacterial fluid from gestational days 17.5 to 19.5, and uterine infusion with pathogenic E. coli on the first and second days postpartum. Five hundred microliters of johnsonii (1 × 107 CFU/mL) was used in this study. (iv) Recombinant L. johnsonii group (Lc-GM-CSF, n = 32), receiving the same treatment as the Lc group.
The uterine tissue specimens were isolated, fixed in 4% buffered formaldehyde, and subsequently subjected to standard paraffin embedding for a duration exceeding 48 h. Following this, the samples underwent staining utilizing conventional hematoxylin-eosin (H&E) techniques. Cross-sectional visualization and analysis of the uterine tissue were performed using a light microscope (Olympus BX41, Olympus Optical Co., Ltd., Tokyo, Japan) and evaluated with Image-Pro Plus 6.0 software (Media Cybernetics, Bethesda, United States).
Total RNA was extracted from uterine tissues using the Trizol reagent (Gibco BRL; Thermo Fisher Scientific) in accordance with the manufacturer’s instructions. Subsequently, the RNA obtained from each sample was reverse transcribed into complementary DNA (cDNA) utilizing the PrimeScript™ RT reagent Kit with gDNA Eraser (Takara Biotechnology Co., Ltd.). The reverse transcription reaction conditions were as follows: genomic DNA elimination at 42°C for 2 min, reverse transcription at 37°C for 15 min, and inactivation of the reverse transcriptase at 85°C for 5 s. The subsequent step involved performing Quantitative Real-Time PCR using a 7500HT fast real-time PCR system (ABI; Thermo Fisher Scientific, Inc.). The amplification conditions were as follows: pre-denaturation at 95°C for 30 s, followed by 40 cycles of PCR reaction with denaturation at 95°C for 5 s, annealing at 60°C for 30 s. The relative expression levels were determined utilizing the 2−ΔΔCT Cq method. The primers employed for the reverse transcription-quantitative polymerase chain reaction (RT-qPCR) analysis were shown in Table 1.
Mice uterine tissue weighing 1 g was excised, immersed in 9 mL of phosphate-buffered saline (pH 7.2–7.4), and subjected to triple washes. The resulting wash samples were then collected and centrifuged at 4,000 rpm for 20 min at 4°C. The supernatants were collected and preserved at −20°C until further analysis. Bovine blood samples were obtained and subsequently centrifuged at 2,000 rpm for 15 min at 4°C, followed by storage at −80°C for subsequent analysis. Cotton swabs containing bovine uterine mucus were added to 300 μL PBS, shake for 2 min, freeze and thaw repeatedly in liquid nitrogen three times, centrifuge at 4°C 12,000 rpm for 15 min, collected the supernatant for further analysis. Commercial enzyme-linked immunosorbent assay (ELISA) kits (BYabscience) were employed for the assessment of IL-6, IL-1β and TNF-α protein expression levels. Absorbance values were read at 450 nm. Determination of myeloperoxidase (MPO) content and NO content in mouse uterine tissue.
The level of myeloperoxidase was assessed using the myeloperoxidase assay kit (procured from Nanjing Jiancheng Biology Co., Ltd., Nanjing, China), with absorbance readings at 450 nm conducted using a spectrophotometer. Uterine tissue samples were collected and homogenized for subsequent analysis of NO concentration in the supernatant, employing the NO assay kit (Solarbio Life Science, Beijing, China).
Statistical analyses were conducted using the one-way ANOVA program in SPSS22, while GraphPad Prism 7 was employed for further statistical analyses. The data were presented as mean ± standard deviation (SD). For multiple comparisons, one-way ANOVA was performed, followed by Duncan’s post hoc test. The data are expressed as the mean ± SD. Statistical significance was set at *p < 0.05.
The gene encoding bovine GM-CSF (456 bp) was artificially synthesized and inserted into the vector PUC57. Following this, specific primer pairs (Table 1) were utilized to amplify the GM-CSF fragment from the PUC57-GM-CSF, which was then inserted into the expression vector pPG612, resulting in the generation of the recombinant plasmid pPG612-GM-CSF (Figures 1A,B). Validation of this process was achieved through restriction enzyme digestion and sequencing (Figure 1C), confirming the successful construction of recombinant plasmid pPG-GM-CSF.
Subsequently, these recombinant plasmids were inserted into L. johnsonii via electroporation, constructed the recombinant L. johnsonii expressing bovine GM-CSF (Lc-pPG-GM-CSF). As depicted in Figure 1D, the PCR results confirmed the successful transfer of the recombinant plasmid pPG612-GM-CSF into L. johnsonii, and sequencing result confirmed that the inserted sequence corresponds to bovine GM-CSF (456 bp). Moreover, the stability of the recombinant L. johnsonii pPG612-GM-CSF over 40 generations is demonstrated in lanes 1–5 of Figure 1E, providing evidence of its successful inheritance. Finally, Western blotting revealed a 15 kDa band corresponding to the GM-CSF protein in the cell lysates of Lc-pPG-GM-CSF, confirming the successful construction of the L. johnsonii expressing bovine GM-CSF (Figure 1F).
We used the E. coli perfusion method to establish a mouse postpartum endometritis model, reported in our previous studies (20, 21). The immunological and constructive process of the postpartum endometritis mice model is shown in Figure 2A. Following two consecutive days of perfusion with E. coli, a statistical analysis was conducted to assess the incidence of postpartum endometritis in the respective mouse groups (Figure 2B). During the development and administration of the model, mice encompassed a control group (C, n = 28), an E. coli perfusion control group (E, n = 28), an L. johnsonii-immunization group (Lc, n = 34), and a recombinant L. johnsonii-immunization group (Lc-GM-CSF, n = 32). The results showed a substantial disparity, with the incidence rate in group E reaching 87.86 ± 6.23%. In contrast, the Lc-GM-CSF group exhibited a remarkable reduction in the incidence rate, dropping to 15.14 ± 9.9%. However, the incidence rate in the Lc group was 28.09% ± 7.02%, which is significantly higher than that of the Lc-GM-CSF group (p > 0.05). These findings underscore the significant protective efficacy of recombinant L. johnsonii expressing GM-CSF against E. coli-induced postpartum endometritis.
Figure 2. Recombinant L. johnsonii provides significant protection against E. coli-induced postpartum endometritis in mice model. (A) Immune and construction process of a mouse model of postpartum endometritis. (B) The incidence of postpartum endometritis in C, E, Lc, Lc-GM-CSF group. One-way ANOVA analysis was used for statistical analysis and all data are presented as mean ± standard deviation (SD). ns, p > 0.05, *p < 0.05, **p < 0.01, and ***p < 0.001.
Three days postpartum, uterine specimens were obtained and their morphological characteristics are shown in Figure 3A. As illustrated, the uteri in the E. coli group exhibited engorged, edema, and hypertrophy, accompanied by a diminished gland. Conversely, uteri in the control group displayed a normal appearance. The uteri in the Lc group had a similar length to the control group, but showed signs of redness, swelling, and increased thickness compared to the control group. The uteri in the Lc-GM-CSF group shared a similar length to the control group, and notably, evinced a substantial mitigation of engorged and edema compared to the E. coli group, approaching a normative uterine state.
Figure 3. The effect of Lc-pPG-GM-CSF on the morphology of uterine tissue in mice with endometritis. (A) Morphological observation of mouse uterus after three days of delivery. (B) H&E staining was used to observe the uterus of mice three days after delivery (magnification: ×200). C group, Control group; E group, an inflammatory control group; Lc group, vaginal perfusion with L. johnsonii from gestational days 17.5 to 19.5, and uterine infusion with pathogenic E. coli on the first and second days postpartum; Lc-GM-CSF group, vaginal perfusion with recombinant L. johnsonii expressing GM-CSF from gestational days 17.5 to 19.5, and uterine infusion with pathogenic E. coli on the first and second days postpartum. The red arrow represents uterine glands, and the black arrow represents capillaries.
The uterine morphological alterations are depicted in Figure 3B. Within the control cohort, a state of normal tissue morphology prevailed, characterized by a distinct demarcation between the endometrium and the myometrium, the number and morphology of the gland was normal. Conversely, the E. coli group exhibited conspicuous endometrial hyperemia and interstitial edema, along with an indistinct boundary between the endometrium and the myometrium, a reduction in glandular abundance, and partial exfoliation of the uterine epithelium. In the Lc group, although amelioration of hyperemia was observed, vestiges of hyperemic regions persisted, accompanied by the presence of a limited number of glands. The Lc-GM-CSF group showed close-to-normal uterine morphology, devoid of any hyperemic regions and showing discernible glandular structures. Following E. coli-induced inflammation, mice subjected to recombinant L. johnsonii expressing GM-CSF displayed diminished uterine pathological damage and histopathological alterations.
To further investigate the impact of recombinant L. johnsonii expressing GM-CSF on pro-inflammatory factors, we conducted an analysis on IL-6, IL-1β, and TNF-α at both the mRNA (q-PCR) and protein (ELISA, sensitivity: 0.1 pg/mL) levels of the mice uterine tissue. The mRNA and protein expressions of IL-6, IL-1β, and TNF-α were markedly elevated in the E. coli group (Figure 4). In contrast, the Lc group exhibited a substantial reduction in the expression of inflammation-associated mRNA and proteins when compared to the E. coli group (p < 0.001). Moreover, the Lc-GM-CSF group demonstrated a significant decrease in mRNA expression of IL-6 and TNF-α, as well as protein expressions of IL-6, IL-1β, and TNF-α, compared to the Lc group (p < 0.005). These findings suggested the potent anti-inflammatory effects of Lactobacillus interventions in mitigating the inflammatory response.
Figure 4. Effects of Lc-pPG-GM-CSF on the expression of pro-inflammatory factors at the protein and gene levels in mice with endometritis. Effects of Lc-pPG-GM-CSF on the expression of IL-6 (A), TNF-α (B) and IL-1β (C) in the uterine tissue of mice with endometritis at the gene level. Effect of Lc-pPG-GM-CSF on the expression of IL-6 (D) TNF-α (E) and IL-1β (F) in the uterine tissue of mice with endometritis at the protein level. C group, Control group; E group, an inflammatory control group; Lc group, vaginal perfusion with L. johnsonii from gestational days 17.5 to 19.5, and uterine infusion with pathogenic E. coli on the first and second days postpartum; LcGM-CSF group, vaginal perfusion with recombinant L. johnsonii expressing GM-CSF from gestational days 17.5 to 19.5, and uterine infusion with pathogenic E. coli on the first and second days postpartum. One-way ANOVA analysis was used for statistical analysis and all data are presented as mean ± standard deviation (SD). ns, p > 0.05, *p < 0.05, **p < 0.01, and ***p < 0.001.
The heightened myeloperoxidase (MPO) activity and augmented nitric oxide (NO) concentration serve as pivotal indicators of inflammatory pathologies, with alterations in their levels reflecting the extent of oxidative stress. Following uterine exposure to pathogenic E. coli, a notable increase in both MPO activity (Figure 5A) and NO concentration (Figure 5B) (p < 0.05) was observed compared to the Control group. Conversely, mice vaginal perfusion with Lc-GM-CSF demonstrated reduced MPO activity (Figure 5A) and NO concentration (Figure 5B) relative to the E. coli group and Lc group (p < 0.05). These observations indicate the potential of Lc-GM-CSF in the mitigating impact of uterine E. coli-induced inflammation by regulating MPO activity and NO concentration.
Figure 5. Effects of Lc-pPG-GM-CSF on the inflammatory response in mice with endometritis. Effect of Lc-pPG-GM-CSF on the MPO activity (A) and NO concentration (B) in the uterine tissue of mice with endometritis. C group, Control group; E group, an inflammatory control group; Lc group, vaginal perfusion with L. johnsonii from gestational days 17.5 to 19.5, and uterine infusion with pathogenic E. coli on the first and second days postpartum; Lc-GM-CSF group, vaginal perfusion with recombinant L. johnsonii expressing GM-CSF from gestational days 17.5 to 19.5, and uterine infusion with pathogenic E. coli on the first and second days postpartum. One-way ANOVA analysis was used for statistical analysis and all data are presented as mean ± standard deviation (SD). ns, p > 0.05, *p < 0.05, **p < 0.01, and ***p < 0.001.
To further evaluate the therapeutic effect of recombinant L. johnsonii on bovine endometritis, we conducted further investigation of pro-inflammatory factors in protein (ELISA) levels on bovine serum and uterine mucus from control and the treatment group. The protein expression levels of pro-inflammatory cytokines (IL-1, IL-6, and TNF-α) after treatment for clinical endometritis is presented in Figure 6. The highest serum and mucus concentrations of pro-inflammatory cytokines were observed in the E group (bovine endometritis group). While all studied pro-inflammatory cytokines showed a decrease in the treatment group (Lc and Lc-GM-CSF). Moreover, the LC-GM-CSF group (recombinant L. johnsonii treatment group) had the lowest concentrations (p < 0.005), similar to the normal control. These findings indicate that recombinant L. johnsonii expressing GM-CSF exert a favorable influence by decreasing the level of cytokines involved in endometritis inflammation.
Figure 6. Effects of Lc-pPG-GM-CSF on the expression of pro-inflammatory factors at the protein and gene levels in cows with endometritis. Effect of Lc-pPG-GM-CSF on the expression of IL-6 (A), TNF-α (B), and IL-1β (C) in the uterine mucus of cows. Effect of Lc-pPG-GM-CSF on the expression of IL-6 (D), TNF-α (E), and IL-1β (F) in the serum of cows with endometritis. C group, Control group; E group, an inflammatory control group; Lc group, vaginal perfusion with L. johnsonii from gestational days 17.5 to 19.5, and uterine infusion with pathogenic E. coli on the first and second days postpartum; Lc-GM-CSF group, vaginal perfusion with recombinant L. johnsonii expressing GM-CSF from gestational days 17.5 to 19.5, and uterine infusion with pathogenic E. coli on the first and second days postpartum. One-way ANOVA analysis was used for statistical analysis and all data are presented as mean ± standard deviation (SD). ns, p > 0.05, *p < 0.05, **p < 0.01, and ***p < 0.001.
Endometritis has always been an important disease causing reproductive disorders in dairy cows (22). In the research related to the treatment of bovine endometritis, the application of antibiotic treatment is relatively extensive, but in the long run, the combined use of antibiotics will often accelerate the drug resistance of pathogens and lead to antibiotic residues in milk, bringing huge food safety risks to the health of consumers (23). Therefore, it is an urgent problem for clinical treatment to avoid the side effects of antibiotics in treating bovine endometritis. In this study, we successfully constructed a recombinant Lactobacillus johnsonii strain expressing bovine GM-CSF, which was found to significantly reduce inflammation levels induced by E. coli infection postpartum endometritis mice model and demonstrated a significant therapeutic effect on cow endometritis.
Lactic acid bacteria or their extracts and metabolites trigger the innate immune response and activate NF-κB by stimulating TNF-α signaling in epithelial cells (24). Meanwhile, GM-CSF has a potential stimulating function on the expansion and maturation of monocyte-macrophages, dendritic cells, and granulocytes derived from hematopoietic progenitor cells. Thus, we combined lactic acid bacteria and GM-CSF. A recombinant plasmid pPG612-GM-CSF was successfully constructed and introduced into L. johnsonii. A 15 kDa band corresponding to the GM-CSF protein was detected in the cell lysate of recombinant Lc-pPG-GM-CSF, indicating that the bovine GM-CSF was indeed expressed in the recombinant lactic acid bacteria. In addition, other studies have shown that GM-CSF can increase the production of antimicrobial agents, promote phagocytosis, and improve immune function (25–27). These results further suggest the potential protective effect of recombinant L. johnsonii expressing bovine GM-CSF against inflammation.
The immune system plays an important role in maintaining health and is crucial in resisting infections and defending tissue integrity (28, 29). In recent years, the immunomodulatory effects of probiotics as a novel therapeutic tool have been extensively studied (30). Lactic acid bacteria, a common probiotic, has been widely used in clinical settings and demonstrates significant advantages in immune regulation (31). Studies have shown that lactic acid bacteria can affect host immune responses through the production of metabolites such as lactic acid and bacteriocins (32). In addition, the presence of recombinant lactic acid bacteria can inhibit the expression levels of inflammation-related genes and proteins in uterine tissues, regulating immune function (19). We found that recombinant L. johnsonii (Lc-GM-CSF) can effectively prevent the occurrence of uterine infection in mouse models, reduce cytokine expression levels, specifically, lower the cytokines expression levels of clinical cow endometritis significantly, demonstrating a significant therapeutic effect on the disease. Cytokines (IL-1β, IL-6 and TNF-α) are key regulatory factors in the inflammatory response, and their abnormal expression is closely related to the development and progression of inflammation. Our finding indicated that recombinant lactic acid bacteria may play a protective role in endometritis by regulating the expression of these inflammation-related genes.
GM-CSF has a crucial protective role in inflammation-induced postpartum endometritis. When stimulated by LPS, all the extra-cervical cells, intra-cervical cells, and mesenchymal stem cells in amniotic fluid produce GM-CSF (33). Upregulation of GM-CSF in the cervix and uterus was also observed in a mouse model of postpartum endometritis. Importantly, the use of exogenous GM-CSF drugs reduces the incidence of inflammation-induced postpartum endometritis (34). Recombinant GM-CSF lactobacillus, with the dual immunomodulatory effects of lactobacillus and GM-CSF, can reduce the inflammatory response and release of inflammatory mediators in postpartum endometritis by inhibiting the activation and aggregation of neutrophils (35). For example, recombinant GM-CSF lactobacillus may reduce the production of MPO by regulating the activation state of neutrophils (36). In addition, recombinant GM-CSF lactobacillus may inhibit the inflammatory response by regulating the release of NO from neutrophils. NO released by neutrophils can serve as an important inflammatory mediator, and its inhibition may help alleviate the severity of postpartum endometritis (37). Consistent with the above researches, we found that in the recombinant GM-CSF lactobacillus group, both MPO activity and NO concentration in uterine tissue were significantly decreased. This suggests that recombinant lactobacillus may enhance the function of neutrophils, and reduce the inflammatory response, and oxidative stress.
In conclusion, this study elucidates the protective effect of recombinant L. johnsonii expressing bovine GM-CSF against postpartum endometritis (Figure 7). The recombinant plasmid was successfully constructed and introduced into L. johnsonii, resulting in the expression of bovine GM-CSF protein. We found that recombinant L. johnsonii inhibited the expression of inflammation-related genes and proteins in the uterine tissues of the mouse model, suggesting a role in regulating immune function. Furthermore, recombinant L. johnsonii demonstrated a significant therapeutic effect on cow endometritis. However, further studies are needed to advance the potential clinical application of an engineered strain that carries GM-CSF for bovine disease treatment. Nevertheless, our findings provide a new strategy for the prevention and treatment of bovine endometritis.
Figure 7. Protective effects of engineered Lactobacillus johnsonii expressing bovine GM-CSF on bovine postpartum endometritis. We successfully constructed a recombinant Lactobacillus johnsonii strain that expresses bovine granulocyte-macrophage colony-stimulating factor (GM-CSF), which significantly reduced the incidence and inflammation levels of endometritis in a mouse model, as well as exerted beneficial effects on bovine endometritis, presumably through the stimulation of neutrophil function. These findings suggest that the recombinant Lactobacillus johnsonii strain expressing bovine GM-CSF has potential clinical application value for the prevention and treatment of postpartum uterine inflammation.
The original contributions presented in the study are included in the article/supplementary material, further inquiries can be directed to the corresponding authors.
The animal study was approved by Animal Management Committee, Jilin Agricultural University. The study was conducted in accordance with the local legislation and institutional requirements.
JG: Formal analysis, Methodology, Writing – original draft. XC: Formal analysis, Methodology, Writing – original draft. ZL: Project administration, Resources, Writing – review & editing. CW: Project administration, Resources, Writing – review & editing. CZ: Investigation, Methodology, Writing – review & editing. SW: Methodology, Writing – review & editing. ZF: Methodology, Writing – review & editing. JZ: Formal analysis, Writing – review & editing. JW: Investigation, Writing – review & editing. YF: Formal analysis, Writing – review & editing. HL: Investigation, Writing – review & editing. HD: Investigation, Writing – review & editing. XM: Funding acquisition, Writing – review & editing. WL: Funding acquisition, Writing – review & editing.
The author(s) declare that financial support was received for the research, authorship, and/or publication of this article. This work was supported by grant from Science and Technology Development Project of Jilin Province (20210202046NC); National Key R&D Program of China (2021YFF1000701); National Natural Science Foundation of China International Cooperative Research and Exchange Program (31861143014); China Agriculture Research System of MOF and MARA (CARS-37).
The authors declare that the research was conducted in the absence of any commercial or financial relationships that could be construed as a potential conflict of interest.
All claims expressed in this article are solely those of the authors and do not necessarily represent those of their affiliated organizations, or those of the publisher, the editors and the reviewers. Any product that may be evaluated in this article, or claim that may be made by its manufacturer, is not guaranteed or endorsed by the publisher.
1. Garverick, HA. Ovarian follicular cysts in dairy cows. J Dairy Sci. (1997) 80:995–1004. doi: 10.3168/jds.S0022-0302(97)76025-9
2. Genís, S, Bach, À, Fàbregas, F, and Arís, A. Potential of lactic acid bacteria at regulating Escherichia coli infection and inflammation of bovine endometrium. Theriogenology. (2016) 85:625–37. doi: 10.1016/j.theriogenology.2015.09.054
3. Harris, HR, Chavarro, JE, Malspeis, S, Willett, WC, and Missmer, SA. Dairy-food, calcium, magnesium, and vitamin D intake and endometriosis: a prospective cohort study. Am J Epidemiol. (2013) 177:420–30. doi: 10.1093/aje/kws247
4. Zaragoza-Marti, A, Cabrera-Gonzalez, K, Martin-Manchado, L, Moya-Yeste, AM, Sanchez-Sansegundo, M, and Hurtado-Sanchez, JA. The importance of nutrition in the prevention of endometriosis: systematic review. Nutr Hosp. (2023) 40:177–85. doi: 10.20960/nh.04909
5. Pascottini, OB, and LeBlanc, SJ. Modulation of immune function in the bovine uterus peripartum. Theriogenology. (2020) 150:193–200. doi: 10.1016/j.theriogenology.2020.01.042
6. Hammon, DS, Evjen, IM, Dhiman, TR, Goff, JP, and Walters, JL. Neutrophil function and energy status in Holstein cows with uterine health disorders. Vet Immunol Immunopathol. (2006) 113:21–9. doi: 10.1016/j.vetimm.2006.03.022
7. Herath, S, Fischer, DP, Werling, D, Williams, EJ, Lilly, ST, Dobson, H, et al. Expression and function of Toll-like receptor 4 in the endometrial cells of the uterus. Endocrinology. (2006) 147:562–70. doi: 10.1210/en.2005-1113
8. Galvão, KN, Flaminio, MJ, Brittin, SB, Sper, R, Fraga, M, Caixeta, L, et al. Association between uterine disease and indicators of neutrophil and systemic energy status in lactating Holstein cows. J Dairy Sci. (2010) 93:2926–37. doi: 10.3168/jds.2009-2551
9. LeBlanc, SJ. Reproductive tract inflammatory disease in postpartum dairy cows. Animal. (2014) 8:54–63. doi: 10.1017/S1751731114000524
10. Turner, ML, Healey, GD, and Sheldon, IM. Immunity and inflammation in the uterus. Reprod Domest Anim. (2012) 47:402–9. doi: 10.1111/j.1439-0531.2012.02104.x
11. Sheldon, IM, Lewis, GS, LeBlanc, S, and Gilbert, RO. Defining postpartum uterine disease in cattle. Theriogenology. (2006) 65:1516–30. doi: 10.1016/j.theriogenology.2005.08.021
12. Li, C, Chen, C, Kang, X, Zhang, X, Sun, S, Guo, F, et al. Decidua-derived granulocyte macrophage colony-stimulating factor induces polymorphonuclear myeloid-derived suppressor cells from circulating CD15+ neutrophils. Hum Reprod. (2020) 35:2677–91. doi: 10.1093/humrep/deaa217
13. Padilla, L, Martínez-Hernández, J, Barranco, I, Lucas, X, Pastor, LM, Rodriguez-Martínez, H, et al. Granulocyte-macrophage colony stimulating factor (GM-CSF) is fully expressed in the genital tract, seminal plasma and spermatozoa of male pigs. Sci Rep. (2020) 10:13360. doi: 10.1038/s41598-020-70302-9
14. Liu, J, Ying, Y, Wang, S, Li, J, Xu, J, Lv, P, et al. The effects and mechanisms of GM-CSF on endometrial regeneration. Cytokine. (2020) 125:154850. doi: 10.1016/j.cyto.2019.154850
15. Genís, S, Bach, À, and Arís, A. Effects of intravaginal lactic acid bacteria on bovine endometrium: implications in uterine health. Vet Microbiol. (2017) 204:174–9. doi: 10.1016/j.vetmic.2017.04.025
16. Cheng, C, Zhang, L, Mu, J, Tian, Q, Liu, Y, Ma, X, et al. Effect of Lactobacillus johnsonii strain SQ0048 on the TLRs-MyD88/NF-κB signaling pathway in bovine vaginal epithelial cells. Front Vet Sci. (2021) 8:670949. doi: 10.3389/fvets.2021.670949
17. Tarahomjoo, S. Development of vaccine delivery vehicles based on lactic acid bacteria. Mol Biotechnol. (2012) 51:183–99. doi: 10.1007/s12033-011-9450-2
18. Trombert, A. Recombinant lactic acid bacteria as delivery vectors of heterologous antigens: the future of vaccination? Benef Microbes. (2015) 6:313–24. doi: 10.3920/BM2014.0068
19. Genis, S, Sanchez-Chardi, A, Bach, A, Fabregas, F, and Aris, A. A combination of lactic acid bacteria regulates Escherichia coli infection and inflammation of the bovine endometrium. J Dairy Sci. (2017) 100:479–92. doi: 10.3168/jds.2016-11671
20. Ding, H, Wang, Y, Li, Z, Liu, H, Zhao, J, Lu, W, et al. Baogong decoction treats endometritis in mice by regulating uterine microbiota structure and metabolites. Microb Biotechnol. (2022) 15:2786–99. doi: 10.1111/1751-7915.14127
21. Li, Z, Shi, L, Li, Q, Zhao, J, Lu, W, and Wang, J. The expression and bioinformatics analysis of circular RNAs in endometritis mouse uterus tissues. Molecules. (2022) 27:3682. doi: 10.3390/molecules27123682
22. Lindsay, CV, Potter, JA, Grimshaw, AA, Abrahams, VM, and Tong, M. Endometrial responses to bacterial and viral infection: a scoping review. Hum Reprod Update. (2023) 29:675–93. doi: 10.1093/humupd/dmad013
23. Haimerl, P, and Heuwieser, W. Invited review: antibiotic treatment of metritis in dairy cows: a systematic approach. J Dairy Sci. (2014) 97:6649–61. doi: 10.3168/jds.2014-8462
24. Ren, C, Zhang, Q, de Haan, BJ, Zhang, H, Faas, MM, and de Vos, P. Identification of TLR2/TLR6 signalling lactic acid bacteria for supporting immune regulation. Sci Rep. (2016) 6:34561. doi: 10.1038/srep34561
25. Mercer, DK, Francis, ML, and Fraser-Pitt, D. Antimicrobial immunotherapeutics: past, present and future. Emerg Top Life Sci. (2021) 5:609–28. doi: 10.1042/ETLS20200348
26. Sharma, L, Wu, W, Dholakiya, SL, Gorasiya, S, Wu, J, Sitapara, R, et al. Assessment of phagocytic activity of cultured macrophages using fluorescence microscopy and flow cytometry. Methods Mol Biol. (2014) 1172:137–45. doi: 10.1007/978-1-4939-0928-5_12
27. Kumar, A, Taghi Khani, A, Sanchez Ortiz, A, and Swaminathan, S. GM-CSF: a double-edged sword in cancer immunotherapy. Front Immunol. (2022) 13:901277:901277. doi: 10.3389/fimmu.2022.901277
28. Greenwood, SM, and Moran, JJ. Chronic endometritis: morphologic and clinical observations. Obstet Gynecol. (1981) 58:176–84.
29. Sattler, S. The role of the immune system beyond the fight against infection. Adv Exp Med Biol. (2017) 1003:3–14. doi: 10.1007/978-3-319-57613-8_1
30. Schabussova, I, and Wiedermann, U. Lactic acid bacteria as novel adjuvant systems for prevention and treatment of atopic diseases. Curr Opin Allergy Clin Immunol. (2008) 8:557–64. doi: 10.1097/ACI.0b013e328317b88b
31. Mojgani, N, Shahali, Y, and Dadar, M. Immune modulatory capacity of probiotic lactic acid bacteria and applications in vaccine development. Benef Microbes. (2020) 11:213–26. doi: 10.3920/BM2019.0121
32. Anjana,, and Tiwari, SK. Bacteriocin-producing probiotic lactic acid bacteria in controlling dysbiosis of the gut microbiota. Front Cell Infect Microbiol. (2022) 12:851140. doi: 10.3389/fcimb.2022.851140
33. Nold, C, Stone, J, O’Hara, K, Davis, P, Kiveliyk, V, Blanchard, V, et al. Block of granulocyte-macrophage colony-stimulating factor prevents inflammation-induced preterm birth in a mouse model for parturition. Reprod Sci. (2019) 26:551–9. doi: 10.1177/1933719118804420
34. Hussain, AM, and Daniel, RC. Bovine endometritis: current and future alternative therapy. Zentralbl Veterinarmed A. (1991) 38:641–51. doi: 10.1111/j.1439-0442.1991.tb01060.x
35. LeBlanc, SJ. Review: postpartum reproductive disease and fertility in dairy cows. Animal. (2023) 17:100781. doi: 10.1016/j.animal.2023.100781
36. Ramadass, M, Johnson, JL, and Catz, SD. Rab27a regulates GM-CSF-dependent priming of neutrophil exocytosis. J Leukoc Biol. (2017) 101:693–702. doi: 10.1189/jlb.3AB0416-189RR
Keywords: postpartum endometritis, GM-CSF, Lactobacillus johnsonii, protective effects, inflammation
Citation: Guo J, Cao X, Li Z, Wang C, Zhong C, Wang S, Fan Z, Zhao J, Wang J, Fang Y, Liu H, Ding H, Ma X and Lu W (2024) Protective effects of engineered Lactobacillus johnsonii expressing bovine granulocyte-macrophage colony-stimulating factor on bovine postpartum endometritis. Front. Vet. Sci. 11:1418091. doi: 10.3389/fvets.2024.1418091
Received: 03 May 2024; Accepted: 31 July 2024;
Published: 08 August 2024.
Edited by:
Rebecca K. Poole, Texas A&M University, United StatesReviewed by:
Noble K. Kurian, B. S. Abdur Rahman Crescent Institute of Science and Technology, IndiaCopyright © 2024 Guo, Cao, Li, Wang, Zhong, Wang, Fan, Zhao, Wang, Fang, Liu, Ding, Ma and Lu. This is an open-access article distributed under the terms of the Creative Commons Attribution License (CC BY). The use, distribution or reproduction in other forums is permitted, provided the original author(s) and the copyright owner(s) are credited and that the original publication in this journal is cited, in accordance with accepted academic practice. No use, distribution or reproduction is permitted which does not comply with these terms.
*Correspondence: Wenfa Lu, d2VuZmEyMDA0QDE2My5jb20=; Xin Ma, bWF4aW4zMjAyQDE2My5jb20=
†These authors have contributed equally to this work
Disclaimer: All claims expressed in this article are solely those of the authors and do not necessarily represent those of their affiliated organizations, or those of the publisher, the editors and the reviewers. Any product that may be evaluated in this article or claim that may be made by its manufacturer is not guaranteed or endorsed by the publisher.
Research integrity at Frontiers
Learn more about the work of our research integrity team to safeguard the quality of each article we publish.