- 1School of Life Science, Ningxia University, Yinchuan, China
- 2Ningxia Feed Engineering Technology Research Center, Ningxia University, Yinchuan, China
- 3Key Lab of Ministry of Education for Protection and Utilization of Special Biological Resources in Western China, Ningxia University, Yinchuan, China
- 4Agricultural College, Ningxia University, Yinchuan, China
- 5Khon Kaen Univ, Fac Agr, Trop Feed Resources Res & Dev Ctr TROFREC, Dept Anim Sci, Khon Kaen, Thailand
This study was conducted to investigate both fruit and different levels of leaf supplementation on the growth performance, organ indices and intestinal microflora of rats. Twenty-five healthy male Sprague–Dawley rats were randomly divided into five groups. The rats in the control (NC) and positive control (PC) groups were fed by gavage a basal diet and a basal diet with 4 g/kg of L. barbarum fruit homogenate, respectively. The test (LD, MD, and HD) groups were fed basal diets with additional 2, 4, and 8 g/kg of L. barbarum leaf homogenate, respectively. The feeding period was 35 d. The result revealed that the rats in the LD group had the highest average weight gain (p < 0.05). The cardiac and renal indexes in the LD and MD groups were significantly higher than in NC group, respectively (p < 0.05). Diversity analysis revealed that adding low concentrations of L. barbarum leaf homogenates markedly reduced the Shannon index of the rats cecum (p < 0.05). The relative abundance of Verrucomicrobiota was higher in the LD group than those in other groups (p < 0.05). The relative abundance of Actinobacteriota was found significantly higher in PC group than others (p < 0.05). The relative abundance of Akkermansia in LD group was the highest (p < 0.05). The relative abundance of Romboutsia in the PC group was considerably higher than that in other groups. The relative abundance of Candidatus_Saccharimonas in the supplementation groups was appreciably lower than those found in other groups. The relative abundance of Alloprevotella was significantly lower in PC, LD, and MD groups than in NC and HD groups (p < 0.05). The relative abundance of Oscillibacter was significantly higher in HD group than in other groups (p < 0.05). Thus, L. barbarum leaf homogenate fed to rats could increase their growth performance, internal organ weights and additionally enhance the relative abundance of beneficial bacteria. Therefore, based on the obtained data in the current study, a dose of L. barbarum leaf homogenate supplemented with 2 g/kg in diet is recommended, however, further studies are required to confirm, especially in animals.
1 Introduction
Lycium barbarum, a perennial shrub with unique drought-resistant characteristics, is widely distributed in northwestern China. Studies have shown that L. barbarum is rich in several active ingredients, such as L. barbarum polysaccharides (LBP), betaine, polyphenols, amino acids, and trace elements (1), which are crucial for boosting immunity, relieving inflammation, and protecting the liver (2). Furthermore, L. barbarum is widely used in health products, functional foods, and feed additives (3). Compared to its fruit, the leaves have been neglected as a byproduct, with lower utilization levels for a long time (4). However, the chemical composition of L. barbarum leaves in terms of their active ingredients, nutrients, and trace elements is similar to that of its fruits and has the same utility value (4). Recently, L. barbarum leaves are preferred by increasing number of consumers as vegetable, tea, and food ingredients. LBP, the main active ingredient in L. barbarum leaves, has been shown to improve immune responses, maintain the structural integrity of the intestinal tract, increase the relative abundance of beneficial bacteria in the intestinal tract microflora, and enhance animal growth performance (5). Yin et al. (6) showed that adding LBP to the diet improved the structure of intestinal microflora and improved the growth performance and immunity of weaned piglets. Mo et al. (7) showed that adding 2 g/kg LBP to the diet significantly improved the growth performance of grass carp and Nile tilapia. Liang et al. (8) found that supplementing the diet of mice with 200 mg/kg LBP increased the relative abundance of beneficial intestinal bacteria and altered the structure of intestinal microflora. The leaves of L. barbarum have high application potential in livestock and poultry production. Relatively few studies focuse on the supplementation of L. barbarum leaves in diets, with the majority of research concentrating on LBP functional aspects. The use of L. barbarum leaves in animals and their subsequent utilization as a feed source is devoid of theoretical direction, which in turn restricts the industrial application of L. barbarum leaves in production.
This study investigated the effects of different doses of L. barbarum leaf homogenates on the body weight, visceral organ index, and intestinal microorganisms, and further evaluated its effects on rat growth performance, organ development, and intestinal health, with an aim to provide a theoretical basis and technical support for developing and utilizing L. barbarum leaves as a feed resource.
2 Materials and methods
2.1 Test material
Male Sprague–Dawley (SD) rats were purchased from Shaanxi Nuoyou Biotechnology Co. (Xian China). The rats were fed a commercial diet as their basal feed, which was composed of imported fish meal, imported chicken meal, soybean meal, corn, meat and bone meal etc., with the following nutritional values listed on its label: dry matter 90%, crude protein 18%, crude fat 4%, crude fiber 5%, crude ash 8%, calcium 1.0–1.8%, phosphorus 0.6–1.2%. L. barbarum leaves and its fruits were collected in Zhongning County, Zhongwei City, Autonomous Ningxia Hui Region. A homogenate was made by dissolving 720 g of L. barbarum leaves or fruit into 1 L of distilled water, with a concentration of 0.72 g/mL. The freshly harvested L. barbarum leaves and L. barbarum were homogenized in a grinding machine and stored at 4°C (Table 1).
2.2 Experimental design and rat management
Twenty-five healthy male SD rats (5–6 weeks old) were selected and randomly divided into five groups based on similar body weights, and each group had five rats as replicates. The NC and PC groups were administered the basal diet containing 4 g/kg (kg is the weight of the rat) of L. barbarum fruit homogenate by gavage and the rats in LD, MD, and HD groups received a basal diet with 2 g/kg, 4 g/kg, and 8 g/kg of L. barbarum leaves, respectively, which were homogenized by gavage.
Feeding trials were conducted in the Zhixing Building of Ningxia University, where the test site was naturally lit, dried, and ventilated. Each rat in the NC, PC, LD, MD and HD groups received 1 mL (normal saline), 1 mL, 0.5 mL, 1 mL, 2 mL (the positive control group received L. barbarum fruit homogenate, and the experimental group received L. barbarum leaves homogenate by gavage). The experiment was conducted over 35 d. The animals were housed for 7 d for acclimatization and 28 d for the experimental period. Rats were provided water ad libitum, fed, and managed according to routine procedures.
2.3 Sample collection
The rats were weighed on the last day of the entire experiment after fasting for 12 h, anesthetized, and fixed on a dissecting board in a comatose state. Liver, spleen, kidney, thymus, and testis were weighed and washed with pre-cooled saline, and the index of each organ was calculated. The cecum contents were collected in sterile Eppendorf (EP) tubes, rapidly snap-frozen in liquid nitrogen, and then transferred to a − 80°C freezer for later tests.
2.4 Measurement indicators and methods
2.4.1 Growth performance measurement
The initial body weight of the rats was measured on day 1 of the test and the final body weight was also measured on day 28. The amount of food consumed and the amount of feed that remained were used to calculate the daily feed intake. Additionally, the average daily weight gain (ADG), average daily feed intake (ADFI), and ADFI/ADG (F/G) were computed.
ADG = (final weight − initial weight)/number of test days.
ADFI = feed consumption during the feeding period/number of test days.
F/G = ADFI/ADG.
2.4.2 Organ index measurement
The live weight of the rats was recorded at the end of the experiment, and the heart, liver, spleen, kidney, thymus, and testes were aseptically removed after the rats were euthanized under anesthesia. Blood was aspirated using filter paper, and the organ index was calculated (9).
2.4.3 Determining the intestinal microorganisms
The bacterial community structure was characterized using 16S rRNA gene sequencing. The rat cecum contents were collected, stored at −80°C in a freezer, and sent to Novozymes (Beijing, China) for 16S microbial bioinformatics analysis. Polymerase chain reaction (PCR) products were electrophoresed, purified, and mixed on a 2% gel. After the PCR products were completely mixed, PCR was performed and the products were recovered as target band reagents. The samples were clustered with 97% consistency in operational taxonomic unit (OTU) clustering, and the sequence with the highest OTU abundance was selected as the representative sequence for alpha diversity index analysis.
2.5 Statistical analysis
Raw data were processed using Excel 2016, and SPSS 26.0 was used for one-way analysis of variance and Duncan’s method. The correlation analysis of growth performance, organ index, and gut microbiota was investigated using Spearman’s correlation analysis, and p < 0.05 was considered statistically significant.
3 Results
3.1 Effect of Lycium barbarum leaves on the growth performance of rats
The difference in initial weight of rats between the five groups was insignificant (Table 2; p > 0.05). The final weight and ADG of rats in the LD group (338.63 g and 6.25 g/d, respectively) were significantly higher than those in the NC, MD, and PC groups (p < 0.05). The ratio of ADFI and feed weight was the lowest at 5.24; however, the differences between all groups were insignificant (p > 0.05).
3.2 Effect of Lycium barbarum leaves on organ indices of rats
The supplementation of different levels of L. barbarum leaves had markedly affected the cardiac and renal indices of the rats (Table 3; p < 0.05); however, the differences in the indices of the liver, spleen, thymus, and testicular tissues were insignificant (p > 0.05). The cardiac index was significantly higher in the PC and LD groups than that in the NC group (p < 0.05) and tended to decrease progressively with an increase in the amount of L. barbarum leaves supplementation in the diet. The kidney index of rats treated with 4 g/kg L. barbarum leaf homogenate was the highest (p < 0.05) and showed an increasing trend in the beginning, with the increase in the added level of L. barbarum leaves, but rapidly decreased with additional supplementation. The spleen and thymus indices of the rats in the experimental groups showed an upward trend; however, the differences between the groups were insignificant (p > 0.05).
3.3 Effect of Lycium barbarum leaves on the levels of intestinal microorganisms in rats OTU analysis
The OTU Venn diagram obtained by clustering the samples from the five groups revealed 487 identical OTUs (Figure 1). A total of 76 characteristic OTUs, 41 unique OTUs, 79 exclusive OTUs, 66 specific OTUs, and 158 own OTUs were found in the NC, PC, LD, MD, and HD groups, respectively.
3.3.1 Alpha diversity analysis
Figure 2 shows the results of the sparse curve. As the number of sequencing strips increases, the curve becomes flatter, indicating that the amount of sequencing is gradually becomes reasonable. Table 3 shows that the addition of different amounts of L. barbarum leaf homogenates to the basal diet significantly affected the Shannon index of rat gut flora, which was markedly lower in the LD group than in the NC group (p < 0.05) and tended to increase with increasing doses of L. barbarum leaves homogenates in the test groups (p > 0.05; Table 4).
3.3.2 Effect of Lycium barbarum leaves on the structure of intestinal microorganisms in rats
Figure 3 shows that the phylum level results along with an analysis of the compositional structure of the gut microbiota. The dominant bacteria were Firmicutes and Verrucomicrobiota. Table 5 shows that the relative abundance of Verrucomicrobiota was significantly higher in LD than in NC, PC, and HD (p < 0.05), and that the relative abundance of Verrucomicrobiota in the experimental groups rapidly decreased (p < 0.05) with increasing doses of L. barbarum leaves homogenates. The relative abundance of Actinobacteriota was significantly higher in the PC group than in the other groups (p < 0.05). The relative abundance of unidentified bacteria was significantly lower in the LD group than in the PC group (p < 0.05) and increased as more L. barbarum leaves were added.
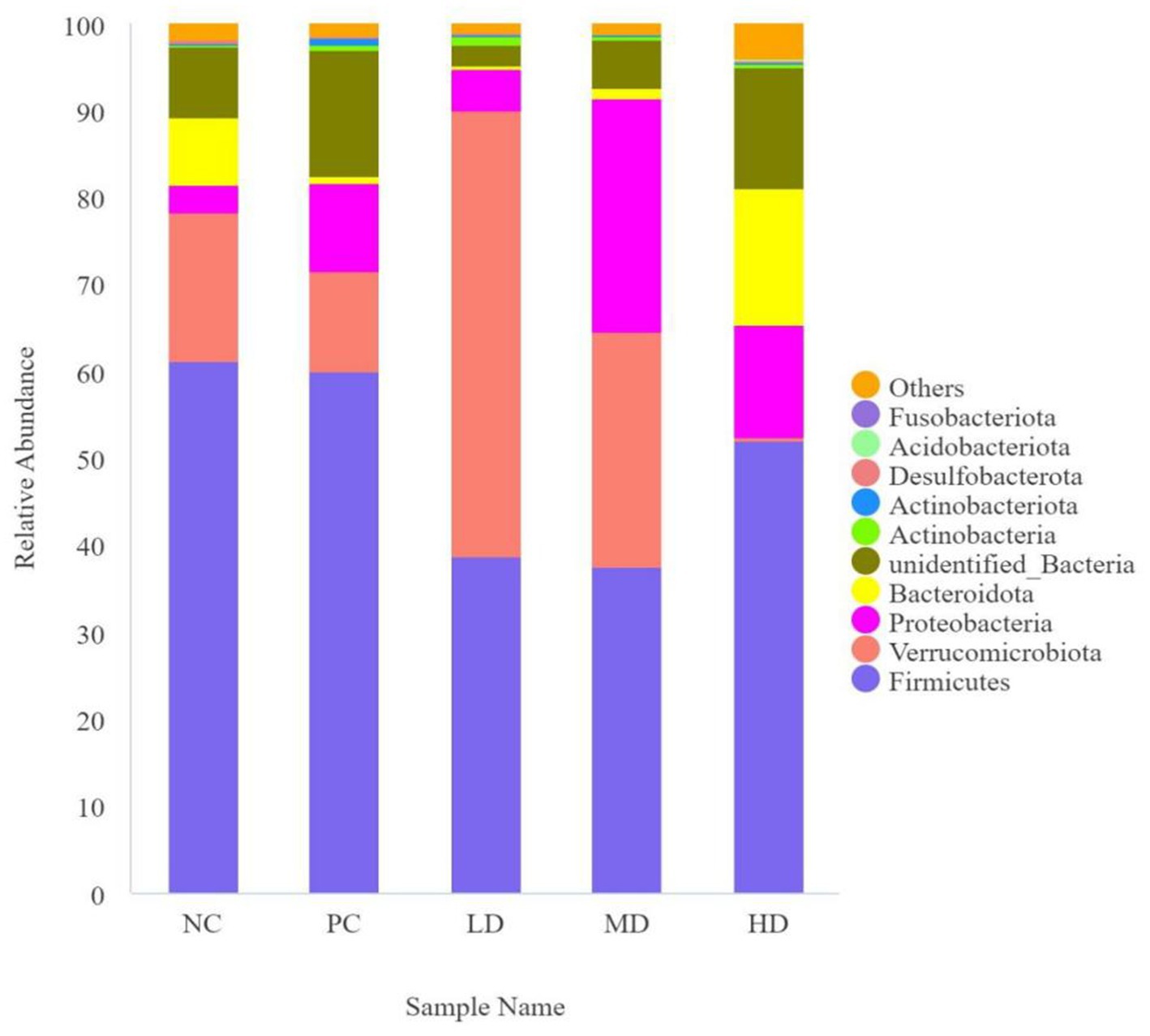
Figure 3. Map of the relative abundance of Lycium barbarum leaf compared to the intestinal flora of different phylum in rats.
Akkermansia and Romboutsia were the dominant genera (Figure 4). Table 6 shows that the LD group had the highest relative abundance of Akkermansia, which was significantly higher than that of the PC group (p < 0.05). The relative abundance of Romboutsia was higher in the PC group than that in the other groups (p < 0.05). The relative abundance of Candidatus_Saccharimonas significantly decreased by the addition of L. barbarum leaves (p < 0.05). The relative abundance of Alloprevotella in the LD, MD, and PC groups rapidly decreased compared with that in the NC and HD groups (p < 0.05). The relative abundance of Oscillibacter was higher in the HD group than that in the other groups (p < 0.05).
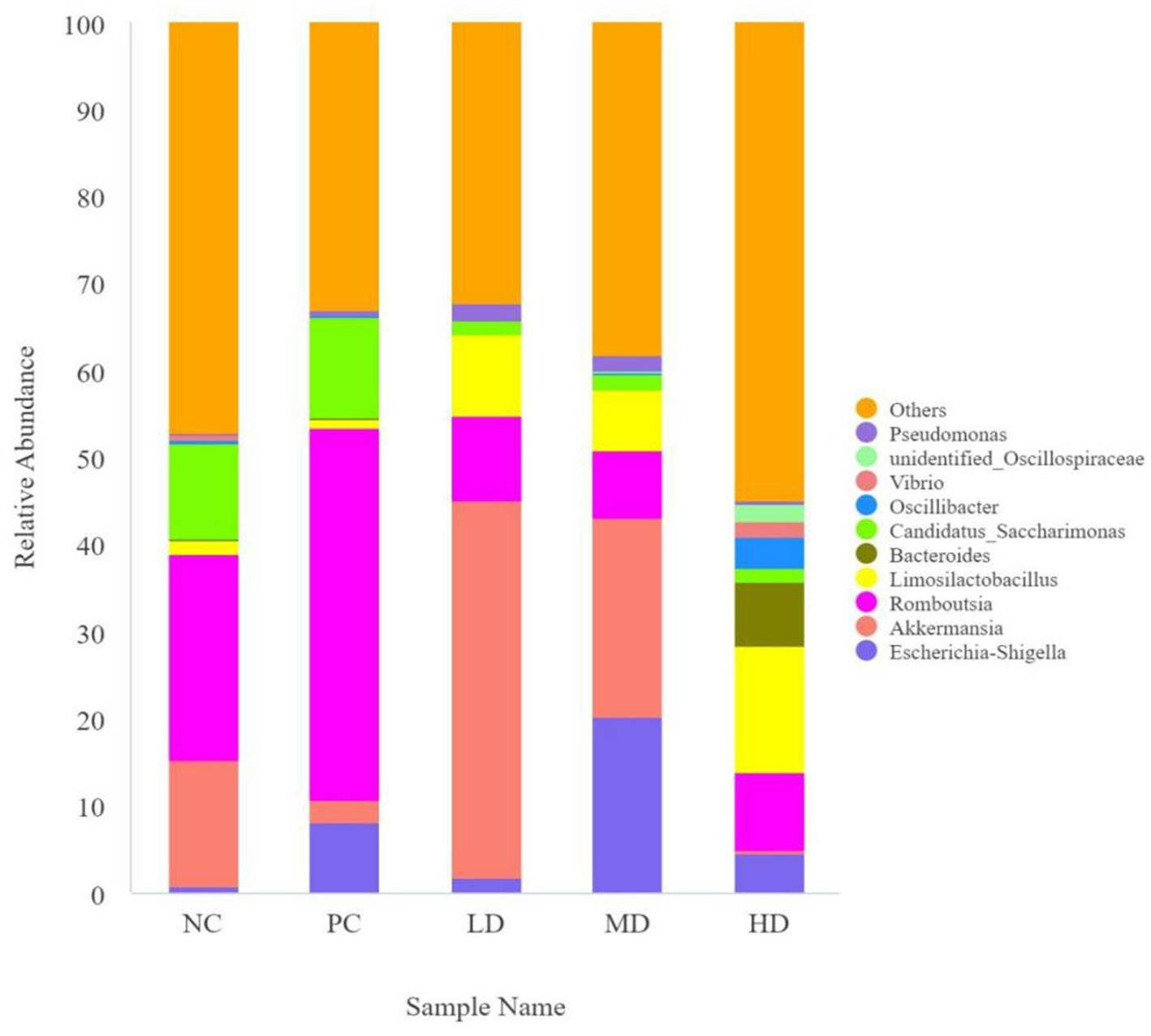
Figure 4. Map of the relative abundance of Lycium barbarum leaves compared to the intestinal flora of different genera in rats.
3.4 Correlation of growth performance, organ index, and gut microbiota
Spearman’s correlation analysis was performed, and heat maps were generated (Figures 5, 6). A correlation was found between the supplementation quantities of L. barbarum leaves and the final weight, daily weight gain, testicular index, spleen index, and intestinal microorganisms at the genus level in rats. Testicular index was negatively correlated with the final weight and ADG (p < 0.01) and positively correlated with F/G (p < 0.01). Bacteroides showed a highly significant positive correlation with spleen index (p < 0.01) and testicular index with Candidatus_Saccharimonas, Alloprevotella, Anaerostipes, and other bacteria (p < 0.05). Notably, the F/G was positively correlated with Alloprevotella, Phascolarctobacterium, and other bacteria (p < 0.05), and the ADFI was significantly and positively correlated with Phascolarctobacterium and other bacteria (p < 0.05). The final weight of the rats was significantly negatively correlated with Anaerostipes, Phascolarctobacterium, and other bacteria (p < 0.01), and daily weight gain was significantly negatively correlated with Alloprevotella, Anaerostipes, Phascolarctobacterium, and other bacteria (p < 0.05).
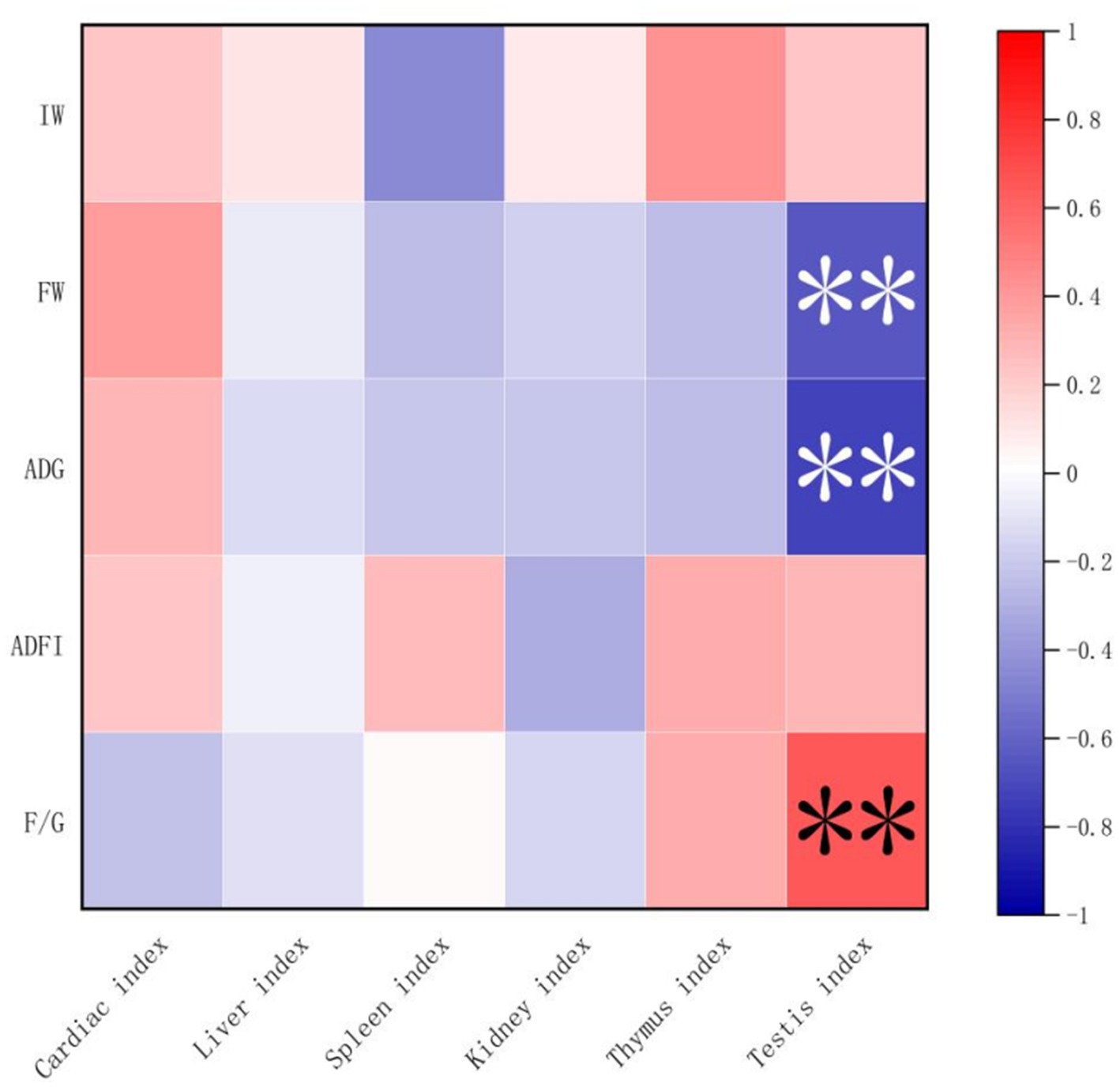
Figure 5. Correlation between growth performance and organ index. IW, initial weight; FW, final weight; ADG, Average daily weight gain; ADFI, Average daily feed intake; F/G, ADFI/ADG. “*” denotes significant correlation, and “**” indicates an extremely significant correlation.
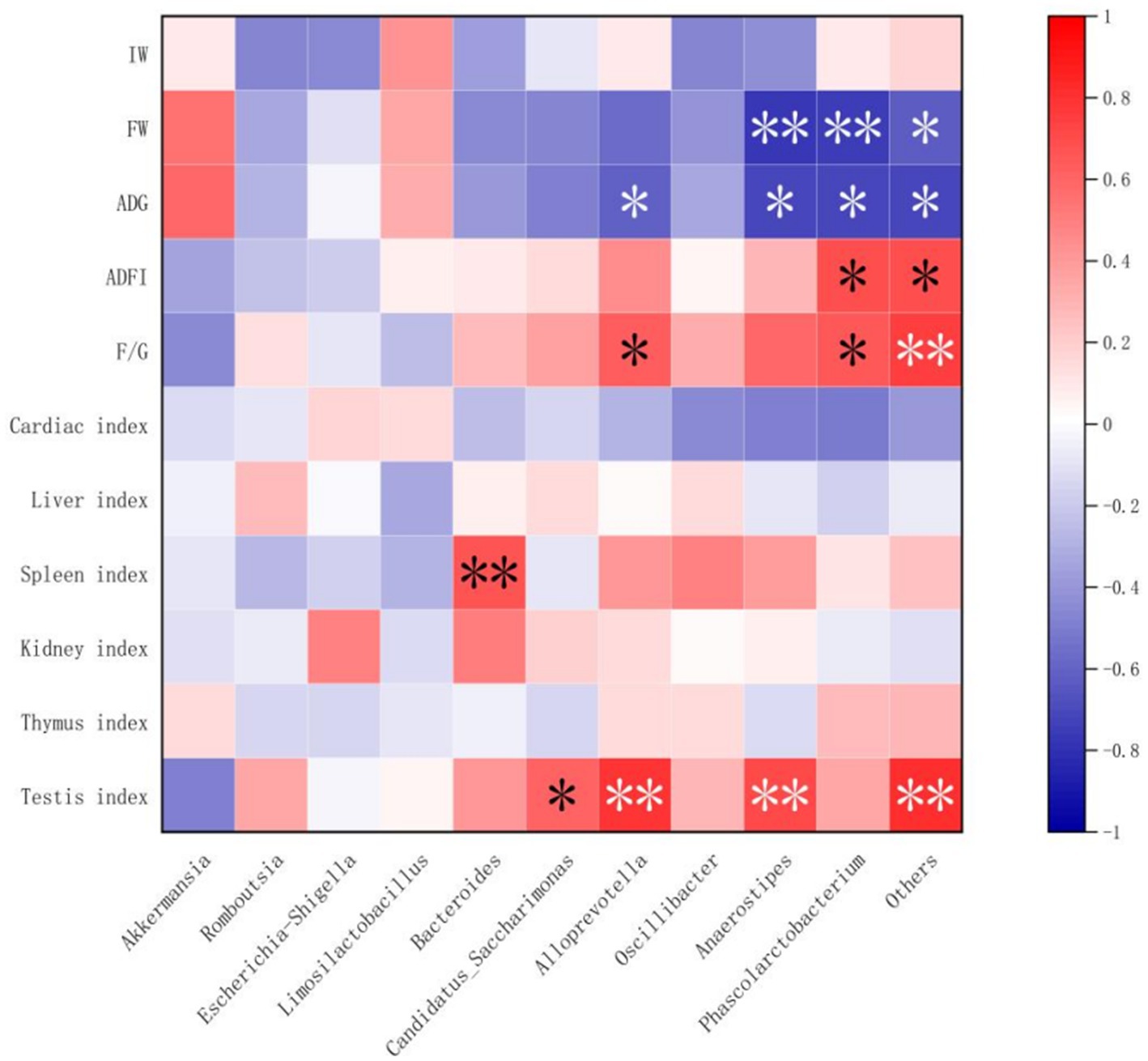
Figure 6. Correlations between growth performance and organ indices and intestinal flora (at genus level). IW, initial weight; FW, final weight; ADG, Average daily weight gain; ADFI, Average daily feed intake; F/G, ADFI/ADG. “*” and “**” denoted extremely significant correlation, respectively.
4 Discussion
Growth performance is an important indicator in animal production, and the average daily gain of animals reflects their growth profile (10). Adding L. barbarum leaves to the animal diet significantly increased the body weight gain of rats in this study. LBP and betaine are the primary active ingredients in L. barbarum leaves (11). Several studies have suggested that active substances such as LBP and betaine enhance animal growth (12). Long et al. (13) found that LBP added to the diets of broilers could increase the ADG and markedly improve growth performance, and recommended a supplementation level of 2000 mg/kg. Zhang et al. (14) found that adding LBP to the diet of Nile tilapia can appreciably increase growth rate, relative length, and body weight gain, and promote growth performance. Chen et al. (15) confirmed that LBP significantly increased the ADG of weaned piglets. Yang et al. (16) suggested that adding betaine to the diet could improve the growth performance in fattening pigs, and the present study showed similar results in improving growth performance compared to those reported by previous studies. The correlation analysis between growth performance and organ weights of rats in this experiment showed a highly significant and negative relationship between the final body weight, daily weight gain, and testicular index, which may be attributed to higher body weight gain than other reproductive organs in later growth stages. Zhou et al. (17) previously reported similar results. Adding L. barbarum leaves to diets has been shown to improve animal growth and increase the weight of immune organs. Zhao et al. (18) reported that dietary supplementation with 0.2% LBP enhances the abundance of beneficial gut bacteria by improving the activity of the intestinal microbiome, thus promoting growth performance of animals. Liu et al. (19) found that LBP promoted broiler growth performance by enhancing immune function. Ma et al. (20) showed that betaine stimulates glucose utilization in animal muscle cells, thereby improving growth performance. The present study examined the variation in the structure of the cecum flora of rats after adding different doses of L. barbarum leaf homogenates using the Illumina MiSeq sequencing platform and using 16S rRNA sequencing technology. However, the interaction between intestinal microorganisms and functional substances in LBP is unknown, and further studies are required to understand the specific molecular mechanisms.
The organ index reflects the development and functioning of animals; the higher the relative weight of an animal organ, stronger its function (21). In the present study, adding L. barbarum leaves sharply increased cardiac and renal indices in rats probably because betaine in the leaves increased growth factor levels in the blood of rats, promoted heart development, and reduced kidney inflammation. Fiorito et al. (22) reported that betaine protects and improves the functions of internal organs. Yu et al. (23) found that LBP has a protective effect on the kidneys. Xie et al. (24) showed that 400 mg/kg LBP reduced kidney inflammation and renal tissue apoptosis in mice by activating the nuclear factor E2-related factor 2 and oxygenase-1 (HO-1) signaling pathways, thereby improving the immune capacity of rats. Deng et al. (25) found that adding LBP to the diet had a consistent effect on the spleen index in mice, consistent with that of previous studies. This is likely because of LBP’s ability to alleviate the inflammatory response and increase antibody counts that ultimately strengthens humoral immunity. Ruo et al. (26) reported that LBP regulates the development and differentiation of immune cells, such as T lymphocytes, B lymphocytes, macrophages, and dendritic cells and downregulates the inflammatory immune response, and inhibits the secretion of pro-inflammatory cytokines. Additionally, dietary LBP has been shown to increase the activity of antioxidant liver enzymes and their gene expression, reduce liver inflammation, increase antibody production, and improve immune function (27). Ding et al. (28) demonstrated that LBP improves immune organ function by modulating intestinal microbiota structure. In this study, the spleen index was significantly and positively correlated with Bacteroides. The spleen is the largest secondary lymphoid organ and performs various immune functions (29). Stimulation of multiple immune cells or multiple pathways may indirectly activate the NF-κB signaling pathway to promote humoral and cellular immunity (30). Bacteroides primarily produce short-chain fatty acids (SCFAs) that can regulate the immune function of rats by increasing mucoprotein 2 expression (31). Liu et al. (19) found that adding 4 g/kg LBP to the diet markedly increased the immune organ index in broiler chickens, which is inconsistent with the results of this study and may be related to the LBP dosage in L. barbarum leaves and the animal species used in the study.
The large number of bacteria in the intestinal tract of animals plays a key role in intestinal and host health and can affect the growth, development, nutrient absorption, and immunity of animals (32). The diversity of intestinal flora is closely related to intestinal homeostasis, and the higher the index of flora diversity, the more difficult it is to destroy balance of flora balance (33). However, the composition of the intestinal microbial community is highly dependent on diet (34). Additionally, Fang et al. (35) reported that adding LBP changed the structure of the intestinal microbiota in mice, increased the abundance of beneficial bacteria, and inhibited the proliferation of harmful bacteria, which were similar to the results of the present study when L. barbarum leaves were used at different concentrations; however, the distribution pattern and stability of the intestinal microorganisms were disturbed. Sun et al. (21) added different LBP values to fish diets and found that the Shannon index showed an upward trend with increasing LBP supplementation dose, which is consistent with the results of this study. However, the Shannon index was significantly lower in the LD group, possibly because the LBP content was lower in the low-dose homogenate of L. barbarum leaves, which inhibited the growth of some gut microbiota. Sun et al. (21) found that the diversity of intestinal microorganisms (Chao1 and Simpson) in cultured fish slightly decreased with the addition of different levels of LBP to the diet, which is consistent with the results of the present study. In conclusion, infusion of L. barbarum leaves in rats slightly reduced the diversity of the gut microbiome.
The predominant bacterial phyla in the rat cecum were Firmicutes and Verrucomicrobiota, which is in agreement with the findings of Ding et al. (36). In the present study, the relative abundance of Verrucomicrobiota in the intestinal tract of rats was significantly increased by adding low-dose homogenate of L. barbarum leaves. Bai et al. (37) found that administering 150 mg/kg of L. barbarum polysaccharides to leaves significantly increased the relative abundance of Verrucomicrobiota, which is consistent with the results of the present study. Verrucomicrobiota is a potentially beneficial bacterium that can utilize gastrointestinal mucin as an energy source and protect the gut from pathogens by increasing the number of mucin-producing goblet cells in rats (38, 39). The relative abundance of Actinobacteriota was markedly increased in the PC group. The results of Cao et al. (40) were consistent with the results of the present study, which may be related to the higher LBP concentration in L. barbarum leaves. The addition of different concentrations of the L. barbarum leaf homogenates increased the relative abundance of Bacteroidota. Ding et al. (28) fed cyclophosphamide-treated mouse LBP and observed an increase in the relative abundance of Bacteroidota in the mouse intestine, which was similar to the results obtained by Cui et al. (35). Similar results were found in the present study. Bacteroidota, the main bacterium involved in SCFA production, is an anaerobic gram-negative bacterium that improves host nutrient digestion and absorption by improving carbohydrate metabolism (41). The relative abundance of unidentified_Bacteria was rapidly reduced with low homogenate concentrations of L. barbarum leaves, which may be because unidentified bacteria inhibit the growth of active ingredients found in L. barbarum leaves, and the specific molecular mechanism of inhibition requires further study. The addition of high concentrations of L. barbarum leaf homogenate markedly increased the abundance of other bacteria, probably because of some specific active components in the high L. barbarum leaf homogenate group, which additionally favor bacterial growth.
Akkermansia and Romboutsia were the dominant genera, and the relative abundance of Akkermansia in the 2 g/kg LD group significantly increased. This may be related to the presence of LBP in L. barbarum leaves. Xu et al. (42) found an increase in the relative abundance of Akkermansia when rats were fed by gavage with leaves of different LBP, which is consistent with the results of the present study. LBP have been found to contribute to the colonization of beneficial microorganisms in the intestinal tract, improve intestinal barrier function, alleviate intestinal permeability, and maintain intestinal health (43). Beneficial bacteria in appropriate quantities contributes to the maintenance of the intestinal epithelial barrier and modulate immune homeostasis by competitively inhibiting pathogens and producing antimicrobial compounds, thereby reducing inflammation caused by harmful intestinal bacteria (44). Akkermansia regulates mucus thickness, intestinal barrier integrity, and immune function and is a beneficial bacterium that promotes health and has a positive effect on regulating host metabolic disorders (45). The abundance of Romboutsia and Candidatus_Saccharimonas was significantly lower in the MD group than in the PC group, which may be associated with the presence of LBP in the leaves. Liu et al. (46) reported that dietary supplementation of 400 mg/kg LBP significantly reduced the relative abundance of Romboutsia bacteria in the rat intestines, which was consistent with the results of the present study. Romboutsia ensures a healthy state in the intestine and is abundant in the healthy intestinal mucosa (47, 48). However, rats with acute necrotizing pancreatitis exhibit reduced relative abundance of Candidatus_Saccharimonas, suggesting that it plays a crucial role in maintaining normal intestinal function (49). In the present study, the relative abundance of Candidatus_Saccharimonas rapidly decreased possibly because some active components in L. barbarum leaves inhibited the growth of Candidatus_Saccharimonas. Specific functional substances need to be further studied. The relative abundance of Alloprevotella was significantly higher in the HD group than in the PC group, which may be related to the carbohydrate content of L. barbarum leaves. Wu et al. (50) showed that the abundance of Alloprevotella correlate with the addition of high-carbohydrate diets, which could be used to improve digestion in animals. The relative abundance of Oscillibacter was significantly higher in the HD group than in the other groups. Oscillibacter is involved in preventing inflammation by breaking down polysaccharides into butyrate. Furthermore, it exerts additional protective effects on the host by modulating abundant acid-producing bacteria to influence SCFA content in the rat intestine (51). Zhou et al. (52) reported that adding LBP to the diet significantly affected bacterial growth and secondary metabolite synthesis by influencing the structure of intestinal microflora. A link between the presence of certain beneficial bacteria in the animal guts and growth performance has previously been elucidated (53). Correlation analysis showed that the final weight and daily weight gain of the rats were significantly negatively correlated with Anaerostipes, Alloprevotella, and Phascolarctobacterium. The F/G ratio and daily food intake of the rats showed a significant positive correlation with Phascolarctobacterium and Alloprevotella. Phascolarctobacterium has been reported to produce SCFA, which is critical in regulating metabolic balance in the intestine. This genus is commonly found in the intestine and is beneficial to organisms (54). Zhang et al. (55) reported that the gram-negative bacteria Anaerostipes, Phascolarctobacterium, and Alloprevotella can aggravate intestinal inflammation, destroy intestinal structure, affect nutrient absorption, and ultimately reduce growth performance. Furthermore, butyrate has been reported to store 10–15% of the energy required by the host (56). A high butyrate concentration can have the opposite effect and damage the integrity of the intestinal barrier (57). In the present study, the growth performance of rats was significantly negatively correlated with the presence of Alloprevotella, Anaerostipes, and Phascolarctobacterium. In addition, daily food intake of rats was significantly positively correlated with the presence of some unclassified bacteria among other bacteria, that can promote nutrient digestion and absorption and thus improve the growth performance of rats.
5 Conclusion
Dietary supplementation with L. barbarum leaves in the diet improved the structure of intestinal flora, increased the relative abundance of beneficial bacteria in the gut microbiota, and enhanced the growth performance and organ weight in rats. The recommended dietary supplement level of L. barbarum leaves is 2 g/kg. However, rats were used as an experimental model in this study, and their application in animal production requires further studies especially in animals such as poultry and swine. In addition, the functional mechanism of L. barbarum leaves needs more investigation to provide a theoretical basis for its future application.
Data availability statement
The original contributions presented in the study are publicly available. This data can be found here: China National Center for Bioinformation (CNCB), PRJCA028224.
Ethics statement
The animal study was approved by Attitude of Ningxia University Technology Ethics Committee. The study was conducted in accordance with the local legislation and institutional requirements.
Author contributions
YG: Conceptualization, Data curation, Formal analysis, Investigation, Methodology, Writing – original draft, Writing – review & editing. JL: Conceptualization, Formal analysis, Supervision, Writing – review & editing. QT: Conceptualization, Formal analysis, Supervision, Writing – review & editing. DZ: Conceptualization, Supervision, Writing – review & editing. MW: Supervision, Writing – review & editing. GX: Conceptualization, Funding acquisition, Supervision, Writing – review & editing.
Funding
The author(s) declare that financial support was received for the research, authorship, and/or publication of this article. This research was supported by the Ningxia Key Research and Development Project (Yinchuan, China) [grant number 2023BCF01037].
Conflict of interest
The authors declare that the research was conducted in the absence of any commercial or financial relationships that could be construed as a potential conflict of interest.
Publisher’s note
All claims expressed in this article are solely those of the authors and do not necessarily represent those of their affiliated organizations, or those of the publisher, the editors and the reviewers. Any product that may be evaluated in this article, or claim that may be made by its manufacturer, is not guaranteed or endorsed by the publisher.
References
1. Ma, RH, Zhang, XX, Thakur, K, Zhang, JG, and Wei, ZJ. Research progress of lycium barbarum l. as functional food: phytochemical composition and health benefits. Curr Opin Food Sci. (2022) 47:100871. doi: 10.1016/j.cofs.2022.100871
2. Jin, M, Huang, Q, Zhao, K, and Shang, P. Biological activities and potential health benefit effects of polysaccharides isolated from lycium barbarum l. Int J Biol Macromol. (2013) 54:16–23. doi: 10.1016/j.ijbiomac.2012.11.023
3. Agradi, S, Draghi, S, Cotozzolo, E, Barbato, O, Castrica, M, Quattrone, A, et al. Goji berries supplementation in the diet of rabbits and other livestock animals: a mini-review of the current knowledge. Front Vet Sci. (2022) 8:823589. doi: 10.3389/fvets.2021.823589
4. Lei, ZL, Chen, XQ, Cao, FL, Guo, QR, and Wang, JH. Phytochemicals and bioactivities of goji (lycium barbarum l. And lycium chinense mill.) leaves and their potential applications in the food industry: a review. Int J Food Sci Technol. (2022) 57:1451–61. doi: 10.1111/ijfs.15507
5. Li, XL, He, LP, Yang, Y, Liu, FJ, Cao, Y, and Zuo, JJ. Effects of extracellular polysaccharides of ganoderma lucidum supplementation on the growth performance, blood profile, and meat quality in finisher pigs. Livest Sci. (2015) 178:187–94. doi: 10.1016/j.livsci.2015.04.001
6. Yin, YX, Wang, F, Yang, M, Tan, B, Yin, YL, Chen, JS, et al. Lycium barbarum polysaccharides as antibiotic substitutes improve growth performance, serum immunity, antioxidant status, and intestinal health for weaned piglets. Front Microbiol. (2022) 12:819993. doi: 10.3389/fmicb.2021.819993
7. Mo, WY, Lun, C, Choi, WM, Man, YB, and Wong, MH. Enhancing growth and non-specific immunity of grass carp and nile tilapia by incorporating chinese herbs (astragalus membranaceus and lycium barbarum) into food waste based pellets. Environ Pollut. (2016) 219:475–82. doi: 10.1016/j.envpol.2016.05.055
8. Liang, J, Li, X, Lei, W, Tan, P, Han, M, Li, H, et al. Serum metabolomics combined with 16s rrna sequencing to reveal the effects of lycium barbarum polysaccharide on host metabolism and gut microbiota. Food Res Int. (2023) 165:112563. doi: 10.1016/j.foodres.2023.112563
9. Ghalamkari, G, Toghyani, M, Tavalaeian, E, Landy, N, Ghalamkari, Z, and Radnezhad, H. Efficiency of different levels of satureja hortensis l. (savory) in comparison with an antibiotic growth promoter on performance, carcass traits, immune responses and serum biochemical parameters in broiler chickens. Afr J Biotechnol. (2011) 10:13318–23. doi: 10.5897/AJB11.911
10. Hao, C, Gao, Q, Zhang, J, Muhammad, I, Waqar, K, and Xin, G. Effects of grape residue supplementation in diet on liangfeng chicken growth performance, nutrient metabolism and blood biochemistry. J Appl Anim Res. (2022) 50:567–73. doi: 10.1080/09712119.2022.2113083
11. Varoni, MV, Pasciu, V, Gadau, SD, Baralla, E, Serra, E, Palomba, D, et al. Possible antioxidant effect of lycium barbarum polysaccharides on hepatic cadmium-induced oxidative stress in rats. Environ Sci Pollut Res. (2017) 24:2946–55. doi: 10.1007/s11356-016-8050-x
12. Feng, YQ, Song, YT, Zhou, J, Duan, YQ, Kong, TY, Ma, HL, et al. Recent progress of lycium barbarum polysaccharides on intestinal microbiota, microbial metabolites and health: a review. Crit Rev Food Sci Nutr. (2022) 64:2917–2940. doi: 10.1080/10408398.2022.2128037
13. Long, LN, Kang, BJ, Jiang, Q, and Chen, JS. Effects of dietary lycium barbarum polysaccharides on growth performance, digestive enzyme activities, antioxidant status, and immunity of broiler chickens. Poult Sci. (2020) 99:744–51. doi: 10.1016/j.psj.2019.10.043
14. Zhang, X, Huang, K, Zhong, H, Ma, YQ, Guo, ZB, Tang, ZY, et al. Effects of lycium barbarum polysaccharides on immunological parameters, apoptosis, and growth performance of nile tilapia (oreochromis niloticus). Fish Shellfish Immunol. (2020) 97:509–14. doi: 10.1016/j.fsi.2019.12.068
15. Chen, JS, Long, LN, Jiang, Q, Kang, BJ, Li, YH, and Yin, J. Effects of dietary supplementation oflycium barbarum polysaccharides on growth performance, immune status, antioxidant capacity and selected microbial populations of weaned piglets. J Anim Physiol Anim Nutr. (2020) 104:1106–15. doi: 10.1111/jpn.13247
16. Yang, HS, Lee, JI, Joo, ST, and Park, GB. Effects of dietary glycine betaine on growth and pork quality of finishing pigs. Asian Australas J Anim Sci. (2009) 22:706–11. doi: 10.5713/ajas.2009.80645
17. Zhou, WT, Yang, TT, Xu, WQ, Huang, YJ, Ran, LW, Yan, YM, et al. The polysaccharides from the fruits of lycium barbarum l. confer anti-diabetic effect by regulating gut microbiota and intestinal barrier. Carbohydr Polym. (2022) 291:119626. doi: 10.1016/j.carbpol.2022.119626
18. Zhao, QH, Li, JJ, Yan, J, Liu, S, Guo, YL, Chen, DJ, et al. Lycium barbarum polysaccharides ameliorates renal injury and inflammatory reaction in alloxan-induced diabetic nephropathy rabbits. Life Sci. (2016) 157:82–90. doi: 10.1016/j.lfs.2016.05.045
19. Liu, YL, Yin, RQ, Liang, SS, Duan, YL, Yao, JH, Duan, YL, et al. Effect of dietary lycium barbarum polysaccharide on growth performance and immune function of broilers. J Appl Poult Res. (2017) 26:200–8. doi: 10.3382/japr/pfw063
20. Ma, JN, Meng, XL, Kang, SY, Zhang, J, Jung, HW, and Park, YK. Regulatory effects of the fruit extract of lycium chinense and its active compound, betaine, on muscle differentiation and mitochondrial biogenesis in c2c12 cells. Biomed Pharmacother. (2019) 118:109297. doi: 10.1016/j.biopha.2019.109297
21. Sun, S, Li, B, Wu, MM, Deng, YF, Li, J, Xiong, YJ, et al. Effect of dietary supplemental vitamin c and betaine on the growth performance, humoral immunity, immune organ index, and antioxidant status of broilers under heat stress. Trop Anim Health Prod. (2023) 55:96. doi: 10.1007/s11250-023-03500-y
22. Fiorito, S, Preziuso, F, Epifano, F, Scotti, L, Bucciarelli, T, Taddeo, VA, et al. Novel biologically active principles from spinach, goji and quinoa. Food Chem. (2019) 276:262–5. doi: 10.1016/j.foodchem.2018.10.018
23. Yu, X, Zhang, L, Zhang, P, Zhi, J, Xing, RN, and He, LQ. Lycium barbarumpolysaccharides protect mice from hyperuricaemia through promoting kidney excretion of uric acid and inhibiting liver xanthine oxidase. Pharm Biol. (2020) 58:944–9. doi: 10.1080/13880209.2020.1817951
24. Xie, W, Huang, YY, Chen, HG, and Zhou, X. Study on the efficacy and mechanism of lycium barbarum polysaccharide against lead-induced renal injury in mice. Nutrients. (2021) 13:2945. doi: 10.3390/nu13092945
25. Deng, XL, Luo, S, Luo, X, Hu, MH, Ma, FL, Wang, YY, et al. Polysaccharides from chinese herbal lycium barbarum induced systemic and local immune responses in H22 tumor-bearing mice. J Immunol Res. (2018) 2018:1–12. doi: 10.1155/2018/3431782
26. Bo, RN, Liu, ZG, Zhang, J, Gu, PF, Ou, N, Sun, YQ, et al. Mechanism of lycium barbarum polysaccharides liposomes on activating murine dendritic cells. Carbohydr Polym. (2019) 205:540–9. doi: 10.1016/j.carbpol.2018.10.057
27. Tan, XH, Sun, ZZ, Ye, CX, and Lin, HZ. The effects of dietary lycium barbarum extract on growth performance, liver health and immune related genes expression in hybrid grouper (epinephelus lanceolatus male x e. Fuscoguttatus female) fed high lipid diets. Fish Shellfish Immunol. (2019) 87:847–52. doi: 10.1016/j.fsi.2019.02.016
28. Ding, Y, Yan, YM, Chen, D, Ran, LW, Mi, J, Lu, L, et al. Modulating effects of polysaccharides from the fruits of lycium barbarum on the immune response and gut microbiota in cyclophosphamide-treated mice. Food Funct. (2019) 10:3671–83. doi: 10.1039/c9fo00638a
29. Liu, YM, Huang, WK, Dai, K, Liu, N, Wang, JQ, Lu, XY, et al. Inflammatory response of gut, spleen, and liver in mice induced by orally administered porphyromonas gingivalis. J Oral Microbiol. (2022) 14:2088936. doi: 10.1080/20002297.2022.2088936
30. Gong, GP, Dang, TT, Deng, YN, Han, JL, Zou, ZH, Jing, S, et al. Physicochemical properties and biological activities of polysaccharides from lycium barbarum prepared by fractional precipitation. Int J Biol Macromol. (2018) 109:611–8. doi: 10.1016/j.ijbiomac.2017.12.017
31. Xie, HL, Fang, JY, Farag, MA, Li, ZH, Sun, PL, and Shao, P. Dendrobium officinale leaf polysaccharides regulation of immune response and gut microbiota composition in cyclophosphamide-treated mice. Food Chem X. (2022) 13:100235. doi: 10.1016/j.fochx.2022.100235
32. Yang, YX, Dai, ZL, and Zhu, WY. Important impacts of intestinal bacteria on utilization of dietary amino acids in pigs. Amino Acids. (2014) 46:2489–501. doi: 10.1007/s00726-014-1807-y
33. Forno, E, Onderdonk, AB, McCracken, J, Litonjua, AA, Laskey, D, Delaney, ML, et al. Diversity of the gut microbiota and eczema in early life. Clin Mol Allergy. (2008) 6:11. doi: 10.1186/1476-7961-6-11
34. Zhang, J, Wang, W, Wang, Y, Hu, H, Yu, B, Zhou, Z, et al. Modulation of broiler plasma metabolic spectrum by the addition of lysine residue to the diet. J Anim Physiol Anim Nutr. (2022) 106:1072–85. doi: 10.1111/jpn.13627
35. Cui, F, Shi, CL, Zhou, XJ, Wen, W, Gao, XP, Wang, LY, et al. Lycium barbarum polysaccharide extracted from lycium barbarum leaves ameliorates asthma in mice by reducing inflammation and modulating gut microbiota. J Med Food. (2020) 23:699–710. doi: 10.1089/jmf.2019.4544
36. Ding, Y, Yan, YM, Peng, YJ, Chen, D, Mi, J, Lu, L, et al. In vitro digestion under simulated saliva, gastric and small intestinal conditions and fermentation by human gut microbiota of polysaccharides from the fruits of lycium barbarum. Int J Biol Macromol. (2019) 125:751–60. doi: 10.1016/j.ijbiomac.2018.12.081
37. Min, B, Zhang, Y, Li, Q, Ji, J, Jin, Y, And Wang, QZ, et al. Effect of lycium barbarum leaves polysaccharides on biological metabolism, antioxidant capacity and immune function in mice. Sci Technol Food Ind. (2023) 413-19. doi: 10.13386/j.issn1002-0306.2022110055
38. Shin, NR, Lee, JC, Lee, HY, Kim, MS, Whon, TW, Lee, MS, et al. An increase in the Akkermansia spp. population induced by metformin treatment improves glucose homeostasis in diet-induced obese mice. Gut. (2014) 63:727–35. doi: 10.1136/gutjnl-2012-303839
39. Zhou, K . Strategies to promote abundance of Akkermansia muciniphila, an emerging probiotics in the gut, evidence from dietary intervention studies. J Funct Foods. (2017) 33:194–201. doi: 10.1016/j.jff.2017.03.045
40. Cao, C, Wang, ZF, Gong, GP, Huang, WQ, Huang, LJ, Song, S, et al. Effects of lycium barbarum polysaccharides on immunity and metabolic syndrome associated with the modulation of gut microbiota: a review. Food Secur. (2022) 11:3177. doi: 10.3390/foods11203177
41. Rios-Covian, D, Salazar, N, Gueimonde, M, and de Los Reyes-Gavilan, CG. Shaping the metabolism of intestinal bacteroides population through diet to improve human health. Front Microbiol. (2017) 8:6. doi: 10.3389/fmicb.2017.00376
42. Xu, T, Ge, Y, Du, H, Li, Q, Xu, X, Yi, H, et al. Berberis kansuensis extract alleviates type 2 diabetes in rats by regulating gut microbiota composition. J Ethnopharmacol. (2021) 273:113995. doi: 10.1016/j.jep.2021.113995
43. Sun, Y, Meng, XW, Hu, XW, Liu, R, Zhao, ZG, Wang, SH, et al. Dietary supplementation with lycium barbarum polysaccharides conducive to maintaining the health of luciobarbus capito via the enhancement of enzyme activities and the modulation of gut microbiota. Int J Biol Macromol. (2023) 232:123500. doi: 10.1016/j.ijbiomac.2023.123500
44. van Zyl, WF, Deane, SM, and Dicks, L. Molecular insights into probiotic mechanisms of action employed against intestinal pathogenic bacteria. Gut Microbes. (2020) 12:1831339. doi: 10.1080/19490976.2020.1831339
45. Derrien, M, Belzer, C, and de Vos, WM. Akkermansia muciniphila and its role in regulating host functions. Microb Pathog. (2017) 106:171–81. doi: 10.1016/j.micpath.2016.02.005
46. Liu, Y, Liu, L, Luo, JM, and Peng, XC. Metabolites from specific intestinal bacteria in vivo fermenting lycium barbarum polysaccharide improve collagenous arthritis in rats. Int J Biol Macromol. (2023) 226:1455–67. doi: 10.1016/j.ijbiomac.2022.11.257
47. Li, ZR, Jia, RB, Wu, J, Lin, L, Ou, ZR, Liao, B, et al. Sargassum fusiforme polysaccharide partly replaces acarbose against type 2 diabetes in rats. Int J Biol Macromol. (2021) 170:447–58. doi: 10.1016/j.ijbiomac.2020.12.126
48. Mangifesta, M, Mancabelli, L, Milani, C, Gaiani, F, De'Angelis, N, De'Angelis, GL, et al. Mucosal microbiota of intestinal polyps reveals putative biomarkers of colorectal cancer. Sci Rep. (2018) 8:13974. doi: 10.1038/s41598-018-32413-2
49. Xie, YR, Sun, J, Hu, CQ, Ruan, B, and Zhu, B. Oral microbiota is associated with immune recovery in human immunodeficiency virus-infected individuals. Front Microbiol. (2021) 12:794746. doi: 10.3389/fmicb.2021.794746
50. Wu, GD, Chen, J, Hoffmann, C, Bittinger, K, Chen, YY, Keilbaugh, SA, et al. Linking long-term dietary patterns with gut microbial enterotypes. Science. (2011) 334:105–8. doi: 10.1126/science.1208344
51. Leth, ML, Ejby, M, Workman, C, Ewald, DA, Pedersen, SS, Sternberg, C, et al. Differential bacterial capture and transport preferences facilitate co-growth on dietary xylan in the human gut. Nat Microbiol. (2018) 3:570–80. doi: 10.1038/s41564-018-0132-8
52. Zhou, F, Jiang, XY, Wang, T, Zhang, BL, and Zhao, HF. Lycium barbarum polysaccharide (lbp): a novel prebiotics candidate for bifidobacterium and lactobacillus. Front Microbiol. (2018) 9:1034. doi: 10.3389/fmicb.2018.01034
53. Zheng, ML, Mao, PC, Tian, XX, Guo, Q, and Meng, L. Effects of dietary supplementation of alfalfa meal on growth performance, carcass characteristics, meat and egg quality, and intestinal microbiota in Beijing-you chicken. Poult Sci. (2019) 98:2250–9. doi: 10.3382/ps/pey550
54. Wu, FF, Guo, XF, Zhang, JC, Zhang, M, Ou, ZH, and Peng, YZ. Phascolarctobacterium faecium abundant colonization in human gastrointestinal tract. Exp Ther Med. (2017) 14:3122–6. doi: 10.3892/etm.2017.4878
55. Zhang, XL, Akhtar, M, Chen, Y, Ma, ZY, Liang, YY, Shi, DS, et al. Chicken jejunal microbiota improves growth performance by mitigating intestinal inflammation. Microbiome. (2022) 10:107. doi: 10.1186/s40168-022-01330-y
56. Tremaroli, V, and Backhed, F. Functional interactions between the gut microbiota and host metabolism. Nature. (2012) 489:242–9. doi: 10.1038/nature11552
57. Vancamelbeke, M, Laeremans, T, Vanhove, W, Arnauts, K, Ramalho, AS, Farre, R, et al. Butyrate does not protect against inflammation-induced loss of epithelial barrier function and cytokine production in primary cell monolayers from patients with ulcerative colitis. J Crohns Colitis. (2019) 13:1351–61. doi: 10.1093/ecco-jcc/jjz064
58. Hou, PX, Ma, JF, Zeng, YX, Yang, YW, Wang, JD, and Liang, XJ. Analysis on nutritional components in different parts of Lycium barbarum. Feed study. (2019) 42:72–4. doi: 10.13557/j.cnki.issn1002-2813
59. Ma, JJ, Zhou, T, and Zhu, LX. Comparison of chemical composition and nutritional components of genuine traditional Lycium barbarum L. Produced from Different Habitats Chinese vegetable. (2009) 12:11–4. doi: 10.19928/j.cnki.1000-6346
Keywords: Lycium barbarum leaves, Lycium barbarum polysaccharides, microbiota, functional feed, organ weight
Citation: Guo Y, Liu J, Tuo Q, Zhang D, Wanapat M and Xin G (2024) The effect of dietary supplementation of Lycium barbarum leaves on the growth performance, organ indexes and intestinal microflora of rats. Front. Vet. Sci. 11:1416793. doi: 10.3389/fvets.2024.1416793
Edited by:
Bing Dong, China Agricultural University, ChinaReviewed by:
Tao Ma, Chinese Academy of Agricultural Sciences, ChinaNasir Landy, Islamic Azad University of Shahrekord, Iran
Copyright © 2024 Guo, Liu, Tuo, Zhang, Wanapat and Xin. This is an open-access article distributed under the terms of the Creative Commons Attribution License (CC BY). The use, distribution or reproduction in other forums is permitted, provided the original author(s) and the copyright owner(s) are credited and that the original publication in this journal is cited, in accordance with accepted academic practice. No use, distribution or reproduction is permitted which does not comply with these terms.
*Correspondence: Guosheng Xin, Z3N4aW5Abnh1LmVkdS5jbg==
†These authors have contributed equally to this work and share first authorship