- 1Population Health Sciences Department, Virginia-Maryland College of Veterinary Medicine, Virginia Tech, Blacksburg, VA, United States
- 2Nicholas School of the Environment, Duke University, Durham, NC, United States
- 3Center for Studies in Demography and Ecology, University of Washington, Seattle, WA, United States
Introduction: Companion animals offer a unique opportunity to investigate risk factors and exposures in our shared environment. Passive sampling techniques have proven effective in capturing environmental exposures in dogs and humans.
Methods: In a pilot study, we deployed silicone monitoring devices (tags) on the collars of a sample of 15 dogs from the Dog Aging Project Pack cohort for a period of 120 h (5 days). We extracted and analyzed the tags via gas chromatography–mass spectrometry for 119 chemical compounds in and around participants’ homes.
Results: Analytes belonging to the following chemical classes were detected: brominated flame retardants (BFRs), organophosphate esters (OPEs), polycyclic aromatic hydrocarbons (PAHs), polychlorinated biphenyls (PCBs), pesticides, phthalates, and personal care products. The types and amounts of analytes detected varied substantially among participants.
Discussion: Data from this pilot study indicate that silicone dog tags are an effective means to detect and measure chemical exposure in and around pet dogs’ households. Having created a sound methodological infrastructure, we will deploy tags to a geographically diverse and larger sample size of Dog Aging Project participants with a goal of further assessing geographic variation in exposures.
1 Introduction
People and pets are exposed to hundreds of potentially harmful and/or toxic chemicals every day (1, 2). Dozens of environmental contaminants representing several classes of compounds regularly found in and around the home and immediate outdoor environments have been associated with risks for chronic and adverse health outcomes (3–5).
Exposure to eight commonly-found classes of chemicals including pesticides, brominated flame retardants (BFRs), polycyclic aromatic hydrocarbons (PAHs), polychlorinated biphenyls (PCBs), personal care products, alkylphenols, organophosphate esters, and phthalates has been linked to health effects in many systems. Some such effects include endocrine and reproductive systems mutation and disruption, liver dysfunction and toxicity, respiratory and cardiac disease, neurotoxicity and neurodevelopmental disorders, among other health complications in humans (6–11). Many of these diseases are physiologically similar in dogs (12). Several studies have also demonstrated links between environmental exposures and companion animal health, a rapidly growing area of research (13–18).
Over the last 75 years, there has been a rise in production and distribution of plastics, batteries, chemical treatments for textiles (eg. stain and water repellents), commercially available pesticides, and other everyday products. This increase in use has caused an exponential rise in the number of chemicals we are exposed to via inhalation, ingestion, and transdermal exposure pathways (19–22). Several regulatory bodies (e.g., FDA, EPA, USDA, CDC, WHO) have set limits and, in some cases, bans, on production and usage of many of these compounds (23–27). Avoiding exposure as a protective measure is not always possible because these chemicals have long half-lives in the environment, which makes them persistent pollutants. Additionally, even when phased-out, people may keep products treated with them for decades resulting in continued exposure (28). Despite the ubiquity of and known health risks associated with chemical exposures, linking adverse health effects to chemicals by which exposure primarily occurs via inhalation or dermal absorption indoors can be especially difficult to track and measure in human populations (1).
Companion animals present an under-realized opportunity to better understand pathways of exposure to such harmful environmental contaminants in and around the home. Pet dogs and cats closely share both physical and social environments with humans. Moreover, the factors that degrade physiological processes are likely to have more immediate and potentially more drastic effects in pets than their longer-lived human companions with whom they share these environments (29, 30). And, while dogs’ lifespans are substantially shorter than our own (31, 32), they do live long enough to experience effects of harmful exposures over time. Thus, viewing dogs as sentinels of human health and wellbeing can enable us to more rapidly assess how environmental risk factors might cause illness in pets and people alike (30, 33).
The Dog Aging Project (DAP) population is a valuable resource for studying environmental exposures and health outcomes due to the responsive and interactive nature of this community and the frequency at which data are collected. Annual health and environmental data are collected throughout the lifespan of all participants enrolled in the DAP. Adding a quantitative component such as silicone passive samplers for detecting environmental chemical contaminants would allow for a more in-depth analysis of exposure related health outcomes.
In capturing exposure to BFRs, OPEs, PCBs, phthalates and pesticides, some of which can have both acute and chronic health impacts, Wise et al. (1, 5) described the use of silicone passive sampling (wearable tags) in 30 pairs of pet dogs and their human companions. Passive sampling strategies enable accessible, broad-scale, and diverse data collection. Here our aim was to test logistic and methodological infrastructure within the Dog Aging Project for future studies that will build and expand on this technique. Our goal was to employ passive sampling to evaluate various classes of chemical contaminants that dogs and humans may be exposed to on a daily basis. We deployed silicone monitoring devices (tags) to a sample of 15 dog participants in the Dog Aging Project for a pilot study.
2 Methods
2.1 Ethical considerations
The University of Washington IRB deemed that recruitment of dogs through their owners for the Dog Aging Project is research that qualifies for Category 2 human subjects exempt status (IRB ID no. 5988, effective 30 October 2018). All study-related procedures involving privately owned dogs were approved by the Texas A&M University IACUC, under AUP 2021-0316 CAM (effective 14 December 2021).
2.2 Recruitment
Dogs were enrolled from the Dog Aging Project (DAP), a long term, longitudinal study of aging and age-related health developments in companion dogs of dogs located throughout the United States (34). Dog owners are recruited through various media outlets and word of mouth to voluntarily nominate their dogs for participation. Owners who opt to enroll their dogs in the study are first led through an extensive informed consent process. Once consent is confirmed, owners are requested to complete the Health and Life Experiences Survey (HLES), which includes several small surveys that collect information about dog demographic characteristics, physical activity, environment, dog behavior, diet, medications and preventatives, dog health status and history, and owner demographic characteristics. Once the HLES has been completed, dogs are enrolled in the DAP Pack. An HLES Annual Follow-Up Survey (AFUS) is sent each year following enrollment. There are additional informed consent processes for dogs selected to participate in additional sampled cohorts, which may involve collecting such data as electronic veterinary medical records, genome-wide sequence information, clinicopathology and molecular phenotypes derived from blood cells, plasma, and fecal samples. The project began enrollment in 2019 and has over 47,000 dogs enrolled as of 1 January 2023. As an open data project, DAP data are available to the public at dogagingproject.org/open_data_access (2023). Any dog enrolled in the DAP whose owner had completed the baseline Health and Life Experience Survey (HLES) about their dog was eligible for participation in this pilot study.
For this pilot study, a call for participants was posted to the DAP “Dog Park” community, an online forum for DAP participants, using a Google Forms survey that remained open until 15 responses were received (see Supplementary material). The pilot participation survey collected the dog owner’s name, contact information, home address and the name of their dog enrolled in DAP. These owners were invited to attend an informational session via Zoom, during which we reviewed the purpose of the study, requirements for participation, items that would be included in the sampling kits, and instructions for attaching and removing the silicone tag, and answered participants’ questions. In order to participate, dogs had to be in their normal home environment experiencing their normal routine and had to wear a collar or harness in order to attach the silicone tag for the duration of the five-day sampling period.
2.3 Sample collection
Silicone tag monitoring devices were pre-cleaned, wrapped in aluminum foil and sealed in an airtight bag as described previously (1). Participants received a package containing the bag with the silicone tag and a fresh piece of aluminum foil, written instructions, an exposure survey (see Supplementary material), and a prepaid return mailer. Individual dogs wore tags for five consecutive days (120-h period) in their typical daily environment. Owners could select any 120-h window between the designated dates of March 1–15, 2023. Upon removal after the five-day period, owners were instructed to wrap the tags in the fresh piece of aluminum foil, place it into the airtight bag, and mail via prepaid envelope back to the lab. The time and date of application and removal were recorded on the exposure survey. Upon confirming receipt of their sample back to the lab, we invited owners to complete a feedback survey (see Supplementary material) regarding their experience with the process.
Along with the tags worn by dogs, we deployed three field blanks (contamination controls) that remained in the laboratory and were subsequently processed with the dogs’ samples.
2.4 Exposure survey
All owners completed an environmental exposure survey (see Supplementary material) during the sampling period. The goal of this survey was to collect data on potential sources of chemical exposure in the dog’s home environment and included questions about the following information: the age and type of home the dog lives in, how long the dog has lived in the home, how many hours per day the dog spends in the home, whether the dog is groomed professionally or at home and the frequency, exposure to smoke from both tobacco and non-tobacco sources, current medications, flea/tick prevention and frequency of use, heartworm prevention and frequency of use, herbicide/pesticide use in the home and yard, and the dog’s access to these areas following treatment.
Owner reported data regarding the household environment were summarized and descriptively analyzed (see Table 1). Geographic regions across the U.S. were consistent with those as defined by the National Geographic Society (35).
2.5 Analysis
All tags (N = 15) and field blanks (N = 3) were returned to the lab for processing. They were analyzed for a suite of target compounds using gas chromatography–mass spectrometry, as has been previously described (1). Tags were screened for a total of 119 analytes belonging to eight chemical classes including: brominated flame retardants, pesticides, phthalates, polychlorinated biphenyls, polycyclic aromatic hydrocarbons (PAHs), organophosphate esters, alkylphenols and personal care products (PCPs). Field blanks were used to assess contamination during sample shipment and processing, and to determine method detection limits (MDLs) for each analyte as previously described (1). MDLs are the minimum level of analyte concentration that can be detected during analysis. Detection frequency, median level, and range were calculated for all 119 compounds.
3 Results
All participants (N = 15) from 12 different states across the U.S. who opted into the study and received a sampling kit successfully implemented the at-home procedure and returned the used monitoring tags to the lab.
3.1 Sample group demographics
Dogs enrolled in the study included mixed breed and purebred dogs representing 17 different breeds in total (Table 2). Eight were regarded as large breed dogs (weight at time of enrollment ≥ 20 kg) and seven were regarded as small breed dogs (weight at time of enrollment < 20 kg). There were nine male and six female dogs in the study, and eight young adult and seven mature adult dogs, per the DAP HLES.
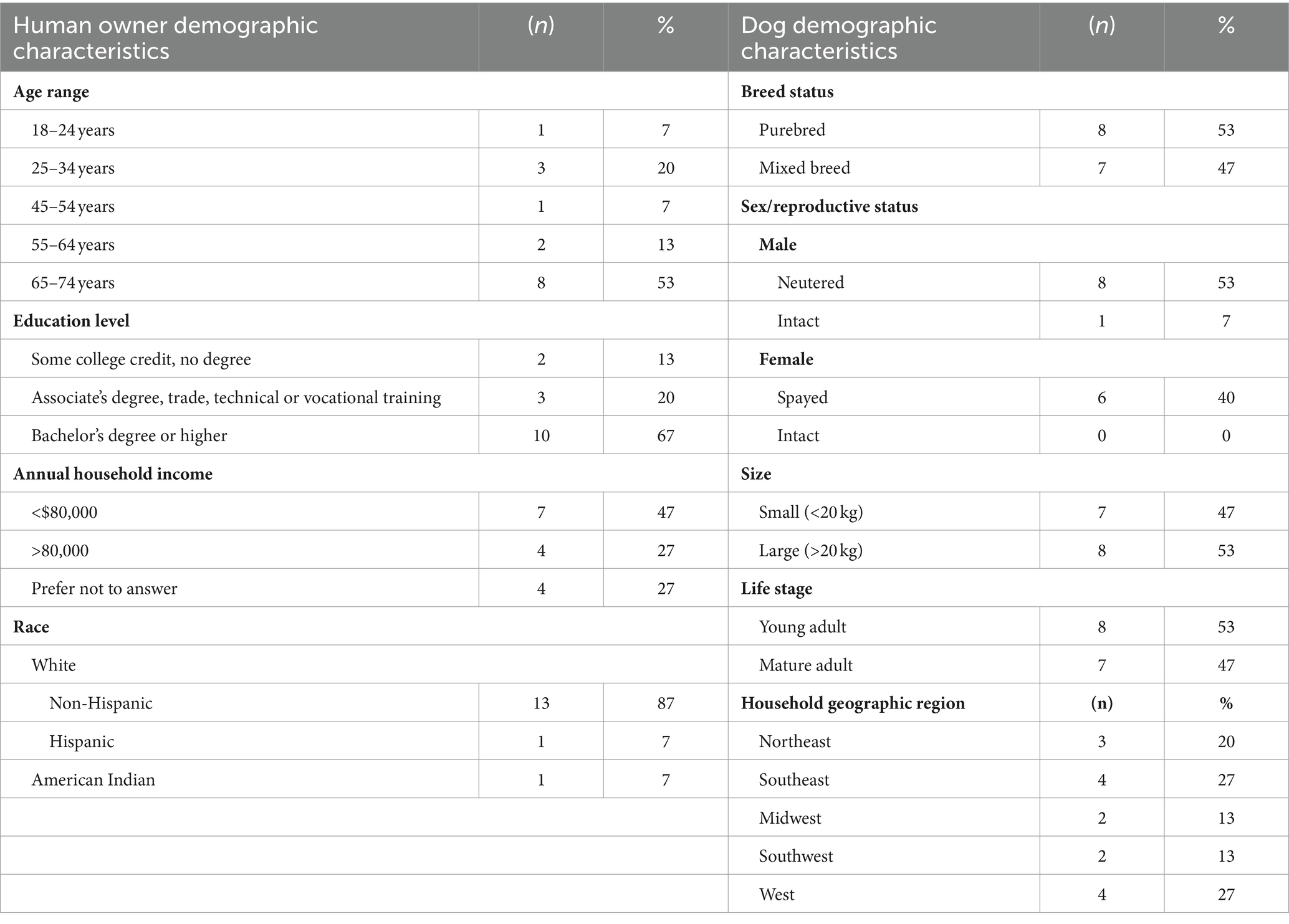
Table 2. Dog and owner demographic characteristics, Dog Aging Project silicone tag pilot study, 2023.
Four dog households were located in the U.S. West, two in the Midwest, two in the Southwest, three in the Northeast, and four in the Southeast (35).
Human dog owners who opted to enroll their dogs ranged in age from 18 to 75. All owners reported having attained an education level of at least some college, with a doctorate degree being the highest attained level. Reported annual income range was ≤$20,000 to ≥$180,000. Fourteen out of 15 human participants self-identified as White and one person self-identified as American Indian. Among the 15 participants, one person identified as being of Hispanic origin.
Sixty percent (9/15) of owners reported believing their dog to be in excellent health, and all but one (14/15) reported their dog in at least good health. Nearly half of dogs (7/15) included in this pilot study were reported to have a newly developed or diagnosed health condition (within the last year) at time of survey.
3.2 Reported household/environmental exposures
Participants responded to a questionnaire regarding their dog’s home environment (indoor and exterior) and routine behaviors in and around the home (Table 1). Two-thirds of participants (10/15) reported living in a suburban environment, two in a rural area, and three in urban areas.
Seventy-three percent of participants (11/15) lived in homes that were ≥20 years old. More than half of dog owners (8/15) reported wearing shoes in the home. No dogs are reported to have been exposed to passive tobacco smoke.
All but two dogs (13/15) had lived in the home for at least 1 year at the time of study. The majority of dogs (9/15) reportedly spent at least 19 h per day inside of the home. Four dogs had been professionally groomed at least twice annually, while an additional seven had received in-home bathing/grooming at various intervals throughout the year.
Eighty-seven percent (13/15) of the dogs had been treated with pest preventative (oral, topical, or collar) at least once in the 12 months leading up to time of study, and 87% had been treated with heartworm preventative at least once in the preceding year. One-third of dog owners (5/15) reported having used a chemical herbicide or pesticide treatment in or around the home at least once during the preceding year.
3.3 Chemical analyses
Using passive sampling monitoring devices (silicone tags) placed on dog’s collars, we detected analytes belonging to all of the chemical classes for which testing was performed. Results for each chemical, in each chemical class, are reported in Table 3, including their detection frequency, median level, and range. Details with full results for each individual tag can be seen in Supplementary Table S1.
The types and amounts of analytes detected varied substantially among participants. In total, 93 out of 119 chemicals (78%) were found in at least one participant household. Fifty-three chemicals (45% of the total number of chemicals) representing all eight chemical classes were detected in 50% (8 out of 15) of participant households (Table 4). These 53 chemicals represent all eight chemical classes.
Twenty-five out of 119 (21%) individual chemicals were detected in all 15 dog tags (100%). The analytes detected in 100% of households included chemicals from the following classes: pesticides, organophosphates, phthalates, PAHs, alkylphenols, PCBs, and PCPs. They include cis-permethrin, di-ethyl phthalate, dibutyl phthalate, benzyl butyl phthalate, bis(2-ethylhexyl) phthalate, bis(2-ethylhexyl) terephthalate, di-isononyl phthalate, trioctylmetallitate, 4-tertoctylphenol, nonylphenol isomer mix, lilial, 3,3′-dichlorobiphenyl, fluoranthene, pyrene, benzo[j,b,k]fluoranthene, benzo[e]pyrene, benzo[a]pyrene, 2-isopropylphenyl diphenyl phosphate, 4-tert-butylphenyl diphenyl phosphate, tri-iso-butyl-phosphate, tris(chloroisopropyl) phosphate, bis(2-chloro-1-methylethyl)(2-chloropropyl) phosphate, triphenyl phosphate, 2-ethylhexyl diphenyl phosphate, and tris(2-ethylhexyl) phosphate.
4 Discussion
We employed a passive sampling method that has previously been shown to successfully assess environmental exposure in humans and their companion dogs (1, 5). We found that in a geographically diverse set of samples collected from dogs’ within the United States, several compounds representing eight chemical classes were detectable on silicone tags worn by dogs over a 120-h period. Finding sound methodology prompts further and broader investigation of chemical exposure in companion dogs via passive sampling.
Chemical exposure, broadly, has important implications for veterinary care and management. Many chemical toxicants found in and around the home environment (e.g., PBDEs, PCBs, phthalates) are the culprits in common companion animal diseases of the reproductive system, thyroid disorders, and various cancers in cats and dogs (14, 33). And, these conditions are among the top most frequently diagnosed (36). Improved understanding of where and how exposure occurs can inform care decisions when these patients present in the clinical setting.
Considering our results, the ubiquitous presence of several chemicals and compounds known to have adverse effects on human and animal health is especially concerning. However, additional data are needed to further assess what those specific concerns are or should be, and when we can and should act on them. With these data alone, we are limited in our ability to discern at what detectable level, frequency, and duration of exposure to one or several of the substances a dog’s health will be affected (or in what ways they will be impacted). Passive sampling techniques such as the silicone tag monitoring devices make it difficult to control for the effects of multiple routes and/or instances of exposure to various chemicals in isolation or in combination (though not likely any less so than more invasive physiological measures).
For example, in at least one case, an individual dog’s tag returned detectable amounts of several compounds at levels markedly higher than all other participants, including the presence of some chemicals not found in any other samples. In particular, the levels of the pesticides permethrin, fipronil, DEET and cypermethrin detected on this dog’s tag were the highest out of all samples. There are some studies that have shown that exposure to mixtures of these pesticides can have adverse effects (37, 38). In this case, the dog was reportedly in very good health according to the owner, with no new or ongoing health conditions at the time of sampling. The owner also reported using a flea and tick preventative that contained fipronil, so this result is consistent with a previous study showing statistically significantly higher levels of fipronil on dog tags worn by dogs treated with these types of flea and tick products (5).
The owner reported not using any pesticides in or around their home or property, but was notably one of only two participants who reported living in a rural area. DEET is a widely used insect repellent. While permethrin is a commonly used general use pesticide for residential and veterinary/medical applications, it is restricted-use for crops and wide areas (limits on application levels, except for as mosquito sprays) due to its aquatic toxicity, per the U.S. EPA. However, cypermethrin does have large scale commercial agricultural uses. We cannot determine if the exposure to the other pesticides represents an ongoing exposure in the immediate environment, or a brief exposure, such as an upwind pesticide application.
Due to the sample size of this initial deployment wherein our goal was to test logistical and methodological soundness, we were unable to investigate any potential direct correlation between risk of exposure and specific health effects and conditions in dogs. However, we do have access to medical records, diagnoses, ongoing care data, as well as household, physical, and social indicators for a potential sample size of up to more than 45,000 dogs. Our next deployment of silicone tags will support us in overcoming the limitations inherent in this pilot study and will be conducted in a way that takes full advantage of these additional data. With a larger sample, we will also be able to evaluate location-based co-factors without jeopardizing participants’ right to privacy, and with the express purpose of evaluating ambient environment data in conjunction with a comprehensive suite of additional variables.
Furthermore, the widespread adoption of low-burden, low-cost passive sampling tools and methods such as silicone monitoring devices should be more seriously considered as a means to increase equity and accessibility in data collection and to reach a more diverse participant demographic.
In feedback we received from 14 of the 15 study participants via a post-deployment survey, dog owners expressed that being a part of the data collection process was non-burdensome. All stated that the informational Zoom call was helpful, the instructions were clear and concise, and that the study activities were manageable. Two respondents reported difficulties with attaching the silicone tag to their dog’s collar, particularly with opening the attachment rings. One respondent reported difficulty with sending or receiving their package, but further details were not provided. When asked about the content they’d like to see in a results report, 10 respondents indicated a desire to receive their dog’s overall findings.
These considerations may be especially important for inclusion of populations of people and their dogs unjustly at higher risk of exposure and with lower access to care. And, because exposures can change considerably over time, seasonally, and in relation to various behaviors and activities, these tags offer an opportunity to repeatedly assess and compare risk, with relative ease.
While we recognize the limitations in attempting to assess multiple environmental exposures that can impact the health of dogs, studies using these passive sampling methods have great potential to support development of improved rapid response protocols. Given the successful detection of multiple exposures in a short period of time (120-h) during this study, silicone dog tags may offer a method for retrieving point-in-time risk exposure, such as during or immediately following an accident or disaster (39). In the past, risk assessment during such events has been only marginally effective, due in part to latency in data collection as well as using dated, non-location specific methods for assessment (40–42). Silicone tag monitoring devices, could enable a more accurate assessment of where and when exposure risk is highest, and to assess the capacity of community response and availability of resources to mitigate possible harm from exposures.
5 Conclusion
Data from this deployment of passive sampling devices indicate that silicone dog tags are an effective means to measure multiple chemical exposures in and around pet dogs’ homes. Given our results, veterinary practitioners should be cognizant of the potential hazards and adverse health outcomes for companion animals exposed to chemicals in the everyday environment. Our study also supports the value of using silicone tags with dogs to investigate potential health impacts on humans from shared environmental exposures. We will investigate this further in a future study that includes a hypothesis-generating deployment to a larger and geographically diverse sample of Dog Aging Project participants. We will deploy via a stratified selection process to recruit 80 additional dogs (20 small size/rural, 20 small size/urban, 20 large size/rural, 20 large size/urban) with a goal of further assessing location-based variation in exposures and potential compounding risk factors for both dogs and humans.
Data availability statement
The datasets presented in this study can be found in online repositories. The names of the repository/repositories and accession number (s) can be found in the article/Supplementary material.
Ethics statement
The animal studies were approved by Texas A&M University IACUC, under AUP 2021-0316 CAM. The studies were conducted in accordance with the local legislation and institutional requirements. Written informed consent was obtained from the owners for the participation of their animals in this study.
Author contributions
RM: Investigation, Writing – original draft. CS: Writing – original draft. CW: Data curation, Formal analysis, Writing – review & editing. JO'B: Formal analysis, Validation, Visualization, Writing – review & editing. AK: Project administration, Writing – review & editing. MK: Data curation, Writing – review & editing. MD: Supervision, Writing – review & editing. HS: Conceptualization, Supervision, Writing – review & editing. AR: Conceptualization, Funding acquisition, Supervision, Writing – review & editing.
DAP consortium
Joshua M. Akey; Brooke Benton; Elhanan Borenstein; Marta G. Castelhano; Amanda E. Coleman; Kate E. Creevy; Kyle Crowder; Matthew D. Dunbar; Virginia R. Fajt; Annette L. Fitzpatrick; Unity Jeffery; Erica C Jonlin; Matt Kaeberlein; Elinor K. Karlsson; Kathleen F. Kerr; Jonathan M. Levine; Jing Ma; Robyn L McClelland; Daniel E.L. Promislow; Audrey Ruple; Stephen M. Schwartz; Sandi Shrager; Noah Snyder-Mackler; M. Katherine Tolbert; Silvan R. Urfer; Benjamin S. Wilfond.
Funding
The author(s) declare that financial support was received for the research, authorship, and/or publication of this article. This research is based on publicly available data collected by the Dog Aging Project, which is supported by U19 grant AG057377 from the National Institute on Aging, a part of the National Institutes of Health, and by additional grants and private donations. These data are housed on the Terra platform at the Broad Institute of MIT and Harvard.
Acknowledgments
The Dog Aging Project thanks study participants, their dogs, and community veterinarians for their important contributions to this paper.
Conflict of interest
The authors declare that the research was conducted in the absence of any commercial or financial relationships that could be construed as a potential conflict of interest.
The author(s) declared that they were an editorial board member of Frontiers, at the time of submission. This had no impact on the peer review process and the final decision.
Publisher’s note
All claims expressed in this article are solely those of the authors and do not necessarily represent those of their affiliated organizations, or those of the publisher, the editors and the reviewers. Any product that may be evaluated in this article, or claim that may be made by its manufacturer, is not guaranteed or endorsed by the publisher.
Supplementary material
The Supplementary material for this article can be found online at: https://www.frontiersin.org/articles/10.3389/fvets.2024.1394061/full#supplementary-material
References
1. Wise, CF, Hammel, SC, Herkert, N, Ma, J, Motsinger-Reif, A, Stapleton, HM, et al. Comparative exposure assessment using silicone passive samplers indicates that domestic dogs are sentinels to support human Health Research. Environ Sci Technol. (2020) 54:7409–19. doi: 10.1021/acs.est.9b06605
2. Rudel, RA, Dodson, RE, Perovich, LJ, Morello-Frosch, R, Camann, DE, Zuniga, MM, et al. Semivolatile endocrine-disrupting compounds in paired indoor and outdoor air in two northern California communities. Environ Sci Technol. (2010) 44:6583–90. doi: 10.1021/es100159c
3. Boffetta, P, and Nyberg, F. Contribution of environmental factors to cancer risk. Br Med Bull. (2003) 68:71–94. doi: 10.1093/bmp/ldg023
4. Bijlsma, N, and Cohen, MM. Environmental chemical assessment in clinical practice: unveiling the elephant in the room. Int J Environ Res Public Health. (2016) 13:181. doi: 10.3390/ijerph13020181
5. Wise, CF, Hammel, SC, Herkert, NJ, Ospina, M, Calafat, AM, Breen, M, et al. Comparative assessment of pesticide exposures in domestic dogs and their owners using silicone passive samplers and biomonitoring. Environ Sci Technol. (2022) 56:1149–61. doi: 10.1021/acs.est.1c06819
6. Faroon, O, and Olson, JR. Toxicological profile for polychlorinated biphenyls (PCBs) U.S. Department of Health and Human Services, Public Health Service, Agency for Toxic Substances and Disease Registry (2000). 765 p.
7. Rauh, V, Arunajadai, S, Horton, M, Perera, F, Hoepner, L, Barr, DB, et al. Seven-year neurodevelopmental scores and prenatal exposure to chlorpyrifos, a common agricultural pesticide. Environ Health Perspect. (2011) 119:1196–201. doi: 10.1289/ehp.1003160
8. Mallah, MA, Changxing, L, Mallah, MA, Noreen, S, Liu, Y, Saeed, M, et al. Polycyclic aromatic hydrocarbon and its effects on human health: an overeview. Chemosphere. (2022) 296:133948. doi: 10.1016/j.chemosphere.2022.133948
9. Lyche, JL, Rosseland, C, Berge, G, and Polder, A. Human health risk associated with brominated flame-retardants (BFRs). Environ Int. (2015) 74:170–80. doi: 10.1016/j.envint.2014.09.006
10. Bekele, TG, Zhao, H, Yang, J, Chegen, RG, Chen, J, Mekonen, S, et al. A review of environmental occurrence, analysis, bioaccumulation, and toxicity of organophosphate esters. Environ Sci Pollut Res Int. (2021) 28:49507–28. doi: 10.1007/s11356-021-15861-8
11. Eales, J, Bethel, A, Galloway, T, Hopkinson, P, Morrissey, K, Short, RE, et al. Human health impacts of exposure to phthalate plasticizers: an overview of reviews. Environ Int. (2022) 158:106903. doi: 10.1016/j.envint.2021.106903
12. Shearin, AL, and Ostrander, EA. Leading the way: canine models of genomics and disease. Dis Model Mech. (2010) 3:27–34. doi: 10.1242/dmm.004358
13. Gautam, S, Sood, NK, Gupta, K, Joshi, C, Gill, KK, Kaur, R, et al. Bioaccumulation of pesticide contaminants in tissue matrices of dogs suffering from malignant canine mammary tumors in Punjab, India. Heliyon. (2020) 6:e05274. doi: 10.1016/j.heliyon.2020.e05274
14. Pocar, P, Grieco, V, Aidos, L, and Borromeo, V. Endocrine-disrupting chemicals and their effects in pet dogs and cats: an overview. Animals. (2023) 13:378. doi: 10.3390/ani13030378
15. Glickman, LT, Raghavan, M, Knapp, DW, Bonney, PL, and Dawson, MH. Herbicide exposure and the risk of transitional cell carcinoma of the urinary bladder in Scottish terriers. J Am Vet Med Assoc. (2004) 224:1290–7. doi: 10.2460/javma.2004.224.1290
16. Smith, N, Luethcke, KR, Craun, K, and Trepanier, L. Risk of bladder cancer and lymphoma in dogs is associated with pollution indices by county of residence. Vet Comp Oncol. (2022) 20:246–55. doi: 10.1111/vco.12771
17. Ka, D, Marignac, G, Desquilbet, L, Freyburger, L, Hubert, B, Garelik, D, et al. Association between passive smoking and atopic dermatitis in dogs. Food Chem Toxicol. (2014) 66:329–33. doi: 10.1016/j.fct.2014.01.015
18. Sonne, C, Larsen, HJS, Kirkegaard, M, Letcher, RJ, and Dietz, R. Trans-generational and neonatal humoral immune responses in West Greenland sledge dogs (Canis familiaris) exposed to organohalogenated environmental contaminants. Sci Total Environ. (2010) 408:5801–7. doi: 10.1016/j.scitotenv.2010.07.076
19. Stapleton, HM, Allen, JG, Kelly, SM, Konstantinov, A, Klosterhaus, S, Watkins, D, et al. Alternate and new brominated flame retardants detected in U.S. house dust. Environ Sci Technol. (2008) 42:6910–6. doi: 10.1021/es801070p
20. Venier, M, and Hites, RA. Flame retardants in the serum of pet dogs and in their food. Environ Sci Technol. (2011) 45:4602–8. doi: 10.1021/es1043529
21. López, A, Fuentes, E, Yusà, V, Ibáñez, M, and Coscollà, C. Identification of unknown substances in ambient air (PM10), profiles and differences between rural, urban and industrial areas. Toxics. (2022) 10:220. doi: 10.3390/toxics10050220
22. Liu, X . Understanding semi-volatile organic compounds (SVOCs) in indoor dust. Indoor Built Environ. (2022) 31:291–8. doi: 10.1177/1420326X211070859
23. Krimsky, S . The unsteady state and inertia of chemical regulation under the US toxic substances control act. PLoS Biol. (2017) 15:e2002404. doi: 10.1371/journal.pbio.2002404
24. US EPA . New chemical exposure limits under TSCA. (2015) Available at: https://www.epa.gov/reviewing-new-chemicals-under-toxic-substances-control-act-tsca/new-chemical-exposure-limits-under (Accessed 14 February 2024).
25. OSHA . Chemical Hazards and Toxic Substances—Overview. (n.d.) Available at: https://www.osha.gov/chemical-hazards (Accessed 14 February 2024).
26. Cordner, A, Richter, L, and Brown, P. Can chemical class approaches replace chemical-by-chemical strategies? Lessons from recent U.S. FDA regulatory action on per- and Polyfluoroalkyl substances. Environ Sci Technol. (2016) 50:12584–91. doi: 10.1021/acs.est.6b04980
27. Kudsk, P, and Mathiassen, SK. Pesticide regulation in the European Union and the glyphosate controversy. Weed Sci. (2020) 68:214–22. doi: 10.1017/wsc.2019.59
28. Kienzler, A, Bopp, SK, van der Linden, S, Berggren, E, and Worth, A. Regulatory assessment of chemical mixtures: requirements, current approaches and future perspectives. Regul Toxicol Pharmacol. (2016) 80:321–34. doi: 10.1016/j.yrtph.2016.05.020
29. Neo, JPS, and Tan, BH. The use of animals as a surveillance tool for monitoring environmental health hazards, human health hazards and bioterrorism. Vet Microbiol. (2017) 203:40–8. doi: 10.1016/j.vetmic.2017.02.007
30. Hegedus, C, Andronie, L, Uiuiu, P, Jurco, E, Lazar, EA, and Popescu, S. Pets, genuine tools of environmental pollutant detection. Animals. (2023) 13:2923. doi: 10.3390/ani13182923
31. Teng, KT-Y, Brodbelt, DC, Pegram, C, Church, DB, and O’Neill, DG. Life tables of annual life expectancy and mortality for companion dogs in the United Kingdom. Sci Rep. (2022) 12:6415. doi: 10.1038/s41598-022-10341-6
32. Montoya, M, Morrison, JA, Arrignon, F, Spofford, N, Charles, H, Hours, M-A, et al. Life expectancy tables for dogs and cats derived from clinical data. Front Vet Sci. (2023) 10:1082102. doi: 10.3389/fvets.2023.1082102
33. National Academies of Sciences, Engineering, and Medicine, Division on Earth and Life Studies, Standing Committee on the Use of Emerging Science for Environmental Health Decisions, Health and Medicine Division, Board on Health Care Services, Forum on Aging, Disability, and Independence, National Cancer Policy Forum . Companion animals as sentinels for predicting environmental exposure effects on aging and Cancer susceptibility in humans: Proceedings of a workshop National Academies Press (2022). 172 p.
34. Creevy, KE, Akey, JM, Kaeberlein, M, and Promislow, DEL. Dog aging project consortium. An open science study of ageing in companion dogs. Nature. (2022) 602:51–7. doi: 10.1038/s41586-021-04282-9
35. United States Regions . Available at: https://education.nationalgeographic.org/resource/united-states-regions/ (n.d.). (Accessed 14 February 2024).
36. O’Neill, DG, James, H, Brodbelt, DC, Church, DB, and Pegram, C. Prevalence of commonly diagnosed disorders in UK dogs under primary veterinary care: results and applications. BMC Vet Res. (2021) 17:69. doi: 10.1186/s12917-021-02775-3
37. Thorson, JLM, Beck, D, Ben Maamar, M, Nilsson, EE, and Skinner, MK. Epigenome-wide association study for pesticide (permethrin and DEET) induced DNA methylation epimutation biomarkers for specific transgenerational disease. Environ Health. (2020) 19:109. doi: 10.1186/s12940-020-00666-y
38. Côté, J, Bonvalot, Y, Carrier, G, Lapointe, C, Fuhr, U, Tomalik-Scharte, D, et al. A novel toxicokinetic modeling of cypermethrin and permethrin and their metabolites in humans for dose reconstruction from biomarker data. PLoS One. (2014) 9:e88517. doi: 10.1371/journal.pone.0088517
39. Badley, JR, Ryce, C, and Lein, PJ. Train derailment in East Palestine, Ohio: the toxic risks of transporting hazardous chemicals. Open Access Government. (2023) 40:380–1. doi: 10.56367/OAG-040-9857
40. Ruple, A . Do our exposure variables tell us what we think they are telling us? 104th Conference of Research Workers in Animal Diseases Proceedings. Chicago, IL, USA. (2024).
41. Samon, SM, Rohlman, D, Tidwell, LG, Hoffman, PD, Oluyomi, AO, and Anderson, KA. Associating increased chemical exposure to hurricane Harvey in a longitudinal panel using silicone wristbands. Int J Environ Res Public Health. (2022) 19:670. doi: 10.3390/ijerph19116670
Keywords: exposure assessment, silicone wristbands, biomonitoring, passive sampling device, dog
Citation: Matheson R, Sexton CL, Wise CF, O’Brien J, Keyser AJ, Kauffman M, Dunbar MD, DAP Consortium, Stapleton HM and Ruple A (2024) Silicone tags as an effective method of monitoring environmental contaminant exposures in a geographically diverse sample of dogs from the Dog Aging Project. Front. Vet. Sci. 11:1394061. doi: 10.3389/fvets.2024.1394061
Edited by:
Chiara Palmieri, The University of Queensland, AustraliaReviewed by:
Wilson Kiiza Rumbeiha, University of California, Davis, United StatesBegum Yurdakok Dikmen, Ankara University, Türkiye
Copyright © 2024 Matheson, Sexton, Wise, O’Brien, Keyser, Kauffman, Dunbar, DAP Consortium, Stapleton and Ruple. This is an open-access article distributed under the terms of the Creative Commons Attribution License (CC BY). The use, distribution or reproduction in other forums is permitted, provided the original author(s) and the copyright owner(s) are credited and that the original publication in this journal is cited, in accordance with accepted academic practice. No use, distribution or reproduction is permitted which does not comply with these terms.
*Correspondence: Audrey Ruple, YXJ1cGxlQHZ0LmVkdQ==
†These authors share first authorship