- 1Veterinary Medicine Study Program, Faculty of Medicine, Padjadjaran University, West Java, Bandung, Indonesia
- 2Division of Reproduction and Obstetrics, School of Veterinary Medicine and Biomedical Sciences, IPB University, West Java, Bogor, Indonesia
- 3Research Center for Applied Zoology, National Research and Innovation Agency (BRIN), West Java, Bogor, Indonesia
Increasing the age of bulls results in a decrease in reproductive function, including a reduction in sperm quality, which plays a vital role in determining the fertility of bulls. Through a proteomic approach, this research aims to analyze the influence of age factors on various proteomes contained in bull sperm. Frozen semen samples from Simmental Bulls were categorized into three age groups: two, four, and ≥10 years old. Subsequently, the post-thaw sperm cells obtained were separated based on molecular weight using 1D-SDS-PAGE. Peptides extracted from the bands produced in each age group were subjected to LC-MS/MS analysis. A total of 72 protein types were identified, with 45 being detected in the 4-year-old group and 41 expressed in both the 2 and ≥10-year-old groups. The results provided insights into proteins' role in sperm metabolism across all age groups. Specifically, the 2-year-old group exhibited the expression of proteins associated with acrosome assembly and spermatid development (SPACA1). In contrast, those in the 4-year-old group were linked to motility (PEBP4) and sperm decapacitation factor (PEBP1). Proteins expressed in the 2 and -year-old groups were discovered to be involved in fertilization processes (TEX101). In contrast, the ≥10-year-old age group was associated with hyperactive movement related to capacitation (Tubulin). In conclusion, age influenced the differences observed in the proteomic profile of post-thaw Simmental bull sperm using the 1D-SDS-PAGE tandem LC-MS/MS approach.
Introduction
Bull fertility is defined as the ability of sperm to fertilize and activate oocytes and support embryo development (1). This factor plays a crucial role in reproductive efficiency and the success of artificial insemination (AI) in bulls. The age of the bull is widely believed to have an impact on fertility. Previous studies categorized bull age as young (1.8-2 years) and adult (3-6 years) (2–4). Satrio et al. (5) showed that optimal semen production in bulls was achieved between 3 and 4 years of age. Collins et al. (6) discovered the highest fertility rates in Holstein and Guernsey bulls at 3-4 years of age. Generally, the reproductive capacity of bulls gradually decreases after reaching the highest fertility age due to age-related declines in various reproductive functions, such as histological (7, 8) and clinical (9) deterioration in testicular function. Changes in testicular function can disrupt spermatogenesis (8) and reduce semen quality (2, 5, 10–12). Despite this, old bulls (over 10 years old) are still maintained and used for frozen semen production in AI activities at the Indonesian Artificial Insemination Center.
Several methods have been employed to predict bull fertility, including evaluating non-return rates (NRR) (13). Additionally, conventional assessments of semen quality, such as plasma membrane integrity, motility, morphology, and acrosome, are routinely performed to predict fertility (1, 14). In Indonesia, the SNI 4869-2:2017 standard for frozen semen quality in bulls includes motility, abnormalities, individual movement, sperm concentration, and recovery rate percentage to achieve good fertility. These standard semen evaluation procedures are visually helpful in determining poor-quality semen but insufficient in accurately detecting potential markers of bull fertility (1, 14). Therefore, sperm quality must be evaluated through a molecular approach to obtain important information concerning the potential markers.
Proteomics has become the most advanced approach for predicting fertility with increased accuracy (15). By employing this method, the molecular aspects of sperm that impact fertility can be identified (16–18). Sperm proteomic analysis is used to determine the essential functions of proteins and their regulatory roles in various fertilization processes (16, 19, 20). Furthermore, alterations in proteomic expression are believed to play a crucial role in the transition of sperm function from the epididymis to capacitation in the female reproductive tract and subsequent fertilization (16, 17). Previous studies discovered fertility markers in bull semen (13, 16, 19, 21, 22). Only limited information exists on the proteomic analysis of post-thaw sperm about bull age. Therefore, this study aims to investigate the variations in the proteomic profile of post-thaw sperm among bulls of different ages.
Materials and methods
Experimental design and semen samples
This study only used frozen semen, a commercial product from an AI center, as the primary sample and did not directly involve bulls. Furthermore, the entire semen collection process was carried out using an artificial vagina for the same period (without seasonal differences), frozen using the same extender. To eliminate any potential for variation in the samples, the bulls were kept in the same environment regarding feeding and handling management. However, each stage follows the operational standards in Indonesia, SNI ISO 9001:2015 No. 824 100 16072, supervised by a veterinarian, considered the principles of animal welfare, which refer to the ethical clearance requirements of the Animal Care and Uses Committee. The AI Center owned all bulls used in this study under the auspices of the government. However, we only use commercial products sold to the public without requiring animal ownership approval. A total of 27 frozen semen straws (nine straws per each group) of nine Simmental bulls of different age groups, namely two (young; n = 3 bulls), four (adult; n = 3 bulls), and ≥10 years old (old; n = 3 bulls). The number of bulls used in this study is the total number of Simental bulls available at the AI Center during the research period.
Extraction of post-thaw sperm proteins
The frozen semen was thawed at 37°C and washed thrice with phosphate-buffered saline through centrifugation at 1800 rpm for 10 min. The sperm pellet was then subjected to extraction using PRO-PREP™ Protein extraction solution (iNtRON Biotechnology, Korea) according to the manufacturer's instructions. 500 μL of PRO-PREP™ solution was added to the pellet, incubated at −20°C for 20 min, and centrifuged at 13000 rpm (4°C) for 5 min. The total soluble protein concentration of the sample was determined before SDS-PAGE analysis using the Bradford method (23), with BSA (Sigma-Aldrich) serving as the standard.
Separation of sperm protein using SDS-PAGE
Protein separation was performed using a 12.5% polyacrylamide gel containing sodium dodecyl sulfate (SDS) and a 4% stacking gel. This process was carried out at a voltage of 60 V and a current of 20 mA for 3.5 h. Subsequently, the gel was stained using Coomassie Brilliant Blue staining (24). The marker employed was Excelband™ 3-color Broad Range Protein Marker PM2700 (SMOBIO® Technology, Inc., Taiwan) with a molecular weight range of ~5-245 kDa.
Liquid chromatography-mass spectrometry (LC-MS/MS) analysis
The protein bands formed on the gel were excised and washed twice with 200 μL of destaining solution [80 mg ammonium bicarbonate in 20 mL acetonitrile (ACN) and 20 mL ultrapure water] for 30 min at 37°C. Before digestion, the protein samples were treated with 30 μL of alkylation buffer [Iodoacetamide (IAA)] for 1 h at room temperature in the dark. Tryptic digestion was performed using 10 ng/μL of activated trypsin (Promega, Fitchburg, WI, USA), with an enzyme/substrate ratio of 1/50 (w/w) for 4 h at 37°C. A total 1% of the final volume of TCA (trichloroacetic acid) solution was added to stop the trypsin activity reaction (25). Furthermore, the activated peptide samples were purified with C18 Spin Columns (Thermo Scientific, Pierce Biotechnology, N Meridian Rd, Rockford, IL, USA), each containing 8 mg of C18 reversed-phase resin (to bind the peptides).
The dried peptide samples were dissolved in 50 μL of dissolving solution (2% ACN, 98% ultrapure water, and 0.1% formic acid) and centrifuged at 12000 rpm for 10 min. Subsequently, 2.5 μL of the peptides were fractionated using the Nano LC Ultimate 3000 Series System coupled with the Q Exactive™ Plus Hybrid Quadrupole-Orbitrap™ Mass Spectrometer (Thermo Fisher Scientific, Bremen, Germany). The trap column used had a diameter of 30 μm and a length of 5 mm (Thermo Scientific™ 164649, Bremen, Germany). The capillary column was the PepMap RSLC C18 column (75 μm inner diameter X 15 cm, 3 μm particle size, 100 pore size, part number ES 800) (Thermo Scientific, Bremen, Germany) with a flow rate of 300 nL/min. The eluents applied were H2O+0.1% formic acid (A) and 98% acetonitrile + 0.1% formic acid (B). The procedure for elution of peptides on a column includes 0–3 min gradient of solvent B; 2–35% solvent B for 3–30 min; 35–90% solvent B for 30–45 min; 90% solvent B for 45–90 min; and 5% solvent B for 60–90 min. The signal peptide was obtained using the LTQ-Orbitrap mass spectrometer (Thermo Scientific, Bremen, Germany) with a 200-2000 m/z mass range. The scans were acquired via 30, 000 MS resolution (at m/z 400) in the Orbitrap analyzer, followed by 10 intensive MS/MS scans of the precursor via collision-induced dissociation (CID) fragmentation at normalized collision energies of 35% (26).
Protein identification
The data collected from the LC-MS/MS instrument were analyzed using Proteome Discoverer 2.2 software (Thermo Fisher Scientific) with the Sequest HT search engine, Uniprot bovine (Bos taurus) protein database (https://www.uniprot.org/). Proteins were required to have a sequence HT score > 0 and a minimum of two unique peptides, with a mass tolerance of 10 ppm. Those originating from contaminants such as keratin, egg yolk extender, and skim milk were excluded from the analysis. Moreover, the identified proteins were subjected to functional analysis using the online PANTHER classification system (pantherdb.org). Venn analysis, representing the intersection of each group, was conducted using Venny 2.1.0 https://bioinfogp.cnb.csic.es/tools/venny/. Protein interactions were analyzed with the STRING version 12.0 (https://string-db.org/) (27).
Results
Protein distribution and venn analysis
The results of the analysis showed that 41 sperm proteins were found in the 2-year age group, 45 sperm proteins in the 4-year age group, and 41 sperm proteins were found in the age group of more than 10 years. The analysis conducted using the Venny software (Figure 1) revealed that 18 proteins (25%) were expressed in all age groups, while 16 (22.2%), 8 (11.1%), and 11 (15.3%) were respectively expressed in each group, and the remaining were present in overlapping age groups.
Identification of the expressed proteins and their function
The expressed proteins related to fertility and age are presented in Table 1. Across all age groups, proteins associated with essential sperm functions such as sperm metabolism, capacitation, acrosome reaction, and fertilization were expressed. In the 2-year-old group, there were proteins related to acrosome assembly (SPACA1), capacitation (LPL, BSP5, SRN), spermatogenesis (RNASE4), fertilization (TIMP2, ARSA, SPADH1, and SPADH2), antioxidant (PRDX5), and apoptosis (CYCT). The 4-year-old group had proteins related to decapacitation (PEBP1), antioxidants (QSOX1), capacitation (GSN and APOA4), and fertilization (C4BPA). The ≥10-year-old groups expressed proteins linked to cytoskeletal integrity (α- and β-tubulin). The 2 and 4-year-old groups contained proteins related to sexual reproduction (TEX101), motility (PRKAR1A), and fertilization (SERPINA5, SERPINE2), while those found in the four and ≥10-year-old groups were associated with sexual reproduction (DLD, ATP1A4, ADM1B, ADAM20).
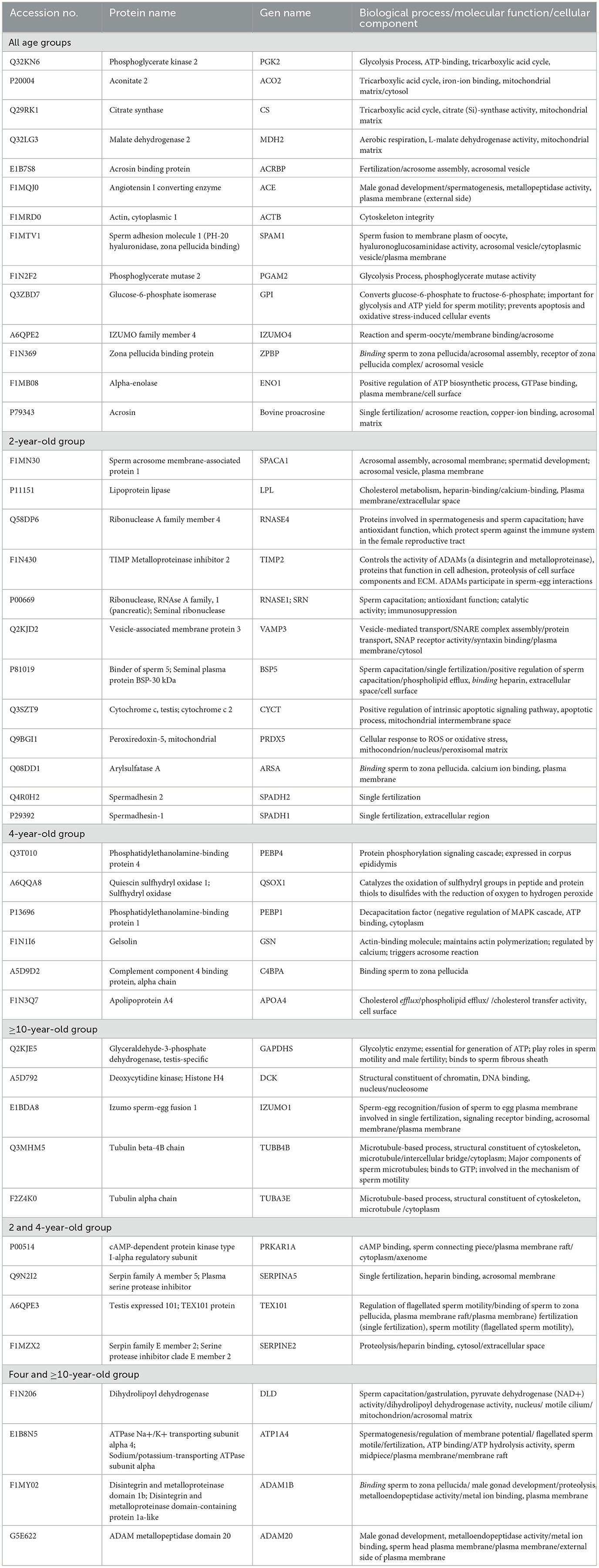
Table 1. Post-thaw sperm protein expression at different ages of bulls is related to reproductive function.
Gene ontology analysis of the proteins
Gene ontology analysis classified the proteins based on their biological processes, molecular functions, and cellular components in sperm, as indicated in Figure 2. The most dominant biological processes in sperm across all age groups were cellular (GO:0009987) and metabolic processes (GO:0008152). Response to a stimulus (GO:0050896) was specifically expressed in the 2-year-old group (PRDX5 and TIMP2), while biological adhesion (GO:0022610) was present only in the ≥10-year-old group (IZUMO1). Locomotion, represented by the protein TEX101, was expressed in both 2 and 4-year-old groups. The 2-year-old group expressed proteins TEX101, ZPBP, and SPACA1 related to reproduction (GO:00000003) and reproductive processes (GO:0022414). The 4-year-old group had proteins TEX101, ZPBP, ADAM1B, and ADAM20 associated with reproduction, while the ≥10-year-old group contained ADAM20, ADAM1B, IZUMO1, and ZPBP. The most dominant molecular functions of sperm across all age groups were binding (GO:0005488) and catalytic activity (GO:0003824). The 2-year-old group expressed VAMP3 involved in molecular adaptor activity (GO:0060090), while the ≥10-year-old group had proteins that participated in structural molecule activity, particularly Tubulin (GO:0005198). ATP-dependent activity in the form of the ATP1A4 protein is only expressed in the age group 4 and over 10 years. Furthermore, the most dominant cellular component of sperm in all age groups is the cellular anatomical entity (GO:0110165).
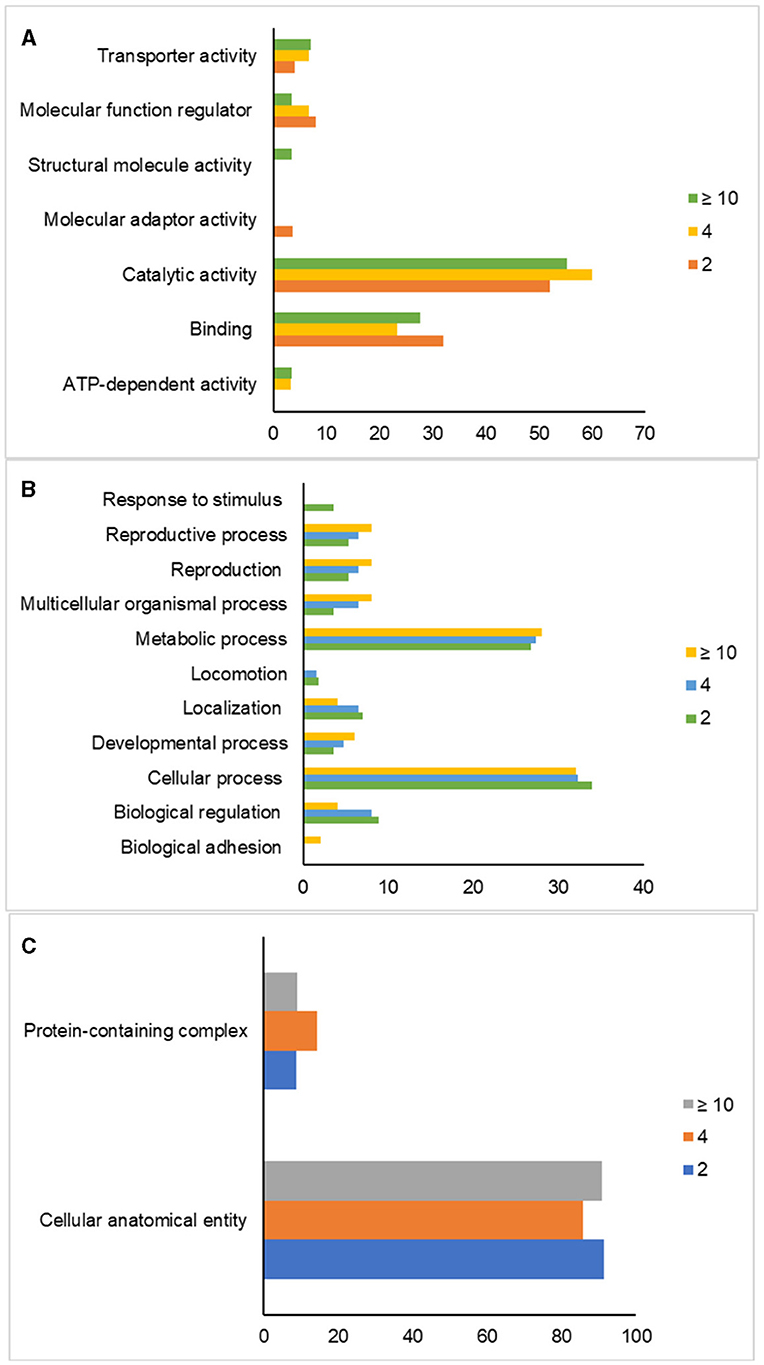
Figure 2. Gene ontology analysis of post-thaw sperm in different ages of bulls. Classification of proteins based on their molecular functions (A), biological processes (B), and cellular components (C) in sperm.
Post-thaw sperm protein interaction
The interactions between post-thaw sperm proteins in different age groups of Simmental bulls were analyzed using STRING, as indicated in Figure 3. Table 2 shows the direct interactions between these proteins related to reproductive processes. Furthermore, the results showed that the sperm proteins found in all age groups are linked to reproductive functions, such as the reproductive process (GO:0022414), sexual reproduction (GO:0019953), fertilization (GO:0009566), and single fertilization (GO: 0007338). Proteins expressed in the 2-year-old group were associated with acrosome assembly (GO:0001675), cellular component assembly involved in morphogenesis (GO:0010927), and spermatid development (GO:0007286). In contrast, those in the -year-old and 2-year-old groups were linked to binding sperm to zona pellucida (GO:0007339). Additionally, ≥10-year-old groups had proteins associated with sperm egg recognition (GO:0035036).
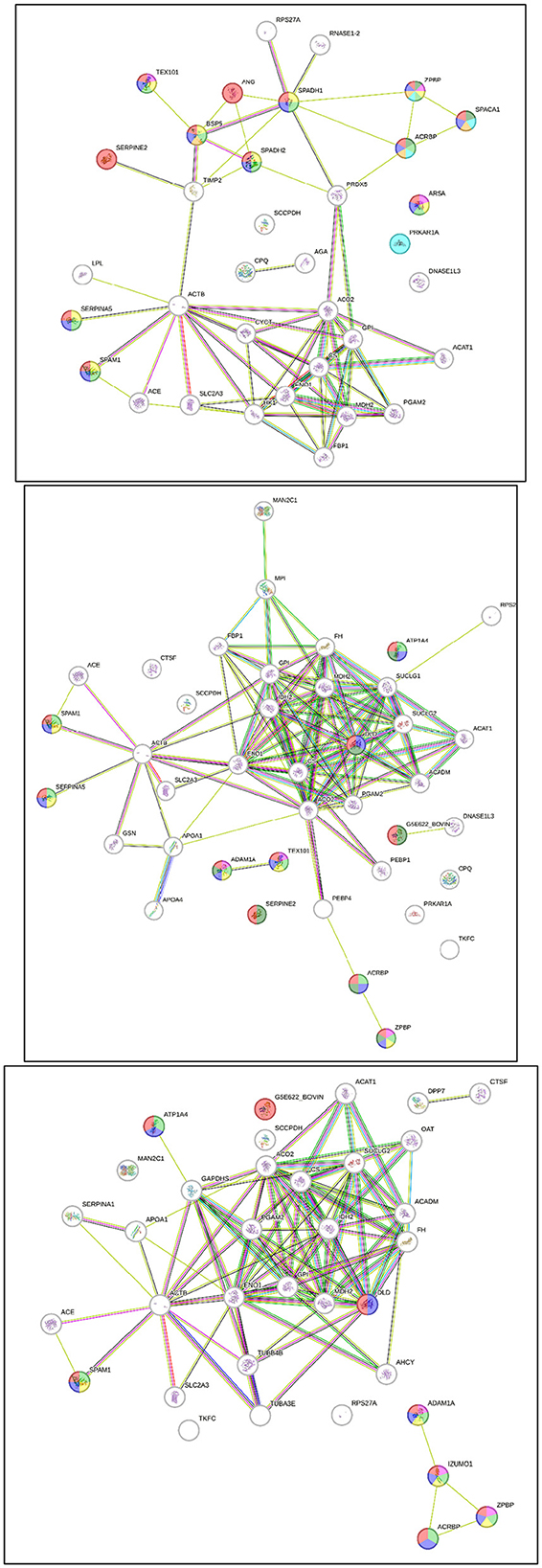
Figure 3. Post-thaw sperm protein interaction at different ages of bull, 2-year-old (top), 4-year-old (middle), ≥ 10-year-old (bottom).
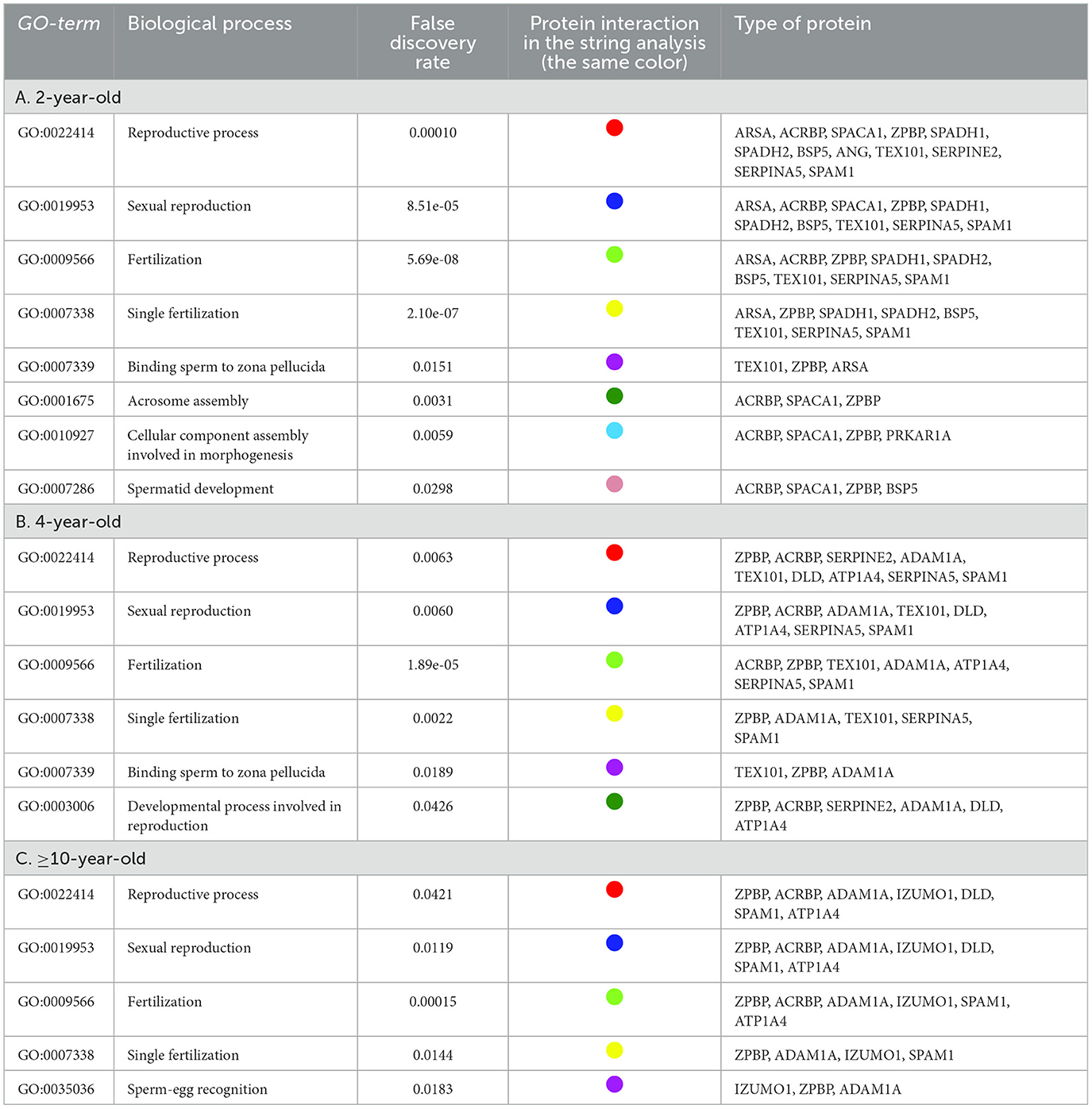
Table 2. STRING analysis of post-thawing sperm proteins at different ages of bulls related to the reproductive process.
Discussion
In this study, the expression of sperm proteins in different age groups of Simmental bulls and their relevance to sperm functions and fertility were examined. The results showed that proteins associated with essential sperm functions, particularly the metabolic process (GO:0008152), were expressed in all age groups of bulls. The sperm metabolic process is necessary for energy production, which fuels various sperm functions. Bull sperm fulfills its energy requirements through two main metabolic pathways: glycolysis and tricarboxylic acid cycle (28–30). Glycolysis breaks down sugar into pyruvate or lactate substrates, generating energy in the cytosol of sperm (30). These substrates then diffuse into the mitochondria through the pyruvate carrier and become decarboxylated by pyruvate dehydrogenase (PDH) to form acetyl-CoA, a citric acid cycle. The citric acid cycle produces ATP and other adenine derivatives, such as NADH and FADH2, which are converted into ATP through the oxidative phosphorylation pathway in the electron transport chain (30, 31).
Based on the analysis, several sperm proteins were found in all age groups of bulls, such as PGK2 (44.7 kDa; pl 8.32), ENO1 (47.3 kDa; pl 6.8), and GPI (62.8 kDa; pl 7.71), related to glycolysis function (GO:0006096). Furthermore, the analysis results also found sperm proteins such as ACO2 (85.3 kDa; pl 7.83), CS (51.7 kDa, pl 8.12), and MDH2 (35.6 kDa; pl 8.54), related to processes in the tricarboxylic acid cycle (GO:0006099). Previous studies reported these metabolic proteins associated with sperm functions: motility and fertility. Proteins such as PGK2 (32, 33), GPI (34), and ENO1 (16, 35) were involved in sperm motility, while decreased expression of mitochondrial proteins, including ACO2 and MDH2, could impact fertility (1, 16, 36). According to the results, the number of proteins associated with sperm metabolism might vary with age, potentially influencing fertility. This was consistent with previous reports indicating that aging in bulls could lead to mitochondrial dysfunction, affecting metabolic processes (37, 38).
Specifically, a sperm protein related to the process of acrosome assembly and spermatid development, known as SPACA1 (31 kDa; pl 4.85), was found in the 2-year-old group. SPACA1 interacting with ACRBP and ZPBP plays a role in the acrosome assembly function (GO:0001675), and SPACA1 interacting with BSP5 and ACRBP plays a role in the spermatid development function (GO:0007286). The impairment of SPACA1, often synthesized in the testis during spermatogenesis (35, 39), could lead to nuclear plate damage and an abnormal shape of the sperm head (40, 41). The expression of this protein indicated that the 2-year-old age group was still going through the sperm development process.
The 4-year-old group showed specific protein expressions, including PEBP1 (21 kDa; pI 7.49) and PEBP4 (25.4 kDa; pI 6.29). PEBP1 located in the acrosomal cap, post-acrosomal region, and flagella (42) inhibits sperm capacitation or acts as a decapacitation factor by binding to glycosylphosphatidylinositol (GPI)-anchored receptors (13, 22). Inhibiting post-thaw sperm capacitation in the 4-year-old age group permitted energy storage, ensuring the viability and quality of sperm while encountering the oocyte. PEBP4, expressed in the tail of spermatozoa, is associated with sperm motility regulation (43) performed through the Pi3k/Akt signaling cascade and serine/threonine phosphorylation (43, 44). Abundant expression of PEBP1 has been associated with high fertility in bull sperm (13, 22), and PEBP4 is more numerous in fertile bull sperm compared to the infertile counterpart (43).
Both the 2 and 4-year-old age groups expressed TEX101 (27.3 kDa; pI 6.49), which was a GPI-anchored glycoprotein synthesized in testicular germ cells (45) and found in the plasma membrane of spermatocytes, spermatids, and mature sperm (46, 47). TEX101 plays a role in single fertilization (GO:0007338) once interacting with proteins SPADH2, BSP5, SPADH1, ATP1A4, ZPBP, ARSA, SPAM1, and SERPINA5. Furthermore, it is involved in sperm binding to the zona pellucida (GO:0007339) during interaction with ZPBP and ARSA. The binding of TEX101 to cumulus cells induces calcium mobilization and progesterone production, facilitating the acrosome reaction and penetration of the cumulus-oocyte layer (47). The absence of TEX101 expression in older age groups may impact bull fertility since the protein has been validated as a fertility biomarker in mice (46, 47).
The proteins α-tubulin (49.9 kDa; pl 5.1) and β-tubulin (49.8 kDa; pl 4.89) were found to be expressed in the sperm of bulls aged more than 10 years, where these proteins were expressed explicitly in intracellular organelles (GO:0043229). This intracellular organelle, known as the cytoskeleton, plays a vital role in maintaining the morphological integrity of sperm (GO:0005200). These proteins form heterodimers, mainly constituting microtubules (48, 49), which play a role in hyperactive sperm motility during capacitation (48–51). Tubulin expression is associated with structural changes in sperm due to capacitation (48). Furthermore, various mechanisms are involved in the hyperactive movement, such as calcium and cAMP regulation, CATSPER channel functioning, flagella protein phosphorylation, and inhibition of dynein activity (51, 52). A previous study indicated a positive relationship between protein phosphorylation and tubulin distribution along the sperm flagellum during capacitation and acrosome reaction (50). The tubulin expression in post-thaw sperm aged ≥ 10 years suggests high sperm capacitation characterized by hyperactive movement.
Conclusions
This research shows that the age of bulls influences differences in the expression of the sperm proteome after thawing. The proteomes found in sperm in each age group are related to metabolic processes. Each age group has specific proteins that are expressed, such as SPACA1 and TEX101, which are only found in the 2 and 4-year age groups; PEBP1 and PEBP4, which are only found in the 4-year age group; and tubulin, which is only found in the two age groups over 10 years. Further research is highly expected from the findings of this research, including quantifying the proteomes expressed in the sperm of each age group and carrying out additional studies using in vivo and in vitro fertility level approaches. Furthermore, although the number of bulls used in this research is a limitation, the results can be an essential reference for further study with a concept similar to that of a more significant number of bulls.
Data availability statement
The data presented in the study are deposited in the jPOST repository, accession number JPST003244.
Ethics statement
Ethical approval was not required for the studies on animals in accordance with the local legislation and institutional requirements because only commercially available established cell lines were used.
Author contributions
FS: Conceptualization, Data curation, Investigation, Methodology, Validation, Visualization, Writing – original draft. NK: Conceptualization, Supervision, Validation, Writing – review & editing. MS: Conceptualization, Supervision, Validation, Writing – review & editing. EK: Conceptualization, Supervision, Writing – review & editing. BPP: Data curation, Formal analysis, Funding acquisition, Project administration, Validation, Visualization, Writing – review & editing. BP: Conceptualization, Formal analysis, Funding acquisition, Project administration, Supervision, Writing – review & editing.
Funding
The author(s) declare that no financial support was received for the research, authorship, and/or publication of this article.
Conflict of interest
The authors declare that the research was conducted in the absence of any commercial or financial relationships that could be construed as a potential conflict of interest.
Publisher's note
All claims expressed in this article are solely those of the authors and do not necessarily represent those of their affiliated organizations, or those of the publisher, the editors and the reviewers. Any product that may be evaluated in this article, or claim that may be made by its manufacturer, is not guaranteed or endorsed by the publisher.
References
1. Özbek M, Hitit M, Kaya A, Jousan FD, Memili E. Sperm functional genome associated with bull fertility. Front Vet Sci. (2021) 8:610888. doi: 10.3389/fvets.2021.610888
2. Carreira JT, Trevizan JT, Carvalho IR, Kipper B, Rodrigues LH, Silva C. Does sperm quality and DNA integrity differ in cryopreserved semen samples from young, adult, and aged Nellore bulls? Basic Clin Androl. (2007) 27:1–8. doi: 10.1186/s12610-017-0056-9
3. Trevizan JT, Carreira JT, Carvalho IR, Kipper BH, Nagata WB, Perri SHV, et al. Does lipid peroxidation and oxidative DNA damage differ in cryopreserved semen samples from young, adult and aged Nellore bulls? Anim Reprod Sci. (2018) 195:8–15. doi: 10.1016/j.anireprosci.2018.04.071
4. Westfalewicz B, Słowinska M, Judycka S, Ciereszko A, Dietrich MA. Comparative proteomic analysis of young and adult bull (Bos taurus) cryopreserved semen. Animals. (2021) 11:2013. doi: 10.3390/ani11072013
5. Satrio FA, Karja NWK, Setiadi MA, Kaiin EM, Gunawan M, Purwantara B. Post-thaw characteristics of the simmental sperm function in different ages of bulls. TASJ. (2022) 45:381–8. doi: 10.5398/tasj.2022.45.4.381
6. Collins WE, Inskeep EK, Dreher WH, TylerWJ, Casida LE. Effect of age on fertility of bulls in artificial insemination. J Dairy Sci. (1962) 45:1015–8. doi: 10.3168/jds.S0022-0302(62)89545-9
7. Jiang XP, Wang SQ, Wang W, Xu Y, Xu Z, Tang JY. Enolase1 (ENO1) and glucose-6-phosphate isomerase (GPI) are good markers to predict human sperm freezability. Cryobiology. (2015) 71:141–5. doi: 10.1016/j.cryobiol.2015.04.006
8. Zhao H, Ma N, Chen Q, You X, Liu C, Wang T, et al. Decline in testicular function in ageing rats: changes in the unfolded protein response and mitochondrial apoptotic pathway. Exp Gerontol. (2019) 127:110271. doi: 10.1016/j.exger.2019.110721
9. Johnson L, Grumbles JS, Bagheri A, Petty CS. Increased germ cell degeneration during postprophase of meiosis is related to increase serum follicle-stimulating hormone concentration and reduce daily sperm production in aged men. Biol Reprod, 42. (1990) 2:281–7. doi: 10.1095/biolreprod42.2.281
10. Bhakat M, Mohanty TK, Raina VS, Gupta AK, Khan HM, Mahapatra RK, et al. Effect of age and season on semen quality parameters in Sahiwal bulls. Trop Anim Health Prod. (2011) 43:1161–8. doi: 10.1007/s11250-011-9817-1
11. Belloc S, Hazouta A, Zini A, Merviel P, Cabry R, Chahine H, et al. How to overcome male infertility after 40: Influence of paternal age on fertility. Maturitas. (2014) 78:22–9. doi: 10.1016/j.maturitas.2014.02.011
12. Kipper BH, Trevizan JT, Carreira JT, Carvalho IR, Mingoti GZ, Beletti ME, et al. Sperm morphometry and chromatin condensation in Nelore bulls of different ages and their effects on in vitro fertilization. Theriogenology. (2016) 87:154–60. doi: 10.1016/j.theriogenology.2016.08.017
13. D'Amours O, Frenette G, Fortier M, Leclerc L, Sullivan R. Proteomic comparison of detergent-extracted sperm proteins from bulls with different fertility indexes. Reproduction. (2010) 139:545–56. doi: 10.1530/REP-09-0375
14. Pardede BP, Karja NWK, Said S, Kaiin EM, Agil M, Sumantri C, et al. Bovine nucleoprotein transitions genes and protein abundance as valuable markers of sperm motility and the correlation with fertility. Theriogenology. (2024) 215:86–94. doi: 10.1016/j.theriogenology.2023.11.015
15. Huang Z, Danshina PV, Mohr K, Qu W, Goodson SG, O'Connell TM, et al. Sperm function, protein phosphorylation, and metabolism differ in mice lacking successive sperm-specific glycolytic enzymes. Biol Reprod. (2017) 1–12. doi: 10.1093/biolre/iox103
16. Aslam MKM, Sharma VK, Pandey S, Kumaresan A, Srinivasan A, Datta TK, et al. Identification of biomarker candidates for fertility in spermatozoa of crossbred bulls through comparative proteomics. Theriogenology. (2018) 119:43–51. doi: 10.1016/j.theriogenology.2018.06.021
17. Selvam MKP, Agarwal A. Upadate on the proteomics of male infertility: a systematic review. Arab J Urol. (2018) 16:103–12. doi: 10.1016/j.aju.2017.11.016
18. Kusumawati A, Satrio FA, Indriastuti R, Rosyada ZNA, Pardede BP, Agil M, et al. Sperm head morphology alterations associated with chromatin instability and lack of protamine abundance in frozen-thawed sperm of Indonesian Local Bulls. Animals. (2023) 13:2433. doi: 10.3390/ani13152433
19. Peddinti D, Nanduri B, Kaya A, Feugang JM, Burgess SC, Memili E. Comprehensive proteomic analysis of bovine spermatozoa of varying fertility rates and identification of biomarkers associated with fertility. BMC Syst Biol. (2008) 22:19. doi: 10.1186/1752-0509-2-19
20. Kovac JR, Pastuszak AW, Lamb DJ. The use of genomics, proteomics, and metabolomics in identifying biomarkers of male infertility. Fertil Steril. (2013) 99:998–1007. doi: 10.1016/j.fertnstert.2013.01.111
21. Park Y-J, Kim J, You Y-A, Pang M-G. Proteomic revolution to improve tools for evaluating male fertility in animals. J Proteome Res. (2013) 12:4738–47. doi: 10.1021/pr400639x
22. Kasimanickam RK, Kasimanickam VR, Arangasamy A, Kastelic JP. Sperm and seminal plasma proteomics of high- versus low-fertility Holstein bulls. Theriogenology. (2019) 126:41–8. doi: 10.1016/j.theriogenology.2018.11.032
23. Bradford AM. A rapid and sensitive method for the quantitation of microgram quantities of protein utilizing the principle of protein-dye binding. Anal Biochem. (1976) 72:248–54. doi: 10.1006/abio.1976.9999
24. Candiano G, Bruschi M, Musante L, Santucci L, Ghiggeri GM, Carnemolla B, et al. Blue silver: a very sensitive colloidal coomassie g-250 staining for proteome analysis. Electrophoresis. (2004) 25:1327–33. doi: 10.1002/elps.200305844
25. Arshid S, Tahir M, Fontes B, de Souza Montero EF, Castro MS, Sidoli S, et al. High performance mass spectrometry-based proteomics reveals enzyme and signaling pathway regulation in neutrophils during the early stage of surgical trauma. Proteom Clin Appl. (2017) 11:1600001. doi: 10.1002/prca.201600001
26. Fu Q, Pan L, Huang D, Wang Z, Hou Z, Zhang M. Proteomic Profiles of Buffalo Spermatozoa and Seminal Plasma. Theriogenology. (2019) 134:74–82. doi: 10.1016/j.theriogenology.2019.05.013
27. Szklarczyk D, Franceschini A, Wyder S, Forslund K, Heller D, Huerta-Cepas J, et al. STRING V10: Protein-Protein Interaction Networks, Integrated over the Tree of Life. Nucleic Acids Res. (2015) 43:D447–52. doi: 10.1093/nar/gku1003
28. Moraes CR, Meyers S. The Sperm Mitochondrion: Organelle of Many Functions. Anim Reprod Sci. (2018) 194:71–80. doi: 10.1016/j.anireprosci.2018.03.024
29. Tourmente M, Villar-Moya P, Rial E, Roldan ERS. Differences in ATP generation via glycolysis and oxidative phosphorylation and relationships with sperm motility in mouse species. J Biol Chem. (2015) 205:20613–26. doi: 10.1074/jbc.M115.664813
30. Magdanz V, Boryshpolets S, Ridzewski C, Eckel B, Reinhardt K. The motility-based swim-up technique separates bull sperm based on differences in metabolic rates and tail length. PLoS ONE. (2019) 14:e0223576. doi: 10.1371/journal.pone.0223576
31. Pena FJ, Ortiz-Rodríguez JM, Gaitskell-Phillips GL, Gil MC, Ortega-Ferrusola C, Martín-Cano FE. An integrated overview on the regulation of sperm metabolism (glycolysis-Krebs cycle-oxidative phosphorylation). Anim Reprod Sci. (2021) 246:106805. doi: 10.1016/j.anireprosci.2021.106805
32. Pardede BP, Kusumawati A, Pangestu M, Purwantara B. Bovine sperm HSP-70 molecules: a potential cryo-tolerance marker associated with semen quality and fertility rate. Front Vet Sci. (2023) 10:1167594. doi: 10.3389/fvets.2023.1167594
33. Liu X, Li Q, Wang W, Liu F. Aberrant expression of sperm-specific glycolytic enzymes are associated with poor sperm quality. Mol Med Rep. (2019) 19:2471–8. doi: 10.3892/mmr.2019.9926
34. Guo Y, Jiang W, Yu W, Niu X, Liu F, Zhou T, et al. Proteomics analysis of asthenozoospermia and identification of glucose-6-phosphate isomerase as an important enzyme for sperm motility. J proteomics. (2019) 208:103478. doi: 10.1016/j.jprot.2019.103478
35. Harayama H, Minami K, Kishida K, Noda T. Protein biomarkers for male artificial insemination subfertility in bovine spermatozoa. Reprod Med Biol. (2017) 16:89–98. doi: 10.1002/rmb2.12021
36. Liang J, Zheng Y, Zeng W, Chen L, Yang S, Du P. Proteomic profile of sperm in infertile males reveals changes in metabolic pathways. Protein J. (2021) 40:929–39. doi: 10.1007/s10930-021-10013-w
37. Amaral S, Amaral A, Ramalho-Santos J. ging and male reproductive function: a mitochondrial perspective. Front Biosci. (2013) 5:181–97. doi: 10.2741/S365
38. Oberoi SLB, Kumar SVAS, Talwar CP. Study of human sperm motility post cryopreservation. Med J Armed Forces India. (2014) 70:349–53. doi: 10.1016/j.mjafi.2014.09.006
39. Pardede BP, Agil M, Supriatna I. Protamine and other proteins in sperm and seminal plasma as molecular markers of bull fertility. Vet World. (2020) 13:556–62. doi: 10.14202/vetworld.2020.556-562
40. Fujihara Y, Satouh Y, Inoue N, Isotani A, Ikawa M, Okabe M. SPACA1-deficient male mice are infertile with abnormally shaped sperm heads reminiscent of globozoospermia. Development. (2012) 139:3583–9. doi: 10.1242/dev.081778
41. Minami K, Arai-Aso MM, Ogura-Kodama Y, Yamada A, Kishida K, Sakase M, Fukushima M. Characteristics of bull sperm acrosome associated 1 proteins. Anim Repro Sci. (2020) 218:106479. doi: 10.1016/j.anireprosci.2020.106479
42. Arangasamy A, Kasimanickam VR, DeJarnette JM, Kasimanickam RK. Association of CRISP2, CCT8, PEBP1 mRNA abundance in sperm and sire conception rate in Holstein bulls. Theriogenology. (2011) 76:570–7. doi: 10.1016/j.theriogenology.2011.03.009
43. Somashekar L, Selvaraju S, Parthipan S, Patil SK, Binsila BK, Venkataswamy MM, et al. Comparative sperm protein profiling in bulls differing in fertility and identification of phosphatidylethanolamine-binding protein 4, a potential fertility marker. Andrology. (2017) 5:1032–51. doi: 10.1111/andr.12404
44. An LP, Maeda T, Sakaue T, Takeuchi K, Yamane T, Du PG, et al. Purification, molecular cloning and functional characterization of swine phosphatidylethanolamine-binding protein 4 from seminal plasma. Biochem Biophys Res Commun. (2012) 423:690–6. doi: 10.1016/j.bbrc.2012.06.016
45. Saleem TH, Okasha M, Ibrahim HM, El-Hamd MA, Fayed HM, Hassan MH. Biochemical assessments of seminal plasma zinc, testis-expressed sequence 101 and free amino acids and their correlations with reproductive hormones in male infertility. Biol Trace Elem Res. (2020) 199:1729–42. doi: 10.1007/s12011-020-02310-9
46. Fujihara Y, Tokuhiro K, Muro Y, Kondoh G, Araki Y, Ikawa M, et al. Expression of TEX101, regulated by ACE, is essential for the production of fertile mouse spermatozoa. Proc Natl Acad Sci U. (2013) 110:8111–6. doi: 10.1073/pnas.1222166110
47. Schiza CG, Jarvi K, Diamandis EP, Drabovich AP. An emerging role of TEX101 protein as a male infertility biomarker. EJIFCC. (2014) 25:9–26.
48. Francou MM, Ten J, Bernabeu R, Juan JD. Capacitation and acrosome reaction changes a-tubulin immunodistribution in human spermatozoa. Reprod BioMed Online. (2014) 28:246–50. doi: 10.1016/j.rbmo.2013.10.001
49. Kwon WS, Rahman MS, Lee JS, Yoon SJ, Park YJ, Pang MG. Discovery of predictive biomarkers for litter size in boar spermatozoa. Mol Cell Proteomics. (2015) 14:1230–40. doi: 10.1074/mcp.M114.045369
50. Sáez-Espinosa P, Ferrández-Rives M, Huerta-Retamal N, Robles-Gómez L, Aizpurua J, Romero A, et al. Proper cytoskeleton α-tubulin distribution is concomitant to tyrosine phosphorylation during in vitro capacitation and acrosomal reaction in human spermatozoa. Cytoskeleton. (2020) 77:333–41. doi: 10.1002/cm.21631
51. Mostek A, Westfalewicz B, Słowińska M, Dietrich MA, Judycka S, Ciereszko A. Differences in sperm protein abundance and carbonylation level in bull ejaculates of low and high quality. PLoS ONE. (2018) 13:e0206150. doi: 10.1371/journal.pone.0206150
Keywords: age, bull, LC-MS/MS, post-thawing sperm, proteomic
Citation: Satrio FA, Karja NWK, Setiadi MA, Kaiin EM, Pardede BP and Purwantara B (2024) Age-dependent variations in proteomic characteristics of spermatozoa in Simmental bull. Front. Vet. Sci. 11:1393706. doi: 10.3389/fvets.2024.1393706
Received: 29 February 2024; Accepted: 28 May 2024;
Published: 08 August 2024.
Edited by:
Manuel Garcia-Herreros, National Institute for Agricultural and Veterinary Research (INIAV), PortugalReviewed by:
Pablo Daniel Cetica, Universidad de Buenos Aires, ArgentinaEjaz Ahmad, Bahauddin Zakariya University, Pakistan
Copyright © 2024 Satrio, Karja, Setiadi, Kaiin, Pardede and Purwantara. This is an open-access article distributed under the terms of the Creative Commons Attribution License (CC BY). The use, distribution or reproduction in other forums is permitted, provided the original author(s) and the copyright owner(s) are credited and that the original publication in this journal is cited, in accordance with accepted academic practice. No use, distribution or reproduction is permitted which does not comply with these terms.
*Correspondence: Bambang Purwantara, cHVyd2FudGFyYSYjeDAwMDQwO2FwcHMuaXBiLmFjLmlk