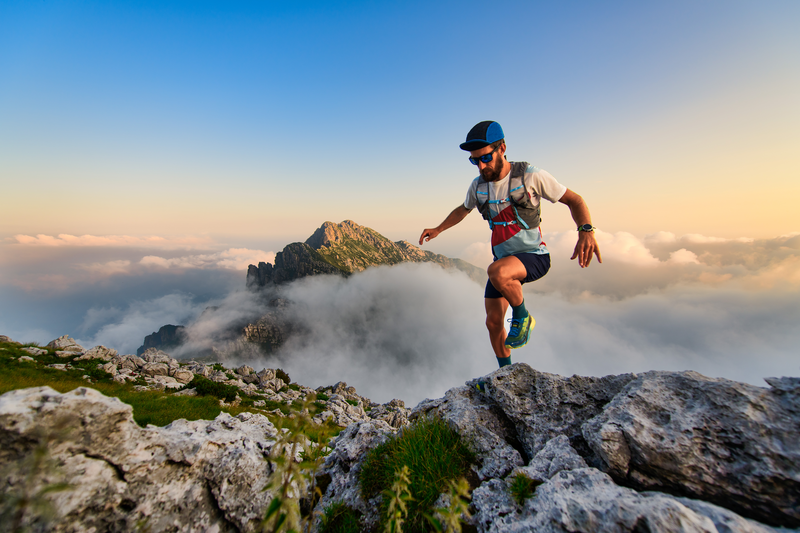
95% of researchers rate our articles as excellent or good
Learn more about the work of our research integrity team to safeguard the quality of each article we publish.
Find out more
ORIGINAL RESEARCH article
Front. Vet. Sci. , 24 May 2024
Sec. Animal Nutrition and Metabolism
Volume 11 - 2024 | https://doi.org/10.3389/fvets.2024.1389981
Examining the microbiological characteristics of pet food is imperative to safeguard the health and well-being of companion animals, pet owners, and the surrounding environment. Domestic animals, known for carrying harmful microorganisms, pose a significant health risk, especially in close proximity to people and children. Notably, no studies have previously investigated pet food quality in the Gulf Cooperation Council countries, in particular, the United Arab Emirates (UAE). This study examined the microbiological quality of all stock keeping units (SKUs) of pet foods marketed in UAE (n = 118). Parameters assessed include Total Aerobic Microbial Count (TAMC), Enterobacteriaceae, Total Yeast and Mold Count (TYMC), Salmonella, Listeria monocytogenes, and Clostridium species. Among the 118 samples, 33 (28%) exceeded the acceptable TAMC limit of 106 CFU/g, highlighting significant variations based on manufacturers and ingredients. Eight samples (7%) surpassed the maximum Enterobacteriaceae limit of 3 × 102 CFU/g. TYMC levels exhibited variation, with 33 (28%) exceeding the limit of 104 CFU/g. L. monocytogenes was identified in 44 (37%) of the samples, while Salmonella was not detected. Clostridium contamination was observed in 28 (24%) of the samples. Statistical analyses revealed associations between pet food characteristics and microbial quality, underscoring the imperative for international standards to ensure the safety of pet food. These findings carry significant implications for pet owners, regulatory bodies, and the pet food industry, emphasizing the need for ongoing efforts to enhance the overall quality and safety of pet food products.
• Dry pet food items exhibited greater levels of contamination in comparison to canned ones.
• (28%) samples had a total aerobic microbial count exceeding 106 CFU/g.
• 8 (7%) samples surpassed the maximum limit of 3×102 CFU/g for Enterobacteriacea.
• 33 (28%) samples had a contamination level of total yeasts and molds above 104 CFU/g.
• Listeria monocytogenes was present in 44 (37%) of the stock keeping units while Salmonella was not detected.
• Clostridium was detected in 28 (24%) of samples.
In contemporary times, the trend of pet ownership, notably centered around cats and dogs, is witnessing a significant surge worldwide. Statistics reveal that roughly ∼80 million households across Europe (1) and approximately 60% of households in the United States (2) embrace at least one pet. This upswing was particularly noticeable during the COVID-19 pandemic, highlighting the invaluable role of pets as companions, offering comfort and aiding individuals in managing stress, ultimately contributing to improved health (3). As the population of pets continues to rise, the pet food market is undergoing dynamic evolution. Since the 1940s, the manufacturing of pet food in Europe and the United States has been based on animal feeds initially intended for livestock, leading to the establishment of numerous pet food manufacturing facilities in most developed nations (4).
The safety of pet food is a paramount concern, not only for the well-being of pets but also for pet owners and environmental conservation. Apart from the nutritional value, ensuring the microbiological safety of pet food is a crucial criterion in delivering safe and healthy sustenance (5). Studies indicate that approximately 90% of pet owners in both the United States and Australia prefer commercial pet food due to its perceived convenience in meeting pets’ nutritional requirements and its cost-effectiveness compared to homemade alternatives (6, 7). Notably, there has been a discernible shift in feeding practices between 2008 and 2018, witnessing an increased inclination toward homemade or non-traditional diets. This shift is driven by perceptions of affordability, taste preferences, nutritional concerns, and apprehensions about added chemicals and preservatives (6–8). Additionally, both canned and dry commercial pet food may degrade in quality over time after purchase, despite initial assurances of safety and health (9). Various hazards lurk within pet food, posing potential health risks to pets. These hazards encompass chemical threats like cyanuric acid (10) and physical hazards such as metal and other hard particles (11). After processing, one of the pivotal aspects of ensuring pet food safety revolves around its microbiological quality, particularly regarding the presence or absence of zoonotic agents. Prior research has identified pathogens such as Enterobacteriaceae, Salmonella, L. monocytogenes, Clostridium species, and molds in both dry and canned pet food products (12–16).
Microorganisms present in pet food not only pose health risks to pets but also to their owners who share close bonds with them. Contaminated pet food has been implicated in human illnesses through various means, including direct interaction or indirect contact between humans and items contaminated with pet food. Moreover, some pets may carry diseases without displaying any symptoms themselves (17). To ensure the safety of pet food, regulatory bodies such as the Food and Drug Administration (FDA), the United States Department of Agriculture, and State feed agencies have established specific guidelines and regulations governing the manufacturing and labeling of pet food. Kukier et al. (18) emphasized the importance of microbiological quality in livestock feed, setting a maximum allowable total aerobic microbial count (TAMC) at 106 CFU/g.
According to EU regulations No 142/2011 (19) processed pet food samples, excluding canned pet food, exceeding 3 × 102 CFU/g of Enterobacteriaceae are deemed unsatisfactory for microbial hygiene. Notably, there are no explicit regulations specifying the limit of L. monocytogenes monocytogenes species in pet food (20). It is presumed that L. monocytogenes species should adhere to the standards established for human foods, requiring their absence in 25 g of the feed (21). Additionally, EU regulations (19) dictate that Salmonella must be entirely absent in 25 g of pet food, and the total count of yeasts and molds should not exceed >104 CFU/g (22). These stringent measures collectively aim to uphold the microbial safety and quality of pet food products.
In the United Arab Emirates (UAE) and the broader Middle East and North Africa (MENA) region, there has been a recent surge in pet ownership. Nevertheless, comprehensive data remains scarce. To the best of our knowledge, no studies have been undertaken on the microbiological safety of pet food in the Gulf Cooperation Council, including the UAE. Furthermore, only one study has been conducted in the Arab region, specifically in Lebanon (16); however, it is important to emphasize the regional specificity in assessing the microbiological safety of pet food products. The United Arab Emirates (UAE) and Lebanon are distinct regions with differences in environmental conditions, manufacturing practices, transportation logistics, and regulatory frameworks. These variations can significantly impact the microbial composition and safety of pet food products. Furthermore, the UAE is a rapidly growing market with unique socio-economic factors and cultural influences that may affect pet food production, distribution, and storage. Therefore, it is crucial to evaluate the microbiological safety of pet food specifically in the UAE to provide region-specific data for consumers, regulatory authorities, and pet food manufacturers. This study addresses the gap in current knowledge by conducting a comprehensive investigation into the microbiological safety of pet food in the UAE, contributing essential data to enhance awareness and inform regulatory measures.
To assess a diverse array of commercially available dog and cat foods across the United Arab Emirates (UAE), the market got screened and 118 stock keeping units (SKUs; 91 canned products and 27 dry products) were identified and gathered from various pet food stores and supermarkets throughout the country in 2023. Table 1 provides a comprehensive breakdown of the SKU details, encompassing sample type, pet type, pet age, protein source, grain or grain-free composition, and country of origin. All collected samples were maintained in their original packaging and remained unopened until analysis. Each sample underwent two separate tests for Salmonella and L. monocytogenes spp. detection, enumeration of Enterobacteriaceae spp., detection of Clostridium spp. and evaluation of total aerobic microbial count (TAMC) and total yeasts and molds count (TYMC).
The processing and dilution of the samples adhered to the standard ISO 6887–1:2017b − 5 protocol (23). A 25 g portion from each sample was combined with nine times its volume (∼225 mL) of buffered peptone water (Biolife, Italy) and homogenized for 1–2 min using a stomacher (BagMixer 400 W, interscience, France). Subsequently, a 10-fold serial dilution in 0.1% (v/v) peptone water was prepared. A volume of 0.1 mL from the mother solution (MS) and each diluted mixture was separately transferred onto petri dishes. The detection of certain microorganisms (Salmonella, L. monocytogenes and Clostridium species) and enumeration of others (TAMC, TYMC, and Enterobacteriaceae species) occurred following specific incubation periods and temperatures. The examination of pet food samples complied with established standards governing the microbiology of food and feeding materials (24).
Total aerobic microbial count (TAMC): Each sample underwent dilution stages of 10−1, 10−2, 10−3, 10−4, 10−5, and 10−6. A 0.1 mL aliquot from each dilution was spread onto plate count agar (PCA) agar (Biolife, Italy) for incubation at 37°C ± 1°C for 42 h. All developed colonies on the plates were enumerated.
Enterobacteriaceae enumeration: The samples underwent dilution at the following ratios: 10−1, 10−2, 10−3, 10−4, 10−5, and 10−6. Subsequently, 0.1 mL from each dilution was plated on Violet Red Bile Glucose (VRBG) agar (Biolife, Italy) for incubation at 37°C ± 1°C for 48 h. Colonies displaying red coloration with red-pink halos on the plates were considered presumptive Enterobacteriaceae species and were enumerated.
Yeasts and molds enumeration: Samples underwent dilutions at 10−1, 10−2, 10−3, 10−4, 10−5, and 10−6 and 0.1 mL from each dilution was spread onto Sabouraud agar (Himedia, India) for incubation at 25°C ± 1°C for 5 days. Colonies observed on the plates were suspected to be yeasts and molds.
Salmonella detection: Isolation of Salmonella spp. involved a two-step enrichment process. After incubating samples at 37°C ± 1°C for 24 h in Buffered Peptone Water, inoculations were made into Rappaport-Vassiliadis RVS broth and tetrathionate broth (Biolife, Italy). Following incubation at 42°C ± 1°C for 24 h, plating onto XLD and Salmonella Shigella Agar (Biolife, Italy) occurred and subsequent incubation at 37°C for 24 h. The appearance of black colonies post-incubation indicates presumptive Salmonella species.
L. monocytogenes detection: Enrichment involved incubation at 37°C ± 1°C for 24 h in Buffered Peptone Water, followed by inoculation into Frazer broth and incubation at 42°C ± 1°C for 24 h. Smears from the broth were spread onto Palcam agar (Biolife, Italy), and the appearance of black colonies post-incubation at 37°C ± 1°C for 24 h implies the presence of presumptive L. monocytogenes species.
Clostridium detection: The samples underwent dilution at the following ratios: 10−1, 10−2, 10−3, 10−4, 10−5, and 10−6. A 0.1 mL volume of each dilution was plated onto Blood Agar Base (Biolife, Italy) supplemented with 5–7% sterile defibrinated horse blood. These plates were then transferred into an anaerobic jar to create anaerobic conditions using OXOID AnaeroGen, Anaerobic generator sachets in 2.5 L jar. Incubation was carried out at 37°C ± 1°C for 48 h. Colonies generated by Clostridium species frequently exhibit distinct characteristics, including irregular edges, central protrusion, and a visual aspect reminiscent of “ground glass.”
Following ISO 7218 guidelines (24), the presence and quantity of microorganisms were analyzed, expressing microbial counts as the logarithm of colony-forming units per gram of sample.
Results, including concentrations of Total Aerobic Microbial Count (TAMC), Enterobacteriaceae, and Total Yeast and Mold Count (TYMC), were systematically categorized based on their proximity to the acceptable limit. Specifically, they were classified as either falling below the acceptable limit, signifying acceptability, or exceeding it, indicating unacceptability. The set limits were 106 for TAMC, 104 for TYMC, and 300 CFU/g (colony-forming units per gram) for Enterobacteriaceae.
Information regarding pet food products and laboratory analysis results were encoded and entered into SPSS V26 for subsequent analysis. Microorganism concentrations, including TAMC, Enterobacteriaceae, and TYMC, were regrouped and subjected to bivariate analysis using Pearson Chi-square, or fisher exact test when expected cell sizes were small, to assess the effect of pet food characteristics. Statistical significance was denoted by a p-value <0.05.
For TAMC, TYMC, and Enterobacteriaceae, concentrations ranged from below the quantification limit to exceeding 3 × 107 CFU/g. Within the TAMC and TYMC categories, 33 samples (28%) exhibited contamination levels surpassing the established limit, while 85 samples (72%) recorded contamination levels below it. Conversely, Enterobacteriaceae was detected in 8 samples (7%).
Salmonella and L. monocytogenes results were stratified based on the presence or absence of these bacteria, complemented by an additional evaluation for Clostridium. L. monocytogenes species were identified in 44 samples (37%), whereas Salmonella species were absent in all samples. Furthermore, 28 (24%) samples were found to be contaminated with Clostridium, with contamination levels ranging from below the quantification limit to 3 × 103 CFU/g. This meticulous categorization ensures a thorough assessment of microbial quality, verifying compliance with specified limits, and offering valuable insights into potential food safety concerns.
Tables 2–7 present statistical findings pertaining to the microbiological quality of both dry and canned pet food. A noteworthy correlation was observed between the nature of pet food (dry/canned) and the contamination level of TAMC at a significance level of p < 0.05. Additionally, a significant disparity was identified in the contamination with L. monocytogenes species based on the type of pet (dog/cat). Furthermore, a significant distinction emerged between the country of origin and the contamination levels of both TAMC and Clostridium species.
Table 3. Microbiological results of total aerobic microbial count (TAMC) in dry and canned pet food.
Table 4. Microbiological results of presumptive Enterobacteriaceae species in canned and dry pet food.
The range of potential biological hazards present in pet food, capable of causing illnesses in animals if not vigilantly monitored, encompasses Enterobacteriaceae, Clostridium, yeasts and molds, Salmonella, and L. monocytogenes (25). As emphasized by Kim et al. (26), the global assurance of food safety and the minimization of food loss necessitate a comprehensive monitoring approach, particularly focusing on biological hazards, throughout the entire food supply chain. For instance, the microbiological quality of meat is contingent on various factors such as the animal’s physiological condition during slaughter, processing methods, as well as storage and transportation conditions (27).
This investigation underscores that both canned and dry pet food products have the potential to harbor food-borne pathogens, including Enterobacteriaceae, Clostridium, L. monocytogenes, and fungi. Consequently, pet owners are strongly advised to exercise caution and adopt stringent safety measures when handling pet food. This precautionary approach is imperative to mitigate the risks associated with these contaminants and safeguard the health of companion animals.
The proliferation of microorganisms in food can lead to undesirable changes, resulting in spoilage and posing potential health risks to consumers. Although there are no stringent regulations specifying maximum permissible levels of bacterial and fungal contamination in pet food (20, 28), Kukier et al. (18) recommend that TAMC should not exceed 106 CFU/g.
Our research reveals a wide range of TAMC in pet food samples, ranging from below the quantification limit (BQL) to values exceeding 3 × 107 CFU/g. Significant variability exists among samples from different manufacturers and among samples from the same manufacturer with distinct main ingredients or intended for different pet groups (specific data not presented). Notably, 28% (33 samples) exhibited TAMC contamination levels exceeding 106 CFU/g. This contrasts with findings from other studies, such as Holda et al. (12) reporting a 75% contamination rate in dry foods with lower ranges (1.0 × 101 to 2.7 × 102 CFU/g) and Kazimierska et al. (28) and Serhan et al. (16) showing lower percentages in European and Lebanese markets, respectively.
The microbiological quality of animal feed, including pet foods, is often compromised by unhygienic conditions during preparation, distribution, and storage, posing health risks to both humans and pets (29). Factors influencing microorganism multiplication during storage include pH, water, light, time, nutrients, inhibitors, and oxygen (30, 31). It is imperative to translate these findings into actionable regulatory measures for the industry, including comprehensive labeling requirements for pet food, adherence to Generally Recognized as Safe (GRAS) standards set by the Association of American Feed Controls Officials (AAFCO) or approved food additives, and strict adherence to good manufacturing practices (GMP), ideally with AAFCO affiliation (32). Rigorous record-keeping and proper storage practices are vital components of GMP, ensuring the integrity of pet food throughout production and distribution. Implementing HACCP is essential to minimize hazards, particularly microbial contamination, through thermal treatments to eliminate pathogens and proactive risk identification and mitigation throughout the production process.
Dry pet food showed a higher prevalence of samples with TAMC levels deemed unacceptable (p-value = 0.030), indicating a significant variance in susceptibility to microbial contamination compared to canned pet foods. This aligns with findings from Lebanon (16) and underscores the importance of considering pet food type for microbiological safety. The elevated risk associated with dry pet food may necessitate stricter quality control measures due to its composition and storage requirements. The primary factors contributing to pathogenic contamination include deviations from GMP and potential for cross-contamination from various sources (33). Furthermore, dry pet products show an increased susceptibility to bacterial contamination following heat treatment, unlike canned alternatives. Canned foods are generally considered safer against biological hazards like bacteria and parasites due to the typical sterilization of cans (34). Extended storage of opened dry foods, driven by their substantial feed content, may contribute to re-contamination, whereas cans and canned foods are typically consumed in a single use. The challenging weather conditions in the United Arab Emirates (UAE), characterized by high temperatures, pose obstacles in maintaining optimal storage conditions for dry pet food. The region’s elevated ambient temperatures and humidity fluctuations create an accelerate microbial growth, further compromising food quality. Manufacturers must therefore implement rigorous quality control measures, adhere to recommended storage conditions, and explore packaging solutions tailored to mitigate contamination risks in the UAE’s climate.
Pet foods intended for cats showed a slightly higher adherence to acceptable TAMC levels compared to pet foods intended for dogs. Samples from puppies or kitten samples show a slightly lower compliance rate than adult pet samples. However, there were no statistical significance for both categories (p-value = 0.244 and p-value = 0.334 respectively).
TAMC levels in grain-free foods (40.00%) show a higher percentage of contamination compared to grain-containing foods (23.90%). Nonetheless, with a p-value of 0.095, the observed difference suggests a lack of statistical significance between the two. The non-significant p-value implies that the observed disparity in microbial counts between samples with and without grains could be due to random chance. However, it is crucial to acknowledge the trend indicating a higher TAMC in samples containing grains. Our research reveals contrasting findings compared to the study conducted in Lebanon by Serhan et al. (16), which illustrated that dry samples containing grains showed higher TAMC contamination than grain-free samples.
In scrutinizing individual ingredients within pet food formulations, such as poultry, fish, beef, liver, and turkey, discernible variations in microbial quality become apparent. Notably, poultry-based pet foods demonstrate a significantly higher proportion of samples adhering to acceptable TAMC levels compared to formulations devoid of poultry. Similar patterns are observed for fish and liver components, where their inclusion correlates with elevated proportions of samples meeting acceptable TAMC criteria. These findings emphasize the influence of ingredient composition on the microbial quality of pet food. The observed patterns emphasize the importance of considering specific ingredients when assessing the microbiological safety of pet food formulations.
The microbial quality of pet food samples significantly varies by the country of origin (p-value = 0.002). Pet foods sourced from the United States demonstrate the highest percentage of samples (90.50%) conforming to acceptable TAMC levels, indicative of superior overall microbiological quality. Conversely, pet foods from Hungary display a higher percentage of samples (61.10%) with unacceptable TAMC levels. These disparities underscore the importance of considering global variations in manufacturing practices, storage conditions, and ingredient sourcing. International collaboration and standardization are crucial to ensure consistent, high-quality pet food products worldwide.
In accordance with EU regulations No 142/2011 (19), pet food samples, excluding canned ones, containing dog chews and processed products that surpass the threshold of 3 × 102 CFU/g for Enterobacteriaceae are deemed unsatisfactory in terms of microbial hygiene.
Presumptive Enterobacteriaceae was identified in 12 out of the 118 samples examined, constituting around 10% of the total. Among the 12 positive samples, 8 (7%) surpassed the limit, indicating elevated contamination. The Enterobacteriaceae levels in our investigation ranged from below the quantification limit (BQL) to more than 3 × 107 CFU/g.
Our results reveal lower levels of Enterobacteriaceae contamination compared to previous studies, such as the one conducted by Kazimierska et al. (35). In their study, Enterobacteriaceae were identified in six samples of dog food (30%) after 24 h of incubation, simulating conditions typical of home storage. Additionally, in the study by Wojdat et al. (36), it was reported that 10% of dry pet food samples in Poland exceeded the contamination level of 3 × 102 CFU/g. Additionally, 30% of the pet food samples from Lebanon were contaminated with Enterobacteriaceae (16). Conversely, Holda et al. (12) reported a higher contamination rate of 60% in dog food samples in Poland. Hellgren et al. (37) demonstrated pathogenic bacteria presence in raw pet food, with 60% exceeding maximum levels. Another study in Switzerland revealed that 72.5% of raw pet food samples did not meet EU microbiological regulations (38). Raw food’s elevated contamination, attributed to the lack of heating and processing, was evident in the screening for extended-spectrum beta-lactamase-producing Enterobacteriaceae, isolated from 77.8% of raw pet food and 0% from non-raw pet food (39).
It is important to take into account the methodological disparities between our study and prior research, as these could be influential in explaining discrepancies in outcomes. An evident contrast lies in the sampling methodologies utilized across studies. For example, Kazimierska et al. (35) simulated scenarios typical of home storage conditions, potentially resulting in elevated contamination rates due to prolonged exposure to environmental factors. Variations in microbial detection methods and pet food processing procedures like incubation periods, culture media, and identification protocols may lead to varying outcomes regarding Enterobacteriaceae presence and quantity. Moreover, discrepancies in pet food processing, such as heating duration and environmental conditions, can affect microbial survival and proliferation. These factors highlight the need for standardized methodologies and consideration of environmental variables in assessing contamination levels in pet food.
Carvalho et al. (40) emphasized that pets, particularly dogs, are significant sources of multiresistant Escherichia coli strains in households, posing health risks through various transmission routes. Takahashi et al. (41) noted that food manufacturers consider Enterobacteriaceae an indicator of hygiene, implying poor sanitation or improper processing in pet food production. Certain Enterobacteriaceae, like E. coli and Enterobacter spp., can lead to extraintestinal opportunistic infections in dogs, including urogenital infections, meningitis, sepsis, and surgical site infections (42). This underscores the importance of monitoring and improving hygiene practices in the pet food processing environment to mitigate health risks for both animals and humans.
In our examination of Enterobacteriaceae contamination across various pet food categories, we found that dry pet food exhibits a higher contamination rate compared to canned food. However, this difference lacks statistical significance (p-value = 0.079). Our results align with Serhan et al.’s (16), who found higher contamination with Enterobacteriaceae contamination in dry samples, likely due to prolonged survival in low-moisture conditions (43). Similar trends in infant formula production suggest risks during the dry phase (44). However, our findings contradict studies by Kukier et al. (18) and Kepińska-Pacelik et al. (20), which indicated a higher level of Enterobacteriaceae contamination in canned foods compared to dry foods.
Dogs demonstrate slightly higher levels of Enterobacteriaceae contamination compared to cats. Among adults, there is a higher contamination rate than among puppies or kittens, the latter consistently displaying a 100% acceptance rate, indicating no contamination above the limit. Statistical analysis yields p-values of 0.664 and 0.999, revealing no significant difference between pet type and age, respectively. Our findings are consistent with Kazimierska et al. (35), whose analyses similarly do not support the claim that canned dog food formulated for various age groups exhibits differences in microbiological purity.
Our study revealed that the inclusion of grains in pet food does not significantly affect contamination levels. This finding aligns with previous research examining the microbiological quality of canned foods for both puppies and adult dogs, which found no confirmed association between the inclusion of a grain component in canned dog food and an increased risk of microbiological contamination by Enterobacteriaceae (35). However, it is essential to highlight that our results contrast with those found in pet food samples obtained from Lebanon. In this context, dry pet food containing grains showed increased contamination of Enterobacteriaceae compared to grain-free alternatives, as indicated by the study conducted by Serhan et al. (16).
Across various food sources and countries of origin, the majority of samples meet acceptable criteria, highlighting the overall safety of pet food. It is important to note that microbiological standards, which specify permissible microorganism levels in pet food, are enforced in the UAE. This stands in contrast to Lebanon, where Asian countries demonstrated an approximately 26% rate of unacceptable Enterobacteriaceae contamination (16).
Cereals, a crucial component in pet food, have the potential to serve as carriers for harmful mycotoxins produced by molds, posing a health risk to both pets and their owners (45). The permissible limit for the total count of yeasts and molds should not exceed 104 CFU/g (22). In the present investigation, contamination levels varied from 0 to 3 × 107 CFU/g. Out of the samples, 33 (27%) surpassed the established contamination limit.
Our findings differ from those presented by Kazimerska (35) where no TYMC contamination was identified in dog pet foods samples, and by Holda et al. (12), who found no fungal contamination exceeding 2 × 102 CFU/g. The sample sizes across studies vary significantly, with Kazimierska investigation comprising 20 samples of commercial canned dog foods, while Holda’s study involved four complete dry foods tailored for growing dogs. In contrast, our research encompasses a notably larger sample size of 118 pet food samples. This considerable difference in sample size might have implications for the observed levels of yeast and mold contamination since smaller sample sizes may not capture the full spectrum of the bacterial contamination. It is plausible that the variation in sample size could contribute to the comparatively lower contamination levels identified in our study, highlighting the importance of sample size considerations in microbial contamination research.
Moreover, our results surpass those of several studies. Serhan et al. (16) reported that 12% of the samples exhibited contamination levels surpassing 104 CFU/g, while Wojdat et al. (36), found a 9% contamination level above 104 CFU/g. Additionally, Kazimierska et al. (28) found that molds, potentially accountable for the presence of mycotoxins, were detected in approximately 20% of the examined dog food samples. Furthermore, in their assessment of pet food’s microbiological quality Bueno et al. (46) observed that all tested commercial dry dog food samples were contaminated with yeasts and molds.
Certain molds and certain yeasts found in food can be harmful to animals due to their capacity to generate mycotoxins. The introduction of these toxins can occur through various pathways. Factors influencing mycotoxin production include weather, presence of inhibitors and nutrients, geographical and seasonal variations, humidity, temperature, crop susceptibility, cultivation methods, harvesting practices, as well as storage and transportation procedures (47). Understanding these factors is crucial for managing mycotoxin risk. For instance, weather and seasonal changes affect pre-harvest mold development, while humidity and temperature during storage/transportation impact post-harvest contamination. Cultivation methods and harvesting practices also influence crop vulnerability to mold infestations.
Dry samples show a slightly higher TYMC contamination rate compared to canned samples, but the computed p-value of 0.479 suggests that there is no statistically significant difference, suggesting comparable microbiological quality for both types. In 2014, research conducted by Blajet-Kosicka et al. (15) revealed that various molds, including Aspergillus, Mucor, and Penicillium, as well as a range of mycotoxins, were identified in dehydrated pet food.
Analyzing both pet type and age reveals insights into microbial contamination levels. Dogs exhibit a slightly higher percentage of samples within acceptable TYMC limits compared to cats. Similarly, adult pet food samples demonstrate a slightly higher percentage within acceptable TYMC limits compared to samples for puppies or kittens. The p-values of 0.717 and 0.445 for pet type and pet age, respectively, indicate no significant association between them and TYMC levels. These findings underscore the importance of considering nutritional requirements, ingredient preferences, and formulation processes specific to each pet species and age group, as these factors could impact microbial contamination patterns.
The calculated p-value of 0.971 for grain and grain-free pet food suggests no significant difference in microbial contamination based on the presence of grains. This contrasts with previous studies, noting the correlation observed between mold presence and cereals in dog food. Specifically, yeast and mold were more frequently detected in dog foods containing grains, with six out of seven positive results corresponding to grain-included foods, accounting for 86% of the cases (28). Analyzing pet food samples from Lebanon, no significant correlation was observed between grain presence and TYMC levels. However, it was noted that all eight samples (12%) exceeding the TYMC limit contained at least one cereal (maize, rice, wheat, and/or oats) (16). Although our study did not find a significant link between the presence of grains and TYMC levels in pet food, further investigation into this discrepancy is warranted. One possible reason could be the diversity in processing methods utilized by pet food manufacturers. Varied processing methods utilized by pet food manufacturers may impact the susceptibility of grains to mold contamination and subsequent mycotoxin production. Additionally, regional disparities in agricultural practices and grain procurement methods could contribute to fluctuations in microbial contamination levels among different batches of pet food.
An analysis of various food sources including poultry, fish, beef, liver, and turkey reveals high percentages of samples within acceptable TYMC limits, indicating good overall microbiological quality. Most p-values for various food sources are above 0.05, suggesting no significant association between specific food sources and TYMC levels. However, variations in the percentage of samples within acceptable TYMC limits are observed among pet food from different countries, although the associated p-value of 0.144 suggests a potential association between the country of origin and TYMC levels, it does not reach statistical significance. These findings highlight the importance of exploring specific ingredients, processing methods, and geographical sources to identify potential sources of contamination and ensure consistent microbiological quality across different pet food products.
Exploring the prevalence of Salmonella in pet food reveals a multifaceted landscape, highlighting global implications for pet food safety. The absence of Salmonella in our tested samples aligns with stringent EU regulations (19) and corroborates findings from D’aoust et al. (48) and Kazimierska (35), providing a reassuring outlook on the safety of pet food in some regions. A study conducted in Poland revealed that only 0.96% of dry pet food products and none of the canned ones tested positive for Salmonella (49). This contrasts with a broader examination of microbial prevalence in pet food, indicating an 8% positive rate for Salmonella species, with all positive cases attributed to raw feed and only 1 case found in dry pet food (14). In Sweden, Hellgren et al. (37) reported a 7% contamination rate in tested raw meat-based products, while Yukawa et al. (50) observed a 2% incidence of Salmonella in dog treats collected in Japan. However, our results stand in contrast to a study conducted in Lebanon, where Salmonella was detected in 41% of the total pet food samples (16). These findings underscore the variability in Salmonella prevalence in pet food across different geographical locations, reinforcing the importance of regional variations.
To thoroughly assess Salmonella prevalence in pet food, we must examine the methodologies used across studies. While many employ culture-based methods, some variations exist, potentially impacting sensitivity levels compared to molecular techniques like PCR, as employed by Yukawa et al. (50). Variations in sample sizes and methodologies may introduce bias; for instance, some studies tested fewer than 100 samples (35, 37, 48), while others examined over 1,000 (14, 49). These differences, alongside variations in sample collection and storage, may affect result reliability. It is crucial to note that each study was conducted in specific regions, underscoring the importance of acknowledging inherent limitations such as potential selection bias and inability to capture temporal fluctuations in Salmonella prevalence.
An alarming instance in the United States involved an outbreak of Salmonella Schwarzengrund associated with dry dog and cat food from a specific manufacturer, leading to 79 identified cases (13). Notably, no Salmonella species were found in any of the pet food samples from the UAE, mitigating the potential transmission of zoonotic pathogens, such as Salmonella, from pets to humans. While the likelihood of transmission from pets to humans is generally rare, it is essential to acknowledge that some pets may carry diseases asymptomatically. Dogs and cats, even without apparent symptoms, can act as carriers for extended durations, potentially infecting individuals who handle contaminated pet food or have direct contact with these animals. Importantly, asymptomatic pets can shed Salmonella for up to 3 months (51), underscoring the necessity for ongoing vigilance and adherence to safety measures when handling pet food.
Enforcing stringent safety protocols during pet food handling and production, including thorough sanitation, pathogen screening, and regulatory adherence, is vital for averting Salmonella contamination. Educating pet owners about potential hazards and encouraging careful feeding practices further reduces the risk of transmission between animals and humans.
In relation to L. monocytogenes, its presence should be either undetectable in 25 g of pet food or its contamination level must be less than 100 CFU/g (21). In our investigation, L. monocytogenes species were identified in 44 out of 118 samples, accounting for 37%. This contamination level was lower than that documented for pet food in Lebanon, where it reached 64% (16), but higher than a previous study by Nemser et al. (14), which revealed 16% positive samples for L. monocytogenes monocytogenes and 14% for another L. monocytogenes spp. Additionally, none of the 20 analyzed canned pet foods in Poland showed any evidence of L. monocytogenes contamination (35).
As outlined by the Center for Veterinary Medicine (17), L. monocytogenes spp. can elicit mild gastrointestinal symptoms, fever, muscle pain, breathing difficulties, pregnancy loss, and even fatality. Pet owners should be aware that, although certain cats and dogs may not exhibit signs of Listeriosis following the consumption of contaminated pet food, they can still serve as carriers of L. monocytogenes monocytogenes, excreting it in their stool and subsequently spreading it within the household. Given the close relationship between pets and their owners, understanding and addressing L. monocytogenes contamination in pet food is crucial.
The data reveals a significant disparity in L. monocytogenes prevalence between dry and canned pet food. Dry pet food exhibits a higher positive rate compared to canned pet food. Nevertheless, the p-value of 0.673 suggests that this distinction lacks statistical significance, implying that the type of pet food may not be a major contributor to L. monocytogenes presence. A study conducted by Mireille et al. (16) identified a notable difference between dry and canned foods, with dry foods showing a higher L. monocytogenes contamination rate than canned ones.
When examining the type of pet, the data indicates that cats exhibit a higher positive rate for L. monocytogenes compared to dogs. The p-value of 0.019 suggests a significant disparity in L. monocytogenes prevalence between cats and dogs, with cats appearing more susceptible. Additionally, Lebanese pet food products for dogs and cats showed a significant distinction in L. monocytogenes monocytogenes prevalence (16). When discussing the higher prevalence of L. monocytogenes in cats compared to dogs, several factors should be considered. One possible explanation is the dietary differences between the two species. Cats’ more acidic digestive systems compared to dogs’ may affect their susceptibility to L. monocytogenes infection. Conversely, dogs being omnivores, can tolerate a wider range of foods, including plant-based ingredients (52), potentially resulting in lower L. monocytogenes contamination in their food. Differences in feeding behaviors, such as scavenging habits and food preferences, could also contribute to the observed disparities. Moreover, cats, especially those allowed outdoors, may have increased exposure to potential sources of L. monocytogenes contamination like rodents or contaminated soil, while indoor cats may also be exposed to L. monocytogenes through contaminated pet food or contact with humans who handle raw meat. Conversely, dogs, especially with wildlife may have fewer opportunities for exposure to L. monocytogenes in their environment.
Age appears to influence L. monocytogenes prevalence, with adult pets demonstrating a lower positive rate compared to puppies or kittens. Although the p-value of 0.073 is not highly significant, it hints at a potential trend toward higher L. monocytogenes presence in puppies or kittens.
Contrary to findings by Mireille et al. (16), the presence or absence of grains in pet food does not significantly impact L. monocytogenes prevalence in our study. Their research indicated higher L. monocytogenes contamination in grain foods.
Table 5 dissects L. monocytogenes prevalence based on various food sources, revealing notable differences. Higher prevalence is noted when beef is present, whereas lower prevalence is associated with the presence of turkey. However, the varying p-values for these categories suggest that the impact of food source on L. monocytogenes presence may be specific to certain types of meat.
The data offers insights into the geographical variation of L. monocytogenes prevalence in pet food. Although differences exist between countries, the p-value of 0.129 indicates that the observed variations may not attain statistical significance.
The packaging conditions of pet food, whether aerobic for dry food or anaerobic for canned food, can have significant implications for the survival and potential growth of Clostridium species. In dry pet food, aerobic packaging conditions inhibit the growth of Clostridium, while in canned pet food, anaerobic packaging conditions may allow Clostridium spores to persist and pose a risk if proper handling and storage practices are not followed. Our study revealed Clostridium species in 28 (24%) out of 118 samples, with bacterial loads ranging from 0 to 3,680 CFU/g. In contrast to a Polish study that reported no Clostridium contamination in pet foods (35), our findings align with Hellgren et al.’s (37) research, which reported a 30% contamination rate, particularly with C. perfringens. Similarly, Wojdat et al. (53) found Clostridium perfringens in 38% of analyzed animal feeding stuffs, influenced by factors such as feed material quality and storage conditions. Other studies focused on the presence of Clostridium difficile in dogs and their households, revealing contamination rates of 10% in dogs and 31% in households. Additionally, a commercial pet food containing turkey as the main ingredient was found to be contaminated with C. difficile (54, 55).
Recent research has discovered Clostridium species in dogs, contributing to both acute and chronic diarrhea. These bacteria, found widely in dog feces, share similarities with those causing diseases in humans (56, 57). Moreover, they are present in various food sources, including meat, poultry, pork, and beef products, particularly in those prepared with sauce (58). That is why, it is crucial to examine the health patterns of pets exposed to contaminated food. Observing whether there are identifiable connections between Clostridium contamination and issues like gastrointestinal problems in pets can provide valuable insights. Furthermore, comprehending the effects on the general well-being of animals not only informs veterinary practices but also contributes to the formulation of preventive measures.
According to Table 7, dry pet food exhibits a contamination rate of 33.30%, slightly higher than canned pet food’s rate of 20.90%. Similarly, while dogs show a slightly higher contamination rate than cats, and puppies or kittens show a slightly higher rate compared to adults, the respective p-values (pet food type: 0.182; pet type:0.215; pet age: 0.390) indicate no statistical significance. These findings suggest that neither the sample type, pet type or pet age significantly influence Clostridium contamination.
Moreover, the presence or absence of grains in pet food does not significantly impact Clostridium prevalence. Table 7 provides insights into Clostridium prevalence based on various food sources, revealing notable differences with variations in contamination rates based on specific ingredients. However, the p-values for these categories vary, suggesting that the impact of food source on Clostridium presence may be specific to certain types of meat.
The country of origin significantly impacts Clostridium contamination. Remarkably, pet foods from France show a 0% contamination rate, while those from the United States exhibit a lower contamination rate (14.30%) compared to others. The p-value of 0.029 indicates that the observed variations in contamination rates between countries may be statistically significant. The substantial influence of the pet food’s country of origin on Clostridium contamination rates indicates a complex interaction involving regulatory standards, production practices, and ingredient procurement. Countries with strict regulations and effective quality control procedures may experience lower contamination rates, while those with lax enforcement measures could encounter more difficulties in ensuring product safety. Cultural choices, ingredient compositions, and environmental conditions are additional factors likely affecting these discrepancies. Further investigation is necessary to comprehend the precise mechanisms driving these variations and devise tailored strategies to mitigate microbial risks in pet food worldwide.
In the context of Clostridium contamination in pet food, a crucial concern is the potential emergence of antibiotic resistance, particularly in strains like Clostridium difficile. Known for their adaptability through genetic changes, C. difficile has a versatile genome with mobile elements encoding antibiotic resistances (59–61). The presence of antibiotic-resistant strains in pet food poses challenges in veterinary medicine and public health. The transfer of antibiotic resistance between pets and humans is a growing concern. Studying the antibiotic susceptibility of Clostridium isolates in pet food can unveil patterns of resistance, providing insights into broader implications for both animal and human health.
Our comprehensive analysis of pet food SKUs marketed in UAE underscores the crucial importance of implementing robust quality control measures within the industry. The prevalence of microbial contamination, particularly the exceeding of acceptable limits in Total Aerobic Microbial Count (TAMC) and Total Yeast and Mold Count (TYMC) in 33 samples (28%), along with the presence of potentially harmful bacteria like Enterobacteriaceae in 8 samples (7%), L. monocytogenes in 44 samples (37%), and Clostridium in 28 samples (24%), highlights significant health risks associated with pet food consumption.
Importantly, our research addresses a critical gap, providing insights into pet food safety in the United Arab Emirates (UAE), emphasizing the necessity for tailored safety measures in this dynamic market. As the UAE plays a global role, our findings contribute to ongoing efforts for the safety and well-being of pets, guiding future research and industry practices. This research serves as a foundation for promoting global cooperation and enhancing the international standards for pet food safety.
The raw data supporting the conclusions of this article will be made available by the authors, without undue reservation.
MH: Data curation, Formal analysis, Methodology, Validation, Writing – original draft. NA: Conceptualization, Funding acquisition, Investigation, Resources, Writing – original draft. HD: Formal analysis, Methodology, Writing – original draft. MD: Data curation, Methodology, Writing – original draft. SK: Resources, Writing – original draft. MS: Project administration, Resources, Supervision, Writing – original draft. HH: Conceptualization, Formal analysis, Funding acquisition, Investigation, Project administration, Resources, Supervision, Validation, Writing – original draft.
The author(s) declare that no financial support was received for the research, authorship, and/or publication of this article.
The authors declare that the research was conducted in the absence of any commercial or financial relationships that could be construed as a potential conflict of interest.
All claims expressed in this article are solely those of the authors and do not necessarily represent those of their affiliated organizations, or those of the publisher, the editors and the reviewers. Any product that may be evaluated in this article, or claim that may be made by its manufacturer, is not guaranteed or endorsed by the publisher.
2. Applebaum, JW, Peek, CW, and Zsembik, BA. Examining U.S. pet ownership using the general social survey. Soc Sci J. (2020) 60:110–9. doi: 10.1080/03623319.2020.1728507
3. Hoy-Gerlach, J, Rauktis, M, and Newhill, C. (non-human)animal companionship: a crucial support for people during the covid-19 pandemic. Soc Register. (2020) 4:109–20. doi: 10.14746/sr.2020.4.2.08
4. Guy, RCE. Pet foods In: Encyclopedia of grain science. Australia: Elsevier Academic Press (2004). 445–50.
5. Chlebicz, A, and Slizewska, K. Campylobacteriosis, salmonellosis, yersiniosis, and listeriosis as zoonotic foodborne diseases: a review. Int J Environ Res Public Health. (2018) 15:863. doi: 10.3390/ijerph15050863
6. Laflamme, DP, Abood, SK, Fascetti, AJ, Fleeman, LM, Freeman, LM, Michel, KE, et al. Pet feeding practices of dog and cat owners in the United States and Australia. J Am Vet Med Assoc. (2008) 232:687–94. doi: 10.2460/javma.232.5.687
7. Vendramini, TH, Pedrinelli, V, Macedo, HT, Zafalon, RV, Risolia, LW, Rentas, MF, et al. Homemade versus extruded and canned commercial diets for dogs: cost comparison. PLoS One. (2020) 15:e0236672. doi: 10.1371/journal.pone.0236672
8. Dodd, S, Cave, N, Abood, S, Shoveller, A, Adolphe, J, and Verbrugghe, A. An observational study of pet feeding practices and how these have changed between 2008 and 2018. Vet Rec. (2020) 186:643–3. doi: 10.1136/vr.105828
9. Hołda, K, and Głogowski, R. Selected quality properties of lipid fraction and oxidative stability of dry dog foods under typical storage conditions. J Therm Anal Calorim. (2016) 126:91–6. doi: 10.1007/s10973-016-5543-2
10. Yu, C, Zhu, L, Xiao, J, Tang, H, Guo, G, Zeng, Q, et al. Ultrasonic extraction and determination of cyanuric acid in pet food. Food Control. (2009) 20:205–8. doi: 10.1016/j.foodcont.2008.04.004
11. Carrión, PA, and Thompson, LJ. Pet food. In: Motarjemi Y and Lelieveld H, editors. Food safety management: A practical guide for the food industry. San Diego, CA: Academic Press (2014). p. 379–96.
12. Hołda, K, Głogowski, R, Hac Szyman Czuk, E, and Wiczuk, WA. Comprehensive microbiological evaluation of dry foods for growing dogs marketed in Poland. Ann Warsaw Univ. Life Sci. (2017) 56:81–9. doi: 10.22630/AAS.2017.56.1.10
13. Behravesh, CB, Ferraro, A, Deasy, M, Dato, V, Moll, M, Sandt, C, et al. Human salmonella infections linked to contaminated dry dog and cat food, 2006-2008. Pediatrics. (2010) 126:477–83. doi: 10.1542/peds.2009-3273
14. Nemser, SM, Doran, T, Grabenstein, M, McConnell, T, McGrath, T, Pamboukian, R, et al. Investigation of L. monocytogenes, Salmonella, and Toxigenicescherichia coliin various pet foods. Foodborne Pathog Dis. (2014) 11:706–9. doi: 10.1089/fpd.2014.1748
15. Błajet-Kosicka, A, Kosicki, R, Twaruzek, M, and Grajewski, J. Determination of moulds and mycotoxins in dry dog and cat food using liquid chromatography with mass spectrometry and fluorescence detection. Food Addit Contamin B. (2014) 7:302–8. doi: 10.1080/19393210.2014.933269
16. Serhan, M, Hadid, M, Dimassi, H, Deghel, M, and Hassan, HF. Microbiological safety of commercial canned and dry pet food products in Lebanon. Front Vet Sci. (2022) 9:995184. doi: 10.3389/fvets.2022.995184
17. Center for Veterinary Medicine. Get the Facts about L. monocytogenes. U.S. Food and Drug Administration (2020). Available online at: https://www.fda.gov/animal-veterinary/animal-health-literacy/get-facts-about-L.monocytogenes (Accessed August 3, 2020).
18. Kukier, E, Goldsztejn, M, Grenda, T, Kwiatek, K, Wasyl, D, and Hoszowski, A. Microbiological quality of compound feed used in Poland. Bull Vet Inst Pulawy. (2012) 56:349–54. doi: 10.2478/v10213-012-0061-x
19. Commission regulation (EU) no 142/2011 of 25 February 2011. Annual Report. (2011). Available online at: https://eur-lex.europa.eu/LexUriServ/LexUriServ.do?uri=OJ:L:2011:054:0001:0254:EN:PDF (Accessed December 2, 2021).
20. Kepin Ska-Pacelik, J, and Biel, W. Microbiological hazards in dry dog chews and feeds. Animals. (2021) 11:631. doi: 10.3390/ani11030631
21. Queen’s Printer of Acts of Parliament. Commission Regulation (EC) No 2073/2005 of 15 November 2005 on microbiological criteria for foodstuffs. EUR- Lex (2006). Available online at: https://www.legislation.gov.uk/eur/2005/2073 (Accessed August 15, 2020).
22. GMP. Regulations on product standards in the animal feed sector. US, FDA: Good Manufacturing Practices (2005).
23. ISO. Method 6887–1, microbiology of the food chain — Preparation of test samples, initial suspension and decimal dilutions for microbiological examination — Part 1: General rules for the preparation of the initial suspension and decimal dilutions. Switzerland: International Organization for Standardization (2017).
24. ISO. Method 7218, Microbiology of food and animal feeding stufs — General requirements and guidance for microbiological examinations. Switzerland: Amendment 1 International Organization for Standardization (2007).
25. FEDIAF. Guide to good practice for the manufacture of safe pet foods. Bruxelles: The European Pet Food Industry (2018).
26. Kim, D, Cao, Y, Mariappan, D, Bono, MS, Hart, AJ, and Marelli, B. A microneedle technology for sampling and sensing bacteria in the food supply chain. Adv Funct Mater. (2020) 31:2005370. doi: 10.1002/adfm.202005370
27. Nychas, GJE, Skandamis, PN, Tassou, CC, and Koutsoumanis, KP. Meat spoilage during distribution. Meat Sci. (2008) 78:77–89. doi: 10.1016/j.meatsci.2007.06.020
28. Kazimierska, K, Biel, W, Witkowicz, R, Karakulska, J, and Stachurska, X. Evaluation of nutritional value and microbiological safety in commercial dog food. Vet Res Commun. (2021) 45:111–28. doi: 10.1007/s11259-021-09791-6
29. Nyenje, ME, Odjadjare, CE, Tanih, NF, Green, E, and Ndip, RN. Foodborne pathogens recovered from ready-to-eat foods from roadside cafeterias and retail outlets in alice, eastern cape province, South Africa: public health implications. Int J Environ Res Public Health. (2012) 9:2608–19. doi: 10.3390/ijerph9082608
30. Banwart, GJ. Factors that affect microbial growth in food. Basic Food Microbiol. (1989):101–63. doi: 10.1007/978-1-4684-6453-5_4
31. Nedwell, DB. Effect of low temperature on microbial growth: lowered affinity for substrates limits growth at low temperature. FEMS Microbiol Ecol. (1999) 30:101–11. doi: 10.1111/j.1574-6941.1999.tb00639.x
32. Eirmann, L, Cowell, C, and Thompson, L. Pet food safety: the roles of government, manufacturers, and veterinarians. Compend Contin Educ Vet. (2012) 34:E4.
33. Meghwal, M, Heddurshetti, U, and Biradar, R. Good manufacturing practices for food processing industries: principles and practical applications. Food Technol. (2017) 11:3–28. doi: 10.1201/9781315365657-1
34. Paulsen, P, Bauer, S, Bauer, F, and Dicakova, Z. Contents of polyamines and biogenic amines in canned pet (dogs and cats) food on the Austrian market. Food Secur. (2021) 10:2365. doi: 10.3390/foods10102365
35. Kazimierska, K, Biel, W, Witkowicz, R, and Kochel-Karakulska, J. The nutritional value adequacy and microbiological quality of canned foods for puppies and adult dogs. Appl Sci. (2024) 14:760. doi: 10.3390/app14020760
36. Wojdat, E, Kwiatek, K, and Kozak, M. Microbiological quality of animal feedingstuffs in Poland. Bull Vet Inst Pulawy. (2005) 49:315–8.
37. Hellgren, J, Hästö, LS, Wikström, C, Fernström, L, and Hansson, I. Occurrence of Salmonella, Campylobacter, Clostridium and enterobacteriaceae in RAW meat- based diets for dogs. Vet Rec. (2019) 184:442–2. doi: 10.1136/vr.105199
38. Nüesch-Inderbinen, M, Treier, A, Zurfluh, K, and Stephan, R. Raw meat- based diets for companion animals: a potential source of transmission of pathogenic and antimicrobial-resistant Enterobacteriaceae. R Soc Open Sci. (2019) 6:191170. doi: 10.1098/rsos.191170
39. Baede, VO, Broens, EM, Spaninks, MP, Timmerman, AJ, Graveland, H, Wagenaar, JA, et al. Raw pet food as a risk factor for shedding of extended- spectrum beta-lactamase-producing Enterobacteriaceae in household cats. PLoS One. (2017) 12:e0187239. doi: 10.1371/journal.pone.0187239
40. Carvalho, AC, Barbosa, AV, Arais, LR, Ribeiro, PF, Carneiro, VC, and Cerqueira, AMF. Resistance patterns, ESBL genes, and genetic relatedness of Escherichia coli from dogs and owners. Braz J Microbiol. (2016) 47:150–8. doi: 10.1016/j.bjm.2015.11.005
41. Takahashi, H, Saito, R, Miya, S, Tanaka, Y, Miyamura, N, Kuda, T, et al. Development of quantitative real-time PCR for detection and enumeration of Enterobacteriaceae. Int J Food Microbiol. (2017) 246:92–7. doi: 10.1016/j.ijfoodmicro.2016.12.015
42. Qekwana, DN, Phophi, L, Naidoo, V, Oguttu, JW, and Odoi, A. Antimicrobial resistance among Escherichia Coli isolates from dogs presented with urinary tract infections at a veterinary teaching hospital in South Africa. BMC Vet Res. (2018) 14:228. doi: 10.1186/s12917-018-1552-7
43. Finn, S, Condell, O, McClure, P, Amézquita, A, and Fanning, S. Mechanisms of survival, responses and sources of Salmonella in low-moisture environments. Front Microbiol. (2013) 4:331. doi: 10.3389/fmicb.2013.00331
44. Cordier, J-L. Production of powdered infant formulae and microbiological controls In: JM Farber and SJ Forsythe, editors. Emerging issues in food safety: Enterobacter sakazakii. Washington, DC: ASM Press (2008). 145–85.
45. Witaszak, N, Waskiewicz, A, Bocianowski, J, and Stepien, U. Contamination of pet food with mycobiota and fusarium mycotoxins—focus on dogs and cats. Toxins. (2020) 12:130. doi: 10.3390/toxins12020130
46. Bueno, D, Silva, J, and Oliver, G. Mycoflora in commercial pet foods. J Food Prot. (2001) 64:741–3. doi: 10.4315/0362-028X-64.5.741
47. D’Mello, J, and Macdonald, A. Mycotoxins. Anim Feed Sci Technol. (1997) 69:155–66. doi: 10.1016/S0377-8401(97)81630-6
48. D’aoust, JY. Salmonella in commercial pet foods. Can Vet J. (1978) 19:99–100. doi: 10.3828/extr.1978.19.2.99
49. Wojdat, E, Kwiatic, K, and Zasadny, R. Microbiological quality of petfood in Poland. Pol J Vet Sci. (2004) 7:207–9.
50. Yukawa, S, Uchida, I, Tamura, Y, Ohshima, S, and Hasegawa, T. Characterisation of antibiotic resistance of Salmonella isolated from dog treats in Japan. Epidemiol Infect. (2019) 147:e102. doi: 10.1017/S0950268819000153
51. Imanishi, M, Rotstein, DS, Reimschuessel, R, Schwensohn, CA, Woody, DH, Davis, SW, et al. Outbreak of Salmonella enterica serotype Infantis infection in humans linked to dry dog food in the United States and Canada, 2012. J Am Vet Med Assoc. (2014) 1:545–53. doi: 10.2460/javma.244.5.545
52. Bourgeois, H, Elliott, D, Marniquet, P, Soulard, Y, Royal Canin, AFRA, et al. Dietary behavior of dogs and cats. Bull Acad Vét France. (2006) 159:301–8. doi: 10.4267/2042/47848
53. Wojdat, E, Kwiatek, K, and Kozak, M. Occurrence and characterization of some Clostridium species isolated from animal Feedingstuffs. Bull Vet Inst Pulawy. (2006) 50:63–7.
54. Weese, JS, Rousseau, J, and Arroyo, L. Bacteriological evaluation of commercial canine and feline raw diets. Can Vet J. (2005) 46:513–6.
55. Weese, JS, Finley, R, Reid-Smith, RR, Janecko, N, and Rousseau, J. Evaluation of clostridium difficile in dogs and the household environment. Epidemiol Infect. (2009) 138:1100–4. doi: 10.1017/s0950268809991312
56. Stone, NE, Sidak-Loftis, LC, Sahl, JW, Vazquez, AJ, Wiggins, KB, Gillece, JD, et al. More than 50% of clostridium difficile isolates from pet dogs in Flagstaff, USA, carry toxigenic genotypes. PLoS One. (2016) 11:e0164504. doi: 10.1371/journal.pone.0164504
57. Diniz, AN, Coura, FM, Rupnik, M, Adams, V, Stent, TL, Rood, JI, et al. The incidence of Clostridioides difficile and Clostridium perfringens netF -positive strains in diarrheic dogs. Anaerobe. (2018) 49:58–62. doi: 10.1016/j.anaerobe.2017.12.003
58. Batt, CA, Robinson, RK, and Popoff, MR. CLOSTRIDIUM | detection of enterotoxin of Clostridium perfringens In: Encyclopedia of food microbiology. second ed. Cambridge, MA, USA: Academic Press (2014). 474–80.
59. Sebaihia, M, Wren, B, Mullany, P, Fairweather, N, Minton, N, Stabler, R, et al. The multidrug-resistant human pathogen Clostridium difficile has a highly mobile, mosaic genome. Nat Genet. (2006) 38:779–86. doi: 10.1038/ng1830
60. He, M, Sebaihia, M, Lawley, T, Stabler, R, Dawson, L, Martin, M, et al. Evolutionary dynamics of Clostridium difficile over short and long time scales. Proc Natl Acad Sci USA. (2010) 107:7527–32. doi: 10.1073/pnas.0914322107
Keywords: pet food, bacteria, microbiological quality, safety, UAE
Citation: Hadid M, Alwan N, Dimassi H, Deghel M, El Khatib S, Serhan M and Hassan HF (2024) Microbial assessment of commercial pet foods marketed in the United Arab Emirates. Front. Vet. Sci. 11:1389981. doi: 10.3389/fvets.2024.1389981
Received: 22 February 2024; Accepted: 13 May 2024;
Published: 24 May 2024.
Edited by:
Vincenzina Fusco, National Research Council (CNR), ItalyReviewed by:
Piera Anna Martino, University of Milan, ItalyCopyright © 2024 Hadid, Alwan, Dimassi, Deghel, El Khatib, Serhan and Hassan. This is an open-access article distributed under the terms of the Creative Commons Attribution License (CC BY). The use, distribution or reproduction in other forums is permitted, provided the original author(s) and the copyright owner(s) are credited and that the original publication in this journal is cited, in accordance with accepted academic practice. No use, distribution or reproduction is permitted which does not comply with these terms.
*Correspondence: Hussein F. Hassan, aHVzc2Vpbi5oYXNzYW5AbGF1LmVkdS5sYg==
†These authors have contributed equally to this work
Disclaimer: All claims expressed in this article are solely those of the authors and do not necessarily represent those of their affiliated organizations, or those of the publisher, the editors and the reviewers. Any product that may be evaluated in this article or claim that may be made by its manufacturer is not guaranteed or endorsed by the publisher.
Research integrity at Frontiers
Learn more about the work of our research integrity team to safeguard the quality of each article we publish.