- 1Department of Veterinary Sciences, University of Turin, Grugliasco, Italy
- 2Department of Agricultural, Forestry and Food Science, University of Turin, Grugliasco, Italy
- 3Department of Veterinary Sciences, University of Bologna, Bologna, Italy
Introduction: Diet is one of the main factors influencing the intestinal microbiota in horses, yet a systematic characterization of the microbiota along the length of the digestive tract in clinically healthy horses, homogenous for age and breed and receiving a specific diet is lacking.
Methods: The study used 16S rRNA amplicon sequencing to characterize the microbiota of the intestinal tracts of 19 healthy Bardigiano horses of 14.3 ± 0.7 months of age fed one of two diets. Nine horses received a high-starch diet (HS), and ten horses received a high-fiber diet (HF). After 129 days, the horses were slaughtered, and samples were collected from the different intestinal tract compartments.
Results and discussion: The microbiota alpha diversity indices were lower in the caecum, pelvic flexure and right dorsal colon of the horses fed the HS diet (False Discovery Rate, FDR < 0.05). The values of beta diversity indicated significant compositional differences between the studied intestinal tract compartments according to the diet received (FDR < 0.05). At the lower taxonomic level (genus or family), the HS diet was associated with a higher relative frequency of Enterobacteriaceae within the small intestine (jejunum and duodenum) (FDR < 0.05). Within the hindgut (caecum and sternal flexure), the HS diet was associated with lower relative frequencies (i.e., a smaller core community) of bacteria belonging to Fibrobacteraceae and Prevotellaceae (FDR < 0.05). Moreover, horses fed the HS diet displayed a higher relative abundance of Streptococcus in the caecum (FDR < 0.05) and Fusobacterium in the sternal flexure (FDR < 0.05), both of which are pathogenic bacteria responsible for inflammation diseases. Samples collected from the pelvic flexure and rectum of horses fed the HS diet showed significantly higher relative frequencies of Succinivibrionaceae (FDR < 0.05) – amylolytic bacteria associated with acidosis. The relative frequencies of the Lachnospiraceae and Ruminococcaceae were lower in the feces collected from the rectum of horses receiving the HS diet vs. HF diet, indicating smaller core communities of these bacteria (FDR < 0.05). Fibrous diets should be promoted to prevent dysbiosis of the microbiota associated with high-starch diet.
1 Introduction
A better understanding of the etiology of digestive disorders is crucial for safeguarding horse welfare and digestive health (1). Several factors related both to intrinsic to the individual and to management conditions can predispose the horse to the onset of digestive disorders (2). One of the main risk factors of digestive disorders is being fed a diet high in starch and low in fiber (3). In fact, the horse’s capacity to digest starch is highly limited due to their low production of pancreatic α-amylase (4, 5). Accordingly, several authors agree that starch consumption should be limited to not more than 1 gram of starch/kg bodyweight (BW)/meal (6–9). Despite this recommendation, horses are commonly fed diets characterized by much higher amounts of starch (10–12). Such a feeding practice results in an impairment of the gut health, causing hindgut acidosis, dysbiosis and colic (13). Systemic consequences are also possible, such as laminitis, weight loss and poor performance (1, 14, 15). The presence of indigested starch in the hindgut can trigger microbial population changes during the fermentation processes (5, 16), yet little research effort has been directed at characterizing the horse microbiota in the different compartments of the intestinal tract according to diet with respect to other animal species (17–19). De Fombelle et al. (20) applied a culture-based methodology to samples obtained from the jejunum, ileum, caecum, right ventral colon and left dorsal colon; this approach thus limited the output to cultivable species only (21). Dougal et al. (22) investigated microbial population communities using a PCR-based method on samples from the caecum, right dorsal colon and rectum of eight equines euthanized for reasons not related to metabolic or intestinal disturbances. However, neither culture-dependent nor PCR-based methodologies permit the full richness and diversity of the bacterial populations resident in the gut to be characterized (23). To the best of our knowledge, Dougal et al. (24) used an amplicon sequencing approach to identify the core bacterial community across seven different regions of the large intestine of ten equines. Moreover, the study carried out by Costa et al. (18) applied a sequencing approach to evaluate the microbiota of the horse in the different compartments of the intestinal tract. However, this latter study only involved eleven horses belonging to different breeds, characterized by a wide age range (2 to 30 years) and which were euthanized for pathologies (unrelated to gastrointestinal diseases). The goal of the present study was to deepen our understanding of the microbial differences in different intestinal tract compartments in healthy horses, homogenous for breed and age, according to the diet being fed, namely a high-fiber diet (HF) vs. a high-starch diet (HS). We hypothesized that that differences in the gut microbiota in the distinct intestinal tract compartments would be observed between the two dietary groups. This study aims to further our understanding of the microbial changes associated with a HS diet in the intestinal environment.
2 Materials and methods
The present study forms part of a larger research project investigating the effects of a high-fiber diet (HF) vs. a high-starch diet (HS). The same horses were also investigated for other parameters of gut health as gut histomorphometry (25) and fermentation end-products (16); behavior (26) and growing performances (15).
The study followed the guidelines of the current European Directive (2010/63/EU) on the care and protection of animals, and it was approved by the Ethics Committee of the Department of Veterinary Sciences of the University of Turin (Italy) (Prot. N. 2,202/2019).
2.1 Animals and feeding management
The study was performed in Piedmont, Northwest Italy between June and October 2019. Nineteen healthy Bardigiano horses of 14.3 ± 0.7 months of age (mean ± standard deviation, SD) were enrolled in the study. Upon their arrival at the farm, all horses were dewormed using an oral gel preparation (1.29 g/100 kg BW, Eqvalan Duo, Merial Animal Health). The horses were first acclimatized to the farm environment in an outdoor paddock area for two weeks before being randomly assigned to two indoor group pens for the following 129 days until slaughter. The group pens were situated inside a barn with two open sides and no access to any outdoor paddock area. Pens were enclosed by horizontal metal rail bars, which also delimited the pens at the feed bunk. The horses fed the HF diet were 7 fillies and 3 colts with a mean ± standard deviation (SD) initial bodyweight (BW) of 221.10 ± 5.00 kg; the horses fed the HS diet were 5 fillies and 4 colts with a mean ± SD initial BW of 217.56 ± 9.28 kg. The horses belonging to the HF and HS groups received the same first-cut meadow hay, but different complementary feeds. Horses were individually fed the complementary feeds that were gradually increased to reach the final amount during the last 72 days of the fattening period (15). Briefly, for the HS, the amount (as fed) of the starch-rich pelleted feed was gradually increased as follow: 3 kg/animal/day for the first 13 days, followed by 4.5 kg/animal/day for the subsequent 6 days, 5 kg/animal day for further 36 days, and 8 kg/animal/day during the last 72 days of the fattening period. Those quantities were fed according to the usual feeding practice adopted by the farmer. For the HF, the amount (as fed) of the fiber-rich pelleted feed was gradually increased as follow: 1 kg/animal/day for 7 days, 2 kg/animal/day for 9 days, 2.5 kg/animal/day for 25 days, 3 kg/animal/day for 9 days, and finally 3.5 kg/animal/day until the end of the fattening period (72 days). The HF diet was planned by a veterinary nutritionist according to the nutritional requirements of the French “Institute National de la Research Agronomique” (INRA) (27).
During the last 72 days of the fattening period, each horse belonging to the HS received 8 kg/horse/day of the starch-rich pelleted feed containing 5.70 g (as fed) of starch per kg BW/meal, whereas each horse belonging to the HF group received 3.5 kg/horse/day of the fiber-rich pelleted feed containing 0.97 g (as fed) of starch per kg BW/meal. The complementary pelleted feeds were individually supplied to the horses twice a day (7 am and 6 pm). Hay was provided at the same time, and the hay consumption was 8 kg/horse/day for HF and 6 kg/horse/day for HS. The ingredients and the chemical composition of hay and complementary feeds are shown in Table 1. The daily nutritional composition of the diets (hay plus feed) are shown in Table 2.
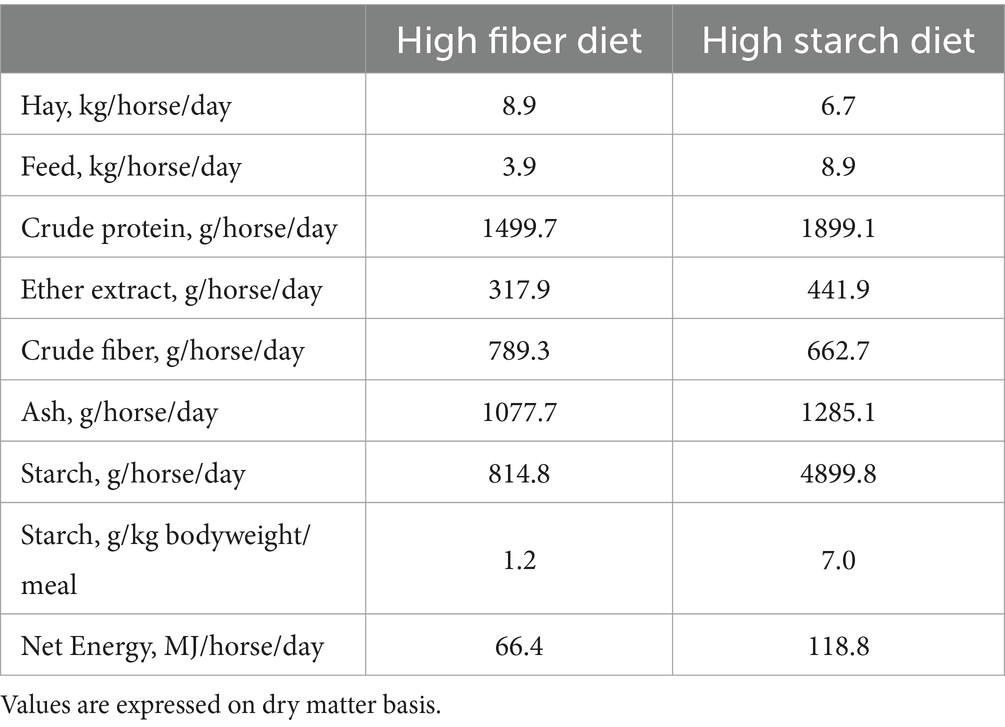
Table 2. Nutritional composition of the overall daily diets (hay plus feed) during the fattening period (72 days).
2.2 Sample collection
Animals were slaughtered 129 days after their arrival at the farm, at the end of the fattening period. The slaughtering procedures were performed according to European Union regulations (EU 2009/853 and EU 627/2019) under the supervision of official veterinarians.
After slaughtering, the following intestinal tract compartments (described in Figure 1) were immediately identified and clamped before being opened for sample collection:
• duodenum
• jejunum
• ileum
• caecum
• sternal flexure
• pelvic flexure
• right dorsal colon
• rectum
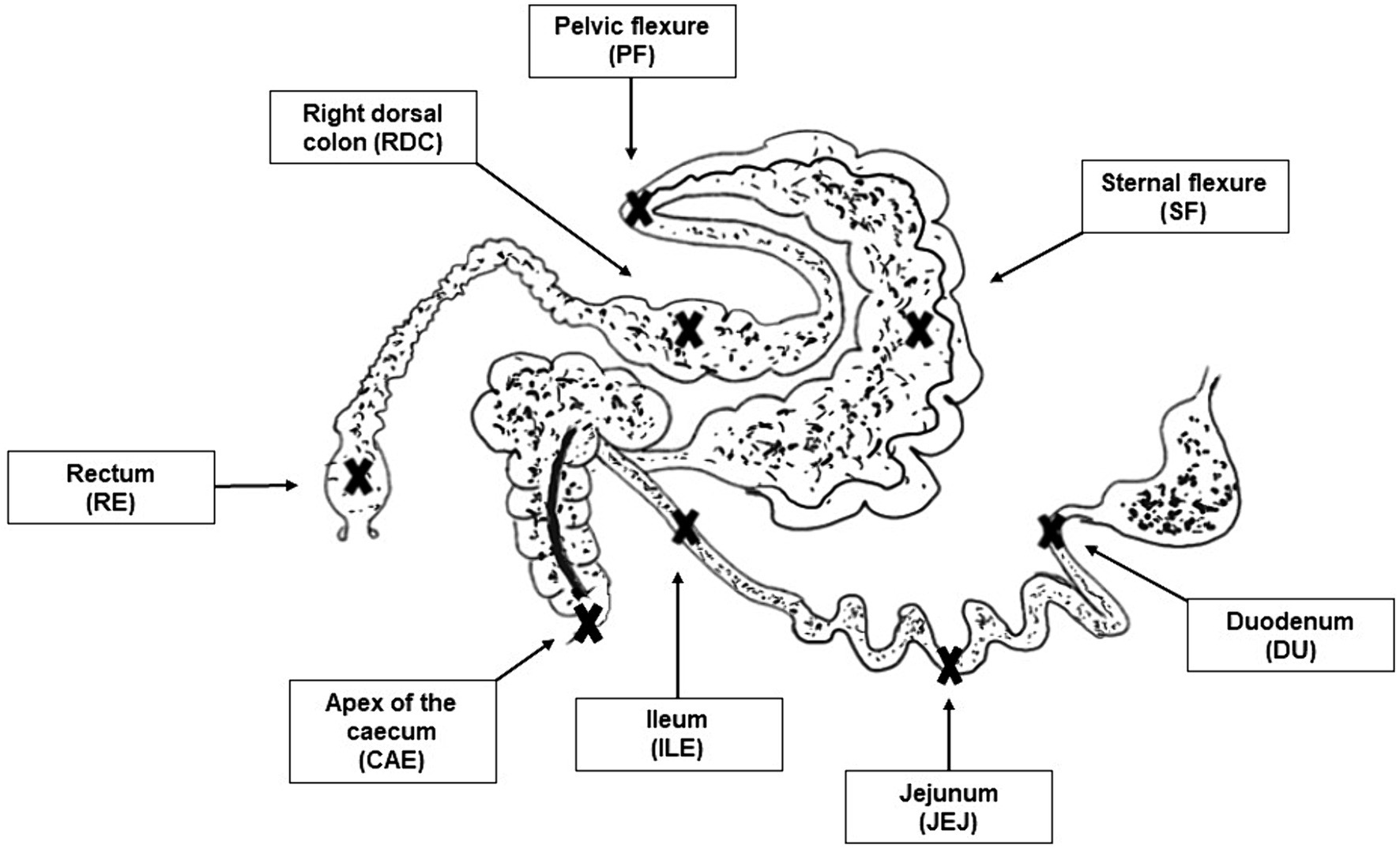
Figure 1. Picture of the sampling sites. Crosses indicate the sampling sites for the selected intestinal tract compartments: duodenum (DU), jejunum (JEJ), ileum (ILE), caecum (CAE), sternal flexure (SF), pelvic flexure (PF), right dorsal colon (RDC), rectum (RE).
2.3 DNA extraction and metataxonomic analysis
A total of 152 samples (8 intestinal tract compartments/horse for 19 horses) were subjected to DNA extraction and sequencing. DNA was isolated using the QIAamp Power Fecal Pro DNA Kit (QIAGEN, Milan, Italy) according to the manufacturer’s instructions. We added one microliter of RNase (50 mg/mL, Illumina Inc., San Diego, CA) to the DNA samples and incubated them for 1 h at 37°C to digest any RNA present. The DNA was normalized to 5 ng/μL and used as the template for the amplification of the V3-V4 region of the 16S rRNA gene (16S Amplicon PCR Forward Primer = 5’TCGTCGGCAGCGTCAGATGTGTATAAGAGACAGCCTACGGGNGGCWGCAG; 16S Amplicon PCR Reverse Primer = 5’GTCTCGTGGGCTCGGAGATGTGTATAAGAGACAGGACTACHVGGGTATCTAATCC) (28). The PCR products were purified and tagged with the Nextera index according to the 16S Metagenomic Sequencing Library Preparation from Illumina (Illumina, San Diego, USA). We sequenced the libraries on the Illumina MiSeq platform (Illumina Italy s.r.l., Milan, Italy) with V3 chemistry and generated 250 bp paired-end reads according to the manufacturer’s instructions.
2.4 Bioinformatics and statistical analysis
Each horse within each diet group was considered as experimental unit. After sequencing, we analyzed the raw reads using Quantitative Insights into Microbial Ecology (QIIME) 2 software (29). Following denoizing, performed by DADA2 (30), amplicon sequence variants (ASVs) were used for taxonomic assignment using the QIIME feature-classifier classify-consensus-vsearch (31) plugin against the greengenes database (v.13). We then used the QIIME-diversity script (29) to calculate the alpha and beta diversity parameters, which were then subjected to pairwise comparisons to assess for differences in the microbiota composition in the different intestinal tract compartments according to the diet used (HF vs. HS). In particular, we analyzed the indices of alpha diversity (Chao1, Shannon and the observed species richness) using Kruskal–Wallis tests, and we compared the beta diversities by pairwise PERMANOVA analysis based on the Bray-Curtis distance matrix using the QIIME2 script (29).
We imported the ASVs (Amplicon sequence variants), at the lowest taxonomic level (family or genus), into R to build a heatmap by applying the function “made4” (32). Moreover, we used pairwise Kruskal-Wallis tests to assess for differences in microbial taxa abundance according to diet (HF vs. HS). p-values were adjusted for multiple testing using the false discovery rate (FDR) method (p-value adjustment methods Benjamini-Hochberg). We considered a FDR < 0.05 as significant. The Picrust2 (Phylogenetic Investigation of Communities by Reconstruction of Unobserved States) software package (33) was then used to predict the potential functions of the microbiota. KEGG orthologs were collapsed at level 3 of hierarchy, and the table imported into the GAGE Bioconductor package (34) to identify the potential pathways either under-or overrepresented in the samples from the HF vs. HS dietary groups.
3 Results
At the end of the fattening period, we revealed no significant difference in the mean ± SD slaughter BW of horses fed the HF vs. the HS diet: 344.40 ± 2.91 kg vs. 347.67 ± 6.71 kg, respectively (15). No clinical signs of colic or gastric ulcerations were recorded during the study period but the horses belonging to the HS group produced unformed feces. Moreover, histopathological evaluation of the different intestinal tract compartments showed higher level of inflammation in the jejunum and pelvic flexure of horse fed the HS diet compared with those fed the HF diet (25).
3.1 Alpha and beta diversity
After sequencing and denoizing, a total of 565,643 raw reads were used for downstream analysis, corresponding to a sample coverage exceeding 99%. First, we compared the measures of alpha and beta diversity to investigate the effect of diet on microbiota diversity. Alpha diversity was measured by means of the Chao1 and Shannon indices and the observed species richness. As shown in Table 3, the Chao1 index was higher in the caecum of horses fed HF compared with HS (p = 0.01). The Shannon index was lower in the ileum of horses fed HF compared with HS (p = 0.04), and it was higher in the caecum (p = 0.04), pelvic flexure (p = 0.01), and right dorsal colon (p = 0.01) of horses fed the HF diet compared with the HS diet. Moreover, the observed species richness was significantly higher in the right dorsal colon of the horses belonging to the HF group compared with those belonging to the HS group (p < 0.01).
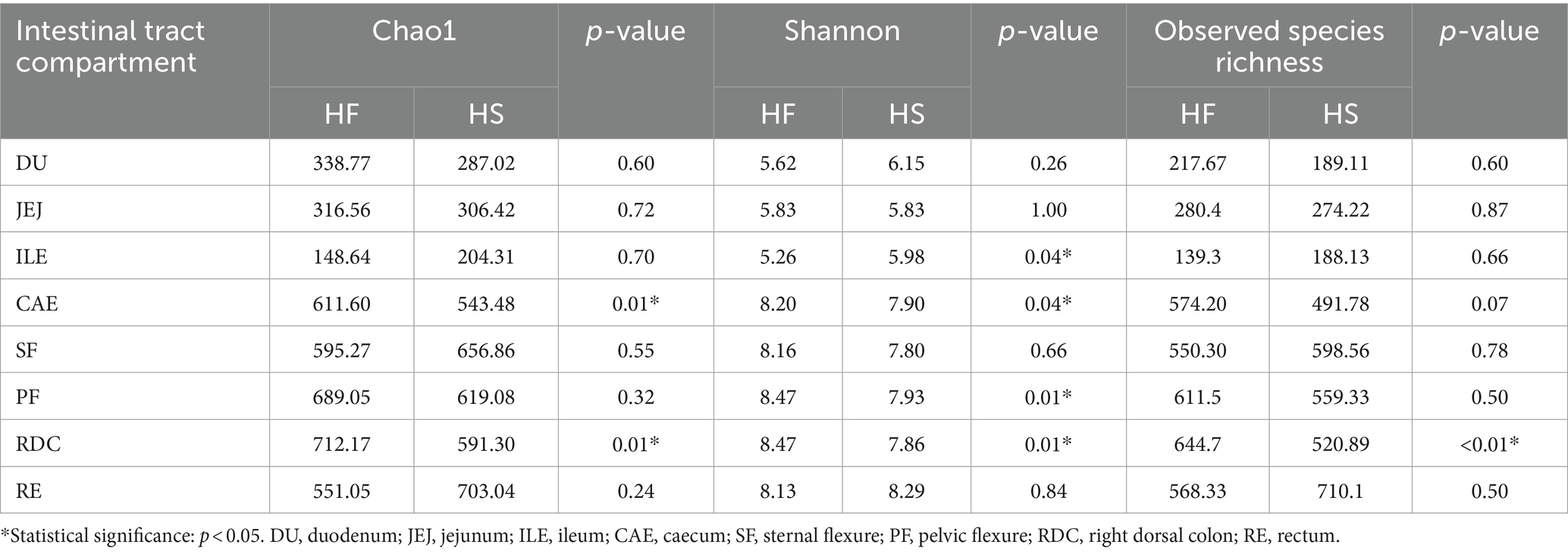
Table 3. Alpha diversity measures of microbiota composition in the selected intestinal tract compartments between horses fed the high-starch diet (HS) and the high-fiber diet (HF).
To assess the effect of the two HF and HS diets on the microbiota composition at the different sampling locations, taking all the detected ASVs into account, we performed pairwise PERMANOVA analysis, based on the Bray Curtis distance matrix, to generate a measure of beta diversity. With the exception of the duodenum, the microbiota of each tract sampled differed significantly according to the feeding practice adopted (HF vs. HS, p < 0.05, Figure 2). These differences were demonstrated by the Principal Coordinate analysis based on the Bray Curtis distance matrix (PCoA, Figure 2). Moreover, the samples formed two distinct clusters, differentiating between the upper (DU, JEJ, ILE) and lower (CAE, SF, PF, RDC, RE) digestive tract of the horse.
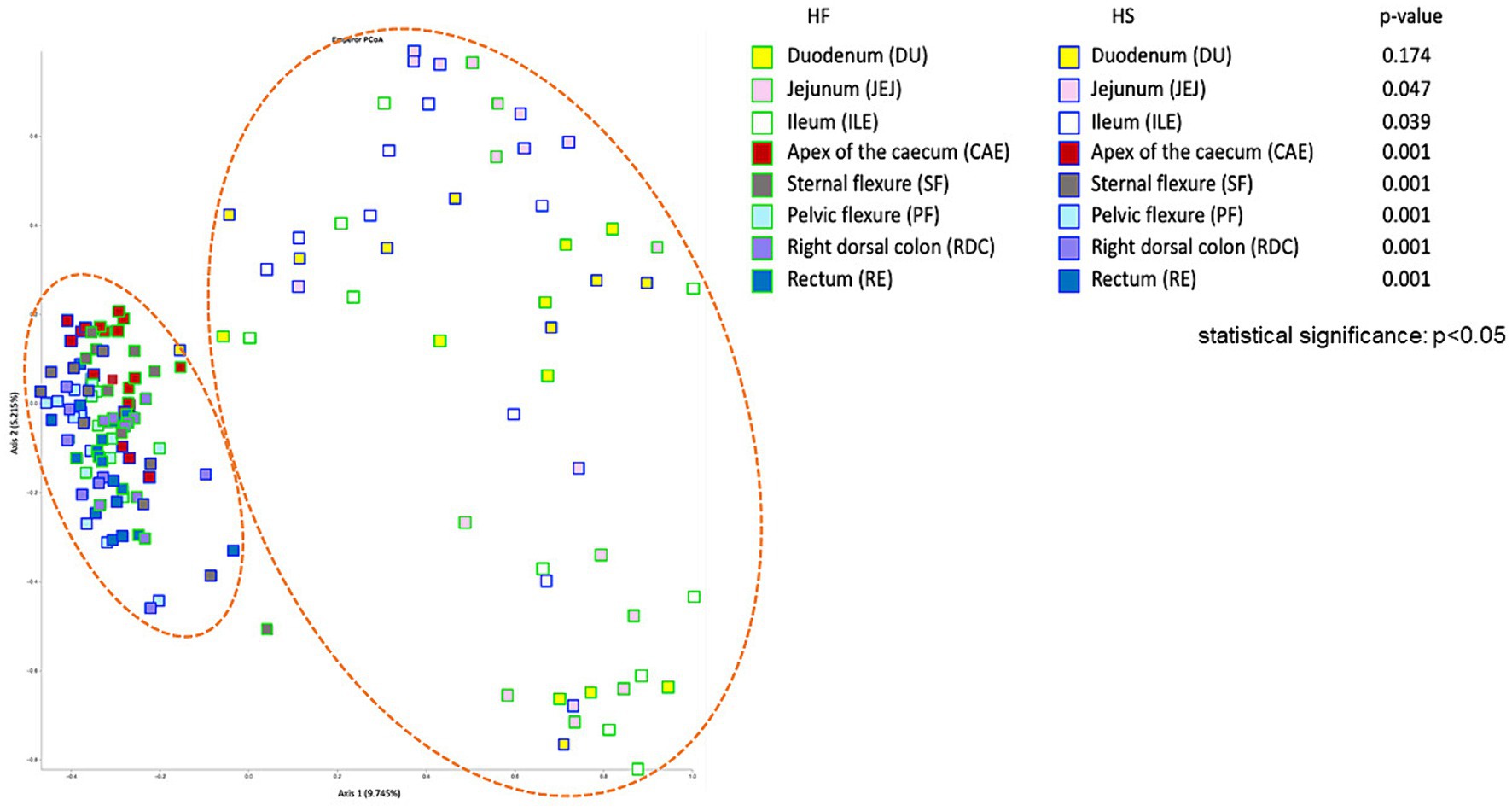
Figure 2. Principal Coordinate analysis (PCoA), based on the Bray Curtis distance, to study the effect of diet (high fiber, HF vs. high starch, HS) on the bacterial microbiota at different intestinal tract compartments of the horse.
3.2 Characterization of the bacterial microbiota at the phylum level
The distribution and relative frequencies (%) of the phyla obtained by 16S rRNA gene sequencing are summarized in Figure 3. Firmicutes represented the dominant phylum in both HF and HS, outnumbering the Bacteroidetes, Proteobacteria and Verrucomicrobia phyla.
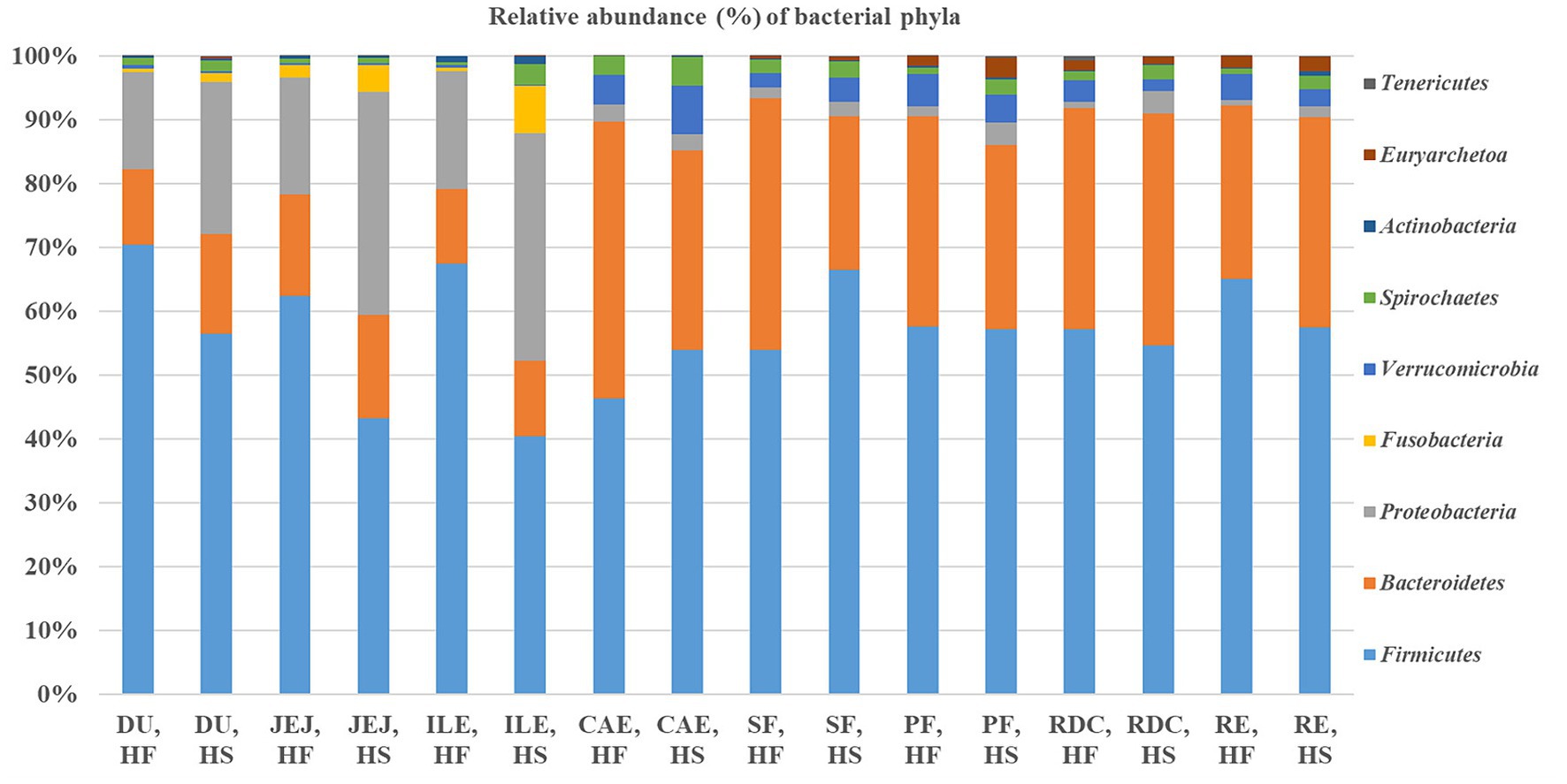
Figure 3. Relative frequency (%) of the bacterial phyla in the selected intestinal tract compartments (DU, duodenum; JEJ, jejunum; ILE, ileum; CAE, caecum; SF, sternal flexure; PF, pelvic flexure; RDC, right dorsal colon; RE, rectum) of the horses fed the high fiber diet (HF) and of the horses fed the high starch diet (HS).
Considering the microbiota according to intestinal site, we found differences in the relative abundance frequencies of the different phyla. In particular, within the small intestine, the abundance of Firmicutes was greater in the ileum of HF compared to HS (67.4% HF vs. 40.4% HS; FDR < 0.05), whereas Proteobacteria were more prolific in the jejunum (18.3% HF vs. 35% HS) and ileum (18.4% HF vs. 35.6% HS) of HS compared with HF (FDR < 0.05). Within the hindgut, the frequency of Firmicutes in the sternal flexure of HS was higher compared with HF (53.9% HF vs. 66.5% HS; FDR < 0.05); whereas the frequency of the phylum Bacteroidetes was greater in horses receiving the HF diet in both the caecum (43.4% HF vs. 31.2% HS, FDR < 0.05) and the sternal flexure (39.4% HF vs. 24.1% HS, FDR < 0.05). Moreover, the relative frequency of Spirochaetes was higher in the rectum of horses fed the HS diet compared to the horses fed the HF diet (0.9% HF vs. 2.2% HS; FDR < 0.05).
3.3 Characterization of the bacterial microbiota at the genus level
At lowest taxonomic level, cluster analysis of the most frequent ASVs confirmed the presence of distinct clusters (Figure 4). One cluster (A) comprised most of the samples collected from the hindgut (caecum, sternal flexure, pelvic flexure, right dorsal colon and rectum). Moreover, within the cluster A, a clear distinction was evident between the caecum and the sternal flexure, and between the pelvic flexure, right dorsal colon and rectum. The second cluster (B) comprised most of the samples collected from the duodenum, jejunum, and ileum.
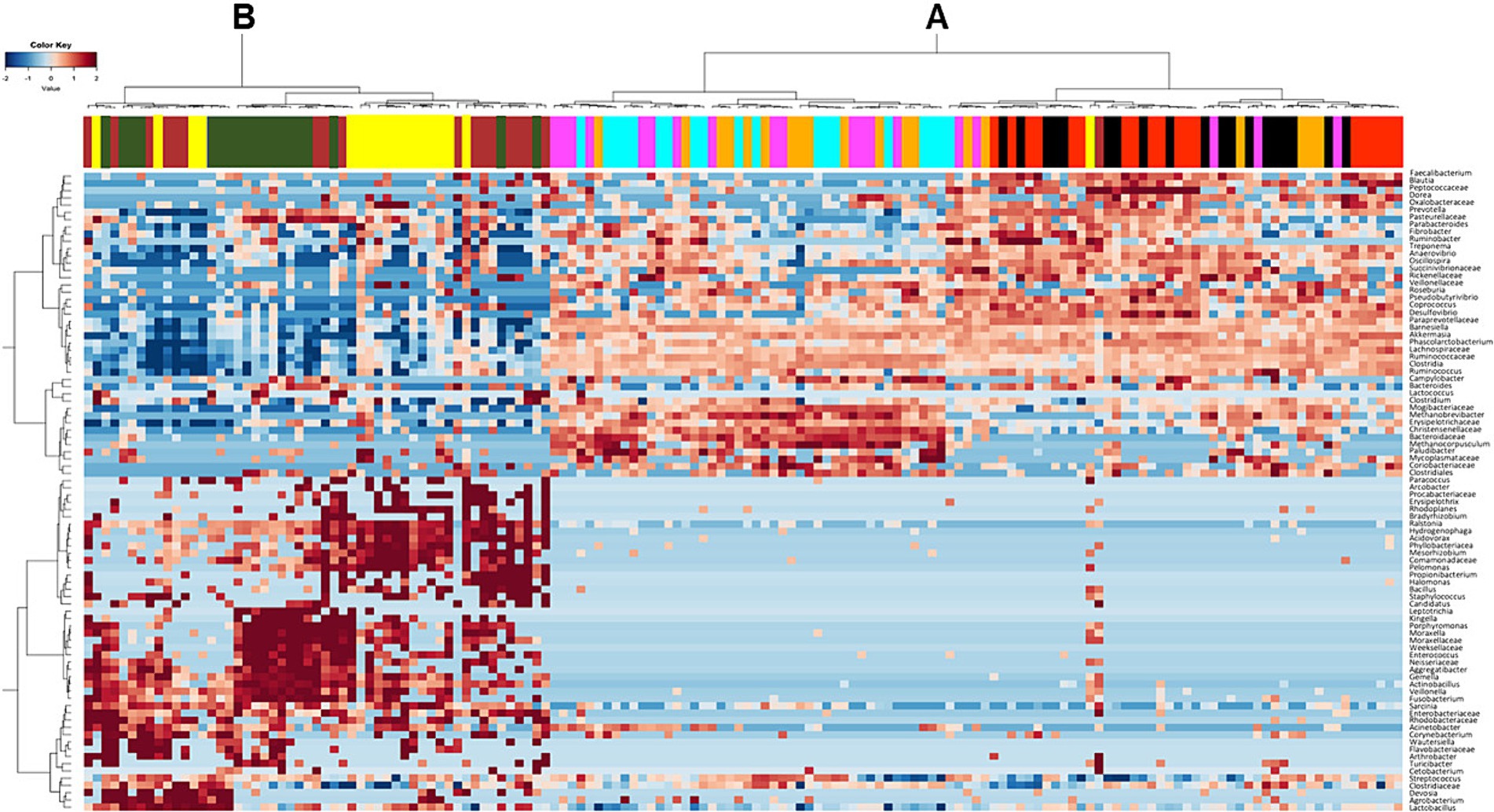
Figure 4. Heatmap showing the distribution of the Amplicon Sequence Variants (ASVs) detected in horses fed the high fiber diet (HF) and the high starch diet (HS) across the different intestinal tract compartments (duodenum = yellow, jejunum = dark green, ileum = brown, apex of the caecum = red, sternal flexure = black, pelvic flexure = pink, right dorsal colon = cyan, rectum = orange). The frequency of the ASVs is represented by color intensity, from dark blue (lowest frequency) through to dark red (highest frequency).
The distribution of the main genera found along the different compartments of the intestinal tract according to the diet received is shown in Figure 5. The comparison of the relative frequency of ASVs in the duodenum between the two groups (HF vs. HS) showed greater levels of both Clostridium (0.1% HF vs. 2.9% HS; FDR < 0.05) and Moraxella (0.2% HF vs. 1.2% HS; FDR < 0.05) in horses fed HS.
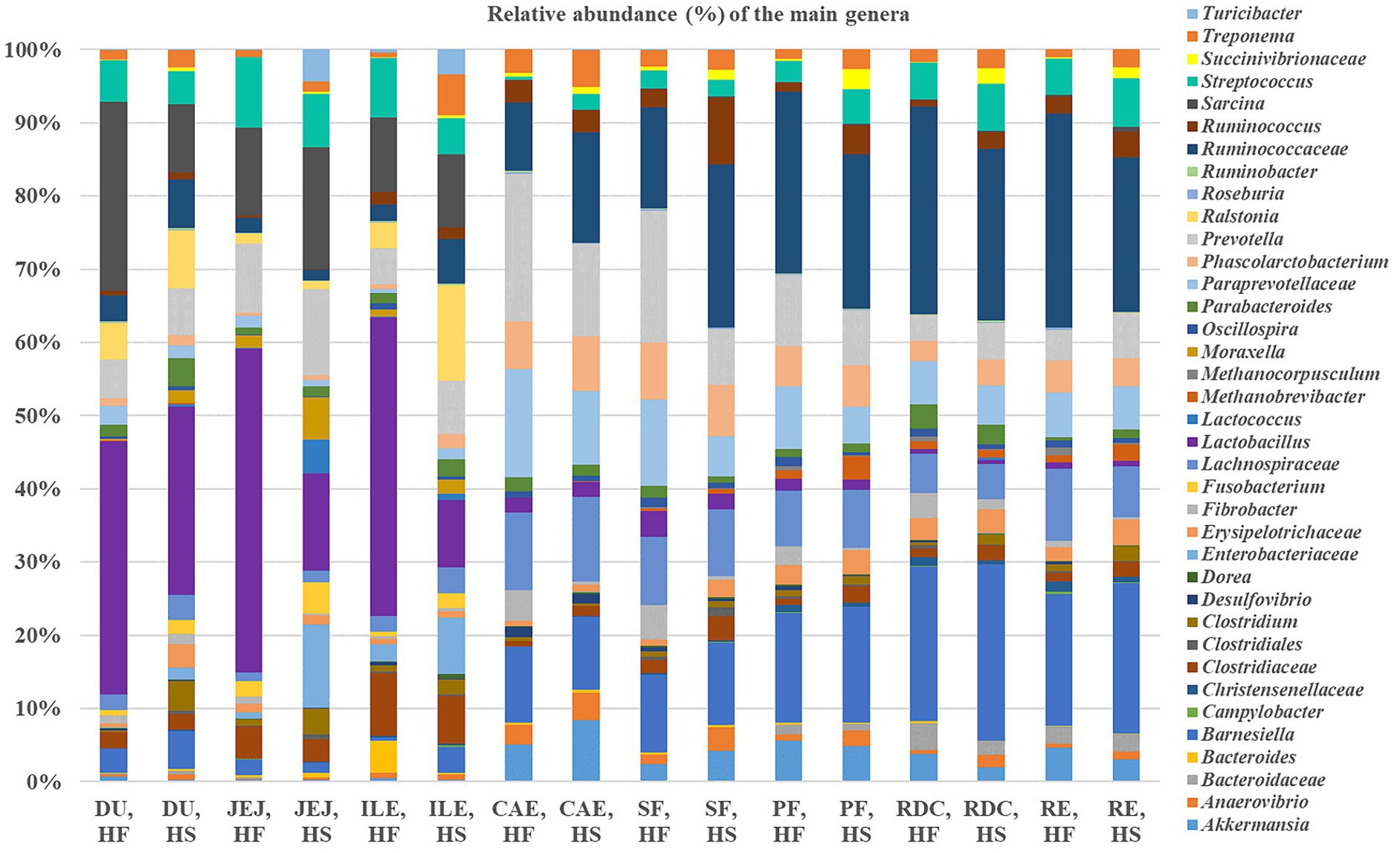
Figure 5. Relative frequencies (%) of the main genera across the different intestinal tract compartments (DU, duodenum; JEJ, jejunum; ILE, ileum; CAE, caecum; SF, sternal flexure; PF, pelvic flexure; RDC, right dorsal colon; RE, rectum) according to dietary group (high fiber, HF and high starch, HS).
In the jejunum, Anaevibrio (0.1% HF vs. 0.3% HS), Lactococcus (0% HF vs. 3% HS) and Turicibacter (2.9% HS vs. 0% HF) were all higher in horses fed the HS diet compared with the horses fed the HF diet (FDR < 0.05); whereas Fibrobacter (0.7% HF vs. 0.1% HS) and Lactobacillus (33.2% HF vs. 8.7% HS) were higher in the horses fed the HF diet compared to the HS diet (FDR < 0.05). A significant difference was found for Enterobacteriaceae which were more abundant in HS than HF (0.7% HF vs. 7.5% HS; FDR < 0.05).
By comparing the relative frequencies of the ASVs in the ileum, we found that Phascolarctobacterium was more abundant in horses fed HS than HF (0.5% HF vs. 1.1% HS; FDR < 0.05). The same was true for Barnesiella (0.4% HF vs. 2.1% HS; FDR < 0.05). Moreover, Enterobacteriaceae (0.7% in HF vs. 1.1% HS) and Ralstonia (2.6% HF vs. 7.8% HS) were higher in the ileum of the horses fed the HS diet compared to the horses fed the HF diet (FDR < 0.05).
The caecum was characterized by higher levels of Fibrobacter in HF compared with HS (3.8% HF vs. 0.3% HS; FDR < 0.05); whereas greater levels of Streptococcus were found in horses fed the HS diet (0.4% HF vs. 1.9% HS; FDR < 0.05).
Regarding the sternal flexure, Fibrobacter were greater in HF compared with HS (4.3% HF vs. 0.5% HS; FDR < 0.05), whereas Anaevibrio (1.2% HF vs. 2.9% HS) and Erysipelotrichaceae (0.8% HF vs. 2.1% HS) were significantly higher in HS (FDR < 0.05). Paraprevotellaceae (10.8% HF vs. 4.9% HS) and Prevotella (16.5% HF vs. 6.9% HS) were higher in HF compared with HS (FDR < 0.05). Moreover, Methanobrevibacter were more abundant in HS compared with HF (0.3% HF vs. 0.6% HS; FDR < 0.05) and Fusobacterium was only detected in horses fed HS (0% HF vs. 0.04% HS; FDR < 0.05).
The comparison of ASVs in the pelvic flexure showed Ruminococcus (1.2% HF vs. 3.6% HS), Anaevibrio (0.7% HF vs. 2% HS), Sarcinia (0% HF vs. 0.2% HS) and Roseburia (0.1% HF vs. 0.2% HS) to be greater in HS compared with HF (FDR < 0.05); whereas Dorea (0.2% HF vs. 0.1% HS) and Oscillospira (1.1% HF vs. 0.4% HS) were greater in horses fed the HF diet compared with HS (FDR < 0.05). Succinivibrionaceae were more abundant in HS compared with HF (0.3% HF vs. 2.5% HS; FDR < 0.05).
The right dorsal colon was characterized by greater levels of Clostridium (0.4% HF vs. 1.2% HS), and Ruminococcus (0.7% HF vs. 2% HS) in horses fed the HS diet compared with the horses fed the HF diet (FDR < 0.05). Clostridiales were more abundant in HF compared with HS (0.4% HF vs. 0.1% HS; FDR < 0.05). Prevotella was more abundant in HS (3% HF vs. 4.5% HS; FDR < 0.05), whereas Bacteroides were only detected in HF (0.3% HF vs. 0% HS; FDR < 0.05). Moreover, Akkermansia (3.3% HF vs. 1.8% HS) and Methanocorpusculum (0.1% HS vs. 0.6% HF) were present at greater levels in HF compared with HS (FDR < 0.05).
Finally, samples collected from the rectum of the horses fed the HF diet revealed higher relative abundances of bacteria belonging to Lachnospiraceae (8.7% HF vs. 6.3% HS; FDR < 0.05) and Ruminococcaceae (25.5% HF vs. 18.8% HS; FDR < 0.05). The results also revealed greater levels of Treponema (0.9% HF vs. 2.2% HS; FDR < 0.05) and Succinivibrionaceae in horses fed the HS diet compared with those fed HF (0.9% HF vs. 2.2% HS; FDR < 0.05).
Regarding functional differences in the microbiota of the two dietary groups, analysis revealed the HS diet to bring about an enrichment in genera involved in fermentative pathways, such as glycolysis/gluconeogenesis (ko00010), pyruvate metabolism, (ko00620), methane metabolism (ko00680) and propanoate metabolism (ko00640). Conversely, the HF diet brought about a reduction in potential microbiota functions related to fatty acid degradation (ko00071), nitrogen metabolism (ko00910) and sulfur metabolism (ko00920).
4 Discussion
The present study looked for variations in the gut microbiota along the length of the digestive tract of healthy horses according to two diets. We compared the effects of a high starch diet (HS), which exceeds the safe recommended level of 1 gram of starch/kg BW/meal (8), and a high fiber diet (HF), which satisfies the nutritional requirements of the horse.
The available scientific literature confirms the negative effects of HS diets on the gut health and welfare of horses (35), but a systematic assessment of the microbiota across the different compartments of the digestive tract is lacking (19). Microbiota composition is influenced by age, breed and the feed management system applied (23, 36). To avoid effects associated with breed or age in the present study, we used horses of the same age (14.3 ± 0.7 months) and breed (Bardigiano breed). They were not homogenous for sex; however, this is unlikely to have influenced the results since no sex-dependent differences in the gut microbiota were found in different gastrointestinal tract compartments of the horse analyzed using the NGS approach (18, 37). Moreover, all the horses of the present study were housed under the same environmental conditions, and the only difference was the diet.
Microbial populations were analyzed from alpha and beta diversity, bacterial populations present, and their potential function within the digestive tract of the horses.
A first important finding was that the horses fed the HF diet showed higher alpha diversity indices in the caecum, pelvic flexure and right dorsal colon compared with those fed the HS diet. This is relevant because high levels of bacterial diversity have been associated with greater stability of the intestinal microbiota and greater colonization resistance against potentially pathogenic bacteria (38, 39). However, the Shannon index was increased in the ileum of the horses fed the HS diet, whereas the alpha diversity indices of the other intestinal tract compartments were not different between the two diets. This could be explained by the individual variability or by the fact that the duration of the feeding trial (72 days) was too short to induce any significant changes in the other intestinal tract compartments. Moreover, the measures of beta diversity revealed significant compositional differences between the different intestinal tract compartments studied according to diet (HF vs. HS). This was not surprising since the diet is well-known to be the main factor affecting microbiota composition in the horse (1).
As already reported in the scientific literature, our findings support the division of the equine digestive tract into two distinct regions according to microbiota composition: the upper (duodenum, jejunum and ileum) and the lower (caecum, sternal flexure, pelvic flexure, right dorsal colon and rectum) digestive tract (see Figure 1) (18, 37). Interestingly, cluster analysis (Figure 4) revealed further differentiation within the hindgut: one cluster comprised the caecum and sternal flexure, and another was made up of the pelvic flexure, right dorsal colon and rectum; once again, confirming the findings of Costa and colleagues (18) who showed that that neighboring intestinal tract compartments are more similar to each other.
We show Firmicutes to be the primary bacterial phylum in all intestinal tract compartments, in accordance with the findings by other authors (18, 24, 40). Bacteroidetes was the second most abundant phylum (41, 42), and the third was Proteobacteria in the small intestine and Verrucomicrobia in the hindgut (19).
When we looked at the microbiota composition at genus/family level, we found interesting differences according to the diet adopted (HF vs. HS). The small intestine (jejunum and ileum) of horses fed the HS diet displayed a higher relative abundance of Proteobacteria compared with horses fed the HF diet as a result of a higher relative abundance of Ralstonia and Enterobacteriaceae. The increased presence of bacteria belonging to Proteobacteria is reported to be associated with inflammatory intestinal diseases in the horse (41). In particular, Enterobacteriaceae comprises some pathogenic bacteria, such as Escherichia Coli and Salmonella spp., which can lead to dysfunctions of the intestinal barrier, generating a condition of leaky gut and enteritis (1). Previous research published by our research team on the same horses fed the HS diet showed they were characterized by greater levels of inflammation of the intestinal wall in the jejunum (25) and by higher levels of intestinal permeability (15). Thus, we can speculate that these conditions were triggered by shifts in the microbiota composition associated with the consumption of a HS diet characterized by an overabundance of bacteria belonging to the Proteobacteria.
Progressing toward the more distal compartments of the digestive tract, the caecum and sternal flexure appeared similar in terms of their microbiota compositions, as shown in the heatmap (Figure 4). However, significant differences at the genus level revealed some positive effects of the HF diet related to an enhancement in metabolic pathways involved in fermentation processes with the production of short chain fatty acids (SCFAs). Specifically, the horses fed the HF diet showed higher frequencies of Fibrobacter in their caecal microbiota communities with respect to those fed the HS diet. Moreover, the abundances of Fibrobacter, Prevotella and Paraprevotellaceae within the sternal flexure were also greater. Dougal et al. (39) identified Fibrobacteraceae and Prevotellaceae as being two of the most abundant members of the core bacterial communities in the horse intestinal tract. Therefore, in our study the HS diet seems to be associated with a reduction in the overall size of the core microbiota within the caecum and sternal flexure. Such a reduction has been reported to increase the risk for digestive diseases and metabolic dysfunctions in the horse (43). Moreover, we found higher abundances of Streptococcus in the caecum and Fusobacterium in the sternal flexure of horses fed the HS diet, both of which are potential pathogenic bacteria associated with the presence of colitis or diarrhea (44). Indeed, the feces of horses fed the HS diet were less formed compared with those fed the HF diet (16).
Considering the distal intestinal tract compartments (pelvic flexure, right dorsal colon and rectum), Succinivibrionaceae were more abundant in the pelvic flexure and rectum of horses fed the HS diet. This is not surprising since these bacteria are amylolytic, and their greater prevalence is likely to be associated with the presence of undigested starch in the hindgut (26, 45). The samples collected from the pelvic flexure and right dorsal colon of both groups of horses showed compositional differences related to genera belonging to Firmicutes and Bacteroidetes. The majority of these taxa (Clostridium, Clostridiales, Ruminococcus, Prevotella and Bacteroides) are typical members of the physiological microbiota of the horse. However, it is interesting to note the positive effect that the HF diet had on the right dorsal colon. Samples collected from the right dorsal colon of horses fed the HF diet displayed a greater relative abundance of Akkermansia, a mucin-degrading genus that helps to maintain the integrity of the mucin layer of the intestinal barrier (19, 46). Finally, the fecal samples collected from the rectum of the horses fed the HF diet displayed an increase in the relative frequency of bacteria belonging to the Lachnospiraceae and Ruminococcaceae families, which form part of the intestinal core microbiota of the horse (39). Moreover, Spirochaetes (in particular Treponema), although at a lower abundance frequency than Lachnospiraceae and Ruminococcacea, were higher in the fecal samples of the horses fed the HS diet. This was an unexpected finding since these bacteria are involved in fiber degradation.
To the best of our knowledge, the present study is the first to evaluate the microbiota composition of all digestive tract compartments in horses homogenous for breed, age and previous management practices. A limitation of the present study was its small sample size. A second was the fact that the animals were not used as their own controls through comparison of their fecal microbiota. Studying the fecal microbiota before and after the feeding trial would have enabled us to discern whether limited changes of the microbiota differences in certain areas of the digestive tract between HF and HS groups were indeed attributable to the microbial composition of the ecological niches or a reflection of variability between horses. Nonetheless, the study provides evidence sustaining the view that each compartment of the intestinal tract behaves as an ecological niche, as first suggested by Costa and colleagues (18). Moreover, we demonstrated that insights into changes occurring at the lower taxonomic level along the length of the digestive tract (such as those caused by diet) cannot be gained by analyzing fecal samples collected from the rectum (which revealed a reduction of the overall size of the core bacterial community and an increase in amylolytic bacteria in the horses from the HS group). This finding has important practical implications for the design of studies aimed at investigating changes in the horse microbiota associated with certain variables, such as diet.
5 Conclusion
This is the first study to evaluate the effects of a high-fiber (HF) vs. a high-starch (HS) diet on the microbiota within the different intestinal tract compartments of healthy horses, homogenous for age and breed. The results confirm that each intestinal tract compartment of the horse’s digestive system represents a distinct ecological niche, and that diet significantly influences the microbiota composition. In particular, the overall size of the core community and the indices of alpha diversity were lower in horses fed the HS diet, which also showed higher levels of amylolytic bacteria. From the clinical perspective, our findings underscore the need to be cautious when drawing conclusions about the intestinal health of horses based on the analysis of the fecal microbiota. Fibrous diets should be promoted to prevent dysbiosis of the microbiota associated with high-starch diets.
Data availability statement
The data presented in the study are deposited in the NCBI repository, accession number PRJNA1105226.
Ethics statement
The animal studies were approved by Ethics Committee of the Department of Veterinary Sciences of the University of Turin (Italy) (Prot. N. 2202/2019). The studies were conducted in accordance with the local legislation and institutional requirements. Written informed consent was obtained from the owners for the participation of their animals in this study.
Author contributions
FR: Conceptualization, Data curation, Investigation, Methodology, Writing – original draft. SC: Data curation, Methodology, Resources, Writing – review & editing. DB: Project administration, Resources, Supervision, Writing – review & editing. PS: Methodology, Resources, Supervision, Writing – review & editing. IF: Formal analysis, Methodology, Software, Writing – review & editing. LC: Data curation, Formal analysis, Methodology, Software, Writing – review & editing. MC: Data curation, Formal analysis, Methodology, Software, Writing – review & editing. RM: Methodology, Writing – review & editing. DC: Data curation, Formal analysis, Writing – review & editing. EV: Conceptualization, Project administration, Resources, Supervision, Writing – review & editing.
Funding
The author(s) declare that no financial support was received for the research, authorship, and/or publication of this article.
Conflict of interest
The authors declare that the research was conducted in the absence of any commercial or financial relationships that could be construed as a potential conflict of interest.
Publisher’s note
All claims expressed in this article are solely those of the authors and do not necessarily represent those of their affiliated organizations, or those of the publisher, the editors and the reviewers. Any product that may be evaluated in this article, or claim that may be made by its manufacturer, is not guaranteed or endorsed by the publisher.
References
1. Stewart, AS, Pratt-Phillips, S, and Gonzalez, LM. Alterations in intestinal permeability: the role of the “leaky gut” in health and disease. J Equine Vet. (2017) 52:10–22. doi: 10.1016/j.jevs.2017.02.009
2. Valle, E, Giusto, G, Penazzi, L, Giribaldi, M, Bergero, D, Fradinho, MJ, et al. Preliminary results on the association with feeding and recovery length in equine colic patients after laparotomy. J Anim Physiol Anim Nutr. (2019) 103:1233–41. doi: 10.1111/jpn.13102
3. Daly, K, Proudman, CJ, Duncan, SH, Flint, HJ, Dyer, J, and Shirazi-Beechey, SP. Alterations in microbiota and fermentation products in equine large intestine in response to dietary variation and intestinal disease. Br J Nutr. (2012) 107:989–95. doi: 10.1017/S0007114511003825
4. Lorenzo-Figueras, M, Morisset, SM, Morisset, J, Lainé, J, and Merrit, AM. Digestive enzyme concentrations and activities in healthy pancreatic tissue of horses. Am J Vet Res. (2007) 68:1070–2. doi: 10.2460/ajvr.68.10.1070
5. Merritt, AM, and Julliand, V. Gastrointestinal physiology In: WB Saunders , editor. Equine applied and clinical nutrition. Amsterdam: Elsevier Ltd (2013). 3–32.
6. Bulmer, LS, Murray, JA, Burns, NM, Garber, A, Wemelsfelder, F, McEwan, NR, et al. High-starch diets alter equine faecal microbiota and increase behavioural reactivity. Sci Rep. (2019) 9:1–11. doi: 10.1038/s41598-019-54039-8
7. Coenen, M, Kienzle, E, Vervuert, I, and Zeyner, A. Recent German developments in the formulation of energy and nutrient requirements in horses and the resulting feeding recommendations. J Equine Vet. (2011) 31:219–29. doi: 10.1016/j.jevs.2011.03.204
8. Harris, P, and Shepherd, M. What would be good for all veterinarians to know about equine nutrition. Vet Clin North Am. (2021) 37:1–20. doi: 10.1016/j.cveq.2020.11.001
9. Vervuert, I, Voigt, K, Hollands, T, Cuddeford, D, and Coenen, M. Effect of feeding increasing quantities of starch on glycaemic and insulinaemic responses in healthy horses. Vet J. (2009) 182:67–72. doi: 10.1016/j.tvjl.2008.04.011
10. Hoffman, CJ, Costa, LR, and Freeman, LM. Survey of feeding practices, supplement use, and knowledge of equine nutrition among a subpopulation of horse owners in New England. J Equine Vet. (2009) 29:719–26. doi: 10.1016/j.jevs.2009.08.005
11. Murray, J-AMD, Bloxham, C, Kulifay, J, Stevenson, A, and Roberts, J. Equine nutrition: a survey of perceptions and practices of horse owners undertaking a massive open online course in equine nutrition. J Equine Vet. (2015) 35:510–7. doi: 10.1016/j.jevs.2015.02.005
12. Raspa, F, Tarantola, M, Bergero, D, Bellino, C, Mastrazzo, CM, Visconti, A, et al. Stocking density affects welfare indicators in horses reared for meat production. Animals. (2020) 10:1103. doi: 10.3390/ani10061103
13. Julliand, V, de Fombelle, A, Drogoul, C, and Jacoto, E. Feeding and microbial disorders in horses: part 3- effects of three hay: grain ratios on microbial profile and activities. J Equine Vet. (2001) 21:543–6. doi: 10.1016/S0737-0806(01)70159-1
14. Davis, JL, Blikslager, AT, Catto, K, and Jones, SL. A retrospective analysis of hepatic injury in horses with proximal enteritis (1984-2002). J Vet Intern Med. (2003) 17:896–901. doi: 10.1111/j.1939-1676.2003.tb02530.x
15. Raspa, F, Dinardo, FR, Vervuert, I, Bergero, D, Bottero, MT, Pattono, D, et al. A fibre-vs. cereal grain-based diet: which is better for horse welfare? Effects on intestinal permeability, muscle characteristics and oxidative status in horses reared for meat production. J Anim Physiol Anim Nutr. (2021) 106:313–26. doi: 10.1111/jpn.13643
16. Raspa, F, Vervuert, I, Capucchio, MT, Colombino, E, Bergero, D, Forte, C, et al. A high-starch vs. high-fibre diet: effects on the gut environment of the different intestinal compartments of the horse digestive tract. BMC Vet Res. (2022) 18:1–11. doi: 10.1186/s12917-022-03289-2
17. Ang, L, Vinderola, G, Endo, A, Kantanen, J, Jingfeng, C, Binetti, A, et al. Gut microbiome characteristics in feral and domesticated horses from different geographic locations. Commun Biol. (2022) 5:1–10. doi: 10.1038/s42003-022-03116-2
18. Costa, MC, Silva, G, Ramos, RV, Staempfli, HR, Arroyo, LG, Kim, P, et al. Characterization and comparison of the bacterial microbiota in different gastrointestinal tract compartments in horses. Vet J. (2015) 205:74–80. doi: 10.1016/j.tvjl.2015.03.018
19. Kauter, A, Epping, L, Semmler, T, Antao, E-M, Kannapin, D, Stoeckle, SD, et al. The gut microbiome of horses: current research on equine enteral microbiota and future perspectives. Anim Microbiome. (2019) 1:1–14. doi: 10.1186/s42523-019-0013-3
20. De Fombelle, A, Varloud, M, Goachet, AG, Jacotot, E, Philippeau, C, Drogoul, C, et al. Characterization of the microbial and biochemical profile of the different segments of the digestive tract in horses given two distinct diets. Anim Sci. (2003) 77:293–304. doi: 10.1017/S1357729800059038
21. Panek, M, Paljetak, HČ, Barešić, A, Perić, M, Matijašić, M, Lojkić, I, et al. Methodology challenges in studying human gut microbiota-effects of collection, storage, DNA extraction and next generation sequencing technologies OPEN. Sci Rep. (2018) 8:5143. doi: 10.1038/s41598-018-23296-4
22. Dougal, K, Harris, PA, Edwards, A, Pachebat, JA, Blackmore, TM, Worgan, HJ, et al. A comparison of the microbiome and the metabolome of different regions of the equine hindgut. FEMS Microbiol Ecol. (2012) 82:642–52. doi: 10.1111/j.1574-6941.2012.01441.x
23. Costa, MC, and Weese, JS. The equine intestinal microbiome. Anim Health Res Rev. (2012) 13:121–8. doi: 10.1017/S1466252312000035
24. Dougal, K, De La Fuente, G, Harris, PA, Girdwood, SE, Pinloche, E, and Newbold, CJ. Identification of a Core bacterial community within the large intestine of the horse. PLoS One. (2013) 8:e77660. doi: 10.1371/journal.pone.0077660
25. Colombino, E, Raspa, F, Perotti, M, Bergero, D, Vervuert, I, Valle, E, et al. Gut health of horses: effects of high fibre vs high starch diet on histological and morphometrical parameters. BMC Vet Res. (2022) 18:1–9. doi: 10.1186/s12917-022-03433-y
26. Raspa, F, Tarantola, M, Muca, E, Bergero, D, Soglia, D, Cavallini, D, et al. Does feeding management make a difference to behavioural activities and welfare of horses reared for meat production? Animals. (2022) 12:1740. doi: 10.3390/ani12141740
27. Martin-rosset, W . Equine nutrition: INRA nutrient requirements, recommended allowances and feed tables. Acad Publ. (2015). doi: 10.3920/978-90-8686-855-1
28. Klindworth, A, Pruesse, E, Schweer, T, Rg Peplies, J, Quast, C, Horn, M, et al. Evaluation of general 16S ribosomal RNA gene PCR primers for classical and next-generation sequencing-based diversity studies. Nucleic Acids Res. (2013) 41:e1. doi: 10.1093/nar/gks808
29. Bolyen, E, Ram Rideout, J, Dillon, MR, Bokulich, NA, Abnet, CC, Al-Ghalith, GA, et al. Reproducible, interactive, scalable and extensible microbiome data science using QIIME 2. Nat Biotechnol. (2019) 37:852–7. doi: 10.1038/s41587-019-0209-9
30. Callahan, BJ, Mcmurdie, PJ, Rosen, MJ, Han, AW, Johnson, AJA, and Holmes, SP. DADA2: high-resolution sample inference from illumina amplicon data. Nat Methods. (2016) 13:581–3. doi: 10.1038/nmeth.3869
31. Bokulich, NA, Kaehler, BD, Rideout, JR, Dillon, M, Bolyen, E, Knight, R, et al. Optimizing taxonomic classification of marker-gene amplicon sequences with QIIME 2’s q2-feature-classifier plugin. Microbiome. (2018) 6:1–17. doi: 10.1186/s40168-018-0470-z
32. Culhane, AC, Thioulouse, J, Perrière, G, and Higgins, DG. MADE4: an R package for multivariate analysis of gene expression data. Bioinformatics. (2005) 21:2789–90. doi: 10.1093/bioinformatics/bti394
33. Douglas, GM, Maffei, VJ, Zaneveld, JR, Yurgel, SN, Brown, JR, Taylor, CM, et al. PICRUSt2 for prediction of metagenome functions. Nat Biotechnol. (2020) 386:685–8. doi: 10.1038/s41587-020-0548-6
34. Luo, W, Friedman, MS, Shedden, K, Hankenson, KD, and Woolf, PJ. GAGE: generally applicable gene set enrichment for pathway analysis. BMC Bioinformatics. (2009) 10:1–17. doi: 10.1186/1471-2105-10-161
35. Al Jassim, RAM, and Andrews, FM. The bacterial Community of the Horse Gastrointestinal Tract and its Relation to fermentative acidosis, laminitis, colic, and stomach ulcers. Vet Clin North Am. (2009) 25:199–215. doi: 10.1016/j.cveq.2009.04.005
36. Carrillo Heredero, AM, Sabbioni, A, Asti, V, Ablondi, M, Summer, A, and Bertini, S. Fecal microbiota characterization of an Italian local horse breed. Front Vet Sci. (2024) 11:1236476. doi: 10.3389/fvets.2024.1236476
37. Ericsson, AC, Johnson, PJ, Lopes, MA, Perry, SC, and Lanter, HR. A microbiological map of the healthy equine gastrointestinal tract. PLoS One. (2016) 11:1–17. doi: 10.1371/journal.pone.0166523
38. Biasato, I, Ferrocino, I, Biasibetti, E, Grego, E, Dabbou, S, Sereno, A, et al. Modulation of intestinal microbiota, morphology and mucin composition by dietary insect meal inclusion in free-range chickens. BMC Vet Res. (2018) 14:1–15. doi: 10.1186/s12917-018-1690-y
39. Dougal, K, de la Fuente, G, Harris, PA, Girdwood, SE, Pinloche, E, Geor, RJ, et al. Characterisation of the faecal bacterial community in adult and elderly horses fed a high fibre, high oil or high starch diet using 454 pyrosequencing. PLoS One. (2014) 9:e87424. doi: 10.1371/journal.pone.0087424
40. Steelman, SM, Chowdhary, BP, Dowd, S, Suchodolski, J, and Janečka, JE. Pyrosequencing of 16S rRNA genes in fecal samples reveals high diversity of hindgut microflora in horses and potential links to chronic laminitis. BMC Vet Res. (2012) 8:1–11. doi: 10.1186/1746-6148-8-231
41. Costa, MC, Arroyo, LG, Allen-Vercoe, E, Stämpfli, HR, Kim, PT, Sturgeon, A, et al. Comparison of the fecal microbiota of healthy horses and horses with colitis by high throughput sequencing of the V3-V5 region of the 16s rRNA gene. PLoS One. (2012) 7:e41484. doi: 10.1371/journal.pone.0041484
42. Daly, K, Stewart, CS, Flint, HJ, and Shirazi-Beechey, SP. Bacterial diversity within the equine large intestine as revealed by molecular analysis of cloned 16S rRNA genes. FEMS Microbiol Ecol. (2001) 38:141–51. doi: 10.1111/j.1574-6941.2001.tb00892.x
43. Julliand, V, and Grimm, P. Horse species symposium: the microbiome of the horse hindgut: history and current knowledge. J Anim Sci. (2016) 94:2262–74. doi: 10.2527/jas.2015-0198
44. Rodriguez, C, Taminiau, B, Brévers, B, Avesani, V, Van Broeck, J, Leroux, A, et al. Faecal microbiota characterisation of horses using 16 rDNA barcoded pyrosequencing, and carriage rate of clostridium difficile at hospital admission. BMC Microbiol. (2015) 15:181. doi: 10.1186/s12866-015-0514-5
45. Harlow, BE, Lawrence, LM, Hayes, SH, Crum, A, and Flythe, MD. Effect of dietary starch source and concentration on equine fecal microbiota. PLoS One. (2016) 11:e0154037. doi: 10.1371/journal.pone.0154037
Keywords: horse, 16S rRNA, microbiota, nutrition, welfare
Citation: Raspa F, Chessa S, Bergero D, Sacchi P, Ferrocino I, Cocolin L, Corvaglia MR, Moretti R, Cavallini D and Valle E (2024) Microbiota characterization throughout the digestive tract of horses fed a high-fiber vs. a high-starch diet. Front. Vet. Sci. 11:1386135. doi: 10.3389/fvets.2024.1386135
Edited by:
Massimo Trabalza-Marinucci, University of Perugia, ItalyReviewed by:
Simon Daniels, Royal Agricultural University, United KingdomAlicia Maria Carrillo Heredero, University of Parma, Italy
Iolanda Altomonte, University of Pisa, Italy
Copyright © 2024 Raspa, Chessa, Bergero, Sacchi, Ferrocino, Cocolin, Corvaglia, Moretti, Cavallini and Valle. This is an open-access article distributed under the terms of the Creative Commons Attribution License (CC BY). The use, distribution or reproduction in other forums is permitted, provided the original author(s) and the copyright owner(s) are credited and that the original publication in this journal is cited, in accordance with accepted academic practice. No use, distribution or reproduction is permitted which does not comply with these terms.
*Correspondence: Federica Raspa, federica.raspa@unito.it