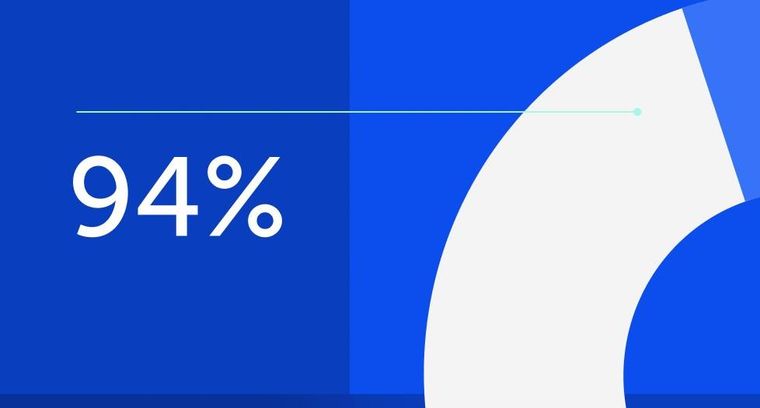
94% of researchers rate our articles as excellent or good
Learn more about the work of our research integrity team to safeguard the quality of each article we publish.
Find out more
ORIGINAL RESEARCH article
Front. Vet. Sci., 26 March 2024
Sec. Animal Nutrition and Metabolism
Volume 11 - 2024 | https://doi.org/10.3389/fvets.2024.1381871
This article is part of the Research TopicAnimal Health and Production: Identifying Challenges and Finding a Way ForwardView all 40 articles
This study conducted a comparison of the effects of non-protein nitrogen with different acid-base properties on feed intake, rumen fermentation, nutrient digestion and antioxidant capacity in fattening Hu sheep. Sixteen fattening male sheep (31.43 ± 2.41 kg) with permanent rumen cannulas were randomly assigned to two dietary treatments: 1% urea and 1.78% ammonium chloride (NH4Cl, AC). A 42 days experimental period was conducted, with 14 days for adaptation and 28 days for treatment. Daily feed intake was recorded and various samples including feed, feces, rumen fluid, and blood were collected at different time points during the final week. The results indicated that the urea group had significantly higher dry matter intake, average daily gain, and gain efficiency in comparison to the AC group (p < 0.01). There was no difference in rumen pH and concentration of ammonia nitrogen between different groups (p > 0.05), but the rumen pH of urea group was higher than that of the AC group at 1 and 3 h after feeding (p < 0.05). The urea group exhibited higher concentrations of total volatile fatty acids (VFA) and individual VFAs compared to the AC group at all-time points (p < 0.01). Compared to the urea group, the intake of all nutrients decreased in the AC group (p < 0.01), but the digestibility of dry matter and organic matter increased significantly (p < 0.01), and the digestibility of CP had an increasing trend (p = 0.06) in the AC group. Additionally, the urea group had lower levels of serum glucagon-like peptide-1, peptide YY, Cl, total protein and globulin than the AC group (p < 0.05). The overall levels of HCO3−, superoxide dismutase, glutathione peroxidase, catalase, albumin/globulin, blood urea nitrogen and total cholesterol in the urea group increased significantly compared to the AC group (p < 0.05). It was concluded that adding urea to the high-concentrate diet resulted in increased rumen pH and improved rumen fermentation and growth performance in fattening sheep compared to NH4Cl addition. Furthermore, urea addition improved sheep’s antioxidant capacity and maintained their acid-base balance more effectively as compared to NH4Cl.
Ruminants play a crucial role in global agricultural production by providing a significant portion of human milk and meat consumption (1). Soybean meal, as the most important protein feed resource for ruminants and other livestock and poultry in China, has faced long-term supply shortages and high import costs (2). Therefore, addressing the pressing need to reduce and replace soybean meal and mitigate feed costs in the face of high-priced protein is imperative for the ruminant industry. The distinctive digestive system of ruminants makes it possible to reduce production costs and dependence on protein resources by adding a minimal quantity of non-protein nitrogen (NPN) to their diet. NPN has been extensively employed in ruminant production in European and American (3, 4), and it exhibits considerable potential for reducing and substituting soybean meal in ruminant feed in China (2).
Depending on their chemical properties, NPNs can be classified into two categories: alkaline NPN, including urea, and diammonium hydrogen phosphate, and acidic NPN, such as NH4Cl, ammonium sulfate, ammonium dihydrogen phosphate. Urea, an alkaline NPN, releases free NH3 and CO2, which can elevate rumen pH (5–7). Studies have shown that appropriate addition of urea in the diet does not negatively affect the DMI, growth performance, nutrient digestion or rumen function of ruminants (8–10). NH4Cl, classified as an acidic NPN, generates NH4+and Cl− ions that have the potential to lower rumen pH (7, 11, 12). Dietary anion-cation difference (DCAD) is commonly used to measure the balance of cations and anions in the diet of animals. Adding NH4Cl to the diet can reduce DCAD, modulate diet acidity, and prevent urinary calculus (13, 14). However, excessive NH4Cl supplementation can negatively impact animal feed intake and growth performance (15). Consequently, the choice of NPN must be tailored to the diet composition, animal species or physiological stage taking into account the varying acidity and alkalinity of NPN. While previous research has concentrated on the diverse concentrations of NPN, there has been limited exploration into the impact of NPN’s acid-base properties on ruminant growth, rumen fermentation, nutrient utilization, and plasma metabolites.
A stable rumen environment is crucial for the efficient digestion and absorption, as well as for promoting the healthy development of ruminants. In both intensive and extensive production systems, most producers opt to provide their animals with a highly concentrated diet to enhance production performance and attain greater economic benefits (16). Nevertheless, a high-concentrate diet can potentially disturb the rumen fermentation environment, resulting in a decrease in rumen pH and the onset of rumen acidosis after prolonged feeding (17). The disruption of the epithelial barrier and subsequent inflammation, such as gastritis, mastitis, and hoof disease, caused by ruminal acidosis can pose a significant risk to the health of animals (18, 19). Given the current production context and the acid-base properties of NPN, it is hypothesized that the acid-base properties of NPN will initially modify the rumen pH, subsequently influencing the rumen fermentation environment and physiological indicators in blood, ultimately resulting in varied effects on feed intake and growth performance of animals. Therefore, the present study was performed to compare the effects of NPN with different acidity and alkalinity on feed intake, rumen fermentation, and antioxidant capacity in fattening Hu sheep by supplementing their high-concentrate diet with isonitrogenous urea or NH4Cl.
Sixteen 5 months fattening male Hu sheep (31.43 ± 2.41 kg) fitted with rumen cannula were assigned into two treatments using a completely randomized design. Two isonitrogenous and isoenergetic diets were prepared using NH4Cl and urea, respectively. The diet of the AC group contained 1.78% NH4Cl, while the diet of the urea group contained 1% urea. The two diets (Table 1) for fattening sheep were designed to meet their growth requirements (20).
This study spanned a period of 42 days, comprising of a 14 days adaptation phase and a subsequent 28 days dietary treatment phase. Throughout the duration of the experiment, all sheep were individually housed and fed twice a day at 07:30 h and 17:30 h respectively, with free access to drinking water. The quantity of diet dispensed was tailored to their daily feed intake, with a surplus of 5–10% being ensured.
Weights of each sheep were measured before morning feeding for three consecutive days before the trial, and subsequently on days 20, 21, and 22, in order to determine the average daily gain (ADG). The diet offered and orts were recorded daily to measure the average daily dry matter intake (DMI). ADG/DMI was used to calculate sheep gain efficiency.
Dietary samples (500 g) were collected at the initial, intermediate, and final stages of the trial, respectively. Fecal samples were collected from each sheep at 08:00 h and 18:00 h on days 22, 23, and 24. The feed and fecal samples of per sheep were subsampled and then stored at −20°C until analysis. All samples were thawed and dried for 48 h at 65°C at the end of the experiment. A Cyclotec mill (Tecator 1093; Tecator AB, Höganäs, Sweden) was used to grind the dried samples through a 1 mm screen before analysis. All samples were analyzed for dry matter (DM), organic matter (OM), crude protein (CP) and ether extract (EE) using the standard procedure of AOAC (21). According to Van Soest et al. (22), contents of acid detergent fiber (ADF) and neutral detergent fiber (NDF) were measured. The acid-insoluble ash (AIA) in the diet and fecal samples was measured and used as an internal marker to determine the apparent total tract nutrient digestibility (23). The dietary contents of Ca, P, Mg, S and Na were analyzed using Inductively Coupled Plasma-Optical Emission Spectrometer (Thermo Scientific, 7400), while the dietary contents of K and Cl were measured by commercial kits (Jiancheng Bioengineering Institute, Nanjing, China). The DCAD were calculated using the formula: mEq/kg of DM = (mEq of Na+ + mEq of K+) − (mEq of Cl− + mEq of S2−) (24).
Rumen fluid was collected through the rumen cannula at 0 h, 1 h, 3 h and 6 h after morning feeding on day 28, and was mixed and filtered through four layers of gauze. The values of rumen pH were instantly measured using a portable pH meter (pHS-10). One mL of each ruminal fluid sample was stored at −20°C and subsequently used to analyze the ammonia concentration by a colorimetric method (25). Another 1 mL of each ruminal fluid sample was supplemented with 0.2 mL of 25% HPO3, which was preserved to analyze VFA concentrations by gas chromatography (7890A, Agilent, Santa Clara, CA, United States) as described by Mao et al. (26).
Blood samples (9 mL) were collected from sheep’s jugular vein using heparinized vacutainer tubes at 0 h, 1 h, 3 h and 6 h after morning feeding on day 26. The samples were centrifuged at a speed of 3,000 × g for 15 min in order to obtain the plasma, which was then stored at −20°C for later analysis. The concentrations of blood urea nitrogen (BUN), glucose, total protein (TP), albumin, globulin, total cholesterol (TCHO), triglyceride (TG), and superoxide dismutase (SOD) were determined using an automatic biochemistry analyzer (Beckman Coulter AU680, United States). The hormone indexes, namely insulin, glucagon-like peptide-1 (GLP-1), and peptide YY (PYY), were analyzed using the ELISA kits (Jiangsu Meimian Industrial Co., Ltd., Jiangsu, China). Glutathione peroxidase (GSH-Px), catalase (CAT) and malondialdehyde (MDA) were measured by commercial kits (Jiancheng Bioengineering Institute, Nanjing, China).
SAS 9.4 (SAS Institute Inc., Cary, NC, United States) were performed for data analysis using the MIXED procedure. Preliminary data examination revealed that all dependent variables were normally distributed. When data for growth performance (DMI, BW, ADG, gain efficiency), nutrient digestion were analyzed, a fixed effect of treatment and a random effect of sheep were included in the statistical model. Data for rumen fermentation parameters and blood indicators were analyzed with time as repeated measures using covariance type of autoregressive (1), which provides the best fit according to Akaike’s information criterion. The statistical model incorporated treatment, time, and the interaction of treatment × time as a fixed effect, while sheep were considered a random effect. A statistically significant difference was considered to exist when the p-values were <0.05, and trends were declared at 0.05 ≤ p < 0.10. Unless otherwise stated, all values are least-squares means.
The chemical composition of the AC group’s diet was comparable to that of the urea group, with the exception of Cl ion levels (Table 1). Consequently, the calculated DCADs for the AC and urea groups were −72.74 and 164.35, respectively. As shown in Figure 1, no significant difference was observed in initial DMI between the AC and urea groups (p > 0.05). Nevertheless, the DMI of the AC group decreased significantly from day 1 to day 28 when compared to the urea group (p < 0.05). Additionally, there was no significant difference in the initial BW of sheep between different treatments (Table 2; p = 0.90). However, the final BW, ADG, DMI and G: F of the urea group were significantly higher compared to the AC group (p < 0.05).
Figure 1. Dynamic changes of feed intake in fattening sheep fed with ammonium chloride and urea. Values are least squares means ± SE (n = 8).
Table 2. Comparative effects of ammonium chloride and urea supplementation on growth performance in fattening sheep.
As shown in Figure 2, there were no significant interaction effects of treatment and time on rumen pH, ammonia concentrations, and VFA (p > 0.05). Rumen pH in the urea group was higher at 1 and 3 h after morning feeding compared to the AC group (p < 0.01). Furthermore, time had a significant impact on rumen pH, with both groups experiencing the lowest levels at 3 h after feeding (p < 0.01). There was no difference in the concentration of ammonia nitrogen between different treatments at all-time points (p > 0.05). However, both concentrations of ammonia nitrogen in the two groups exhibited a rising trend initially, followed by a decline, with the maximum value being attained at 1 h after morning feeding (p < 0.01). The levels of individual and total VFA in the urea group were higher than those in the AC group at all-time points (p < 0.05).
Figure 2. Comparative effects of ammonium chloride and urea supplementation on rumen fermentation parameters in fattening sheep. Values are least squares means ± SE (n = 8).
Sheep in the AC group consumed significantly less DM, OM, CP, NDF, ADF, and EE in comparison to the urea group (p < 0.01; Table 3). However, the apparent digestibility of DM and OM increased (p < 0.01) and that of CP tended to increase (p = 0.06) in the AC group. No significant difference was shown in the apparent digestibility of NDF, ADF, and EE between different treatments (p > 0.05).
Table 3. Comparative effects of ammonium chloride and urea supplementation on nutrients intake and apparent total tract digestibility of nutrients in fattening sheep.
As shown in Figure 3, there was a significant treatment × time interaction effect on HCO3− (p < 0.05), while no interaction effects were observed on GLP-1, PYY, insulin, K, Na, Cl, Ca, and Mg (p > 0.05). Additionally, the overall levels of GLP-1 and PYY in the AC group increased significantly (p < 0.05), and the level of Cl in the AC group was higher at all-time points (p < 0.01) in comparison to the urea group. In contrast, the level of HCO3− in the AC group decreased significantly at all-time points in comparison to the urea group (p < 0.01). No difference was observed in insulin, K, Na, Ca and Mg between the two groups (p > 0.05).
Figure 3. Comparative effects of ammonium chloride and urea supplementation on blood hormones and ions in fattening sheep. Values are least squares means ± SE (n = 8).
As shown in Figure 4, there were significant treatment × time interaction effects on MDA and glucose levels (p < 0.05), while no interaction effects on T-SOD, GSH-Px, CAT, TP, albumin, globulin, albumin/globulin, BUN, TCHO, and TG (p > 0.05). Compared with the AC group, the overall levels of TP and globulin decreased significantly (p < 0.05), while the overall levels of SOD, GSH-Px, CAT, albumin, albumin/globulin, BUN and TCHO increased significantly in the urea group (p < 0.05). Additionally, the concentration of MDA in the urea group decreased significantly at 1 h (p < 0.05) compared to the AC group. No significant difference was observed in glucose and TG between different treatments (p > 0.05).
Figure 4. Comparative effects of ammonium chloride and urea supplementation on antioxidant indices and blood parameters in fattening sheep. Values are least squares means ± SE (n = 8).
Addition dosage of NPN affects the DMI of ruminants (4, 27). Supplementation with 1% urea has been proved by previous studies to improve the DMI of ruminants without negative effects on growth performance (9, 28). In contrast, several studies have demonstrated that high levels of NH4Cl, whether added to the diet of cattle (85.1 g/day equating to approximately 0.89% DM) or injected into the rumen of dairy cows (300 g/day equating to approximately 1.76% DM), can reduce DMI and growth performance of ruminants (15, 29). Therefore, it is reasonable to speculate that the addition of 1.78% NH4Cl to satisfy the isonitrogenous conditions equivalent to 1% urea in this study exceeded the appropriate range, resulting in a notable reduction in the DMI and growth performance of sheep.
NH4Cl can dissociate into NH4+ and Cl−, and dietary addition of NH4Cl could reduce DCAD (30). A low or negative DCAD can result in a decrease in rumen pH and HCO3− levels in the blood (31), which was in line with the present study. A reduction in DCAD has been associated with a decrease in ruminant DMI according to several studies (32, 33). Additionally, Zimpel et al. (34) have reported that ruminants fed with a low DCAD would experience metabolic acidosis, which may result in the observed decrease in DMI. In this study, the rumen pH of sheep in the AC group experienced a decline below 6.0 for over 5 h from 1 h after morning feeding, accompanied by a decrease in the level of HCO3− in the blood. This occurrence may suggest that the sheep in the AC group were in a state of metabolic acidosis, leading to a serious decline in their DMI. Furthermore, it was observed in this study that sheep in the AC group exhibited heightened levels of hormones such as GLP-1 and PYY in their serum, which can inhibit their feed intake (35, 36). This discovery has contributed supplementary knowledge regarding the reduction in ruminant feed consumption.
The growth performance of animals is directly influenced by the intake and apparent digestibility of nutrients (37). According to the present study, sheep belonging to the AC group exhibited reduced feed consumption, consequently leading to insufficient intake of essential nutrients. Nevertheless, the AC group demonstrated an enhanced ability to digest DM, OM, and CP, suggesting the animals’ efforts to enhance nutrient digestion and absorption in order to meet their metabolic requirements. However, despite the observed improvement in digestion, it was not enough to compensate for the inadequate nutrient intake, leading to inferior growth performance in comparison to the urea group. In addition, the elevated feed intake observed in the urea group may improve the rate of diet flow through the gastrointestinal tract, leading to insufficient interaction between the diet and digestive enzymes, ultimately causing incomplete digestion and absorption of a portion of the diet. According to Castaneda et al. (38), the intake of DM, OM, CP, NDF, ADF and EE significantly decreased, while the digestibility of DM, OM, CP and NDF significantly increased with the progressive substitution of urea with isonitrogenous NH4Cl in the diet of Holstein bulls, which showed the same trend as the results of this study.
The rumen is a vital organ for ruminants to digest and absorb nutrients. The optimal internal environment of the rumen, including pH and ammonia nitrogen concentration, plays an essential role in the absorption of ammonia through the rumen epithelium and the proliferation of rumen microorganisms (39). Shen et al. (7) found in an in vitro fermentation trial that urea addition increased rumen pH, while NH4Cl addition slightly reduced rumen pH, which aligns with the findings of this study. It is noteworthy that high-concentrate diets have been extensively utilized in contemporary intensive production to enhance growth performance and economic efficiency. However, this may lead to a decline in the rumen pH and an augmented likelihood of rumen acidosis, which is detrimental to the healthy development of ruminants. In a previous study, Xu et al. (9) found that rumen pH increased with the increase of urea addition to a high-concentrate diet, indicating that urea addition can mitigate the low rumen pH caused by high-concentrate diets and diminish the risk of rumen acidosis.
Ammonia concentration in in vivo rumen fluids is predominantly influenced by the interplay among the rate of ammonia production, microbial utilization, and absorption through the rumen epithelium. The current study revealed that the AC group, which exhibited a lower CP intake but comparable digestibility to the urea group, suggested a decrease in ammonia production. Nevertheless, the AC group exhibited a comparable ammonia nitrogen concentration to the urea group, potentially due to a reduction in ammonia absorption through the rumen epithelium and a decrease in microbial utilization for the production of microbial protein. It has been proved that pH is a crucial determinant of ammonia absorption of rumen epithelium (40). The rate of ammonia absorption through the rumen epithelium increases with the increase in rumen pH (41). Therefore, in the present study, the decrease in rumen pH of the AC group indicated a reduced absorption of ammonia through the rumen epithelium. Besides, a low rumen pH is known to be unfavorable for rumen microbial fermentation (42), which may prevent microorganisms from synthesizing microbial protein from ammonia. The reduced ammonia utilization by microbes in the AC group is substantiated by the decreased pH and total VFA concentration.
Both GLP-1 and PYY serve as satiety signals that can reduce animal feed intake by modulating intestinal movement and increasing bodily satiety (43, 44). It was found by Relling et al. (45) that the serum GLP-1 level of animals increased and their feed intake decreased significantly by injecting GLP-1 into sheep, indicating that blood hormones related to feed intake play a crucial role in regulating sheep’s DMI. Therefore, the substantial reduction in DMI observed in the AC group may be attributed to the increase in GLP-1, PYY, and other hormones in the blood. According to Ahmad and Sarwar (46), a positive correlation was observed between dietary Cl− levels and blood Cl− levels in broilers, and high levels of Cl− were found to reduce the level of HCO3− in the blood, which is consistent with the present findings in sheep. Furthermore, HCO3− can not only improve the buffering capacity and keep the acid-base balance of the blood, but also serve as an indicator of metabolic acidosis in animals (47). In the present study, sheep in the AC group exhibited reduced serum HCO3− levels, indicating a potential decrease in blood buffering capacity and the possibility of metabolic acidosis, which may have contributed to a reduced appetite.
BUN mainly comes from the absorption of ammonia nitrogen through the rumen epithelium and the decomposition of tissue protein, with a strong relationship to the concentration of ammonia nitrogen in the rumen (48). While ammonia nitrogen levels in the rumen were comparable between different groups in this study, a variation in rumen pH was observed. A study conducted by Abdoun et al. (40) revealed that an increase in rumen pH led to a corresponding rise in the overall quantity of ammonia absorbed through the rumen epithelium. Consequently, the urea group exhibited a greater rate of absorption of ammonia through the rumen epithelium than the AC group, leading to a significantly higher BUN level in the former. These findings align with those of Castaneda et al. (38), who found a linear decrease in BUN levels with an increase in isonitrogenous NH4Cl substitution for urea in the diet of Holstein bulls.
Both T-SOD and GSH-Px are endogenous antioxidant enzymes that safeguard the body against oxidative stress and injury (49). In contrast, MDA is the final product of lipid peroxidation, and it can give an indication of cell damage (50). In addition, the levels of albumin and globulin can serve as markers of the immune status of the body (51). It has been shown that the levels of T-SOD, CAT, and GSH-Px in serum decrease, while the level of MDA increases when sheep are under stress (52). In this study, sheep in the AC group exhibited reduced levels of T-SOD, CAT, and GSH-Px, and an increased level of MDA, indicating a decline in their antioxidant capacity and the potential occurrence of oxidative stress following the consumption of a diet containing NH4Cl. Under such conditions, the oxidation of albumin may result in a loss of its activity (53). Concurrently, in an effort to mitigate oxidative harm, sheep in the AC group may elevate globulin levels and enhance their own immunity, potentially leading to hyper humoral immunity. In a previous study, De and Dey found that goats with lower antioxidant capacity displayed a decreased level of albumin, while experiencing an elevation in globulin level (54), which is consistent with the present study.
The addition of isonitrogenous 1% urea and 1.78% NH4Cl resulted in variations in feed intake, rumen fermentation, nutrient digestion and antioxidant capacity of fattening sheep. Adding urea to the basal high-concentrate diet led to an increased rumen pH and an enhancement of rumen fermentation and growth performance in fattening sheep, as compared to NH4Cl addition. Urea addition was found to be more conducive to maintaining the acid-base balance and enhancing the antioxidant capacity of sheep in comparison to NH4Cl.
The original contributions presented in the study are included in the article/supplementary material, further inquiries can be directed to the corresponding author.
All animal protocols were approved by the Animal Care and Use Committee of Nanjing Agricultural University (Protocol number: SYXK2017-0007). The study was conducted in accordance with the local legislation and institutional requirements.
WZ: Data curation, Formal analysis, Investigation, Visualization, Writing – original draft. HD: Investigation, Visualization, Writing – original draft. LC: Investigation, Visualization, Writing – original draft. SM: Methodology, Resources, Supervision, Writing – review & editing. JS: Conceptualization, Data curation, Funding acquisition, Methodology, Project administration, Resources, Writing – review & editing.
The author(s) declare financial support was received for the research, authorship, and/or publication of this article. This work was financially supported by the National Natural Science Foundation of China (32272897).
The authors declare that the research was conducted in the absence of any commercial or financial relationships that could be construed as a potential conflict of interest.
All claims expressed in this article are solely those of the authors and do not necessarily represent those of their affiliated organizations, or those of the publisher, the editors and the reviewers. Any product that may be evaluated in this article, or claim that may be made by its manufacturer, is not guaranteed or endorsed by the publisher.
1. Cholewińska, P, Czyż, K, Nowakowski, P, and Wyrostek, A. The microbiome of the digestive system of ruminants—a review. Anim Health Res Rev. (2020) 21:3–14. doi: 10.1017/s1466252319000069
2. Shen, J, Zheng, W, Mao, S, Qu, M, and Bu, D. Potential of non-protein nitrogen in the replacement of soybean meal in ruminant livestock feed. China Feed. (2023) 717:14–9. doi: 10.15906/j.cnki.cn11-2975/s.2022050070-11, (in Chinese)
3. Helmer, LG, and Bartley, EE. Progress in the utilization of urea as a protein replacer for ruminants. A review. J Dairy Sci. (1971) 54:25–51. doi: 10.3168/jds.S0022-0302(71)85776-4
4. Kertz, AF . Review: urea feeding to dairy cattle: a historical perspective and review. Prof Anim Sci. (2010) 26:257–72. doi: 10.15232/s1080-7446(15)30593-3
5. Webb, DW, Bartley, EE, and Meyer, RM. A comparison of nitrogen metabolism and ammonia toxicity from ammonium acetate and urea in cattle. J Anim Sci. (1972) 35:1263–70. doi: 10.2527/jas1972.3561263x
6. Nichols, K, de Carvalho, IPC, Rauch, R, and Martín-Tereso, J. Review: unlocking the limitations of urea supply in ruminant diets by considering the natural mechanism of endogenous urea secretion. Animal. (2022) 16:100537. doi: 10.1016/j.animal.2022.100537
7. Shen, J, Zheng, W, Xu, Y, and Yu, Z. The inhibition of high ammonia to in vitro rumen fermentation is Ph dependent. Front Vet Sci. (2023) 10:1163021. doi: 10.3389/fvets.2023.1163021
8. Benedeti, PDB, Paulino, PVR, Marcondes, MI, Valadares Filho, SC, Martins, TS, Lisboa, EF, et al. Soybean meal replaced by slow release urea in finishing diets for beef cattle. Livest Sci. (2014) 165:51–60. doi: 10.1016/j.livsci.2014.04.027
9. Xu, Y, Li, Z, Moraes, LE, Shen, J, Yu, Z, and Zhu, W. Effects of incremental urea supplementation on rumen fermentation, nutrient digestion, plasma metabolites, and growth performance in fattening lambs. Animals. (2019) 9:652. doi: 10.3390/ani9090652
10. Kraiprom, T, Jantarat, S, Yaemkong, S, Cherdthong, A, and Incharoen, T. Feeding Thai native sheep molasses either alone or in combination with urea-fermented sugarcane bagasse: the effects on nutrient digestibility, rumen fermentation, and hematological parameters. Vet Sci. (2022) 9:415. doi: 10.3390/vetsci9080415
11. Kertz, AF, Davidson, LE, Cords, BR, and Puch, HC. Ruminal infusion of ammonium chloride in lactating cows to determine effect of Ph on ammonia trapping. J Dairy Sci. (1983) 66:2597–601. doi: 10.3168/jds.S0022-0302(83)82133-X
12. Patra, AK, and Yu, Z. Essential oils affect populations of some rumen bacteria in vitro as revealed by microarray (RumenBactArray) analysis. Front Microbiol. (2015) 6:297. doi: 10.3389/fmicb.2015.00297
13. Mavangira, V, Cornish, JM, and Angelos, JA. Effect of ammonium chloride supplementation on urine Ph and urinary fractional excretion of electrolytes in goats. J Am Vet Med Assoc. (2010) 237:1299–304. doi: 10.2460/javma.237.11.1299
14. Peleki, C, Kiosis, E, Polizopoulou, ZS, Tsousis, G, Fthenakis, GC, Giadinis, ND, et al. Effect of sugar beet pulp and anionic salts on metabolic status and mineral homeostasis during the peri-parturient period of dairy sheep. Animals. (2023) 13:213. doi: 10.3390/ani13020213
15. Wang, K, Nan, X, Zhao, P, Liu, W, Drackley, JK, Liu, S, et al. Effects of low dietary cation-anion difference induced by ruminal ammonium chloride infusion on performance, serum, and urine metabolites of lactating dairy cows. Asian Australas J Anim Sci. (2018) 31:677–85. doi: 10.5713/ajas.17.0530
16. Elmhadi, ME, Ali, DK, Khogali, MK, and Wang, H. Subacute ruminal acidosis in dairy herds: microbiological and nutritional causes, consequences, and prevention strategies. Anim Nutr. (2022) 10:148–55. doi: 10.1016/j.aninu.2021.12.008
17. Monteiro, HF, and Faciola, AP. Ruminal acidosis, bacterial changes, and lipopolysaccharides. J Anim Sci. (2020) 98:skaa248. doi: 10.1093/jas/skaa248
18. Aschenbach, JR, Zebeli, Q, Patra, AK, Greco, G, Amasheh, S, and Penner, GB. Symposium review: the importance of the ruminal epithelial barrier for a healthy and productive cow. J Dairy Sci. (2019) 102:1866–82. doi: 10.3168/jds.2018-15243
19. Zhang, H, Peng, AL, Zhao, FF, Yu, LH, Wang, MZ, Osorio, JS, et al. Thiamine ameliorates inflammation of the ruminal epithelium of Saanen goats suffering from subacute ruminal acidosis. J Dairy Sci. (2020) 103:1931–43. doi: 10.3168/jds.2019-16944
20. AOAC . Official methods of analysis. 18th ed. Gaithersburg: Association of Official Analytical chemists (2007).
21. Ministry of Agriculture and Rural Affairs of the People’s Republic of China . Feeding standards of meat-producing sheep and goats (NY/T 816–2004). Beijing, China: China Agriculture Press (2004).
22. Van Soest, PJ, Robertson, JB, and Lewis, BA. Methods for dietary fiber, neutral detergent Fiber, and nonstarch polysaccharides in relation to animal nutrition. J Dairy Sci. (1991) 74:3583–97. doi: 10.3168/jds.S0022-0302(91)78551-2
23. Van Keulen, J, and Young, BA. Evaluation of acid-insoluble ash as a natural marker in ruminant digestibility studies. J Anim Sci. (1977) 44:282–7. doi: 10.2527/jas1977.442282x
24. Tucker, WB, Hogue, JF, Waterman, DF, Swenson, TS, Xin, Z, Hemken, RW, et al. Role of sulfur and chloride in the dietary cation-anion balance equation for lactating dairy cattle. J Anim Sci. (1991) 69:1205–13. doi: 10.2527/1991.6931205x
25. Chaney, AL, and Marbach, EP. Modified reagents for determination of urea and ammonia. Clin Chem. (1962) 8:130–2. doi: 10.1093/clinchem/8.2.130
26. Mao, SY, Zhang, G, and Zhu, WY. Effect of disodium fumarate on ruminal metabolism and rumen bacterial communities as revealed by denaturing gradient gel electrophoresis analysis of 16s ribosomal DNA. Anim Feed Sci Technol. (2008) 140:293–306. doi: 10.1016/j.anifeedsci.2007.04.001
27. Patra, AK . Urea/ammonia metabolism in the rumen and toxicity in ruminants In: Rumen microbiology: from evolution to revolution. New Delhi: Springer (2015). 329–41.
28. Emmanuel, N, Patil, NV, Bhagwat, SR, Lateef, A, Xu, K, and Liu, H. Effects of different levels of urea supplementation on nutrient intake and growth performance in growing camels fed roughage based complete pellet diets. Anim Nutr. (2015) 1:356–61. doi: 10.1016/j.aninu.2015.12.004
29. Crookshank, HR, Smalley, HE, Furr, D, and Ellis, GF. Ammonium chloride and ammonium sulfate in cattle feedlot finishing rations. J Anim Sci. (1973) 36:1007–9. doi: 10.2527/jas1973.3661007x
30. Iwaniuk, ME, and Erdman, RA. Intake, milk production, ruminal, and feed efficiency responses to dietary cation-anion difference by lactating dairy cows. J Dairy Sci. (2015) 98:8973–85. doi: 10.3168/jds.2015-9949
31. Sharif, M, Shahzad, MA, Nisa, M-u, and Sarwar, MA. Dietary cation anion difference: impact on productive and reproductive performance in animal agriculture. Afr J Biotechnol. (2010) 9:7976–88. doi: 10.5897/AJB09.1926
32. Charbonneau, E, Pellerin, D, and Oetzel, GR. Impact of lowering dietary cation-anion difference in nonlactating dairy cows: a meta-analysis. J Dairy Sci. (2006) 89:537–48. doi: 10.3168/jds.S0022-0302(06)72116-6
33. Nguyen, T, Chanpongsang, S, Chaiyabutr, N, and Thammacharoen, S. Effects of dietary cation and anion difference on eating, ruminal function and plasma leptin in goats under tropical condition. Asian Australas J Anim Sci. (2020) 33:941–8. doi: 10.5713/ajas.19.0288
34. Zimpel, R, Poindexter, MB, Vieira-Neto, A, Block, E, Nelson, CD, Staples, CR, et al. Effect of dietary cation-anion difference on acid-base status and dry matter intake in dry pregnant cows. J Dairy Sci. (2018) 101:8461–75. doi: 10.3168/jds.2018-14748
35. Batterham, RL, Cowley, MA, Small, CJ, Herzog, H, Cohen, MA, Dakin, CL, et al. Gut hormone PYY(3-36) physiologically inhibits food intake. Nature. (2002) 418:650–4. doi: 10.1038/nature00887
36. Smith, NK, Hackett, TA, Galli, A, and Flynn, CR. GLP-1: molecular mechanisms and outcomes of a complex signaling system. Neurochem Int. (2019) 128:94–105. doi: 10.1016/j.neuint.2019.04.010
37. Soares, LFP, Guim, A, de Mello, ACL, de Andrade, FM, de Carvalho, FFR, de Lima, SJ, et al. Dwarf and tall elephant grass silages: intake, nutrient digestibility, nitrogen balance, ruminal fermentation, and ingestive behavior in sheep. Trop Anim Health Prod. (2023) 55:93. doi: 10.1007/s11250-023-03502-w
38. Castaneda, RD, Branco, AF, Coneglian, SM, Barreto, JC, Granzotto, F, and Teixeira, S. Replacing urea with ammonium chloride in cattle diets: digestibility, synthesis of microbial protein, and rumen and plasma parameters/Substituicao De Ureia por Cloreto De Amonio Em Dietas De Bovinos: Digestibilidade, Sintese De Proteina Microbiana, Parametros Ruminais E Sanguineos. Acta Sci. (2009) 31:271. doi: 10.4025/actascianimsci.v31i3.5938
39. Hailemariam, S, Zhao, S, He, Y, and Wang, J. Urea transport and hydrolysis in the rumen: a review. Anim Nutr. (2021) 7:989–96. doi: 10.1016/j.aninu.2021.07.002
40. Abdoun, K, Stumpff, F, Wolf, K, and Martens, H. Modulation of electroneutral Na transport in sheep rumen epithelium by luminal ammonia. Am J Physiol Gastrointest Liver Physiol. (2005) 289:G508–20. doi: 10.1152/ajpgi.00436.2004
41. Abdoun, K, Stumpff, F, and Martens, H. Ammonia and urea transport across the rumen epithelium: a review. Anim Health Res Rev. (2006) 7:43–59. doi: 10.1017/S1466252307001156
42. Calsamiglia, S, Cardozo, PW, Ferret, A, and Bach, A. Changes in rumen microbial fermentation are due to a combined effect of type of diet and Ph. J Anim Sci. (2008) 86:702–11. doi: 10.2527/jas.2007-0146
43. Flint, A, Raben, A, Astrup, A, and Holst, JJ. Glucagon-like peptide 1 promotes satiety and suppresses energy intake in humans. J Clin Invest. (1998) 101:515–20. doi: 10.1172/jci990
44. Zapata, RC, Singh, A, and Chelikani, PK. Peptide YY mediates the satiety effects of diets enriched with whey protein fractions in male rats. FASEB J. (2018) 32:850–61. doi: 10.1096/fj.201700519RR
45. Relling, AE, Reynolds, CK, and Loerch, SC. Effect of feeding fat or Intrajugular infusion of glucagon-like peptide-1 and cholecystokinin on dry matter intake, digestibility, and digesta rate of passage in growing wethers. J Anim Sci. (2011) 89:168–78. doi: 10.2527/jas.2010-2976
46. Ahmad, T, and Sarwar, M. Dietary electrolyte balance: implications in heat stressed broilers. Worlds Poult Sci J. (2006) 62:638–53. doi: 10.1017/S0043933906001188
47. Kraut, JA, and Madias, NE. Metabolic acidosis: pathophysiology, diagnosis and management. Nat Rev Nephrol. (2010) 6:274–85. doi: 10.1038/nrneph.2010.33
48. Chumpawadee, S, Sommart, K, Vongpralub, T, and Pattarajinda, V. Effects of synchronizing the rate of dietary energy and nitrogen release on ruminal fermentation, microbial protein synthesis, blood urea nitrogen and nutrient digestibility in beef cattle. Asian Australas J Anim Sci. (2005) 19:181–8. doi: 10.5713/ajas.2006.181
49. Cecerska-Heryć, E, Surowska, O, Heryć, R, Serwin, N, Napiontek-Balińska, S, and Dołęgowska, B. Are antioxidant enzymes essential markers in the diagnosis and monitoring of cancer patients—a review. Clin Biochem. (2021) 93:1–8. doi: 10.1016/j.clinbiochem.2021.03.008
50. Del Rio, D, Stewart, AJ, and Pellegrini, N. A review of recent studies on malondialdehyde as toxic molecule and biological marker of oxidative stress. Nutr Metab Cardiovasc Dis. (2005) 15:316–28. doi: 10.1016/j.numecd.2005.05.003
51. Belinskaia, DA, Voronina, PA, and Goncharov, NV. Integrative role of albumin: evolutionary, biochemical and pathophysiological aspects. J Evol Biochem Physiol. (2021) 57:1419–48. doi: 10.1134/s002209302106020x
52. Shi, L, Xu, Y, Mao, C, Wang, Z, Guo, S, Jin, X, et al. Effects of heat stress on antioxidant status and immune function and expression of related genes in lambs. Int J Biometeorol. (2020) 64:2093–104. doi: 10.1007/s00484-020-02000-0
53. Wada, Y, Takeda, Y, and Kuwahata, M. Potential role of amino acid/protein nutrition and exercise in serum albumin redox state. Nutrients. (2017) 10:17. doi: 10.3390/nu10010017
Keywords: urea, ammonium chloride, rumen fermentation, nutrient digestion, antioxidant capacity
Citation: Zheng W, Duan H, Cao L, Mao S and Shen J (2024) Acid-base properties of non-protein nitrogen affect nutrients intake, rumen fermentation and antioxidant capacity of fattening Hu sheep. Front. Vet. Sci. 11:1381871. doi: 10.3389/fvets.2024.1381871
Received: 04 February 2024; Accepted: 14 March 2024;
Published: 26 March 2024.
Edited by:
Yangchun Cao, Northwest A&F University, ChinaReviewed by:
Chong Wang, Zhejiang Agriculture and Forestry University, ChinaCopyright © 2024 Zheng, Duan, Cao, Mao and Shen. This is an open-access article distributed under the terms of the Creative Commons Attribution License (CC BY). The use, distribution or reproduction in other forums is permitted, provided the original author(s) and the copyright owner(s) are credited and that the original publication in this journal is cited, in accordance with accepted academic practice. No use, distribution or reproduction is permitted which does not comply with these terms.
*Correspondence: Junshi Shen, c2hlbmp1bnNoaUBuamF1LmVkdS5jbg==
Disclaimer: All claims expressed in this article are solely those of the authors and do not necessarily represent those of their affiliated organizations, or those of the publisher, the editors and the reviewers. Any product that may be evaluated in this article or claim that may be made by its manufacturer is not guaranteed or endorsed by the publisher.
Research integrity at Frontiers
Learn more about the work of our research integrity team to safeguard the quality of each article we publish.