- 1College of Animal Science and Technology, Hebei Normal University of Science & Technology, Qinhuangdao, China
- 2College of Animal Science and Technology, Jilin Agricultural University, Changchun, China
- 3Zhejiang Institute of Freshwater Fisheries, Huzhou, China
Introdction: Aeromonas veronii is a significant pathogen to various aquatic life. Infections in fish can lead to high mortality rates, causing substantial economic losses in aquaculture. Vaccination is proposed as a substitute for antibiotics in aquaculture to decrease disease-related mortality and morbidity. Our study previously constructed a hisJ-deleted strain of A. veronii, which provided protective effect to Loach.
Methods: To further assess the vaccine’s applicability, this study evaluated its genetic stability and safety, and the immune protective effects in Carassius auratus through four distinct administration routes: intraperitoneal injection, intramuscular injection, oral administration, and immersion, to determine the efficacy of these administration routes.
Results: The results showed that the vaccine remained genetically stable after 45 generations. Immunization via these administration routes was safe for Carassius auratus, with intraperitoneal and intramuscular injections causing stronger adverse reactions. Immersion immunization resulted in mild adverse reactions, and no significant adverse reactions were observed following oral immunization. Immunizing Carassius auratus at safe concentrations via these routes enhanced the phagocytic activity in serum, increased the levels of non-specific immune-related enzymes (ACP, AKP, C3, C4, LZM, SOD, and IgM), and improved specific serum antibody levels. It also elevated levels of cytokines related to inflammatory responses (IL-1β, IL-10, TNF-α, TGF-β) in organ tissues (liver, spleen, kidney, mid-post intestine, and gills). The survival rates of Carassius auratus were measured after challenging with the virulent strain A. veronii TH0426, resulting in the relative survival rates of 64% for Intraperitoneal vaccine group, 56% for Intramuscular vaccine group, 52% for oral vaccine group, and 48% for immersion vaccine group. Analysis of bacterial load in the liver, spleen, and kidney post-challenge showed a decreasing trend in the control group, indicating that the vaccine strain ΔhisJ could gradually restrict the rapid proliferation of bacteria in these tissues, thereby providing a certain level of immune protection against A. veronii.
Discussion: In brief, the vaccine strain ΔhisJ can serve as a safe live attenuated vaccine for Carassius auratus, and this study lays the foundation for the development of live attenuated vaccines against Aeromonas veronii.
1 Introduction
Carassius auratus, a term encompassing varieties like Goldfish, Fangzheng Carassius auratus, Pengze Carassius auratus, and Polyploid Carassius auratus, is a species with a wide range of varieties (1, 2). It is a historically significant freshwater cultured fish in China and East Asia. Due to its delicious taste and high economic value, it is favored by aquaculturists and has a huge market demand (3). With the widespread popularization of intensive farming, bacterial diseases in Carassius auratus have become frequent, among which Aeromonas is the most common bacterial pathogen. Aeromonas is widely distributed in freshwater and sewage environments and can also be isolated from food, such as meat, aquatic products, and milk, posing certain hazards to humans and aquatic animals (4–6). Aeromonas veronii (A.veronii), one of the main pathogens in the Aeromonas genus, can infect many species of freshwater and marine fish, including Carassius auratus, Tilapia, Catfish, Largemouth bass, Loach, Salmon, and Turbot, as well as Crustaceans and Mollusks (7–9). Clinical symptoms of infection mainly include septicemia, gill rot, enteritis, skin ulcers, and visceral infections. In aquaculture, A.veronii infection can lead to high mortality rates, causing significant economic losses.
Antibiotic treatment is the preferred method to control bacterial infections, providing good preventive and therapeutic effects, but also brings many challenges, such as antibiotic residues and emergence of multi drug-resistant strains, which are passed to humans through drinking water and food, posing serious threats to human health. Vaccine immunization is another important measure to prevent and control bacterial infections. In aquaculture, vaccines mainly include attenuated live vaccines, inactivated vaccines, subunit vaccines, DNA and mRNA vaccines (10–12). Among them, the live attenuated vaccines can simulate the natural infection process, stimulating innate immunity, cellular immunity, and specific immunity, and have been proven to provide more effective and lasting immune protection. Using genetic engineering technology to knock out the virulence genes is a popular method for preparing live attenuated vaccines. Researchers prepared a live attenuated A.veronii vaccine by suppressing the expression of SmpB, to immunize Zebrafish, and achieved a survival rate of 65% after challenge with a virulent strain (13). A tolC gene-deleted live attenuated vaccine significantly reduced tissue damage in Hyriopsis cumingii infected by a virulent strain (14). An hcp gene-deleted attenuated vaccine immunized the largemouth bass, achieving a 100% survival rate, significantly higher than the 76.67% survival rate of the formalin inactivated vaccine immunization group (15). Our research group prepared a live attenuated vaccine by knocking out the hisJ gene, which enabled a survival rate of 65.66% in the Loach against the virulent strain (16). Despite these advancements, numerous challenges persist. The safety of the live attenuated vaccines raises concerns, particularly regarding their potential reversion to virulence or adverse effects in immunocompromised individuals. And, the precise duration of immunity these vaccines confer remains elusive, prompting inquiries into the necessity for booster shots and sustained protection for vaccinated groups (13–15, 17). Therefore, while A.veronii vaccine development is a promising strategy for infection control, it necessitates ongoing research to address these prevailing issues.
The immunization pathway is an important factor determining the vaccine’s immune protective effect. The administration routes of fish vaccine include injection, oral, and immersion. Injection immunization includes muscle injection and intraperitoneal injection (14, 18). The site for muscle injection is generally the muscle at the base of the dorsal fin or tail fin of the fish, while the intraperitoneal injection site is often inside the peritoneum at the base of the ventral fin. The injection route produces strong immune protection and long-lasting immunity, but the injection process is time-consuming, labor-intensive, and highly stressful for the fish. The oral immunization route involves mixing the vaccine with feed and administering it orally when the fish feed, saving a lot of manpower and avoiding the stress and irreversible damage caused to the fish during the injection process. However, the oral immunization method still requires fishing operation, uses a large amount of vaccine, and vaccine components are easily degraded by gastrointestinal proteases, losing immunogenicity, resulting in a relatively poor immune protection effect (19). Immersion immunization involves immersing fish in water containing the vaccine for a period, which requires less manpower, allows immunization during transport, causes minimal fish injury, and can even avoid fishing during immunization. However, immersion immunization consumes a large amount of vaccine and often provides low protection and short immunity duration (20).
Our research group’s earlier construction of an hisJ-deleted vaccine of A.veronii (namely ΔhisJ) achieved good immune protection in the Loach (16, 21). Genetic stability, virulence, and immune protective efficacy are key indicators for evaluating live attenuated vaccines. To analyze the immunological effects of different administration routes on Carassius auratus and further evaluate its potential as a live attenuated vaccine, this study utilized passage experiments to assess the genetic stability of the live attenuated vaccine ΔhisJ. The safety evaluating results of the vaccine showed that intraperitoneal and intramuscular injection of the vaccine caused inflammatory reactions at the injection site, while oral and immersion immunization routes did not result in significant stress response. The relative survival rates were obtained as 64% in the IpV group, 56% in the ImV group, 52% in the OV group, and 48% in the IV group. The results laid a foundation for the development of a live attenuated vaccine ΔhisJ.
2 Materials and methods
2.1 Rearing of Carassius auratus
The experimental Carassius auratus were purchased from a fish farm in Changchun, with each fish weighing an average of 50 ± 5 g. After disinfection of the fish surfaces through immersion in potassium permanganate and saline solution, they were temporarily housed in aquarium tanks in the Aquaculture Laboratory of Jilin Agricultural University. Consistent oxygen supply and temperature were maintained in the tanks, with one-third of the water being changed every 2 ds. Once the fish had stable growth and adapted to the water environment, they were randomly assigned to groups for bacterial infection and immunity experiments.
2.2 Strains and cultural methods
In earlier studies, a live attenuated vaccine strain, designated as ΔhisJ, was developed by knocking out the hisJ gene in the wild type A.veronii TH0426 (GenBank accession no. CP012504.1) (21).
The wild-type A.veronii TH0426 (which are Ampicillin-resistant) and the attenuated vaccine strain ΔhisJ, preserved in the laboratory, were streaked onto RS agar plates (Hopebiol, Qingdao, China) and incubated at 30°C for 12 h. Single colonies were selected and inoculated into liquid LB medium (containing 0.1% Ampicillin), then cultured at 30°C with agitation at 180 rpm for 12 h. The bacterial culture was subsequently centrifuged at 6000 rpm for 5 min; the supernatant was discarded, and the pellet was washed three times with PBS and resuspended in the same volume. Finally, 25% glycerol was added, and the mixture was thoroughly mixed before being stored at −80°C in an ultra-low-temperature refrigerator. The next day, a portion of the frozen bacterial culture was retrieved and counted using a serial dilution technique. The process involves diluting a bacteria sample several times to break the sample into manageable concentration levels. After each dilution, a portion of the sample may be plated onto a LB agar plate, and after incubation, the colonies are counted. This step was crucial as it preceded the quantitative determination of the average colony-forming units per milliliter (CFU/mL), a critical measure determining the bacterial concentration used for subsequent challenge and vaccinations.
Employing the method outlined above, Staphylococcus aureus CMCC(B)26,003 was cultivated and enumerated, with the culture conditions maintained at a temperature of 37°C. The bacterial culture was then adjusted to a concentration of 1 × 107 CFU/mL, suitable for subsequent phagocytosis assays.
2.3 Verification of the genetic stability of the vaccine strain ΔhisJ
The vaccine strain ΔhisJ is being tested for genetic stability over multiple generations of growth in LB medium, using PCR amplification. The strain ΔhisJ was continuously passaged for 45 generations in LB liquid medium containing 0.1% Ampicillin, with genome extraction every 5 generations. At the same time, PCR identification was performed using the primers hisJ-for/hisJ-rev (Table 1). The target bands were sent to Kumei (Changchun) Biotech Co., Ltd. for sequencing. The purpose of the experiment is to confirm that the mutant remains consistent over many generations, ensuring the strain’s stability for any application in vaccine development.
2.4 Determination of the median lethal dose (LD50) of the Carassius auratus
To determine the optimal immune dosage for immunizing Carassius auratus and confronting them with a bacterial challenge, we employed various administration routes for the wild-type strain A. veronii TH0426 and the live attenuated vaccine ΔhisJ, including intraperitoneal and muscular injections, along with immersion and oral routes. A total of 120 Carassius auratus, evenly divided into 8 groups, were immunized with different types and doses of vaccines. Over a period of 14 ds, meticulous daily monitoring was conducted to record the mortality rates of Carassius auratus. Subsequently, these data were utilized to calculate the LD50 for the respective bacterial strains employing the modified Karber method. The calculation formula is as follows:
LD50 = log-1[Xm − i(p − 0.5)], note: i is the dose interval (i.e., the difference between the logarithmic doses of two adjacent dose groups); Xm is the logarithm of the maximum dose; p is the mortality rate of each dose group (mortality rates are all expressed in decimals); p is the sum of the mortality rates of each dose group.
Different types of vaccines are prepared according to the methods described below. Both strains, TH0426 and ΔhisJ, were initially cultured in LB medium before being washed and resuspended in PBS. TH0426 was administered through intraperitoneal injection, each injection consisting of 200 μL of bacterial solution at concentrations ranging from 2 × 108 to 2 × 104 CFU/mL. In contrast, ΔhisJ was injected either intraperitoneally or into the muscle near the dorsal fin, with each injection being 200 μL and concentrations varying from 2 × 109 to 2 × 105 CFU/mL. During the immersion tests, the TH0426 and ΔhisJ culture was added to the aquarium, with the fish being immersed for 30 min in solutions containing bacterial concentrations ranging from 2 × 108 to 2 × 104 CFU/mL, and from 2 × 109 to 2 × 105 CFU/mL. Following this treatment, the fish were relocated to a bacteria-free aquarium for subsequent rearing. For the oral tests, the concentration of ΔhisJ was adjusted to levels ranging from 2 × 108 to 2 × 104 CFU/mL, and from 2 × 109 to 2 × 105 CFU/mL, respectively. Every 200 μL of this bacterial solution was combined with 100 g of fish feed (supplied by TONGWEI Co., LTD., China). Additionally, to ensure the bacteria were adequately encapsulated, 3 g of sodium alginate powder (sourced from Sigma-Aldrich, United States) was used at a 1.5% ratio during the preparation of the fish feed. After the encapsulation process, which involved drying, the feed was refrigerated at 4°C and preserved for future feeding sessions.
2.5 Preparation of different types of vaccines
For vaccines administered by intraperitoneal (IpV group) or intramuscular injection (ImV group), the concentration of the attenuated vaccine strain ΔhisJ is 2 × 107 CFU/mL. For vaccines used in immersion immunization (IV group), the culture of ΔhisJ is added to the aquarium to achieve a concentration of 2 × 107 CFU/mL. For vaccines used in oral immunization (OV group), the concentration of the ΔhisJ is adjusted to 2 × 107 CFU/mL, and for every 200 μL of bacterial solution, it is mixed with 100 g of fish feed.
2.6 Animal safety assessment of vaccine
To assess the safety of the attenuated vaccine strain ΔhisJ, the Carassius auratus were randomly divided into five groups, with 10 fish in each group, for a challenge test. For the IpV group and the ImV group, the dose of the vaccine was 200 μL per fish. For the IV group, the Carassius auratus were first soaked in an aquarium containing the attenuated vaccine for 30 min, and after the immunization, the fish were transferred to a vaccine-free aquarium. For the OV group, fish were fed vaccine-coated feed at a dose of 2% of body weight, continuously for 5 ds, and after the immunization, they were fed non-vaccine-coated feed. After the immunization, continuous observation was carried out for 1 week, and the clinical symptoms and mortality rate of Carassius auratus in each group were recorded. Carassius auratus were intraperitoneally and intramuscular injected with an equal volume of PBS as a control group (namely Control group).
2.7 Vaccine immunization
Carassius auratus were divided into 7 groups, each consisting of 60 fish. In the intraperitoneal vaccine group (IpV group) and the intramuscular vaccine group (ImV group), the fish were subjected to either intraperitoneal injections or dorsal fin muscle injections of the attenuated vaccine strain ΔhisJ. A second dose of the vaccine was administered on the 14th d, following the same procedures. For the immersion vaccination group (IV group), the fish were immersed in an aquarium with a 2 × 107 CFU/mL concentration of the vaccine. After soaking for 30 min, they were transferred to a vaccine-free aquarium. A second round of immersion vaccination was conducted 14 ds later. In the oral vaccine group (OV group), each fish received vaccine-coated feed amounting to 2% of its body weight. This method of oral immunization continued for 5 ds, followed by another 5 ds session starting on the 14th day. After immunization, the fish were given feed not coated with the vaccine.
For both the intraperitoneal PBS group (IpP group) and the intramuscular PBS group (ImP group), the Carassius auratus were injected with 0.2 mL of PBS per fish, either intraperitoneally or in the dorsal fin muscle, with a follow-up injection on the 14th d. Furthermore, a Blank Control group (BC group) was established in which the fish did not receive any form of vaccine immunization or PBS injections.
2.8 Sample collection
For serum samples, blood was drawn from the caudal vein of 10 randomly chosen healthy Carassius auratus on the 0, 7th, 14th, 21st and 28th d post immunization. The collection was performed post-anesthesia. Half of the obtained blood was treated with a sodium heparin solution (heparin sodium 1,000 IU/mg in 0.85% NaCl) to serve as an anticoagulant, intended for subsequent leukocyte phagocytic activity analysis. The remaining blood was subjected to centrifugation at 3000 rpm for 15 min to separate the supernatant, earmarked for evaluating various serum immune indices and antibody levels.
As for tissue samples, after the blood collection, the same fish were dissected to harvest specific organs and tissues, including the liver, kidneys, spleen, intestines, and gills. These specimens were promptly stored in a −80°C freezer, preserving them for future RNA extraction processes and cytokine assays.
2.9 Detection of non-specific immune indicators in serum
According to the manufacturer’s instructions, we utilized ELISA kits from the Nanjing Jiancheng Bioengineering Institute, Jiangsu, China, to analyze the enzyme activities of acid phosphatase (ACP), alkaline phosphatase (AKP), superoxide dismutase (SOD), and lysozyme (LZM). One Ginoff unit of AKP and ACP activity corresponds to 1 mg of phenol produced in 100 mL of serum reacting with the substrate at 37°C for 30 min, with the absorbance measured at 520 nm. One unit of SOD activity refers to the amount of enzyme that results in a 50% inhibition rate of SOD in the reaction system, measured by the absorbance at 450 nm. One unit of LZM activity was defined as the amount of lysozyme that causes a 0.001 unit reduction in absorbance per minute at 530 nm. All analyses were conducted in triplicate.
Based on the instruction manuals of the fish IgM ELISA kit (Nanjing Jiancheng Bioengineering Institute, Jiangsu, China), and the fish complement proteins C3 and C4 ELISA kits (Yan Domain Chemical Reagent Co., Ltd., Shanghai, China), the levels of IgM antibodies, C3 and C4 in serum were measured using a double-antibody sandwich ELISA method. The coating micropores provided in these kits were pre-coated with mouse anti-carp IgM, complement C3, and complement C4 antibodies. Standards, serum samples, and horseradish peroxidase (HRP)-labeled detection antibodies were added sequentially to the wells of the ELISA plate, followed by incubation and washing. Subsequently, tetramethylbenzidine (TMB) substrate solution was added for color development, and the reaction was stopped with H2SO4. The optical density (OD) was measured using a spectrophotometer at a wavelength of 450 nm. This value positively correlated with the corresponding antibodies in the sample. The concentration of complement C3 and C4 in the samples was calculated using a standard curve. All analyses were conducted in triplicate.
2.10 Serum specific antibody detection
Using a 96-well V-bottom plate to determine the agglutination titer of serum, the level of specific antibodies is evaluated. The specific procedure is as follows:
A.veronii TH0426 is cultured until it reaches the logarithmic phase. After centrifugation, it is washed three times with PBS, and the bacterial suspension’s concentration is adjusted to 1 × 107 CFU/mL. The bacteria are then inactivated by heating at 56°C for 30 min, serving as the inactivated bacterial antigen for the test. Take a 96-well plate and add 80 μL of saline solution to the first well, and 50 μL of saline solution to the remaining wells. Then add 20 μL of serum to the first well. Mix it evenly, then transfer 50 μL from this well to the second well. Using the same method, continue the process until the penultimate seventh well, discarding 50 μL each time. This creates a series of dilutions of the serum antibodies in the wells at 1:4, 1:8, 1:16, 1:32, 1:64, 1:128, 1:256, and 1:512 concentrations. The eighth well functions as a control and does not contain any serum. Distribute 50 μL of the deactivated bacterial antigen into every well, ensuring thorough mixing. Subsequently, allow the plate to incubate at 37°C for a duration of 2 h, and then let it remain at ambient temperature throughout the night. In the final step, assess the extent of bacterial agglutination through microscopic examination.
2.11 Detection of white blood cell phagocytic activity
Staphylococcus aureus CMCC(B)26,003 was inoculated into TSB medium (Hopebio, Qingdao, Shandong, China) and subsequently washed three times with PBS. The bacterial suspension was then adjusted to a concentration of 1 × 107 CFU/mL, used for phagocytic antigen. We then mixed 0.2 mL of anticoagulated blood with 0.02 mL of the prepared phagocytic antigen. This mixture was incubated in a water bath set at 25°C for 60 min, with gentle agitation every 10 min to ensure uniform consistency. After incubation, the mixture was centrifuged at 4000 rpm for 10 min, resulting in the formation of distinct layers. We carefully pipetted the intermediate layer enriched with white blood cells, depositing 5–10 μL droplets onto a glass slide to create a blood smear. The smear was allowed to air-dry and was then secured for the Wright-Giemsa staining process. We applied the stain to the blood smear for 10 min. Any residual stain was removed with distilled water, and the slide was left to dry completely. For the analysis, the slide was positioned under a microscope. We chose a random field of vision to scrutinize the white blood cells, evaluating their phagocytic activity towards bacteria. This observation was pivotal for determining the Phagocytic Percentage (PP) of white blood cells using the formula Equation 1:
2.12 Extraction of RNA and qRT-PCR analysis of cytokines
RNA was extracted from the liver, kidneys, spleen, intestines, and gills of Carassius auratus following vaccination, according to the instructions of the RNA extraction kit (TaKaRa, Beijing, China). Reverse transcription was carried out using a reverse transcription kit (TaKaRa, Beijing, China) to obtain cDNA. The cDNA obtained from reverse transcription served as a template for qPCR, performed with the TB Green® Premix Ex Taq™ II kit (TaKaRa, Beijing, China). The primer sequences for each cytokine are presented in Table 1. RNA-free water was added for the negative control, and β-actin was used as the internal reference gene. The relative expression levels of each gene were calculated using the 2−ΔΔCT method. All qRT-PCR reactions were set up in triplicate.
2.13 The challenge test by the wild type strain A.veronii TH0426
On the 35th d after the first immunization, 25 fish from each group were randomly selected for the challenge test by intraperitoneal injection of A.veronii TH0426. The immune protective effect in each experimental group was observed. A.veronii TH0426 was revived and, after bacterial counting, the concentration was adjusted to 1.2 × 107 CFU/mL to serve as the challenge bacterial solution. Each Carassius auratus was injected intraperitoneally with a dose of 0.2 mL. The experiment and control groups were observed for 14 ds, and any dead fish were promptly removed and recorded.
The relative percent survival for each group was calculated based on the mortality rates using the formula:
2.14 Determination of bacterial load in tissues after challenge
On the 1st, 3rd, and 5th d following the challenge, liver, spleen, and kidney tissues were collected from moribund fish in each group as samples for testing. The organs were weighed, and then 0.4 g of tissue from each organ was randomly cut and placed into an EP tube containing 4 mL of sterile PBS. After mincing, the tissues were homogenized, and the homogenate was diluted in a 10-fold gradient and then spread onto RS agar plates. Following overnight incubation at 37°C, colony counting was conducted. The bacterial load in the liver, spleen, and kidneys at different time intervals for each experimental group was calculated to assess the immune protection efficacy of the vaccine.
2.15 Statistical analysis
Statistical analyses were conducted using SPSS 26.0 and GraphPad Prism v9.5.0, employing one-way Analysis of Variance (ANOVA) followed by t-tests. Data are presented as mean ± standard error. Significant differences are indicated with an asterisk (*), where *p < 0.05, **p < 0.01, and ***p < 0.001 are considered to represent statistically significant differences in mean values.
3 Results
3.1 Identification of genetic stability in the vaccine strain ΔhisJ
To assess the genetic stability of the hisJ-deleted strain, the ΔhisJ was serially passaged for 45 generations, and PCR amplification was conducted using the primers hisJ-for and hisJ-rev. In the wild type strain A.veronii TH0426, a 2,648 bp target band (including the ORF of hisJ) was amplified. In the ΔhisJ across different generations, a consistent 1874 bp target band was amplified, as shown in Figure 1. This result aligns with the expectations, demonstrating that the hisJ-deleted strain has good genetic stability.
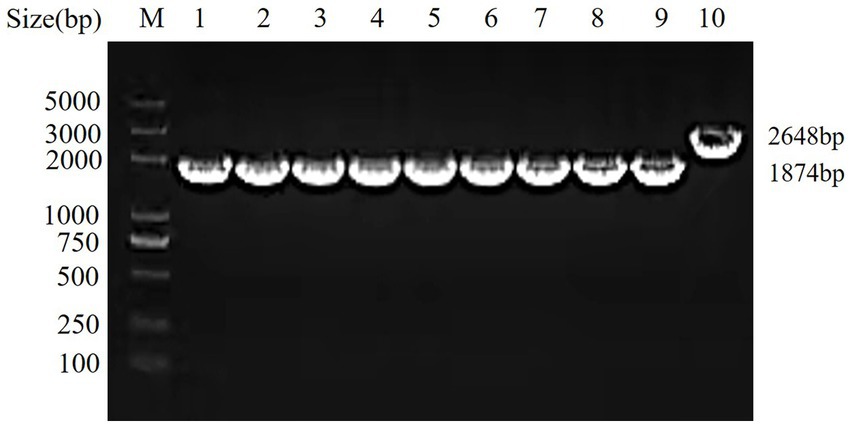
Figure 1. Genetic stability test of the vaccine strain ΔhisJ. M: DL2000 DNA Marker; 1–9: the vaccine strain ΔhisJ: a PCR verification is performed after every five passages; 10: the wild type strain A.veronii TH0426.
3.2 Median lethal dose
To determine the immunization and the challenge dose, the LD50 of A.veronii TH0426 and ΔhisJ. The results (Table 2) showed that under different administration routes, the LD50 of ΔhisJ significantly increased compared to the wild type strain TH0426. Specifically, in the IpV group, the LD50 increased by 357.8 times (2.10 × 108/5.87 × 105), the largest increase, while in the IV group, it increased by 146.9 times (2.16 × 108/1.47 × 105), the smallest increase. Across the four inoculation routes, the highest bacterial concentration at which Carassius auratus did not die when inoculated with ΔhisJ was 2 × 107 CFU/mL. The maximum LD50 for the wild-type strain TH0426 was 3.55 × 106 CFU/tail. It is noteworthy that under the IpV injection route, the LD50 for fish was the smallest, indicating that intraperitoneal injection is the most virulent route for the strain in fish. Therefore, this study chose a dose of 4 LD50 (≈2.4 × 106 CFU/tail) for intraperitoneal injection to conduct immune protection experiments. This involved adjusting the concentration of ΔhisJ to 2 × 107 CFU/mL with an immunization dose of 0.2 mL, and adjusting the concentration of TH0426 to 1.2 × 107 CFU/mL with a virulent dose of 0.2 mL. The clinical symptoms exhibited by Carassius auratus infected with the wild-type strain A.veronii TH0426 and the vaccine ΔhisJ included darkening of the body, slowed swimming, floating to the surface, surface bleeding, abdominal swelling, and in severe cases, skin ulcers.
3.3 Animal safety test
In the groups of Carassius auratus immunized via intraperitoneal vaccination (IpV), intramuscular vaccination (ImV), immersion vaccination (IV), oral vaccination (OV), and intraperitoneal PBS injection (IpP), there were no instances of death following the administration of the vaccine or PBS. Fish in the IpV and ImV, groups exhibited strong stress responses, showing signs of poor mental state, reduced feeding, slow swimming, and floating to the water surface, but they returned to normal the next day. Fish in the IV group showed mild stress responses, with a slight reduction in feeding, but recovered to normal 6 h after immunization. Fish in the OV group did not show any stress responses. Upon dissection of fish from each group, there were no obvious pathological changes observed in the intestines, gills, kidneys, spleen, or liver of any group (Figure 2A). Notably, some fish in the ImV group experienced partial scale loss and even swelling and ulceration at the injection site (Figure 2B).
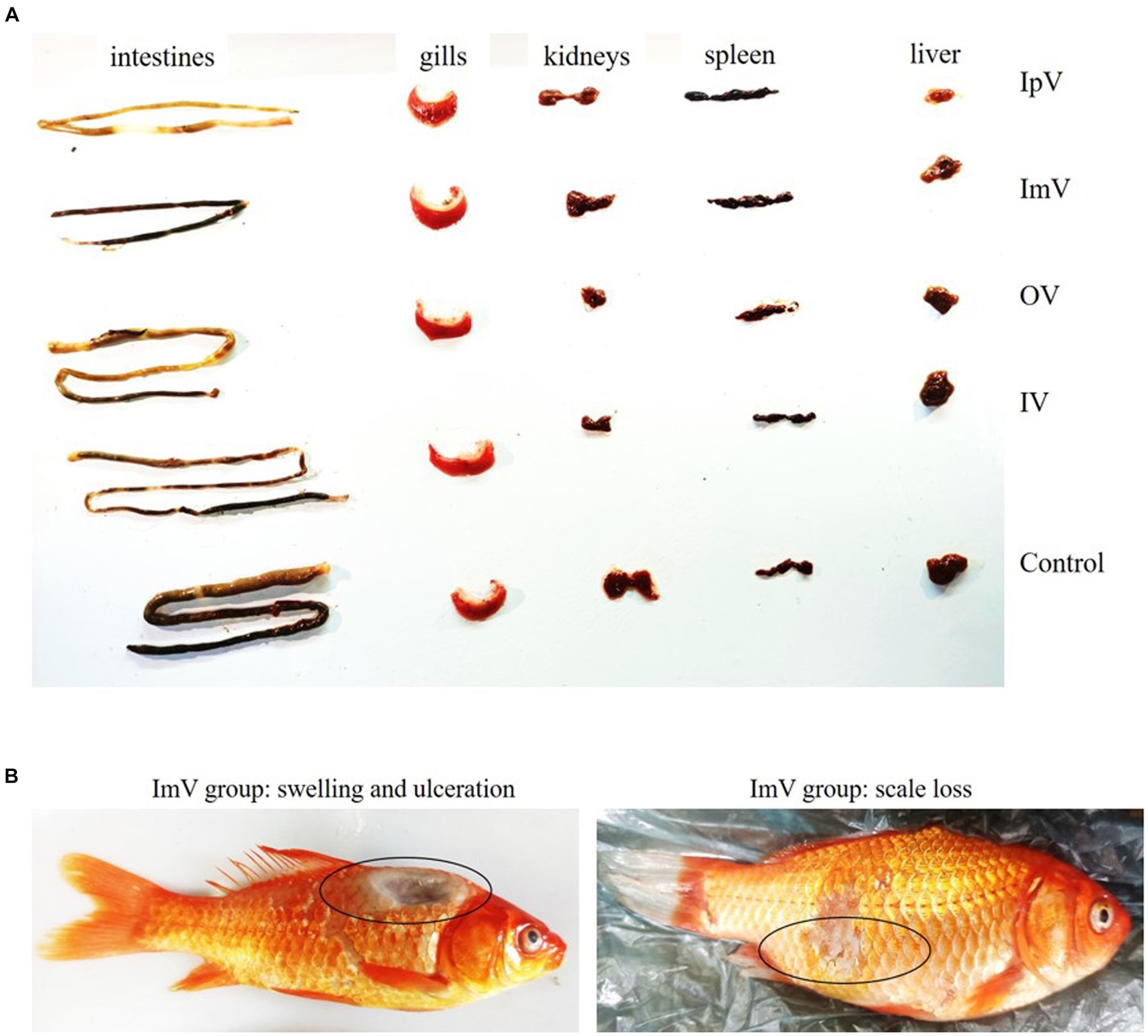
Figure 2. The animal safety evaluation of the vaccine strain ΔhisJ. (A) Organ diagram of Carassius auratus in the immunized and control group. The organs of the fish in each group did not show significant pathological changes. (B) In the ImV group, there was scale loss, swelling, and ulceration at the injection site.
3.4 Nonspecific immune-related analysis in serum
After immunizing Carassius auratus with the vaccine strain ΔhisJ, non-specific immune related molecules in the serum of the Carassius auratus were detected, including IgM, ACP, AKP, LZM; complement C3, complement C4, and SOD. Taking IgM as an example, the results (Figure 3A) show that the IgM content in the serum of Carassius auratus in various vaccine-immunized groups generally showed an upward trend after the primary immunization, and continued to increase after the secondary immunization. The IgM content in the serum of Carassius auratus in the IpV group, OV group, and IV group showed a continuous upward trend from the start of the initial immunization, reaching their respective peaks on the 28th d, then the IgM content began to decline, and by the 35th day after immunization, compared to the control group, the IgM content in the serum of the Carassius auratus in each immunized group was still significantly increased (p < 0.001). The IgM content in the ImV group showed a continuous upward trend from the 0 day of the primary immunization until the 21st d, reaching a peak, and then showed a downward trend. By the 35th d post immunization, compared to the control group, the IgM content in the immunized groups of Carassius auratus significantly increased (p < 0.001). It is worth noting that, on the 28th and 35th d post immunization, the non-specific immune molecules in the IpV group were higher compared to the other three vaccine immunization groups, suggesting that the intraperitoneal injection immunization route stimulates Carassius auratus to produce a more powerful non-specific immune effect. The BC group, IpP group, and ImP group showed no obvious trends in IgM content during each immunization period (p > 0.0.05), and there was little difference in IgM content in the serum between the groups. The trends in the levels of ACP, AKP, LZM, complement C3, complement C4, and SOD in the serum were the same as those of IgM.
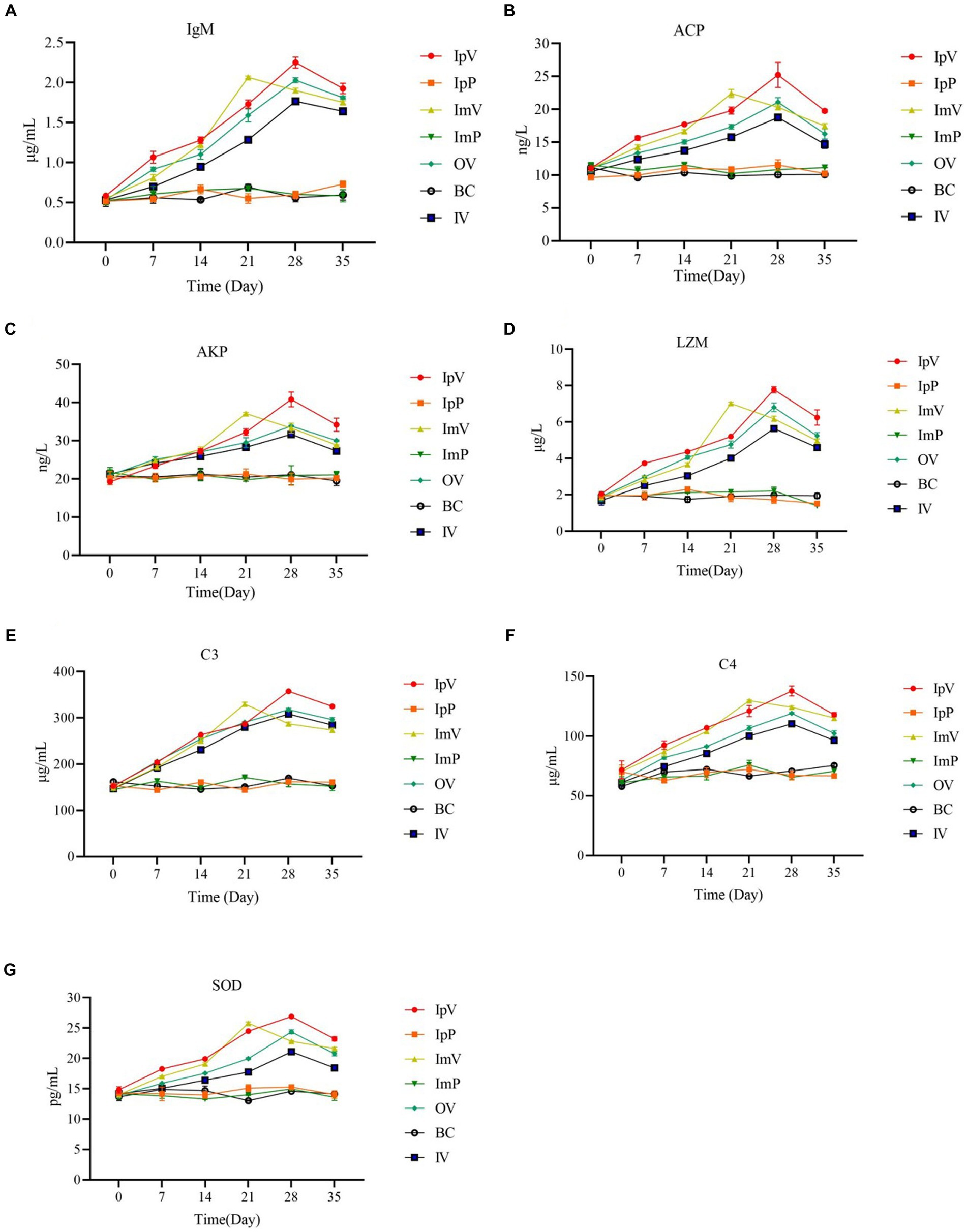
Figure 3. Detection results of the content of IgM (A), ACP (B), AKP (C), LZM (D); complement C3 (E), complement C4 (F), and SOD (G) in serum.
3.5 Detection of the specific antibody against Aeromonas veronii in serum
Using the 96-well hemagglutination assay, the specific antibody titers of Carassius auratus serum immunized via different routes were tested. The results showed (Figure 4) that there were no significant differences in serum titers among the control groups (BC group, ImP group, and IpP group). The specific antibody titers of the Carassius auratus in each group immunized via different routes (IpV group, ImV group, IV and OV groups) were all higher than those of the control groups. Interestingly, the serum titer gradually increased with the increase of immunization times and the extension of time. On the 28th d, the serum antibody titer of the vaccinated Carassius auratus reached its peak and then declined. Among these, the serum antibody titers of the Carassius auratus in the IpV group were always the highest on the 7th, 14th, 21th, and 28th d post immunization. The antibody titers in both the OV and ImV groups rank second in terms of magnitude, with the group receiving immersion immunization displaying the lowest antibody titers. The results indicated that all four vaccine immunization routes could stimulate Carassius auratus to produce specific humoral immunity, but the intraperitoneal injection route of vaccination had the best immunization effect.
3.6 Results of white blood cell phagocytosis activity test
The anticoagulated blood of Carassius auratus post-vaccine immunization was taken and Staphylococcus aureus CMCC(B)26,003 was added for a white blood cell phagocytosis experiment. The phagocytosis of 100 randomly observed white blood cells under a microscope was counted. The image of white blood cells engulfing bacteria is shown in Figure 5A, and the phagocytic ratio is displayed in Figure 5B. On the 0 d, the phagocytic ratio ranged between 10.01–13.73%, with no significant differences among the seven groups including the control group. However, following the vaccination of the vaccine test groups, from the 7th d of immunization, there was a significant upward trend in the phagocytic ratio of each vaccine immunization group, followed by a slight decrease on the 14th d. With the secondary immunization, the white blood cell phagocytic ratio continued to rise, reaching the peak phagocytic percentage for each vaccine test group on the 21st or 28th d of immunization, followed by a declining trend. On the 21st d post immunization, the white blood cell phagocytic percentage of the muscle ImV group increased significantly compared to the ImP group (p < 0.001); on the 28th d, the white blood cell phagocytic percentage of the IpV group increased significantly compared to the IpP group (p < 0.001); on the 28th d, the white blood cell phagocytic percentage of the oral vaccine group (OV) significantly increased compared to the BC group (p < 0.001); the white blood cell phagocytic percentage of the IV group showed an upward trend, but only on the 21st and 28th d post immunization, the phagocytic percentage was significantly different from the BC group (p < 0.05), with no significant differences at other time points. The white blood cell phagocytic ratios of the ImV and IpV groups were higher than those of the IV and OV groups, and the IpV group was higher than the ImV group. These results indicate that the intraperitoneal injection immunization route induced a more intense immune response.
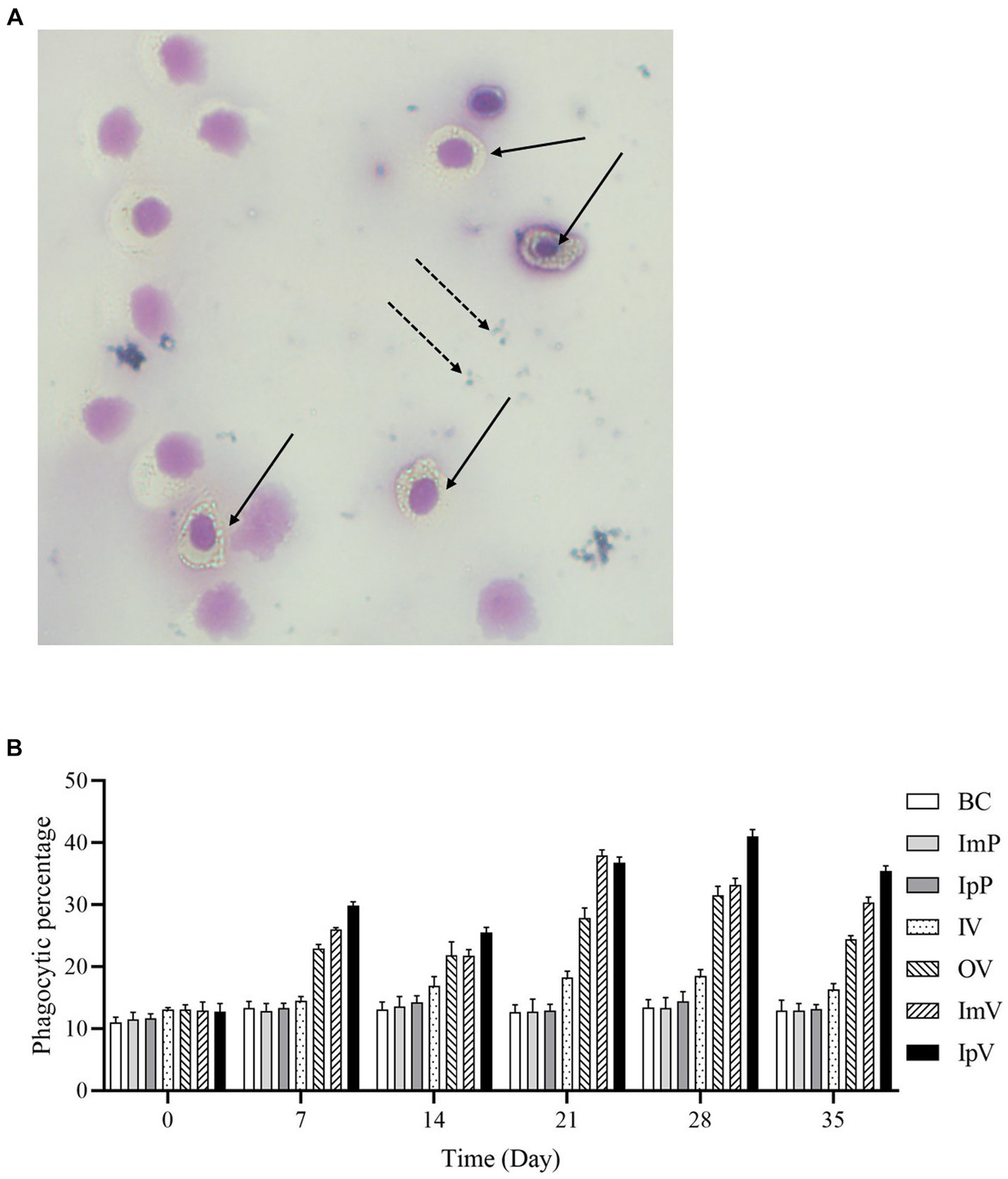
Figure 5. Leukocyte phagocytosis experiment. (A) Microscopic view of leukocyte phagocytosis (×400). Solid arrows indicate leukocytes engulfing S.aureus, while dashed arrows point to S.aureus not engulfed and located outside the cells. (B) Leukocyte phagocytosis ratio.
3.7 Vaccination enhanced the expression of cytokines in different organs of Carassius auratus
Before immunization (on the 0 d), and on the 7th, 14th, 21st, 28th, and 35th d after the first vaccine immunization, Carassius auratus were randomly selected from each group for dissection. The liver, spleen, kidney, intestine, and gills were harvested to extract RNA, and qRT-PCR was performed to analyze the relative expression levels of cytokines IL-1β, TNF-α, IL-10, and TGF-β. The expression levels of the four cytokines in the liver were tested and analyzed. The results showed that (Figure 6), at all the testing days except the 0 d, the expression levels of IL-1β and TNF-α in the OV and IV groups were higher than those in the BC group. The highest levels in the OV, IV, and IpV groups were reached on the 28th d post-immunization, while for the ImV group, the peak was on the 21st d, followed by a decline in expression levels. The expression levels of cytokines in the spleen, kidney, intestine, and gills showed the same trends as those in the liver. Interestingly, the highest expression levels of the four cytokines in different organs of fish in the IpV group (on the 28th d) were higher than those in the other three vaccine immunization groups. The highest expression levels of the four cytokines in the ImV group fish (on the 21st d post-immunization) were higher than those in the OV and IV groups but still lower than those in the IpV group on the 28th d.
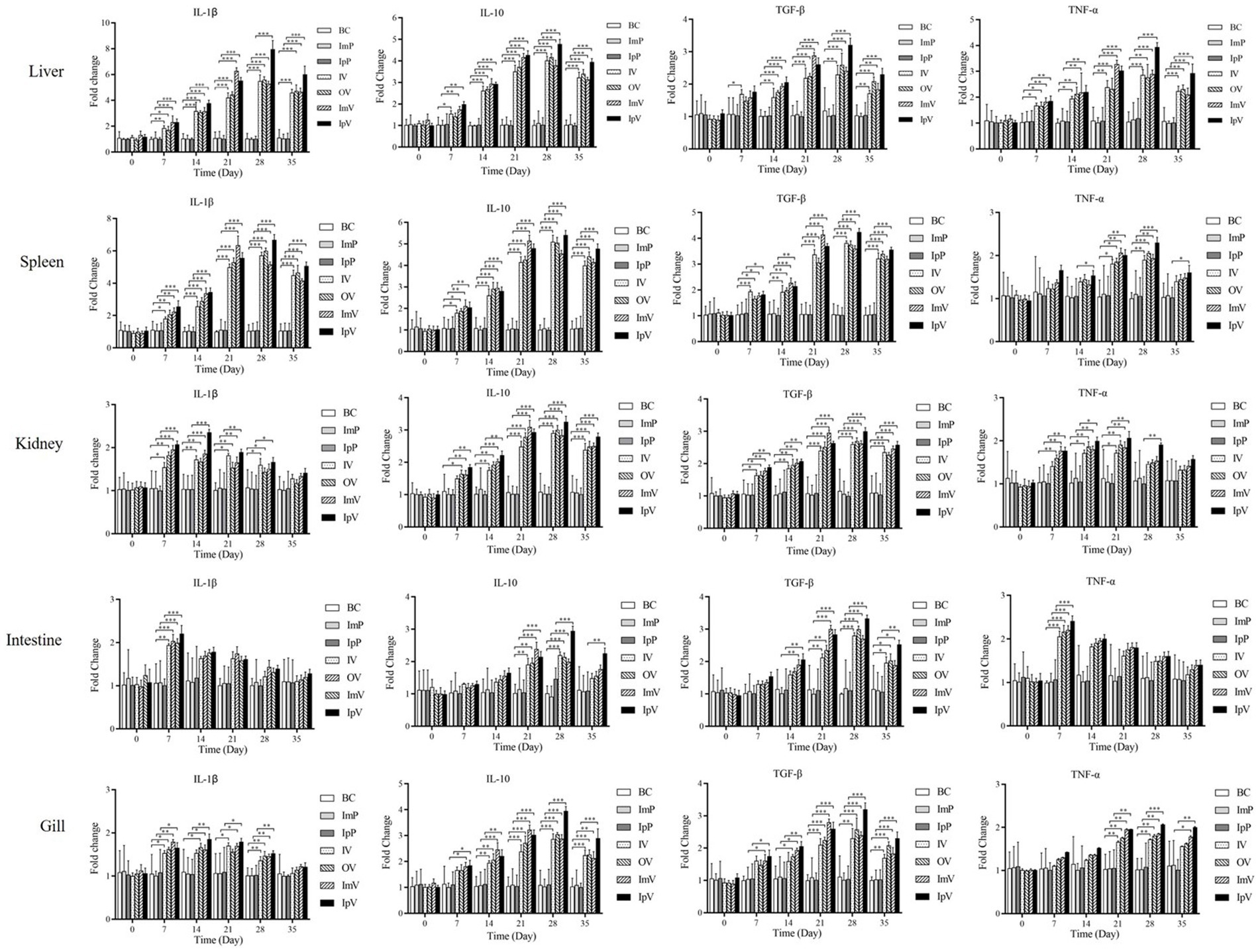
Figure 6. Analysis of cytokine expression in the liver, spleen, kidney, intestine, and gill of Carassius auratus following vaccine immunization.
3.8 Challenge protection test
The Carassius auratus in each group were intraperitoneally injected with the wild strain A.veronii TH0426 on the 35th d after immunization to measure the immune protection effect. The Carassius auratus in the IpP, ImP and BC group all died within 6–7 ds after the challenge, exhibiting typical characteristics such as scale loss, bleeding, and abdominal swelling. Some fish in the various vaccine immunization groups also died within 6–7 ds after the challenge. As shown in Figure 7, On the 14th d after the challenge, the survival rate of the fish was 64% in the IpV group, 56% in the ImV group, 52% in the OV group, and 48% in the IV group. The results indicate that Carassius auratus immunized with the vaccine strain ΔhisJ have a certain protective effect against A.veronii infection. The protection rates vary with different immunization routes. The most effective immunization route is intraperitoneal injection, followed by muscle injection, oral immunization, and soaking immunization, in decreasing order of protection effectiveness.
3.9 Bacterial load in tissues after challenge with the wild strain Aeromonas veronii TH0426
After intraperitoneal injection with the wild strain A.veronii TH0426 in each immunized and control group of Carassius auratus, the liver, spleen, and kidney of dying fish were collected on the 1st, 3th, and 5th d post-challenge to measure the bacterial load in these organs. The results showed that (Figure 8) on the 1st day post-challenge, there was no significant difference in bacterial load compared to the respective control groups. However, on the 3th and 5th d post-challenge, the bacterial load in the organs of fish in the immunized groups was generally lower than that in their respective control groups. Additionally, the bacterial load on the 5th d was lower than on the 3th d, indicating a decreasing trend in bacterial load over time in the immunized groups. In contrast, the bacterial load in the liver, spleen, and kidney of the control group fish showed a trend of increase or maintained a high level without decreasing. Since the control group fish, lacking protective immunity, all died within 7 ds post-challenge, measuring bacterial load in their organ tissues on the 7th d was not feasible; hence, further analysis of the load was not conducted. The results of the bacterial load tests indicate that the vaccine strain ΔhisJ could gradually restrict the rapid proliferation of bacteria in the organ tissues of Carassius auratus, reducing the bacterial load in these tissues, thereby providing a certain level of immune protection against A.veronii. Notably, there were significant differences in the bacterial load in the organs of Carassius auratus immunized via different routes with the vaccine strain ΔhisJ. Fish vaccinated via intraperitoneal injection had the lowest bacterial load in their organs on the 3th and 5th d post-challenge, followed by those in the ImV group, the OV group, and the IV group.
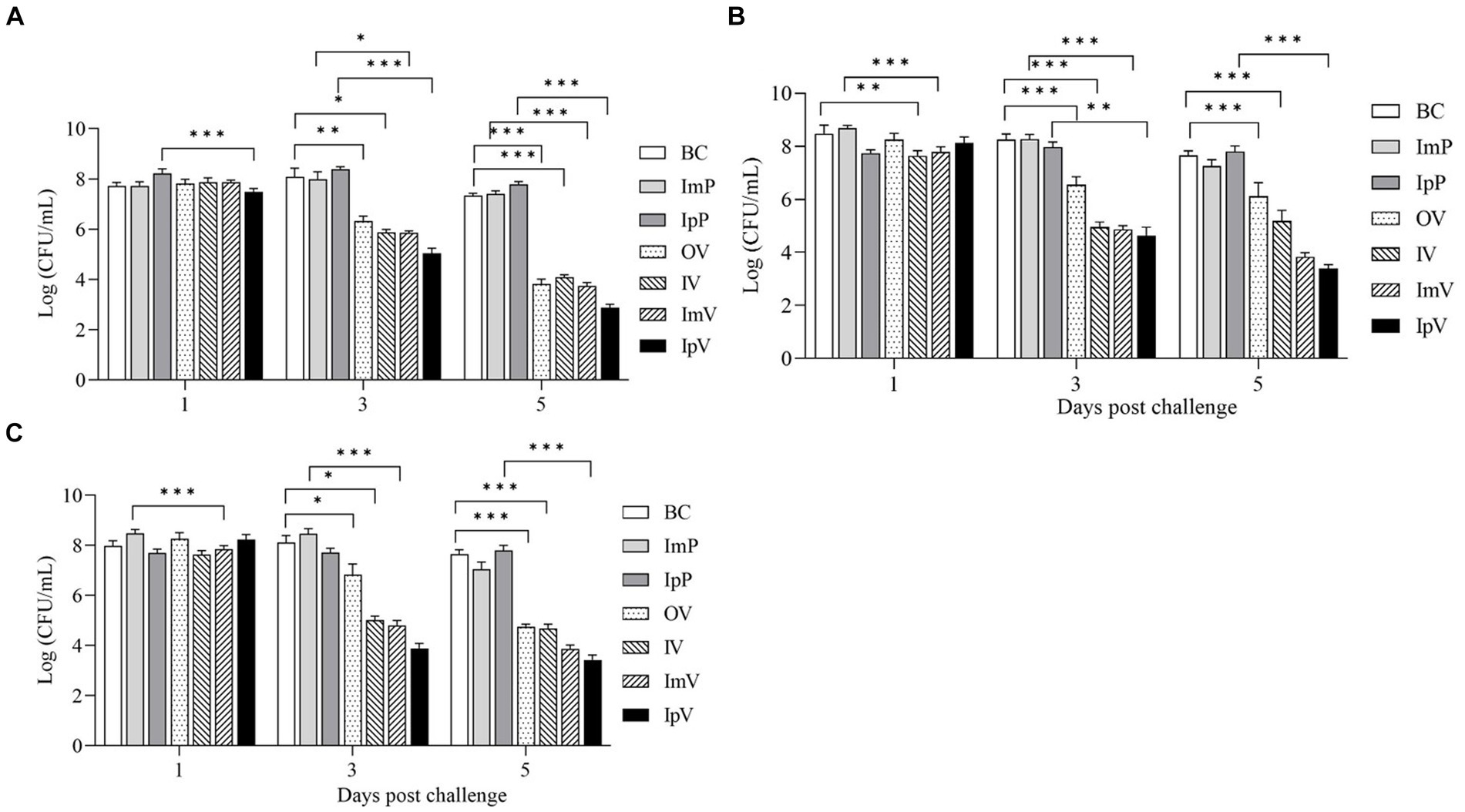
Figure 8. Bacterial load of Carassius auratus organs after challengement. (A) Liver, (B) spleen, and (C) kidney.
4 Discussion
Aeromonas veronii is a significant aquatic animal pathogen that poses a great threat to aquaculture (5, 8). Vaccines are an essential means of preventing infection, and their development is of significant practical importance in aquaculture (22, 23). In China and throughout East Asia, where Carassius auratus farming is extensive, A.veronii infections severely jeopardize the healthful cultivation of these fish, necessitating public attention to vaccine development. In a previous study, a hisJ gene-deleted strain of A.veronii TH0426 (namely ΔhisJ) was constructed and tested for immune protective effects in Loaches, achieving a survival rate of 65.66%, indicating its potential as a live attenuated vaccine (16). However, its efficacy in Carassius auratus remain unclear. Fish vaccines can be administered through injection (intraperitoneal and intramuscular), oral, and immersion routes, each eliciting different immune responses due to the recognition of vaccine antigens by different immune-presenting cells under varying immunization routes. Therefore, it is necessary to study the immune protective effects of ΔhisJ on Carassius auratus using different immunization routes, to evaluate the vaccine’s feasibility in these fish. This study demonstrated good genetic stability of ΔhisJ through passage experiments. Administering the vaccine to Carassius auratus using safe doses by intraperitoneal injection, intramuscular injection, oral immunization, and immersion immunization did not cause severe damage to the fish, indicating good safety of these four immunization methods. Blood immune index tests and challenge protection experiments showed that all four immunization methods provided good immune protection effect, with the effectiveness decreasing in the following order: intraperitoneal injection, intramuscular injection, oral, and immersion vaccination. These results lay an important foundation for the vaccine’s development.
Live attenuated vaccines can provide protection against infection in the host and prevent the spread of pathogens. However, the development of high-quality live attenuated vaccines involves three aspects: genetic stability, low virulence, and effective immune protection. Genetic stability is a critical indicator of vaccine quality (23, 24). To ensure the genetic stability of the BCG vaccine, it is stipulated that no more than 12 generations of seed strains be used in its production (25). Hence, to ensure vaccine quality, it is necessary to study and monitor the vaccine’s stability. Continued passage of vaccine strains, analyzed using techniques such as phenotypic analyses, DNA sequencing, PCR, DNA microarrays, complete genome sequencing, SNP detection, and macrorestriction digest of the genome, can reveal the genetic stability of the vaccine (25, 26). In vitro passage and PCR analysis was conducted in this study, and after 45 generations, the vaccine strain ΔhisJ remained a hisJ-deleted strain, indicating a good genetic stability. It is similarly to findings in vaccine studies of Bordetella pertussis and Salmonella, where the respective vaccines maintained their gene-deleted status after continuous passage, demonstrating good genetic stability.
The second criterion for live attenuated vaccines is to be entirely non-virulent for humans and animals (27, 28). Although previous study about the Loach had demonstrated that the vaccine strain ΔhisJ could establish short-term colonization or transient infection and was quickly cleared by the host, its virulence in Carassius auratus had not been determined. This study found that under the four immunization routes, the LD50 of the vaccine strain ΔhisJ in Carassius auratus was significantly lower than that of the parental strain A.veronii TH0426, and it did not cause severe pathological changes, indicating its safety in Carassius auratus. It’s worth mentioning that fish in the IpV and ImV groups exhibited strong stress reactions.
The live attenuated vaccines developed through genetic engineering techniques must not only ensure the safety of the vaccine strain for humans and animals but also exhibit strong and lifelong immunogenicity (28, 29). They should prevent infection in vital organs, reduce colonization in the host, and be effective against virulent strains. Strains that completely lose virulence are often quickly cleared by the host’s immune system, making it difficult to effectively activate an effective immune response (30). This research evaluated immune protective effects of the vaccine strain ΔhisJ across four immunization routes. The evaluation involved analyzing serum immune indices, white blood cell phagocytic capabilities, and the expression of immune genes in various organs of Carassius auratus. Additionally, the study assessed the survival rate of Carassius auratus during the virulent challenge and monitored changes in bacterial loads across different organs.
Non-specific immunity plays a pivotal role in the primary defense mechanism against diverse pathogenic invasions. In serum, key non-specific immune indicators comprise IgM, ACP, AKP, LZM, complement C3, complement C4, and SOD. This study demonstrated that immunization of Crucian carp through various routes leads to differential increases in these immune indicators (31, 32). Notably, the concentration of immune indicates of IpV group reached peak values on the 28th d after the first immunization, with levels slightly higher than them of other groups, while the concentration of immune indicates of ImV group peaked on the 21st d post-initial immunization, albeit slightly lower than the intraperitoneal group. The concentration of immune indicates of OV and IV groups also had higher levels of these immune indicators compared to the control group. Additionally, using agglutination tests to detect specific antibodies in serum, it was found that antibody titers in all immunization groups peaked on the 28th d after vaccination and then gradually declined. The intraperitoneal vaccination group had higher antibody titers than other groups, with no significant differences between the oral and intramuscular vaccination groups, and the lowest titers in the immersion vaccination group. The white blood cell phagocytosis test, an important indicator for measuring the effectiveness of vaccine immunization, revealed that the phagocytic ratio of white blood cells in all immunized groups continued to rise after the 21st d and peaked, with the concentration of immune indicates of ImV group peaking earlier than them of the others. IL-1β and TNF-α are pro-inflammatory factors in the body, which can limit the spread of pathogens, clear them, and activate the immune system, while IL-10 and TGF-β are anti-inflammatory factors, which can inhibit the excessive production of pro-inflammatory factors and prevent inflammatory damage to the body. This study found that after immunizing Carassius auratus with different immune routes, the expression levels of various cytokines in liver, spleen, kidney, intestine, and gill tissues increased to varying degrees and then declined. In the experiments by Zhang et al., the live attenuated vaccine ΔhisJ immunized Loaches by immersion and intraperitoneal injection, with anti-inflammatory (IL-10 and TGF-β) and pro-inflammatory (IL-1β and TNF-α) factors in the liver and spleen of Loaches peaking and then stabilizing within 28 ds post-immunization, which is not entirely consistent with our findings. In the experiments by Ma et al., a constructed Streptococcus DNA vaccine combined with the polymer PLGA to prepare an oral vaccine for Tilapia showed that the expression levels of pro-inflammatory cytokine genes such as IL-1β and TNF-α in the spleen and kidney tissues of Tilapia increased significantly and then decreased, similarly to the results of the oral vaccine group with Carassius auratus (33). In summary, these results show that using the vaccine strain ΔhisJ and immunizing Carassius auratus through different routes activated both non-specific and specific immune responses, with response intensity from high to low in the order of intraperitoneal injection, intramuscular injection, oral vaccination, and immersion vaccination.
By comparing the survival rates of individuals vaccinated or not vaccinated, the effectiveness of immunization interventions can be directly observed. In this study, Carassius auratus were vaccinated with a live attenuated vaccine through four different immunization routes and then challenged with a virulent strain. The results showed a relative survival rate of 64% in the IpV group, 56% in the ImV group, 52% in the OV group, and 48% in the IV group, indicating that different vaccination routes can provide varying degrees of protection to Carassius auratus. Although the injection immunization routes showed the highest protection rates compared to immersion and oral routes, both intramuscular and intraperitoneal injections caused varying degrees of injury to the fish, potentially leading to inflammation at the injection site and secondary bacterial infections (18, 20, 34). The research indicates that oral and immersion immunization methods are less effective, but they cause relatively less stress and injury to the fish. To address the suboptimal immune protective effect of these methods, various strategies can be employed. For immersion immunization, extending immersion time and increasing the concentration of bacterial suspension could enhance antigen uptake. For oral routes, selecting better vaccine encapsulating materials (such as alginate, chitosan, nano, and microparticles) or optimizing the vaccine preparation process can increase vaccine encapsulation rates and prolong the release of the vaccine in the gut, thereby enhancing the fish’s immune response. This study also measured the bacterial load in various organs of Carassius auratus post-challenge, finding that different immunization routes significantly reduced bacterial load in organs like the liver, spleen, and kidneys. The results suggest that the vaccine can limit the rapid proliferation of bacteria in the tissues of Carassius auratus, potentially helping to alleviate bacterial enteritis and septicemia. This also explains why the vaccinated Carassius auratus gradually stop dying after 7 ds post-immunization.
In conclusion, this study demonstrates that the vaccine ΔhisJ can be used as a live attenuated vaccine for Carassius auratus, with good genetic stability and safety. Vaccination through different routes can enhance the immune level of Carassius auratus and provide certain protection against A.veronii infection. It is particularly noteworthy that, although the immune protective effect of intraperitoneal and intramuscular injection routes is superior to oral and immersion immunization, the injection routes may cause injury to the fish, with oral immunization routes offering better protection than immersion. This research lays a significant foundation for the development of live attenuated vaccines of A.veronii, but more attention needs to be paid to issues such as genetic variation in live attenuated vaccines. Nevertheless, this study possesses inherent limitations. A more extensive scale of experimental animals is essential to thoroughly assess vaccine safety and eliminate possible toxic effects. Moreover, evaluating vaccine efficacy must entail quantifying the duration of immune protection, given its critical role as an efficacy indicator. Addressing these issues is pivotal for advancing the vaccine’s development and application in Carassius auratus.
5 Conclusion
The hisJ gene-deleted strain of A.veronii, as a live attenuated vaccine, is safe for Carassius auratus when administered via intramuscular injection, intraperitoneal injection, oral, and immersion methods. Immunizing Carassius auratus through different routes can enhance the phagocytic activity of phagocytes in their serum, increase the activity of non-specific immune-related enzymes, and raise the level of specific serum antibodies and cytokines in the fish. The survival rate of Carassius auratus after being challenged with a virulent strain shows variation depending on the route of vaccination with the vaccine strain ΔhisJ. Furthermore, post-vaccination, the spread of A.veronii in the organ tissues can be restricted.
Data availability statement
The datasets presented in this study can be found in online repositories. The names of the repository/repositories and accession number(s) can be found in the article/supplementary material.
Ethics statement
Experimental Animal Regulation Ordinances defined by Hebei Provincial Department of Science and Technology (HPDST2020-17) and Regulations for Animal Experimentation at Jilin Agricultural University (JLAU08201409). The study was conducted in accordance with the local legislation and institutional requirements.
Author contributions
TW: Conceptualization, Investigation, Methodology, Writing – original draft, Writing – review & editing. RM: Data curation, Methodology, Project administration, Software, Writing – review & editing. XP: Conceptualization, Investigation, Writing – original draft, Writing – review & editing. FW: Data curation, Formal analysis, Methodology, Writing – original draft. ZZ: Formal analysis, Project administration, Validation, Writing – review & editing. QS: Funding acquisition, Project administration, Resources, Writing – review & editing. XS: Conceptualization, Funding acquisition, Investigation, Resources, Writing – original draft, Writing – review & editing. GG: Funding acquisition, Project administration, Writing – review & editing.
Funding
The author(s) declare that financial support was received for the research, authorship, and/or publication of this article. This study was supported by Hebei Province Major Science and Technology Project (V1586484692073) and Jilin Provincial Department of Science and Technology Project (20210202049NC).
Conflict of interest
The authors declare that the research was conducted in the absence of any commercial or financial relationships that could be construed as a potential conflict of interest.
Publisher’s note
All claims expressed in this article are solely those of the authors and do not necessarily represent those of their affiliated organizations, or those of the publisher, the editors and the reviewers. Any product that may be evaluated in this article, or claim that may be made by its manufacturer, is not guaranteed or endorsed by the publisher.
References
1. Li, J, Yan, Y, Yue, N, Luo, Q, Li, W, and Xie, X. Comparative study of metal accumulation in three fish species (Silurus asotus, Cyprinus carpio, and Carassius auratus auratus) from the Jinsha and Tuo Rivers located upstream of the Yangtze River, China. Bull Environ Contam Toxicol. (2018) 101:26–32. doi: 10.1007/s00128-018-2375-8
2. Chen, W, Zhao, Y, and Yang, C. Genetic variation and population history of three Carassius auratus populations in Huaihe River, China. Mitochondrial DNA A DNA Mapp Seq Anal. (2016) 27:4252–5. doi: 10.3109/19401736.2015.1043543
3. Luo, Q, Zha, G, Lin, L, Huang, Y, and Zou, X. Comparison of physicochemical properties of different tissues from China climbing perch Anabas testudineus and crucian carp Carassius auratus. Food Sci Nutr. (2022) 10:936–44. doi: 10.1002/fsn3.2727
4. Praveen, PK, Debnath, C, Shekhar, S, Dalai, N, and Ganguly, S. Incidence of Aeromonas spp. infection in fish and chicken meat and its related public health hazards: a review. Vet World. (2016) 9:6–11. doi: 10.14202/vetworld.2016.6-11
5. Janda, JM, and Abbott, SL. The genus Aeromonas: taxonomy, pathogenicity, and infection. Clin Microbiol Rev. (2010) 23:35–73. doi: 10.1128/CMR.00039-09
6. Abernathy, JW, Zhang, D, Liles, MR, Lange, MD, Shoemaker, CA, and Beck, BH. Whole-genome sequencing and annotation of Aeromonas veronii isolates from channel catfish. Microbiol Resour Announc. (2023) 12:e123122:e0123122. doi: 10.1128/mra.01231-22
7. Guo, K, Sun, Y, Tang, X, Zhou, X, Jiang, M, Yang, Q, et al. Pathogenicity and inactivated vaccine treatment of Aeromonas veronii JW-4 on crucian carp. Microb Pathog. (2023) 183:106315. doi: 10.1016/j.micpath.2023.106315
8. Li, T, Raza, S, Yang, B, Sun, Y, Wang, G, Sun, W, et al. Aeromonas veronii infection in commercial freshwater fish: a potential threat to public health. Animals. (2020) 10:608. doi: 10.3390/ani10040608
9. Das, R, Sarma, K, Hazarika, G, Choudhury, H, and Sarma, D. Identification and characterisation of emerging fish pathogens Aeromonas veronii and Aeromonas hydrophila isolated from naturally infected Channa punctata. Antonie Van Leeuwenhoek. (2023) 117:4. doi: 10.1007/s10482-023-01896-z
10. Liu, G, Li, J, Jiang, Z, Zhu, X, Gao, X, Jiang, Q, et al. Pathogenicity of Aeromonas veronii causing mass mortalities of Odontobutis potamophila and its induced host immune response. Fish Shellfish Immunol. (2022) 125:180–9. doi: 10.1016/j.fsi.2022.05.009
11. Youssef, HA, Ayoub, HF, Soror, EI, and Matter, AF. Virulence genes contributing to Aeromonas veronii pathogenicity in Nile tilapia (Oreochromis niloticus): approaching the development of live and inactivated vaccines. Aquac Int. (2023) 31:1253–67. doi: 10.1007/s10499-022-01023-1
12. Saichuer, P, Khrisanapant, P, Senapin, S, Rattanarojpong, T, Somsoros, W, Khunrae, P, et al. Evaluate the potential use of TonB-dependent receptor protein as a subunit vaccine against Aeromonas veronii infection in Nile tilapia (Oreochromis niloticus). Protein Expr Purif. (2024) 215:106412. doi: 10.1016/j.pep.2023.106412
13. Liu, P, Huang, D, Hu, X, Tang, Y, Ma, X, Yan, R, et al. Targeting inhibition of SmpB by peptide aptamer attenuates the virulence to protect zebrafish against Aeromonas veronii infection. Front Microbiol. (2017) 8:1766. doi: 10.3389/fmicb.2017.01766
14. Zheng, S, Tang, X, Yang, Q, Zhou, X, Li, Y, and Wu, Z. Aeromonas veronii tolC modulates its virulence and the immune response of freshwater pearl mussels, Hyriopsis cumingii. Dev Comp Immunol. (2024) 153:105137. doi: 10.1016/j.dci.2024.105137
15. Chi, Y, Jiao, H, Ran, J, Xiong, C, Wei, J, and Ozdemir, E. Construction and efficacy of Aeromonas veronii mutant Deltahcp as a live attenuated vaccine for the largemouth bass (Micropterus salmoides). Fish Shellfish Immunol. (2023) 136:108694. doi: 10.1016/j.fsi.2023.108694
16. Zhang, HP, Chen, MY, Xu, YX, Xu, GY, Chen, JR, Wang, YM, et al. An effective live attenuated vaccine against Aeromonas veronii infection in the loach (Misgurnus anguillicaudatus). Fish Shellfish Immunol. (2020) 104:269–78. doi: 10.1016/j.fsi.2020.05.027
17. Suarun, P, Kitiyodom, S, Yostawornkul, J, Chaiin, P, Yata, T, Rodkhum, C, et al. Chitosan-polymer based nanovaccine as promising immersion vaccine against Aeromonas veronii challenge in red tilapia (Oreochromis sp.). Fish Shellfish Immunol. (2022) 129:30–5. doi: 10.1016/j.fsi.2022.08.035
18. Adams, A . Progress, challenges and opportunities in fish vaccine development. Fish Shellfish Immunol. (2019) 90:210–4. doi: 10.1016/j.fsi.2019.04.066
19. Zhang, W, Zhu, C, Xiao, F, Liu, X, Xie, A, Chen, F, et al. pH-controlled release of antigens using mesoporous silica nanoparticles delivery system for developing a fish oral vaccine. Front Immunol. (2021) 12:644396. doi: 10.3389/fimmu.2021.644396
20. Zhao, Z, Zhang, C, Jia, YJ, Qiu, DK, Lin, Q, Li, NQ, et al. Immersion vaccination of mandarin fish Siniperca chuatsi against infectious spleen and kidney necrosis virus with a SWCNTs-based subunit vaccine. Fish Shellfish Immunol. (2019) 92:133–40. doi: 10.1016/j.fsi.2019.06.001
21. Zhang, HP, Kang, YH, Kong, LC, Ju, AQ, Wang, YM, Muhammad, I, et al. Functional analysis of hisJ in Aeromonas veronii reveals a key role in virulence. Ann N Y Acad Sci. (2020) 1465:146–60. doi: 10.1111/nyas.14265
22. Irshath, AA, Rajan, AP, Vimal, S, Prabhakaran, VS, and Ganesan, R. Bacterial pathogenesis in various fish diseases: recent advances and specific challenges in vaccine development. Vaccines. (2023) 11:470. doi: 10.3390/vaccines11020470
23. Kiseleva, I, Dubrovina, I, Fedorova, E, Larionova, N, Isakova-Sivak, I, Bazhenova, E, et al. Genetic stability of live attenuated vaccines against potentially pandemic influenza viruses. Vaccine. (2015) 33:7008–14. doi: 10.1016/j.vaccine.2015.09.050
24. Xu, Y, Zhang, J, Cui, S, Li, M, Zhang, Y, Xue, H, et al. Genetic stability of vaccine strains by multilocus sequence typing and pulsed-field gel electrophoresis analysis: implications for quality control of the leptospiral vaccine. Hum Vaccin Immunother. (2015) 11:1272–6. doi: 10.1080/21645515.2015.1020266
25. Seki, M, Udagawa, T, Sugawara, I, Iwama, K, Honda, I, Fujita, I, et al. The effect of passages during Japanese BCG vaccine production on genetic stability and protective efficacy. Vaccine. (2012) 30:1460–4. doi: 10.1016/j.vaccine.2011.12.101
26. Garcia-Yoldi, D, Le Fleche, P, Marin, CM, De Miguel, MJ, Munoz, PM, Vergnaud, G, et al. Assessment of genetic stability of Brucella melitensis rev 1 vaccine strain by multiple-locus variable-number tandem repeat analysis. Vaccine. (2007) 25:2858–62. doi: 10.1016/j.vaccine.2006.09.063
27. Wang, X, Zhang, X, Zhou, D, and Yang, R. Live-attenuated Yersinia pestis vaccines. Expert Rev Vaccines. (2013) 12:677–86. doi: 10.1586/erv.13.42
28. Wichmann, O, Vannice, K, Asturias, EJ, de Albuquerque, LE, Longini, I, Lopez, AL, et al. Live-attenuated tetravalent dengue vaccines: the needs and challenges of post-licensure evaluation of vaccine safety and effectiveness. Vaccine. (2017) 35:5535–42. doi: 10.1016/j.vaccine.2017.08.066
29. Kumaresan, V, Alam, S, Zhang, Y, and Zhang, G. The feasibility of using Coxiella burnetii Avirulent nine mile phase II viable bacteria as a live attenuated vaccine against Q fever. Front Immunol. (2021) 12:754690. doi: 10.3389/fimmu.2021.754690
30. Kamble, NM, Nandre, RM, and Lee, JH. Inhibition of Salmonella-induced apoptosis as a marker of the protective efficacy of virulence gene-deleted live attenuated vaccine. Vet Immunol Immunopathol. (2016) 169:96–101. doi: 10.1016/j.vetimm.2015.11.002
31. Bilal, S, Lie, KK, Karlsen, OA, and Hordvik, I. Characterization of IgM in Norwegian cleaner fish (lumpfish and wrasses). Fish Shellfish Immunol. (2016) 59:9–17. doi: 10.1016/j.fsi.2016.09.063
32. Mai, W, Liu, P, Chen, H, and Zhou, Y. Cloning and immune characterization of the c-type lysozyme gene in red-spotted grouper, Epinephelus akaara. Fish Shellfish Immunol. (2014) 36:305–14. doi: 10.1016/j.fsi.2013.11.002
33. Wang, J, Zou, LL, and Li, AX. Construction of a Streptococcus iniae sortase a mutant and evaluation of its potential as an attenuated modified live vaccine in Nile tilapia (Oreochromis niloticus). Fish Shellfish Immunol. (2014) 40:392–8. doi: 10.1016/j.fsi.2014.07.028
34. Nayak, SK . Current prospects and challenges in fish vaccine development in India with special reference to Aeromonas hydrophila vaccine. Fish Shellfish Immunol. (2020) 100:283–99. doi: 10.1016/j.fsi.2020.01.064
35. Elorza, A, Rodriguez-Lago, I, Martinez, P, Hidalgo, A, Aguirre, U, and Cabriada, JL. Gastrointestinal infection with Aeromonas: incidence and relationship to inflammatory bowel disease. Gastroenterol Hepatol. (2020) 43:614–9. doi: 10.1016/j.gastrohep.2020.04.014
36. Esteve-Gassent, MD, Fouz, B, and Amaro, C. Efficacy of a bivalent vaccine against eel diseases caused by Vibrio vulnificus after its administration by four different routes. Fish Shellfish Immunol. (2004) 16:93–105. doi: 10.1016/S1050-4648(03)00036-6
Keywords: Aeromonas veronii, hisJ, vaccine, Carassius auratus, immune route
Citation: Wu T, Ma R, Pan X, Wang F, Zhang Z, Shi Q, Shan X and Gao G (2024) Comparison of the efficacy of Aeromonas veronii ΔhisJ vaccine in Carassius auratus via different immunization routes. Front. Vet. Sci. 11:1378448. doi: 10.3389/fvets.2024.1378448
Edited by:
Yuanhuan Kang, Shandong University, ChinaReviewed by:
Jinxin Xie, Xinjiang Agricultural University, ChinaPeng Xiaowei, China Institute of Veterinary Drug Control, China
Xu Jian, Chinese Academy of Agricultural Sciences (CAAS), China
Copyright © 2024 Wu, Ma, Pan, Wang, Zhang, Shi, Shan and Gao. This is an open-access article distributed under the terms of the Creative Commons Attribution License (CC BY). The use, distribution or reproduction in other forums is permitted, provided the original author(s) and the copyright owner(s) are credited and that the original publication in this journal is cited, in accordance with accepted academic practice. No use, distribution or reproduction is permitted which does not comply with these terms.
*Correspondence: Tonglei Wu, NTMyOTY2OTUyQDE2My5jb20=; Qiumei Shi, c2hpcWl1bWVpQDEyNi5jb20=; Xiaofeng Shan, c3hmMTk5N0AxNjMuY29t