- Department of Veterinary Sciences, Faculty of Agrobiology, Food, and Natural Resources, Czech University of Life Sciences Prague, Prague, Czechia
Hydrogen sulfide (H2S) is a gaseous signaling molecule produced in the body by three enzymes: cystathionine-β-synthase (CBS), cystathionine-γ-lyase (CSE) and 3-mercaptopyruvate sulfurtransferase (3-MST). H2S is crucial in various physiological processes associated with female mammalian reproduction. These include estrus cycle, oocyte maturation, oocyte aging, ovulation, embryo transport and early embryo development, the development of the placenta and fetal membranes, pregnancy, and the initiation of labor. Despite the confirmed presence of H2S-producing enzymes in all female reproductive tissues, as described in this review, the exact mechanisms of H2S action in these tissues remain in most cases unclear. Therefore, this review aims to summarize the knowledge about the presence and effects of H2S in these tissues and outline possible signaling pathways that mediate these effects. Understanding these pathways may lead to the development of new therapeutic strategies in the field of women’s health and perinatal medicine.
1 Introduction
Several decades ago, hydrogen sulfide was considered only as a toxic gas. However, after the discovery of endogenous production of nitric oxide (NO) (1) and carbon monoxide (CO) (2) in the organism and their effects on various tissues, a third endogenously produced gasotransmitter, hydrogen sulfide (H2S), was demonstrated (3). H2S is now known to be involved in a wide range of physiological processes, including reducing cellular oxidative stress, regulating the cell cycle and apoptosis, participating in inflammatory processes, and vasodilating blood vessels (4). The regulation of the nervous and reproductive systems are among the other described functions of H2S (4, 5).
Three enzymes are responsible for the endogenous production of H2S, namely cystathionine-β-synthase (CBS), cystathionine-γ-lyase (CSE) and 3-mercaptopyruvate sulfurtransferase (3-MST). In addition to these, cysteine aminotransferase (CAT) is sometimes mentioned as the fourth H2S-producing enzyme (Figure 1) (6, 7). The main substrate for the enzymatic production of H2S is L-cysteine (8) (Figure 1), although physiologically, H2S can also be generated from D-cysteine (9). However, H2S can also be produced through non-enzymatic processes, such as its production by microorganisms in the digestive tract that metabolize sulfur or the simple dissociation of sodium hydrosulfide (NaHS) into H2S. H2S can also be released from acid-labile sulfur, which serves as a reservoir of this molecule in the body (10, 11).
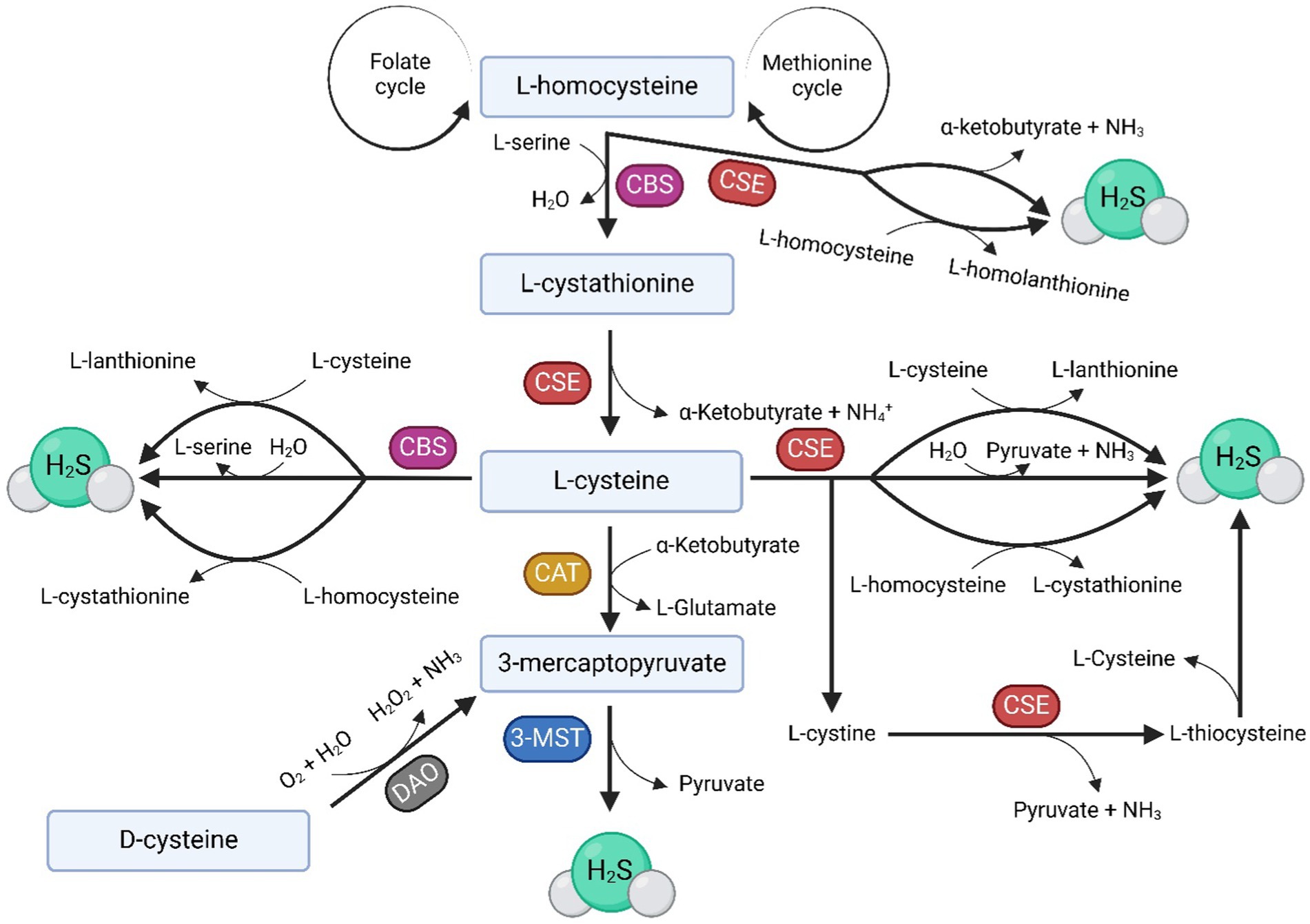
Figure 1. Anabolic pathways of H2S. H2S is enzymatically produced in the body by three enzymes: CBS, CSE, and 3-MST, which also requires CAT for H2S production. The main substrate for H2S formation is L-cysteine, which can, under the influence of H2S-producing enzymes, be produced from homocysteine (Hcy) supplied by the folate and methionine cycles. The image illustrates various pathways involved in the endogenous production of H2S in the body under the influence of CBS, CSE, and 3-MST, as well as the byproducts of these biochemical reactions.
One of the many organ systems affected by H2S is the reproductive tract. H2S has been detected in both male and female reproductive tracts of mammals, fish, and amphibians. In the male reproductive tract, one of the most fundamental roles of H2S is the facilitation of erection (12, 13). In the female reproductive system, H2S has been detected in oocytes (14), follicular cells at all stages (15), the uterus (16), and the placenta (17). In female reproduction, H2S is essential during gravidity and labor initiation (17, 18), in oocyte maturation and ovulation (19). It also influences the vasodilation of uterine and placental vessels, thereby affecting the nutrition of the growing embryo/fetus, in whose epigenetic regulation H2S also participates (20, 21). H2S production also occurs in the vagina and clitoral smooth muscle, where it supports smooth muscle relaxation, vaginal lubrication, and epithelial ion transport (22).
2 Molecular targets of H2S
The effects of H2S on various molecular targets are summarized in Figure 2.The first confirmed target of H2S was cytochrome c oxidase in mitochondria. In high H2S concentrations, mitochondrial activity can be inhibited, and thus adenosine triphosphate (ATP) production is prevented. However, in lower concentrations, H2S can supply electrons to the mitochondrial respiratory chain through sulfide quinone oxidoreductase and cytochrome c oxidase (23, 24). In mitochondria, there has been detected the H2S-producing enzyme – 3-MST (25, 26). H2S is associated with cellular oxidative stress, as it interacts with glutathione, leading to an elevation in its concentration and the subsequent suppression of oxidative stress in mitochondria (27, 28).
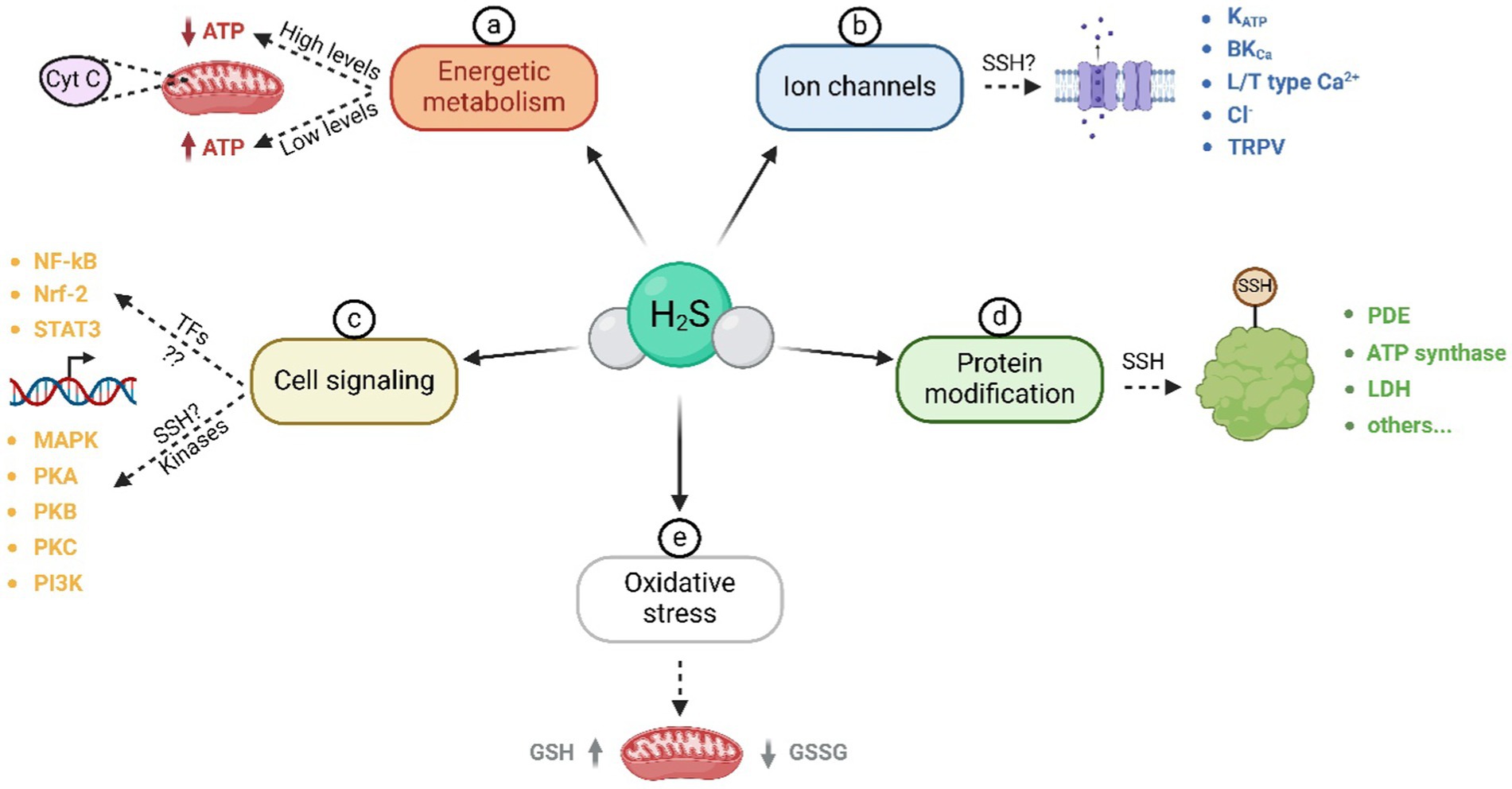
Figure 2. Molecular targets of H2S. These targets can be divided into the following groups: a) Influence of H2S on energy metabolism and cytochrome c oxidase activity; b) activation and inactivation of various types of ion channels, likely through S-sulfhydration; c) influence on cell signaling through transcription factors and kinases; d) modification of a wide range of proteins through S-sulfhydration of cysteine thiol sites; e) reduction of oxidative stress in mitochondria. ATP – adenosine triphosphate; SSH – S-sulfhydration; NF-κB – nuclear factor-kappa-B; Nrf-2 – nuclear factor E2-related factor 2; STAT3 – signal transducer and activator of transcription 3; MAPK – mitogen-activated protein kinase; PKA – protein kinase A; PKB – protein kinase B; PKC – protein kinase C; PI3K – phosphoinositide 3-kinase; PDE – phosphodiesterase; LDH – lactate dehydrogenase; GSH – glutathione; GSSG – glutathione disulfide.
Transcription factors are other H2S intracellular targets during inflammatory processes, as well as during embryonic development. H2S donors such as NaHS, S-diclofenac, or diallyl sulfide can inhibit nuclear factor kappa B (NF-κB) activation, thereby suppressing the production of pro-inflammatory cytokines (29). Conversely, under certain conditions (dose, exposure time), H2S may have pro-inflammatory effects in NF-κB in/dependent manner (30). Both results point to the influence of H2S on inflammatory processes and its tissue specificity. H2S likely impacts other transcription-mediated processes, such as proliferation (31) or angiogenesis (32), and it appears to play a crucial role in the epigenetic regulation of genes in early embryos (33).
A variety of kinases are also cellular targets of H2S. Examples are mitogen-activated protein kinases (MAPK), which H2S can both activate (19, 34) through S-sulfhydration (35) and inhibit (36, 37). H2S also activates protein kinase A (PKA) (38, 39), phosphoinositide 3-kinase (PI3K)/protein kinase B (PKB) (40–42) or protein kinase C (PKC) (41). Additionally, to targeting kinases, H2S also inhibits phosphodiesterase, and consequently regulates the levels of cyclic guanosine monophosphate (cGMP) (43, 44).
Molecular targets of H2S that should be noticed are cellular proteins themselves. An important effect of H2S is the S-sulfhydration of proteins. This process involves the delivery of a sulfur atom derived from the H2S molecule to the thiol group of cysteine residues, leading to the formation of a hydropersulfide group (-SSH) (45, 46). These -SSH cysteines are more reactive than cysteines containing only a thiol group, and S-sulfhydration modifies these proteins (46). Interestingly, in cells, S-sulfhydration is considered a common post-translational modification. Among S-sulfhydrated proteins belong ATP synthase, lactate dehydrogenase, ion channels, phosphodiesterase, and many others (23, 44, 45, 47). In ion channels, H2S is capable of opening ATP-sensitive potassium channels (KATP) in the smooth muscle of arteries (48), myocytes (49), and smooth muscle of the intestine (50) or eye (51). However, H2S also regulates other channels such as large-conductance calcium-activated potassium ion channels (BKCa) (52), L-type and T-type Ca2+ channels (53, 54), Cl− channels (55), and transient receptor potential vanilloid and ankyrin channels (TRPV and TRPA) (56, 57). S-sulfhydration can activate some channels while inhibiting others. Among activated channels belong KATP (58, 59), Cl− (55), TRPV/TRPA (56, 57), T-type Ca2+ (60) and BKCa channels (52, 61). Inhibited ion channels via S-sulfhydration are L-type Ca2+ (45, 53, 62), T-type Ca2+ (54) and BKCa channels (63).
3 Detection of H2S-producing enzymes and the role of H2S in female reproductive tissues
Over the last two decades, H2S-producing enzymes have been detected in various female reproductive tract tissues, spanning different animal models, including humans. Table 1 provide summary of the experiments conducted on this topic across diverse animal species and describe the potential significance of H2S-producing enzymes in these tissues.
Among the initial experiments investigating the function of H2S in the female reproductive system, knockout studies (CBS-KO; CSE-KO) have been described (64–67). These studies demonstrate the importance of CBS in the maintenance of placental and uterine weight in females, as well as its indispensability in the maturation of growing follicles (64, 65) (Figure 3). Furthermore, the effect of CBS on the regularity and length of the estrus cycle was proven, which subsequently affects the fertility rate in females (64, 68). However, the absence of CBS does not cause morphological abnormalities on ovulated oocytes or the ovaries themselves (64). Interestingly, after transplanting CBS-KO ovaries into healthy recipients, the fertility of the females was not affected, indicating that the H2S production through CBS in other reproductive tissues is sufficient but probably not essential for maintaining female fertility (64). As for CSE, the absence of this H2S-producing enzyme in mice appears to have significantly less effect on the incidence of fertility-related defects, as CSE-KO females were fertile, and their pregnancies progressed normally (65, 67, 69). Recent research focused on the fertility of CSE-KO mice showed that CSE-KO leads to a reduced number of successful pregnancies and a higher pro-inflammatory status of fetuses. This suggests that CBS is not the sole key enzyme in H2S production in the context of female reproduction (70).
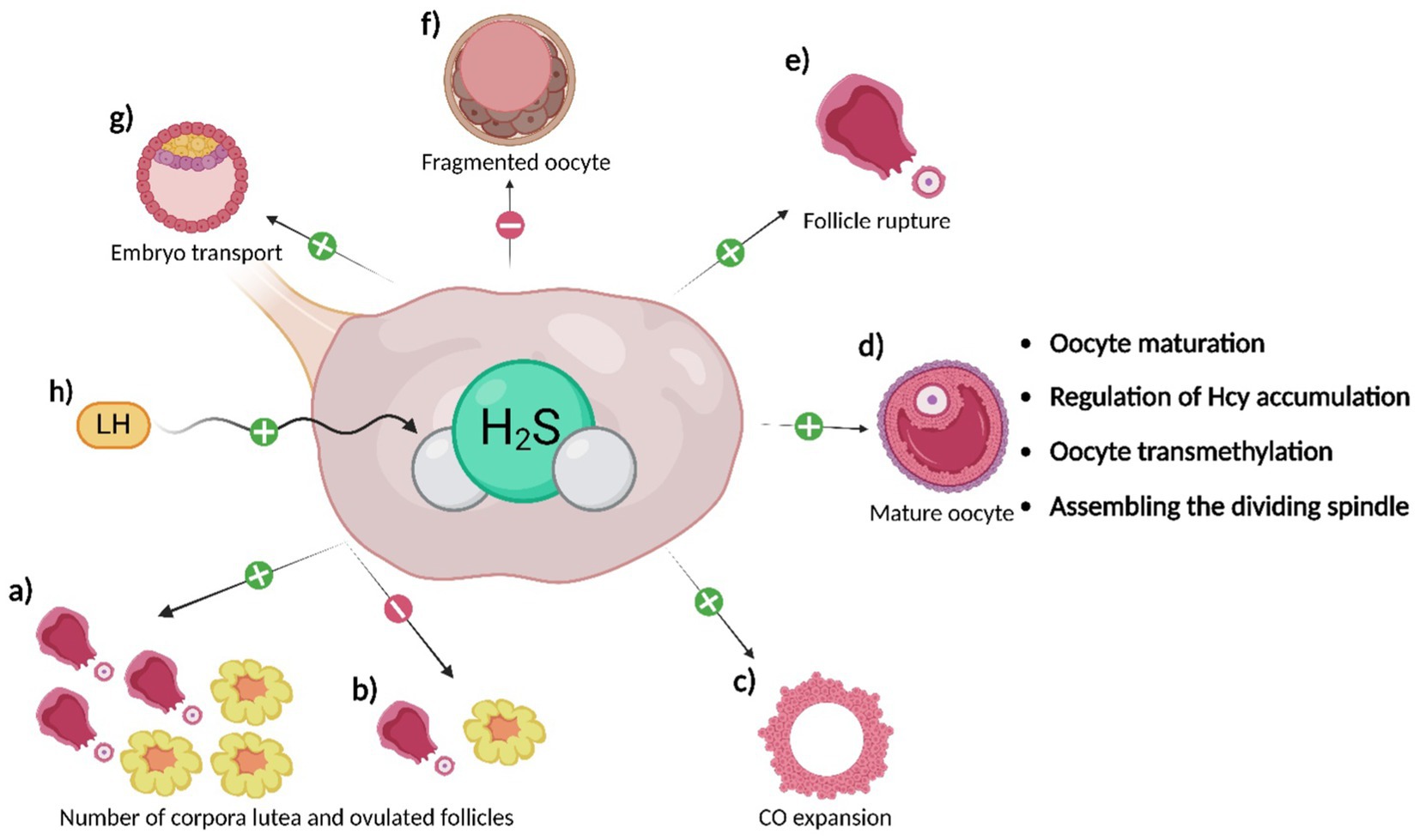
Figure 3. Effects of H2S on oocytes. H2S produced in the ovaries and surrounding reproductive tissues has the following effects: a) H2S promotes ovulation of a more significant number of follicles and the development of a greater number of corpora lutea compared to inhibiting its production (b); c) H2S supports CO expansion; d) H2S promotes oocyte maturation through the regulation of oocyte signaling pathways. Additionally, its production regulates Hcy levels, supports oocyte transmethylation, and ensures proper spindle assembly; e) H2S promotes follicle rupture and, therefore, oocyte ovulation itself; f) H2S delays oocyte aging and reduces the number of fragmented oocytes; its inhibition, on the other hand, leads to an increase in the number of fragmented oocytes; g) H2S supports embryo transport to the uterus; h) LH stimulates H2S production in granulosa cells of follicles, likely through CSE, contributing to the processes mentioned above.
The reason why CBS seems more important for female reproduction in most studies (64, 65, 67) when the final product of both enzymes is H2S, has yet to be investigated. Potential reasons may include variances in homocysteine (Hcy) and cysteine metabolic pathways or differences in the substrate essential for the H2S formation. CBS utilizes Hcy or L-cysteine for H2S production (71, 72), with L-cysteine also generated from Hcy by both CBS and CSE (Figure 1). In reproductive tissues, the prevalence of Hcy may favor CBS (18, 73). CSE primarily uses cystathionine/L-cysteine/cystine as a substrate, but it can also utilize Hcy (74–77). However, the direct production of H2S from Hcy by CSE suggests a potential advantage in following the CBS route, interrupting the reaction at the intermediate product, cystathionine, to regulate both Hcy and H2S levels in the body (Figure 1). This proposition is supported by the fact that hyperhomocysteinemia is a critical factor during pregnancy leading, for example, to preeclampsia, miscarriages, uterine artery blood flow resistance or congenital malformations (73, 76, 78). Furthermore, higher H2S levels can lead to the inhibition of cytochrome c oxidase in mitochondria (24, 79). It is possible that CBS was evolutionarily favored because it can effectively regulate both Hcy levels in tissues and the H2S levels. However, further experiments are necessary to understand CBS’s role in female reproduction precisely.
3.1 The role of H2S in oocytes
The influence of H2S on oocyte maturation (19, 80, 81), ovulation (15, 82), and embryo transport to the uterus (83) has been studied in mice and human oocytes, particularly in connection with luteinizing hormone (LH), which increases CSE production in granulosa cells (82). Inhibition of CSE leads to a reduced number of ovulating follicles and corpus luteum and a higher number of unovulated follicles with retained oocytes (64, 65, 82). LH likely stimulates H2S production in granulosa cells in the preovulatory period (82). Furthermore, the regulation of H2S through a donor increased the levels of proteins essential for cumulus-oophorus (CO) expansion and follicle rupture (82, 84, 85). These results highlight the connection between the hormonal regulation of female reproduction and H2S production (68).
Regarding the role of H2S in oocyte maturation, it has been hypothesized that CBS acts as a mediator between the oocyte and granulosa cells and it may contribute to the proper flow of Hcy in follicular cells, and subsequently support the stability of oocyte transmethylation (15, 80). H2S plays a role during oocyte maturation in the intracellular environment of the oocyte as well. H2S regulates signalling pathways during the cell cycle, likely through S-sulfhydration (35). As was mentioned above, H2S-mediated regulation has been described in the cAMP-PKA, PI3K-PKB, MAPK and maturation promoting factor (MPF) pathways (43, 46, 72, 84). Using H2S donor (Na2S), the supporting effect of the H2S on oocyte maturation has been proven, as the Na2S accelerated the porcine oocyte nuclear maturation and increased MPF activity during GVBD stage. Moreover, this donor increased the number of zygotes with formed pronuclei after the parthenogenetic activation of porcine oocytes (81, 84, 85). During the germinal vesicle stage (GV), CBS is distributed into the nucleus of oocytes, however, from germinal vesicle breakdown (GVBD) to metaphase II, it is localized around the mitotic spindle, where it is probably essential for acetylation of α-tubulin and proper assembly of the mitotic spindle (Figure 3). Conversely, deletion of the CBS gene leads to meiosis arrest, abnormalities in both the meiotic spindle and chromosome structure and disruption of the kinetochore-microtubule attachment (14). Additionally, H2S is produced by cumulus cells, which likely promotes CO expansion (19, 86). The importance of H2S during oocyte maturation is further supported by the findings of Gelaude et al. (72), who confirmed the effect of H2S on meiosis in amphibian oocytes.
It has been previously described that H2S has anti-aging effects and promotes the longevity, health, and condition of many organ systems, including the fetal membranes, probably through the mammalian target of rapamycin (mTOR) signaling pathway and its downstream factor S6 kinase beta-1 (S6K1) (87–89). For this reason, a series of experiments describing the role of H2S during oocyte aging have been reported. H2S-producing enzymes are active in porcine oocytes, and there is a statistically significant decrease in endogenous H2S production during the first day of aging. Inhibition of H2S-producing enzymes induces signs of aging in oocytes and significantly increases the number of fragmented oocytes (Figure 3)(90). Conversely, an exogenous H2S donor (Na2S) can reverse these manifestations. Cultivation in the presence of the H2S donor can also positively affect subsequent embryonic development after parthenogenetic activation (90). These results were supported by research confirming reduced CBS expression in oocytes and ovaries of old mice (14). The mechanism of H2S action on oocytes involves the regulation of KATP and L-type Ca2+ channels, which play a crucial role during oocyte aging through S-sulfhydration. H2S activates KATP channels, delaying cell death, and conversely inhibits L-type Ca2+ channels, which have the opposite effect on oocytes (45, 53, 62, 91). In conclusion, H2S is crucial in most processes occurring in oocytes (Figure 3) and their immediate environment.
3.2 The role of H2S in uterine tissues
3.2.1 Uterine vessels
Given the vasodilatory effects of H2S (58, 92, 93), this function has been investigated concerning the regulation of blood flow in uterine vessels, which affects the exchange of nutrients and respiratory gases between the mother and the fetus, consequently influencing fetal growth and health (94, 95). It appears that the activity of H2S-producing enzymes and the subsequent effect of uterine blood vessel vasodilation are essential, as elevated levels of Hcy (and thus a probable deficiency in H2S-producing enzymes) lead to uterine artery blood flow resistance (96). Vasodilatory effects of H2S have been confirmed in human (97), sheep (98), and rat (99) uterine arteries, as well as in human umbilical arteries and veins, with this effect occurring primarily during the proliferative phase of the menstrual (estrus) cycle and in gravidity (Figure 4) (20). The mechanism of vasodilation in the vascular system generally occurs through KATP channels (62, 100, 101). The same mechanism is employed in uterine vessels, as was confirmed by subsequent studies describing an increased number of KATP channels in human and sheep smooth muscle cells of uterine arteries during pregnancy (20, 102). However, Li et al. (103) contributed to this topic by elucidating the regulation of BKCa channels by H2S in human uterine arteries, so it is conceivable that multiple types of ion channels contribute to the vasodilation of uterine arteries by H2S.
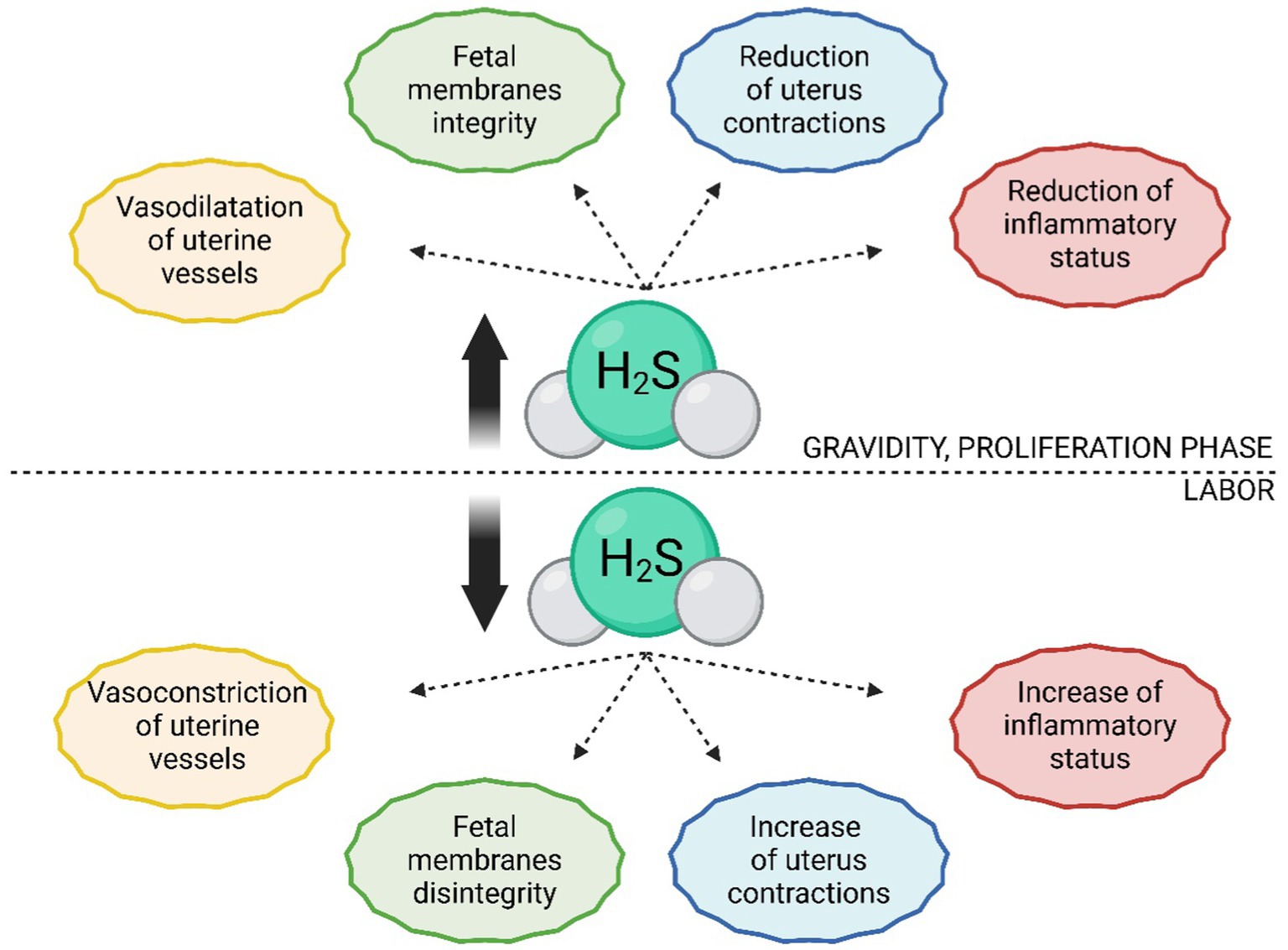
Figure 4. Comparison of H2S levels and their functions during pregnancy and labor. In estrogen-dominant phases, such as pregnancy or the proliferative phase of the estrus/menstrual cycle, there is an increased production of H2S. In uterine tissues, H2S promotes dilation of uterine blood vessels, contributing to tissue perfusion and proper development of the placenta, fetus, and nutrient supply to the fetus. It also maintains the integrity of fetal membranes and has tocolytic effects on the uterus, thereby delaying labor. Before and during labor, there is a significant reduction in H2S in uterine tissues, leading to constriction of uterine blood vessels, rupture of fetal membranes, and increased uterine contractions.
In the past decade, studies have emerged reporting the regulation of uterine vessel vasodilation by estrogens through their influence on promoting H2S synthesis via CBS and CSE (104). For example, it has been described that during estrogen-dominant phases of the female cycle (i.e., proliferation, pregnancy), CBS production is higher than the secretory phase. Specifically, CBS seems to be the primary H2S-producing enzyme responding to elevated estrogen levels, as the expression of CSE and 3-MST does not change in gravid tissue compared to non-gravid tissue (20, 105). Interestingly, Zeigler et al. (106) found a decrease in plasma H2S levels in the later stages of pregnancy compared to postpartum. This could be explained more likely as an increase in H2S consumption, as it is essential for S-sulfhydration of proteins necessary for the growth of maternal and fetal tissues. S-sulfhydrated proteins are extensively involved in processes such as the contraction and relaxation of smooth muscle in blood vessels (107, 108). Additionally, during pregnancy, the H2S dilution is more significant as the volume of maternal blood plasma can increase by up to 50% (106, 109). This hypothesis is supported by the increased production of H2S in intrauterine tissues during pregnancy, which leads to a higher rate of S-sulfhydration of proteins compared to non-pregnant tissue. These results support the finding that the expression of CBS is greater in estrogen-dominant phases, as the consumption of H2S is also higher (110).
While the precise mechanism describing estrogen-induced stimulation of H2S biosynthesis in uterine arteries is unknown, a hypothesis suggests estrogen receptors’ important role in this signaling pathway (104). This hypothesis has been recently confirmed, as it was found that estrogen receptors activate CBS promoters, thereby stimulating its production. In contrast, the activity of the CSE promoter remains unchanged (110). When it comes to vascular dilation, it is worth noting the previously established influence of another gasotransmitter – NO, which is also a potent vasodilator in the bloodstream and interacts with H2S in many organ systems (111–113). It is presumable that H2S, NO, and estrogens, which interact with both H2S and NO, synergistically contribute to the vasodilation of uterine vessels and that these systems behave towards each other as backup mechanisms because pathology occurs only after the inhibition of both signaling pathways (111, 113).
3.2.2 Uterus and pregnancy
One of the most referred impacts of H2S on the human (114) and rat (115) uterus is its tocolytic effects, which can be caused by H2S itself, as well as its precursor (L-cysteine) (116) or donor (NaHS) (117). These effects, promoting uterine relaxation, are significant for gravidity maintenance. Therefore, it is not surprising that the expression of CBS and CSE and production of H2S increases during gravidity and, conversely, abruptly decreases with the onset of labor (110, 114, 118). H2S also effectively prolongs the duration of labor and reduces the frequency of uterine contractions, which can contribute to a smooth delivery process (Figure 4) (119). It is assumed that the mechanism of the tocolytic effects of H2S lies in the opening of channels, as the body utilizes the exact mechanism in the bloodstream and other smooth muscle tissues (18, 120). Additionally, it has been demonstrated that the inhibition of KATP channels leads to the absence of relaxation effects of H2S donors (45, 92). It is possible that H2S also regulates activity of BKCa channels and L-type Ca2+ channels, as they also influence the relaxation of myometrium (52, 73, 118). Furthermore, the tocolytic effects of H2S may lie in inhibition of contraction-associated proteins (CAPs) and suppressing the toll-like receptor 4 (TLR4)/NF-κB signaling pathway (Figure 5) (16, 29, 42). Besides its tocolytic effects, H2S may also impact uterine immune response and placental vessel remodeling through the modulation of the uterine natural killer (uNK) cells (121–123). H2S signaling is also essential for maintaining early pregnancy, and its deficiency can lead to reduced litter size due to early embryo loss or placental inflammation (70, 124). H2S may further facilitate the physiological implantation of the embryo by regulating ion transport activity in the endometrial epithelium and supporting DNA synthesis (125–127).
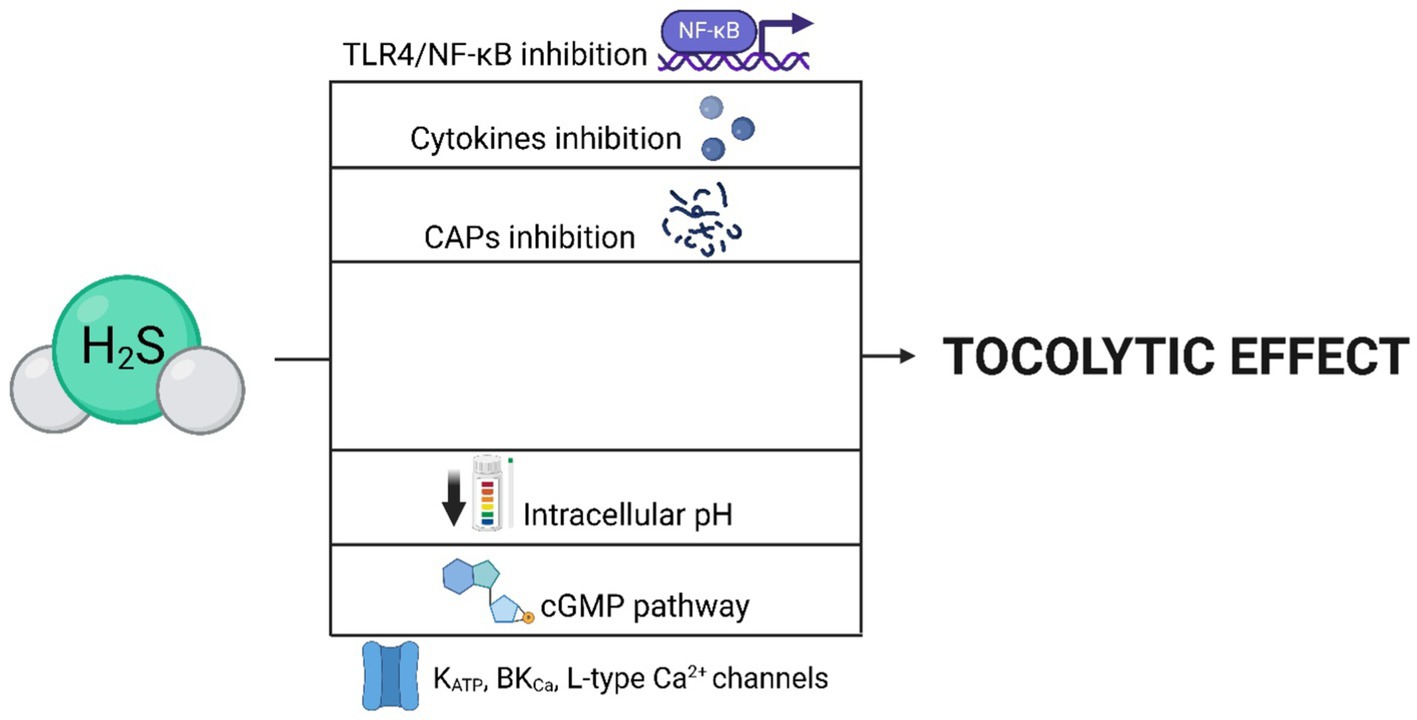
Figure 5. Mechanisms leading to the tocolytic effect of H2S. The tocolytic effects of H2S can be mediated by the opening of ion channels (KATP, BKCa, L-type Ca2+), as well as through the cGMP pathway or a reduction in intracellular pH. H2S achieves these effects by inhibiting CAPs, pro-inflammatory cytokines, and the TLR4/NF-κB signaling pathway.
The relationship between H2S and estradiol (E2) is intriguing because both contribute to uterine quiescence during pregnancy by regulating the expression of CAPs (128, 129). Estrogens, in general, appear to regulate H2S-producing enzymes, consequently affecting the levels of H2S itself (98). The increased production of CBS and H2S in the uterine arteries during pregnancy is influenced by endogenous estrogens acting through specific estrogen receptors (ER) in pregnant rats. This indicates that the physiological changes associated with pregnancy, such as elevated levels of endogenous estrogens, play a role in stimulating the expression of CBS and subsequent H2S production in the uterine arteries. The specific ER-mediated mechanism implies that ER are involved in regulating this process, highlighting the importance of endogenous estrogen signaling in mediating vascular adaptations during pregnancy (110). Specifically, E2 modulates gene expression and redox balance in the uterus by inducing transsulfuration via CBA and CSE, for which this metabolic pathway is unique (20, 130). The effects of E2 may also influence the metabolism of myometrial cysteine, which is utilized by H2S-producing enzymes to generate H2S, particularly during periods of elevated E2 levels such as estrus and gravidity. This pathway is mediated through sulfur amino acids and myometrial cysteine sulfinic acid decarboxylase (CSAD), the activity of which is reduced by E2. Estrogen-mediated regulation of H2S-producing enzymes and H2S itself occurs not only in uterine tissue but also in uterine vessels, where E2 activates CBS promotors leading to increased production of H2S in estrogen-dominant phases, leaving no doubt about the connection between H2S and estrogens (20, 105).
H2S-producing enzymes plays a vital role in intrauterine tissues by regulating Hcy levels and thus preventing pathological conditions. Uncontrolled Hcy levels can lead to hyperhomocysteinemia (73, 77) associated with various adverse outcomes in pregnancy, including impaired implantation (131), reduced litter size (124, 131), neural tube defects (132), miscarriages (5, 64), preeclampsia (5, 133, 134), hypertension (76, 134), and fetal growth restrictions (135). However, CSE is not a secondary enzyme in this matter. Is it also capable of generating H2S from Hcy and effectively regulating its levels (Figure 1) (74, 76, 77, 136, 137). Interestingly, CBS-KO in the uterus itself is not a direct cause of infertility in these individuals. Infertility in CBS-KO individuals occurs due to the resulting hyperhomocysteinemia or due to the action of another factor in the uterine environment of CBS-KO homozygotes. This indicates that the prominent role of H2S-producing enzymes during pregnancy is regulating Hcy levels around the growing fetus (64). It is worth noting that although CBS-KO may lead to reduced fertility or even infertility in female offspring, this is not the case for male offspring (64, 122, 131). It is possible that the effect is related to the pathways of female sex hormones, such as LH and E2, as described in previous sections, and may not necessarily affect male fertility. However, further research would be needed to confirm these assumptions.
Given that H2S-producing enzymes play a specific role during pregnancy, it can be assumed that the gas they produce also plays a role. In the context of previously mentioned pathogenic states, H2S likely inhibits the soluble fms-like tyrosine kinase-1 (sFlt1), a vascular endothelial growth factor (VEGF) antagonist associated with hypertension and preeclampsia (138). Because elevated Hcy levels are a risk factor for preeclampsia, H2S may also prevent the onset of preeclampsia through this pathway (18, 73, 133, 139).
3.2.3 The role of H2S in placenta
Like the uterus, a reduced CBS expression towards the end of gestation has been described in placental and decidual tissues. H2S likely serves to maintain the integrity of the chorion/amnion before birth by slowing down the aging of the fetal membranes’ cells, so it is not surprising that its expression in these tissues decreases with the onset of labor (Figure 4) (18, 89). It is also interesting to note that both CBS and CSE expression in fetal membranes decreases much more during physiological labor than in infants delivered by cesarean section (17, 140). This shows that H2S is necessary for maintaining pregnancy, and a decrease in its expression appears to be one of the critical factors leading to the physiological onset of labor (16, 140). However, it should be noted that the role of H2S in the placenta may vary between species. For example, hypoxic conditions in the human placenta lead to increased H2S production, which is not observed in rat placenta (18). H2, S also contributes to proper placental development by promoting angiogenesis through placental growth factor (PIGF), VEGF, and signaling pathways PKB, nitric oxide synthases (NOS)/NO, and MAPK3/1 (141–143). VEGF is a key factor in regulating placental angiogenesis and this process is stimulated by activation of MAPK pathway in placental endothelial cells (144). However, in contrast to these positive effects of H2S, an association has been described between increased CBS expression in placentas and infants with Down syndrome, indicating that proper regulation of H2S expression in intrauterine tissues is crucial for physiologically ongoing gravidity (145–147).
The relationship between H2S and two other gasotransmitters in fetal membranes is intriguing. While the CO donor (hemin) in fetal membranes does not affect H2S production, the NO donor (sodium nitroprusside) leads to a significant increase in H2S production in this tissue (18). It is, therefore, possible that both H2S and NO synergistically contribute to maintaining the integrity of fetal membranes and pregnancy. This would imply that intrauterine tissues can be included among many other tissues where a mutual relationship between H2S and NO has been observed (112, 148–151).
4 The role of H2S during embryo development
For several years, it has been known that the human trophoblast produces H2S through the expression of CBS and CSE, with some studies indicating that CSE is the primary
H2S-producing enzyme in the first trimester of pregnancy (33, 143). Generally, supplementing the culture medium with H2S and NO donors supports embryo development in vitro. Once again, the synergy between these two gasotransmitters was described in this tissue, as H2S produced by the trophoblast, like VEGF, stimulates endothelial nitric oxide synthase (eNOS) activation (143). However, the precise role of these gasotransmitters in embryogenesis remains unclear. One of the main roles of H2S during embryo development is likely epigenetic regulation of embryogenesis, cell cycle, support of DNA formation, and proliferation (152, 153). Based on previous research confirming the regulation of specific promoters by H2S, for example, in vascular smooth muscle cells (154), it can be hypothesized that this regulation is also functional in mammalian embryo cells. This hypothesis is supported by research confirming that H2S modulates genes encoding proteins involved in early embryo epigenetic regulation (152). Even though the precise mechanism of embryonic epigenetic regulation by H2S is unknown, it can be assumed that H2S has a positive effect on early embryonic development, and it may even be essential for enhancing transcription and modification of specific embryonic genes related primarily to metabolism (33). Conversely, reduced expression of H2S-producing enzymes may lead, for example, to reduced PIGF production causing fetal growth restriction (FGR) or recurrent spontaneous miscarriages (124, 155).
Furthermore, H2S appears to be an important factor in transporting the morula from the oviduct to the uterus, as inhibition of CBS expression leads to embryo retention or prolongs its transport. H2S likely acts against contractile endothelins, facilitating oviduct peristalsis and, consequently, the transit of the embryo itself (83). H2S also promotes proliferation, migration, cytoskeleton remodeling, and invasion of trophoblast cells, where it activates various types of kinases (e.g., FAK, Src, ERK), Rho GTPases, and upregulates metalloproteinases 2 and 9 (89). On the other hand, excessive expression of CBS and CSE in the oviducts may be a sign of ectopic gravidity or embryonal carcinoma, so it cannot be conclusively stated that higher levels of CBS and CSE expression in this tissue indicate physiological embryo transport (83). Proper regulation of H2S expression is also essential in preventing the development of intrauterine growth restriction (IUGR) and preeclampsia (155). Additionally, H2S protects the heart of chicken embryos by regulating myocardial KATP channels (156).
H2S-producing enzymes have been identified even in zebrafish embryos, where there were described 2 cbs orthologs – cbsa and cbsb (157). Cbsb is crucial for ion homeostasis, while cbsa appears redundant (158, 159). These results indicate that H2S is essential in embryonic development across various taxa.
5 Conclusion
The production of H2S has been demonstrated in all female reproductive tissues, primarily through the enzymes CBS and CSE and, to a lesser extent, through 3-MST (Table 1). We can assume that H2S plays a crucial role in various physiological processes associated with female reproduction, given its ability to vasodilate uterine and umbilical vessels, as well as maintain pregnancy through both the tocolytic effects of H2S and its capability to preserve the integrity of fetal membranes. Additionally, H2S has anti-aging effects on mammalian oocytes, supporting their maturation and ovulation, aiding in the transport of early embryos into the uterus, and epigenetic regulation of their genes. Further on, an important characteristic of H2S-producing enzymes, CBS and CSE, is their ability to regulate homocysteine levels in the vicinity of cells through the production of H2S. This mechanism within the female reproductive tract serves to prevent pathological conditions such as hyperhomocysteinemia, which can lead to preeclampsia, miscarriages, congenital fetal abnormalities, and others.
Conversely, dysregulation of H2S signaling may be associated with various pathological conditions. It has been reported that aberrant H2S metabolism results in impaired oviductal transport of embryos and developmental delay of preimplantation embryos in mice (83). It has also been shown that dysregulated placental CBS/H2S signaling significantly contributes to increased embryonic resorption in mice (124). Notably, H2S production was found to be upregulated in the human oviduct in ectopic pregnancy, suggesting the involvement of dysregulation of H2S homeostasis (83). Dysregulation of H2S signaling has been also linked to the pathogenesis of preeclampsia (5, 140). Abnormal H2S signaling has recently been reported to be involved in diabetes-related uterine dysfunction as it was found that in non-obese diabetic mice, uterine H2S production is 2-fold higher than in the control group. This increase in H2S associated with 3-MST has been shown to cause a reduction in spontaneous endogenous uterine contractions (160). In addition, CBS has been proposed to promote ovarian cancer progression, tumor growth, and drug resistance (161), while CSE has been associated with breast cancer metastasis promotion (162).
The effects of H2S and subsequent signaling pathways in the aforementioned tissues are well-described. These effects are mediated by kinases (PKA, PKB, MAPKs), ion channels (T and L-type Ca2+, KATP, BKCa), transcription factors (NF-κB), and other cellular messengers (NO, E2, PIGF, cytokines). A particularly interesting function of H2S is its epigenetic effects, involving chromatin modification and activation of specific promoters, as well as its interaction with female sex hormones (LH, E2). Yet, these effects are not sufficiently elucidated, although clarifying their precise molecular aspects might result in the development of new methods and drugs, particularly in the field of women’s health and perinatal medicine.
In conclusion, a comprehensive understanding of H2S function could lead to its therapeutic use in disorders related to reproduction. For instance, its tocolytic and vasodilatory effects could be utilized to maintain pregnancy, support embryo implantation, and prevent miscarriages. The interaction of H2S with LH and E2 could also be used in the development of new drugs regulating the menstrual cycle or supporting superovulation in women undergoing oocyte aspiration prior to in vitro fertilization. H2S could also enhance the culture media of oocytes and embryos in IVF clinics, promoting their proper development and increasing the chances of successful pregnancy. Additionally, it may serve as an effective treatment for conditions like hyperhomocysteinemia, preeclampsia, or irregular estrus/menstrual cycles.
Author contributions
AP: Conceptualization, Writing – original draft, Writing – review & editing. ZP: Conceptualization, Writing – original draft, Writing – review & editing. BK: Writing – review & editing. NZ: Writing – review & editing. EC: Writing – review & editing. PP: Supervision, Writing – review & editing. MS: Supervision, Writing – review & editing.
Funding
The author(s) declare financial support was received for the research, authorship, and/or publication of this article. This review was supported by the Internal Grant Agency of the Czech University of Life Sciences in Prague (SV22-10-21230).
Acknowledgments
Artificial intelligence ChatGPT 3 was used to translate text into English. The prompts utilized are present in Supplementary Table 1.
Conflict of interest
The authors declare that the research was conducted in the absence of any commercial or financial relationships that could be construed as a potential conflict of interest.
Publisher's note
All claims expressed in this article are solely those of the authors and do not necessarily represent those of their affiliated organizations, or those of the publisher, the editors and the reviewers. Any product that may be evaluated in this article, or claim that may be made by its manufacturer, is not guaranteed or endorsed by the publisher.
Supplementary material
The Supplementary material for this article can be found online at: https://www.frontiersin.org/articles/10.3389/fvets.2024.1378435/full#supplementary-material
SUPPLEMENTARY TABLE 1 | This file contains the original prompt in Czech and its translation into English. AI was used primarily as a guide for the translation, and our team edited and refined the resulting text.
References
1. Bredt, DS, and Snyder, SH. Nitric oxide, a novel neuronal messenger. Neuron. (1992) 8:3–11. doi: 10.1016/0896-6273(92)90104-L
2. Maines, MD. The heme oxygenase system: a regulator of second messenger gases. Annu Rev Pharmacol Toxicol. (1997) 37:517–54. doi: 10.1146/annurev.pharmtox.37.1.517
3. Abe, K, and Kimura, H. The possible role of hydrogen sulfide as an endogenous neuromodulator. J Neurosci. (1996) 16:1066–71. doi: 10.1523/JNEUROSCI.16-03-01066.1996
4. Olas, B. Hydrogen sulfide in signaling pathways. Clin Chim Acta. (2015) 439:212–8. doi: 10.1016/j.cca.2014.10.037
5. Wang, K, Ahmad, S, Cai, M, Rennie, J, Fujisawa, T, Crispi, F, et al. Dysregulation of hydrogen sulfide producing enzyme cystathionine γ-lyase contributes to maternal hypertension and placental abnormalities in preeclampsia. Circulation. (2013) 127:2514–22. doi: 10.1161/CIRCULATIONAHA.113.001631
6. Olas, B. Hydrogen sulfide in hemostasis: friend or foe? Chem Biol Interact. (2014) 217:49–56. doi: 10.1016/j.cbi.2014.04.006
7. Xu, S, Liu, Z, and Liu, P. Targeting hydrogen sulfide as a promising therapeutic strategy for atherosclerosis. Int J Cardiol. (2014) 172:313–7. doi: 10.1016/j.ijcard.2014.01.068
8. Kimura, H. Hydrogen sulfide and Polysulfides as biological mediators. Molecules. (2014) 19:16146–57. doi: 10.3390/molecules191016146
9. Shibuya, N, Koike, S, Tanaka, M, Ishigami-Yuasa, M, Kimura, Y, Ogasawara, Y, et al. A novel pathway for the production of hydrogen sulfide from D-cysteine in mammalian cells. Nat Commun. (2013) 4:1366. doi: 10.1038/ncomms2371
10. Fiorucci, S, Distrutti, E, Cirino, G, and Wallace, J. The emerging roles of hydrogen sulfide in the gastrointestinal tract and liver. Gastroenterology. (2006) 131:259–71. doi: 10.1053/j.gastro.2006.02.033
11. Olson, K, DeLeon, E, Gao, Y, Hurley, K, Sadauskas, V, Batz, C, et al. Thiosulfate: a readily accessible source of hydrogen sulfide in oxygen sensing. Am J Phys Regul Integr Comp Phys. (2013) 305:592–603. doi: 10.1152/ajpregu.00421.2012
12. d'Emmanuele di Villa Bianca, R, Sorrentino, R, Maffia, P, Mirone, V, Imbimbo, C, Fusco, F, et al. Hydrogen sulfide as a mediator of human corpus cavernosum smooth-muscle relaxation. Proc Natl Acad Sci U S A. (2009) 106:4513–8. doi: 10.1073/pnas.0807974105
13. d’Emmanuele di Villa Bianca, R, Sorrentino, R, Mirone, V, and Cirino, G. Hydrogen sulfide and erectile function: a novel therapeutic target. Nat. Rev. Uro. (2011) 8:286–9. doi: 10.1038/nrurol.2011.45
14. Cao, Y, Zhu, X, Zhen, P, Tian, Y, Ji, D, Xue, K, et al. Cystathionine β‐synthase is required for oocyte quality by ensuring proper meiotic spindle assembly. Cell Prolif. (2022) 55:e13322. doi: 10.1111/cpr.13322
15. Liang, R, Yu, W, Du, J, Yang, L, Yang, J, Xu, J, et al. Cystathionine β synthase participates in murine oocyte maturatione mediated by homocysteine. Reprod Toxicol. (2007) 24:89–96. doi: 10.1016/j.reprotox.2007.04.002
16. You, X, Chen, Z, Zhao, H, Xu, C, Liu, W, Sun, Q, et al. Endogenous hydrogen sulfide contributes to uterine quiescence during pregnancy. Reproduction. (2017) 153:535–43. doi: 10.1530/REP-16-0549
17. Sun, Q, Chen, Z, He, P, Li, Y, Ding, X, Huang, Y, et al. Reduced expression of hydrogen sulfide–generating enzymes Down-regulates 15-Hydroxyprostaglandin dehydrogenase in chorion during term and preterm labor. Am J Pathol. (2018) 188:63–71. doi: 10.1016/j.ajpath.2017.09.006
18. Patel, P, Vatish, M, Heptinstall, J, Wang, R, and Carson, R. The endogenous production of hydrogen sulphide in intrauterine tissues. Reprod Biol Endocrinol. (2009) 7:10. doi: 10.1186/1477-7827-7-10
19. Nevoral, J, Žalmanová, T, Zámostná, K, Kott, T, Kučerová-Chrpová, V, Bodart, J, et al. Endogenously produced hydrogen sulfide is involved in porcine oocyte maturation in vitro. Nitric Oxide. (2015) 51:24–35. doi: 10.1016/j.niox.2015.09.007
20. Sheibani, L, Lechuga, T, Zhang, H, Hameed, A, Wing, D, Kumar, S, et al. Augmented H2S production via cystathionine-beta-synthase upregulation plays a role in pregnancy-associated uterine vasodilation†. Biol Reprod. (2017) 96:664–72. doi: 10.1095/biolreprod.116.143834
21. Wang, M, Wang, Z, Miao, Y, Wei, H, Peng, J, and Zhou, Y. Diallyl Trisulfide promotes placental angiogenesis by regulating lipid metabolism and alleviating inflammatory responses in obese pregnant mice. Nutrients. (2022) 14:2230. doi: 10.3390/nu14112230
22. Sun, Q, Huang, J, Yue, Y, Xu, J, Jiang, P, Yang, D, et al. Hydrogen sulfide facilitates vaginal lubrication by activation of epithelial ATP-sensitive K+ Channels and cystic fibrosis transmembrane conductance regulator. J Sex Med. (2016) 13:798–807. doi: 10.1016/j.jsxm.2016.03.001
23. Módis, K, Ju, Y, Ahmad, A, Untereiner, A, Altaany, Z, Wu, L, et al. S- Sulfhydration of ATP synthase by hydrogen sulfide stimulates mitochondrial bioenergetics. Pharmacol Res. (2016) 113:116–24. doi: 10.1016/j.phrs.2016.08.023
24. Borisov, V, and Forte, E. Impact of hydrogen sulfide on mitochondrial and bacterial bioenergetics. Int J Mol Sci. (2021) 22:12688. doi: 10.3390/ijms222312688
25. Nagahara, N, Ito, T, Kitamura, H, and Nishino, T. Tissue and subcellular distribution of mercaptopyruvate sulfurtransferase in the rat: confocal laser fluorescence and immunoelectron microscopic studies combined with biochemical analysis. Histochem Cell Biol. (1998) 110:243–50. doi: 10.1007/s004180050286
26. Fräsdorf, B, Radon, C, and Leimkühler, S. Characterization and interaction studies of two isoforms of the dual localized 3-Mercaptopyruvate Sulfurtransferase TUM1 from humans. J Biol Chem. (2014) 289:34543–56. doi: 10.1074/jbc.M114.605733
27. Forman, H. Glutathione – from antioxidant to post-translational modifier. Arch Biochem Biophys. (2016) 595:64–7. doi: 10.1016/j.abb.2015.11.019
28. Maclean, K, Jiang, H, Aivazidis, S, Kim, E, Shearn, C, Harris, P, et al. Taurine treatment prevents derangement of the hepatic γ‐glutamyl cycle and methylglyoxal metabolism in a mouse model of classical homocystinuria: regulatory crosstalk between thiol and sulfinic acid metabolism. FASEB J. (2018) 32:1265–80. doi: 10.1096/fj.201700586R
29. Chen, Z, Zhang, M, Zhao, Y, Xu, W, Xiang, F, Li, X, et al. Hydrogen sulfide contributes to uterine quiescence through inhibition of NLRP3 Inflammasome activation by suppressing the TLR4/NF-κB Signalling pathway. J Inflamm Res. (2021) 14:2753–68. doi: 10.2147/JIR.S308558
30. Stuhlmeier, K, Bröll, J, and Iliev, B. NF-KappaB independent activation of a series of Proinflammatory genes by hydrogen sulfide. Exp Biol Med. (2009) 234:1327–38. doi: 10.3181/0904-RM-137
31. Aggarwal, B, Kunnumakkara, A, Harikumar, K, Gupta, S, Tharakan, S, Koca, C, et al. Signal transducer and activator of transcription‐3, inflammation, and Cancer. Ann N Y Acad Sci. (2009) 1171:59–76. doi: 10.1111/j.1749-6632.2009.04911.x
32. Papapetropoulos, A, Pyriochou, A, Altaany, Z, Yang, G, Marazioti, A, Zhou, Z, et al. Hydrogen sulfide is an endogenous stimulator of angiogenesis. Proc Natl Acad Sci. (2009) 106:21972–7. doi: 10.1073/pnas.0908047106
33. Sun, Q, Zhong, H, Yue, Y, Xiong, F, Chen, L, Peng, X, et al. Endogenous hydrogen sulfide promotes human preimplantation embryonic development by regulating metabolism-related gene expression. Nitric Oxide. (2022) 120:9–15. doi: 10.1016/j.niox.2021.12.008
34. Yang, G, Sun, X, and Wang, R. Hydrogen sulfide‐induced apoptosis of human aorta smooth muscle cells via the activation of mitogen‐activated protein kinases and caspase‐3. FASEB J. (2004) 18:1782–4. doi: 10.1096/fj.04-2279fje
35. Zhao, K, Ju, Y, Li, S, Altaany, Z, Wang, R, and Yang, G. S‐sulfhydration of MEK 1 leads to PARP ‐1 activation and DNA damage repair. EMBO Rep. (2014) 15:792–800. doi: 10.1002/embr.201338213
36. Du, J, Hui, Y, Cheung, Y, Bin, G, Jiang, H, Chen, X, et al. The possible role of hydrogen sulfide as a smooth muscle cell proliferation inhibitor in rat cultured cells. Heart Vessel. (2004) 19:75–80. doi: 10.1007/s00380-003-0743-7
37. Rinaldi, L, Gobbi, G, Pambianco, M, Micheloni, C, Mirandola, P, and Vitale, M. Hydrogen sulfide prevents apoptosis of human PMN via inhibition of p38 and caspase 3. Lab Investig. (2006) 86:391–7. doi: 10.1038/labinvest.3700391
38. Kimura, H. Hydrogen sulfide induces cyclic AMP and modulates the NMDA receptor. Biochem Biophys Res Commun. (2000) 267:129–33. doi: 10.1006/bbrc.1999.1915
39. Njie-Mbye, Y, Kulkarni, M, Opere, C, and Ohia, S. Mechanism of action of hydrogen sulfide on cyclic AMP formation in rat retinal pigment epithelial cells. Exp Eye Res. (2012) 98:16–22. doi: 10.1016/j.exer.2012.03.001
40. Cai, W, Wang, M, Moore, P, Jin, H, Yao, T, and Zhu, Y. The novel proangiogenic effect of hydrogen sulfide is dependent on Akt phosphorylation. Cardiovasc Res. (2007) 76:29–40. doi: 10.1016/j.cardiores.2007.05.026
41. Yong, Q, Lee, S, Foo, C, Neo, K, Chen, X, and Bian, J. Endogenous hydrogen sulphide mediates the cardioprotection induced by ischemic postconditioning. Am J Phys Heart Circ Phys. (2008) 295:1330–40. doi: 10.1152/ajpheart.00244.2008
42. Wang, S, Chen, Y, Chen, N, Wang, L, Chen, D, Weng, H, et al. Hydrogen sulfide promotes autophagy of hepatocellular carcinoma cells through the PI3K/Akt/mTOR signaling pathway. Cell Death Dis. (2017) 8:2688–8. doi: 10.1038/cddis.2017.18
43. Bucci, M, Papapetropoulos, A, Vellecco, V, Zhou, Z, Pyriochou, A, Roussos, C, et al. Hydrogen sulfide is an endogenous inhibitor of phosphodiesterase activity. Arterioscler Thromb Vasc Biol. (2010) 30:1998–2004. doi: 10.1161/ATVBAHA.110.209783
44. Bibli, S, Yang, G, Zhou, Z, Wang, R, Topouzis, S, and Papapetropoulos, A. Role of cGMP in hydrogen sulfide signaling. Nitric Oxide. (2015) 46:7–13. doi: 10.1016/j.niox.2014.12.004
45. Mustafa, A, Gadalla, M, Sen, N, Kim, S, Mu, W, Gazi, S, et al. H2S signals through protein S-Sulfhydration. Sci Signal. (2009):2. doi: 10.1126/scisignal.2000464
46. Li, L, Rose, P, and Moore, P. Hydrogen Sulfide and Cell Signaling. Annu Rev Pharmacol Toxicol. (2011) 51:169–87. doi: 10.1146/annurev-pharmtox-010510-100505
47. Untereiner, A, and Wu, L. Hydrogen sulfide and glucose homeostasis: a tale of sweet and the stink. Antioxid Redox Signal. (2018) 28:1463–82. doi: 10.1089/ars.2017.7046
48. Fiorucci, S, Antonelli, E, Mencarelli, A, Orlandi, S, Renga, B, Rizzo, G, et al. The third gas: H2S regulates perfusion pressure in both the isolated and perfused normal rat liver and in cirrhosis. Hepatology. (2005) 42:539–48. doi: 10.1002/hep.20817
49. Hosoki, R, Matsuki, N, and Kimura, H. The possible role of hydrogen sulfide as an endogenous smooth muscle relaxant in synergy with nitric oxide. Biochem Biophys Res Commun. (1997) 237:527–31. doi: 10.1006/bbrc.1997.6878
50. Distrutti, E, Sediari, L, Mencarelli, A, Renga, B, Orlandi, S, Antonelli, E, et al. Evidence that hydrogen sulfide exerts Antinociceptive effects in the gastrointestinal tract by activating K ATP Channels. J Pharmacol Exp Ther. (2005) 316:325–35. doi: 10.1124/jpet.105.091595
51. Monjok, E, Kulkarni, K, Kouamou, G, McKoy, M, Opere, C, Bongmba, O, et al. Inhibitory action of hydrogen sulfide on muscarinic receptor-induced contraction of isolated porcine irides. Exp Eye Res. (2008) 87:612–6. doi: 10.1016/j.exer.2008.09.011
52. Li, Y, Zang, Y, Fu, S, Zhang, H, Gao, L, and Li, J. H2S relaxes vas deferens smooth muscle by modulating the large conductance Ca2+‐activated K+ (BKCa) Channels via a redox mechanism. J Sex Med (2012) 9:2806–2813. doi: 10.1111/j.1743-6109.2012.02879.x
53. Nevoral, J, Zalmanova, T, Hoskova, K, Stiavnicka, M, Hosek, P, Petelak, A, et al. Involvement of K+ATP and Ca2+ channels in hydrogen sulfide-suppressed ageing of porcine oocytes. Biol Res. (2018) 51:38. doi: 10.1186/s40659-018-0187-2
54. Elies, J, Scragg, J, Dallas, M, Huang, D, Huang, S, Boyle, J, et al. Inhibition of T-type Ca2+ Channels by hydrogen sulfide In: C Peers, P Kumar, C Wyatt, E Gauda, C Nurse, and N Prabhakar, editors. Arterial chemoreceptors in physiology and pathophysiology. Cham: Springer International Publishing (2015)
55. Kiss, L, Deitch, E, and Szabó, C. Hydrogen sulfide decreases adenosine triphosphate levels in aortic rings and leads to vasorelaxation via metabolic inhibition. Life Sci. (2008) 83:589–94. doi: 10.1016/j.lfs.2008.08.006
56. Trevisani, M, Patacchini, R, Nicoletti, P, Gatti, R, Gazzieri, D, Lissi, N, et al. Hydrogen sulfide causes vanilloid receptor 1-mediated neurogenic inflammation in the airways. Br J Pharmacol. (2005) 145:1123–31. doi: 10.1038/sj.bjp.0706277
57. Streng, T, Axelsson, H, Hedlund, P, Andersson, D, Jordt, S, Bevan, S, et al. Distribution and function of the hydrogen sulfide–sensitive TRPA1 Ion Channel in rat urinary bladder. Eur Urol. (2008) 53:391–400. doi: 10.1016/j.eururo.2007.10.024
58. Kloesch, B, Steiner, G, Mayer, B, and Schmidt, K. Hydrogen sulfide inhibits endothelial nitric oxide formation and receptor ligand-mediated Ca2+ release in endothelial and smooth muscle cells. Pharmacol Rep. (2016) 68:37–43. doi: 10.1016/j.pharep.2015.05.026
59. Meng, G, Zhao, S, Xie, L, Han, Y, and Ji, Y. Protein S‐sulfhydration by hydrogen sulfide in cardiovascular system. Br J Pharmacol. (2018) 175:1146–56. doi: 10.1111/bph.13825
60. Fukami, K, Fukami, K, Sekiguchi, F, Sekiguchi, F, Kawabata, A, and Kawabata, A. Hydrogen sulfide and T-type Casup2+/sup Channels in pain processing. Pharmacology. (2017) 99:196–203. doi: 10.1159/000449449
61. Kubo, S, Doe, I, Kurokawa, Y, Nishikawa, H, and Kawabata, A. Direct inhibition of endothelial nitric oxide synthase by hydrogen sulfide: contribution to dual modulation of vascular tension. Toxicology. (2007) 232:138–46. doi: 10.1016/j.tox.2006.12.023
62. Tang, G, Wu, L, Liang, W, and Wang, R. Direct stimulation of K ATP Channels by exogenous and endogenous hydrogen sulfide in Vascular smooth muscle cells. Mol Pharmacol. (2005) 68:1757–64. doi: 10.1124/mol.105.017467
63. Zhao, Y, Wei, H, Kong, G, Shim, W, and Zhang, G. Hydrogen sulfide augments the proliferation and survival of human induced pluripotent stem cell–derived mesenchymal stromal cells through inhibition of BKCa. Cytotherapy. (2013) 15:1395–405. doi: 10.1016/j.jcyt.2013.06.004
64. Guzmán, M, Navarro, M, Carnicer, R, Sarría, A, Acín, S, Arnal, C, et al. Cystathionine β-synthase is essential for female reproductive function. Hum Mol Genet. (2006) 15:3168–76. doi: 10.1093/hmg/ddl393
65. Yang, G, Wu, L, Jiang, B, Yang, W, Qi, J, Cao, K, et al. H2S as a physiologic Vasorelaxant: hypertension in mice with deletion of cystathionine γ-Lyase. Science. (2008) 322:587–90. doi: 10.1126/science.1162667
66. Bok, R, Guerra, D, Lorca, R, Wennersten, S, Harris, P, Rauniyar, A, et al. Cystathionine γ-lyase promotes estrogen-stimulated uterine artery blood flow via glutathione homeostasis. Redox Biol. (2021) 40:101827. doi: 10.1016/j.redox.2020.101827
67. Wang, R. Physiological implications of hydrogen sulfide: a whiff exploration that blossomed. Physiol Rev. (2012) 92:791–896. doi: 10.1152/physrev.00017.2011
68. Coletti, R, Almeida-Pereira, G, Elias, LLK, and Antunes-Rodrigues, J. Effects of hydrogen sulfide (H2S) on water intake and vasopressin and oxytocin secretion induced by fluid deprivation. Horm Behav. (2015) 67:12–20. doi: 10.1016/j.yhbeh.2014.11.008
69. Ishii, I, Akahoshi, N, Yamada, H, Nakano, S, Izumi, T, and Suematsu, M. Cystathionine γ-Lyase-deficient mice require dietary cysteine to protect against acute lethal myopathy and oxidative injury. J Biol Chem. (2010) 285:26358–68. doi: 10.1074/jbc.M110.147439
70. Panagiotopoulos, D, Andriopoulou, T, Spanou, V, Droggiti, D, Gkavogianni, T, Giamarellos‐Bourboulis, E, et al. Deficiency of hydrogen sulfide production and pregnancy rate in an experimental model: association with preterm delivery. Am J Reprod Immunol. (2023) 90:13764. doi: 10.1111/aji.13764
71. Stipanuk, M, and Ueki, I. Dealing with methionine/homocysteine sulfur: cysteine metabolism to taurine and inorganic sulfur. J Inherit Metab Dis. (2011) 34:17–32. doi: 10.1007/s10545-009-9006-9
72. Gelaude, A, Slaby, S, Cailliau, K, Marin, M, Lescuyer-Rousseau, A, Molinaro, C, et al. Hydrogen sulfide impairs meiosis resumption in Xenopus laevis oocytes. Cells. (2020) 9:237. doi: 10.3390/cells9010237
73. Carson, R, and Konje, J. Role of hydrogen sulfide in the female reproductive tract. Expert Rev Obstet Gynecol. (2014) 5:203–13. doi: 10.1586/eog.10.5
74. Chen, X, Jhee, K, and Kruger, W. Production of the neuromodulator H2S by cystathionine β-synthase via the condensation of cysteine and homocysteine. J Biol Chem. (2004) 279:52082–6. doi: 10.1074/jbc.C400481200
75. Łowicka, E, and Bełtowski, J. Hydrogen sulfide (H2S) - the third gas of interest for pharmacologists. Pharmacol Rep. (2007) 59:4–24.
76. Aubard, Y, Darodes, N, and Cantaloube, M. Hyperhomocysteinemia and pregnancy — review of our present understanding and therapeutic implications. Eur J Obstet Gynecol Reprod Biol. (2000) 93:157–65. doi: 10.1016/S0301-2115(00)00282-7
77. Chiku, T, Padovani, D, Zhu, W, Singh, S, Vitvitsky, V, and Banerjee, R. H2S biogenesis by human cystathionine γ-Lyase leads to the novel sulfur metabolites Lanthionine and Homolanthionine and is responsive to the grade of Hyperhomocysteinemia. J Biol Chem. (2009) 284:11601–12. doi: 10.1074/jbc.M808026200
78. Fayed, MR, Youssef, M, and Odah, MM. Hyperhomocysteinemia is a risk marker for development of maternal pre-eclampsia. Boll Chim Farm. (2004) 143:281–7.
79. Powell, M, and Somero, G. Adaptations to sulfide by hydrothermal vent animals: sites and mechanisms of detoxification and metabolism. Biol Bull. (1986) 171:274–90. doi: 10.2307/1541923
80. Liang, R, Yu, W, Du, J, Yang, L, Shang, M, and Guo, J. Localization of cystathionine β synthase in mice ovaries and its expression profile during follicular development. Chin Med J. (2006) 119:1877–83. doi: 10.1097/00029330-200611020-00006
81. Nevoral, J, Zamostna, K, Zalmanova, T, Kucerova-Chrpova, V, Dvorakova, M, Hoskova, K, et al. Physiological production of hydrogen sulfide is involved in porcine oocytes maturing in vitro. Nitric Oxide. (2015) 47:59–60. doi: 10.1016/j.niox.2015.02.147
82. Estienne, A, Portela, V, Choi, Y, Zamberlam, G, Boerboom, D, Roussel, V, et al. The endogenous hydrogen sulfide generating system regulates ovulation. Free Radic Biol Med. (2019) 138:43–52. doi: 10.1016/j.freeradbiomed.2019.03.028
83. Ning, N, Zhu, J, Du, Y, Gao, X, Liu, C, and Li, J. Dysregulation of hydrogen sulphide metabolism impairs oviductal transport of embryos. Nat Commun. (2014) 5:4107:10.1038/ncomms5107.
84. Nevoral, J, Petr, J, Gelaude, A, Bodart, J, Kucerova-Chrpova, V, Sedmikova, M, et al. Dual effects of hydrogen sulfide donor on meiosis and cumulus expansion of porcine cumulus-oocyte complexes. PLoS One. (2014) 9:e99613. doi: 10.1371/journal.pone.0099613
85. Nevoral, J, Kolbabova, T, Krejcova, T, and Sedmikova, M. Influence of hydrogen-sulfide on meiotic maturation and cumulus-expansion of porcine cumulus-oocyte complexes cultivated in vitro. J Reprod Devel Suppl. (2012) 105:1049. doi: 10.14882/jrds.105.0_1049
86. Nevoral, J, Bodart, J, and Petr, J. Gasotransmitters in gametogenesis and early development: holy trinity for assisted reproductive technology—a review. Oxidative Med Cell Longev. (2016) 2016:1–12. doi: 10.1155/2016/1730750
87. Hine, C, Zhu, Y, Hollenberg, A, and Mitchell, J. Dietary and endocrine regulation of endogenous hydrogen sulfide production: implications for longevity. Antioxid Redox Signal. (2018) 28:1483–502. doi: 10.1089/ars.2017.7434
88. Tyshkovskiy, A, Bozaykut, P, Borodinova, A, Gerashchenko, M, Ables, G, Garratt, M, et al. Identification and application of gene expression signatures associated with lifespan extension. Cell Metab. (2019) 30:573–93. doi: 10.1016/j.cmet.2019.06.018
89. Wang, X, and Tang, J. Focal adhesion kinase signaling is necessary for the hydrogen sulfide-enhanced proliferation, migration, and invasion of HTR8/SVneo human trophoblasts. Reprod Dev Med. (2023) 7:75–82. doi: 10.1097/RD9.0000000000000047
90. Krejcova, T, Smelcova, M, Petr, J, Bodart, J, Sedmikova, M, Nevoral, J, et al. Hydrogen sulfide donor protects porcine oocytes against aging and improves the developmental potential of aged porcine oocytes. PLoS One. (2015) 10:0116964. doi: 10.1371/journal.pone.0116964
91. Tang, G, Zhang, L, Yang, G, Wu, L, and Wang, R. Hydrogen sulfide-induced inhibition of L-type Ca2+ channels and insulin secretion in mouse pancreatic beta cells. Diabetologia. (2013) 56:533–41. doi: 10.1007/s00125-012-2806-8
92. Srilatha, B, Adaikan, P, and Moore, P. Possible role for the novel gasotransmitter hydrogen sulphide in erectile dysfunction—a pilot study. Eur J Pharmacol. (2006) 535:280–2. doi: 10.1016/j.ejphar.2006.02.001
93. King, A, Polhemus, D, Bhushan, S, Otsuka, H, Kondo, K, Nicholson, C, et al. Hydrogen sulfide cytoprotective signaling is endothelial nitric oxide synthase-nitric oxide dependent. Proc Natl Acad Sci. (2014) 111:3182–7. doi: 10.1073/pnas.1321871111
94. Reynolds, L, Caton, J, Redmer, D, Grazul‐Bilska, A, Vonnahme, K, Borowicz, P, et al. Evidence for altered placental blood flow and vascularity in compromised pregnancies. J Physiol. (2006) 572:51–8. doi: 10.1113/jphysiol.2005.104430
95. Carr, D, Wallace, J, Aitken, R, Milne, J, Martin, J, Zachary, I, et al. Peri- and postnatal effects of prenatal adenoviral VEGF gene therapy in growth-restricted sheep. Biol Reprod. (2016) 94:1–12. doi: 10.1095/biolreprod.115.133744
96. Yang, X, Tian, X, Liu, H, Wang, J, and Wang, F. Homocysteine increases uterine artery blood flow resistance in women with pregnancy loss. J Gynecol Obstet Hum Reprod. (2023) 52:102533. doi: 10.1016/j.jogoh.2023.102533
97. Zhang, H, Chen, J, Sheibani, L, Lechuga, T, and Chen, D. Pregnancy augments VEGF-stimulated in vitro angiogenesis and vasodilator (NO and H2S) production in human uterine artery endothelial cells. J Clin Endocrinol Metabol. (2017) 102:2382–93. doi: 10.1210/jc.2017-00437
98. Lechuga, T, Zhang, H, Sheibani, L, Karim, M, Jia, J, Magness, R, et al. Estrogen replacement therapy in Ovariectomized nonpregnant ewes stimulates uterine artery hydrogen sulfide biosynthesis by selectively up-regulating cystathionine β-synthase expression. Endocrinology. (2015) 156:2288–98. doi: 10.1210/en.2015-1086
99. Krishnamoorthy-Natarajan, G, and Koide, M. BK channels in the vascular system. Int Rev Neurobiol. (2016) 128:401–38. doi: 10.1016/bs.irn.2016.03.017
100. Kang, M, Hashimoto, A, Gade, A, and Akbarali, H. Interaction between hydrogen sulfide-induced sulfhydration and tyrosine nitration in the K ATP channel complex. American journal of physiology-gastrointestinal and liver. Physiology. (2015) 308:532–9. doi: 10.1152/ajpgi.00281.2014
101. Zhao, W. The vasorelaxant effect of H2S as a novel endogenous gaseous KATP channel opener. EMBO J. (2001) 20:6008–16. doi: 10.1093/emboj/20.21.6008
102. Xiao, D, Longo, L, and Zhang, L. Role of KATP and L-type Ca2+ channel activities in regulation of ovine uterine vascular contractility: effect of pregnancy and chronic hypoxia. Am J Obstet Gynecol. (2010) 203:596–6. doi: 10.1016/j.ajog.2010.07.038
103. Li, Y, Bai, J, Yang, Y, Hoshi, N, and Chen, D. Hydrogen sulfide relaxes human uterine artery via activating smooth muscle BKCa Channels. Antioxidants. (2020) 9:1127. doi: 10.3390/antiox9111127
104. Lechuga, T, Qi, Q, Kim, T, Magness, R, and Chen, D. E2β stimulates ovine uterine artery endothelial cell H2S production in vitro by estrogen receptor-dependent upregulation of cystathionine β-synthase and cystathionine γ-lyase expression†. Biol Reprod. (2019) 100:514–22. doi: 10.1093/biolre/ioy207
105. Lechuga, T, Qi, Q, Magness, R, and Chen, D. Ovine uterine artery hydrogen sulfide biosynthesis in vivo: effects of ovarian cycle and pregnancy. Biol Reprod. (2019) 100:1630–6. doi: 10.1093/biolre/ioz027
106. Zeigler, M, Fay, E, Moreni, S, Mao, J, Totah, R, and Hebert, M. Plasma hydrogen sulfide, nitric oxide, and thiocyanate levels are lower during pregnancy compared to postpartum in a cohort of women from the Pacific northwest of the United States. Life Sci. (2023) 322:121625. doi: 10.1016/j.lfs.2023.121625
107. Bibli, S, Hu, J, Looso, M, Weigert, A, Ratiu, C, Wittig, J, et al. Mapping the endothelial cell S -Sulfhydrome highlights the crucial role of integrin Sulfhydration in Vascular function. Circulation. (2021) 143:935–48. doi: 10.1161/CIRCULATIONAHA.120.051877
108. Bai, J, Jiao, F, Salmeron, A, Xu, S, Xian, M, Huang, L, et al. Mapping pregnancy-dependent Sulfhydrome unfolds diverse functions of protein Sulfhydration in human uterine artery. Endocrinology. (2023) 164:107. doi: 10.1210/endocr/bqad107/7223556
109. Soma-Pillay, P, Nelson-Piercy, C, Tolppanen, H, and Mebazaa, A. Physiological changes in pregnancy. Cardiovasc J Afr. (2016) 27:89–94. doi: 10.5830/CVJA-2016-021
110. Bai, J, Li, Y, Yan, G, Zhou, J, Salmeron, A, Fategbe, O, et al. ICI 182,780 attenuates selective upregulation of uterine artery cystathionine β-synthase expression in rat pregnancy. Int J Mol Sci. (2023) 24:14384. doi: 10.3390/ijms241814384
111. Whiteman, M, and Moore, P. Hydrogen sulfide and the vasculature: a novel vasculoprotective entity and regulator of nitric oxide bioavailability? J Cell Mol Med. (2009) 13:488–507. doi: 10.1111/j.1582-4934.2009.00645.x
112. Coletta, C, Papapetropoulos, A, Erdelyi, K, Olah, G, Módis, K, Panopoulos, P, et al. Hydrogen sulfide and nitric oxide are mutually dependent in the regulation of angiogenesis and endothelium-dependent vasorelaxation. Proc Natl Acad Sci. (2012) 109:9161–6. doi: 10.1073/pnas.1202916109
113. Mata-Greenwood, E, and Chen, D. Racial differences in nitric oxide—dependent Vasorelaxation. Reprod Sci. (2008) 15:9–25. doi: 10.1177/1933719107312160
114. You, X, Xu, C, Lu, J, Zhu, X, Gao, L, Cui, X, et al. Expression of cystathionine β-synthase and cystathionine γ-lyase in human pregnant myometrium and their roles in the control of uterine contractility. PLoS One. (2011) 6:23788. doi: 10.1371/journal.pone.0023788
115. Hayden, L, Franklin, K, Roth, S, and Moore, G. Inhibition of oxytocin-induced but not angiotensin-induced rat uterine contractions following exposure to sodium sulfide. Life Sci. (1989) 45:2557–60. doi: 10.1016/0024-3205(89)90239-7
116. Sidhu, R, Singh, M, Samir, G, and Carson, R. L-cysteine and sodium Hydrosulphide inhibit spontaneous contractility in isolated pregnant rat uterine strips in vitro. Pharmacol Toxicol. (2001) 88:198–203. doi: 10.1111/j.1600-0773.2001.880407.x
117. Pierce, S, Kupittayanant, S, Shmygol, T, and Wray, S. The effects of pH change on ca++ signaling and force in pregnant human myometrium. Am J Obstet Gynecol. (2003) 188:1031–8. doi: 10.1067/mob.2003.229
118. Robinson, H, Wray, S, and Sun, K. A new slow releasing, H2S generating compound, GYY4137 relaxes spontaneous and oxytocin-stimulated contractions of human and rat pregnant myometrium. PLoS One. (2012) 7:46278. doi: 10.1371/journal.pone.0046278
119. Hu, R, Lu, J, You, X, Zhu, X, Hui, N, and Ni, X. Hydrogen sulfide inhibits the spontaneous and oxytocin-induced contractility of human pregnant myometrium. Gynecol Endocrinol. (2011) 27:900–4. doi: 10.3109/09513590.2010.551563
120. Srilatha, B, Hu, L, Adaikan, G, and Moore, P. Initial characterization of hydrogen sulfide effects in female sexual function. J Sex Med. (2009) 6:1875–84. doi: 10.1111/j.1743-6109.2009.01291.x
121. Kulandavelu, S, Whiteley, K, Qu, D, Mu, J, Bainbridge, S, and Adamson, S. Endothelial nitric oxide synthase deficiency reduces uterine blood flow, spiral artery elongation, and placental oxygenation in pregnant mice. Hypertension. (2012) 60:231–8. doi: 10.1161/HYPERTENSIONAHA.111.187559
122. Nuño-Ayala, M, Guillén, N, Arnal, C, Lou-Bonafonte, J, de Martino, A, García-de-Jalón, J, et al. Cystathionine β-synthase deficiency causes infertility by impairing decidualization and gene expression networks in uterus implantation sites. Physiol Genomics. (2012) 44:702–16. doi: 10.1152/physiolgenomics.00189.2010
123. Gong, X, Chen, Z, Liu, Y, Lu, Q, and Jin, Z. Gene expression profiling of the paracrine effects of uterine natural killer cells on human endometrial epithelial cells. Int J Endocrinol. (2014) 2014:1–15. doi: 10.1155/2014/393707
124. Wang, B, Xu, T, Li, Y, Wang, W, Lyu, C, Luo, D, et al. Trophoblast H2S maintains early pregnancy via regulating maternal-fetal Interface immune hemostasis. J Clin Endocrinol Metabol. (2020) 105:4275–89. doi: 10.1210/clinem/dgaa357
125. Xu, J, Gao, D, Peng, L, Qiu, Z, Ke, L, Zhu, Y, et al. The gasotransmitter hydrogen sulfide inhibits transepithelial anion secretion of pregnant mouse endometrial epithelium. Nitric Oxide. (2019) 90:37–46. doi: 10.1016/j.niox.2019.05.011
126. Cai, S, Ye, Q, Zeng, X, Yang, G, Ye, C, Chen, M, et al. CBS and MAT2A improve methionine‐mediated DNA synthesis through SAMTOR/mTORC1/S6K1/CAD pathway during embryo implantation. Cell Prolif. (2021) 54:12950. doi: 10.1111/cpr.12950
127. Cai, S, Quan, S, Yang, G, Zeng, X, Wang, X, Ye, C, et al. DDIT3 regulates key enzymes in the methionine cycle and flux during embryonic development. J Nutr Biochem. (2023) 111:109176. doi: 10.1016/j.jnutbio.2022.109176
128. Anamthathmakula, P, Kyathanahalli, C, Ingles, J, Hassan, S, Condon, J, and Jeyasuria, P. Estrogen receptor alpha isoform ERdelta7 in myometrium modulates uterine quiescence during pregnancy. EBioMedicine. (2019) 39:520–30. doi: 10.1016/j.ebiom.2018.11.038
129. Condon, J, Kyathanahalli, C, Anamthathmakula, P, and Jeyasuria, P. Estrogen/estrogen receptor action and the pregnant myometrium. Curr Opin Physio. (2020) 13:135–40. doi: 10.1016/j.cophys.2019.10.017
130. Guerra, D, Bok, R, Breen, K, Vyas, V, Jiang, H, MacLean, K, et al. Estrogen regulates local cysteine metabolism in mouse myometrium. Reprod Sci. (2021) 28:79–90. doi: 10.1007/s43032-020-00284-6
131. Watanabe, M, Osada, J, Aratani, Y, Kluckman, K, Reddick, R, Malinow, M, et al. Mice deficient in cystathionine beta-synthase: animal models for mild and severe homocyst(e)inemia. Proc Natl Acad Sci. (1995) 92:1585–9. doi: 10.1073/pnas.92.5.1585
132. Vanaerts, L, Blom, H, Deabreu, R, Trijbels, F, Eskes, T, Peereboom-Stegeman, J, et al. Prevention of neural tube defects by and toxicity ofL-homocysteine in cultured postimplantation rat embryos. Teratology. (1994) 50:348–60. doi: 10.1002/tera.1420500506
133. Mislanova, C, Martsenyuk, O, Huppertz, B, and Obolenskaya, M. Placental markers of folate-related metabolism in preeclampsia. Reproduction. (2011) 142:467–76. doi: 10.1530/REP-10-0484
134. Akahoshi, N, Handa, H, Takemoto, R, Kamata, S, Yoshida, M, Onaka, T, et al. Preeclampsia-like features and partial lactation failure in mice lacking cystathionine γ-Lyase—an animal model of Cystathioninuria. Int J Mol Sci. (2019) 20:3507. doi: 10.3390/ijms20143507
135. Wang, K, Cai, M, Abu-Alkheir, W, Ahmad, S, and Ahmed, A. Dysregulation of placental cystathionine-β-synthase promotes fetal growth restriction. Nitric Oxide. (2015) 47:57–8. doi: 10.1016/j.niox.2015.02.138
136. Stipanuk, M. Sulfur amino acid metabolism: pathways for production and removal of homocysteine and cysteine. Annu Rev Nutr. (2004) 24:539–77. doi: 10.1146/annurev.nutr.24.012003.132418
137. Iciek, M, Bilska, A, Ksiazek, L, Srebro, Z, and Włodek, L. Allyl disulfide as donor and cyanide as acceptor of sulfane sulfur in the mouse tissues. Pharmacol Rep. (2005) 57:212–8.
138. Holwerda, K, Burke, S, Faas, M, Zsengeller, Z, Stillman, I, Kang, P, et al. Hydrogen sulfide attenuates sFlt1-induced hypertension and renal damage by upregulating Vascular endothelial growth factor. J Am Soc Nephrol. (2014) 25:717–25. doi: 10.1681/ASN.2013030291
139. Solanky, N, Requena Jimenez, A, D'Souza, S, Sibley, C, and Glazier, J. Expression of folate transporters in human placenta and implications for homocysteine metabolism. Placenta. (2010) 31:134–43. doi: 10.1016/j.placenta.2009.11.017
140. Holwerda, K, Bos, E, Rajakumar, A, Ris-Stalpers, C, van Pampus, M, Timmer, A, et al. Hydrogen sulfide producing enzymes in pregnancy and preeclampsia. Placenta. (2012) 33:518–21. doi: 10.1016/j.placenta.2012.02.014
141. Cindrova-Davies, T. The therapeutic potential of antioxidants, ER chaperones, NO and H2S donors, and statins for treatment of preeclampsia. Front Pharmacol. (2014) 5:119. doi: 10.3389/fphar.2014.00119
142. Hu, T, Wang, G, Guo, X, Sun, Q, He, P, Gu, H, et al. MiR 20a,-20b and -200c are involved in hydrogen sulfide stimulation of VEGF production in human placental trophoblasts. Placenta. (2016) 39:101–10. doi: 10.1016/j.placenta.2016.01.019
143. Chen, D, Feng, L, Hodges, J, Lechuga, T, and Zhang, H. Human trophoblast-derived hydrogen sulfide stimulates placental artery endothelial cell angiogenesis†. Biol Reprod. (2017) 97:478–89. doi: 10.1093/biolre/iox105
144. Chen, DB, and Zheng, J. Regulation of placental angiogenesis. Microcirculation. (2014) 21:15–25. doi: 10.1111/micc.12093
145. Belardinelli, M, Chabli, A, Chadefaux-Vekemans, B, and Kamoun, P. Urinary sulfur compounds in down syndrome. Clin Chem. (2001) 47:1500–1. doi: 10.1093/clinchem/47.8.1500
146. Kamoun, P. Endogenous production of hydrogen sulfide in mammals. Amino Acids. (2004) 26:243–54. doi: 10.1007/s00726-004-0072-x
147. Pinilla, J, Ayala-Ramírez, P, García-Robles, R, Olaya-C, M, and Bermúdez, M. Expression of cystathionine beta-synthase and histopathological observations in placentas of patients with down syndrome. J Neonatal-Perinatal Med. (2015) 8:77–84. doi: 10.3233/NPM-15814092
148. Oh, G, Pae, H, Lee, B, Kim, B, Kim, J, Kim, H, et al. Hydrogen sulfide inhibits nitric oxide production and nuclear factor-κB via heme oxygenase-1 expression in RAW264.7 macrophages stimulated with lipopolysaccharide. Free Radic Biol Med. (2006) 41:106–19. doi: 10.1016/j.freeradbiomed.2006.03.021
149. Moustafa, A, and Habara, Y. Hydrogen sulfide regulates ca 2+ homeostasis mediated by concomitantly produced nitric oxide via a novel synergistic pathway in exocrine pancreas. Antioxid Redox Signal. (2014) 20:747–58. doi: 10.1089/ars.2012.5108
150. Salmina, A, Komleva, Y, Szijártó, I, Gorina, Y, Lopatina, O, Gertsog, G, et al. H2S- and NO-signaling pathways in Alzheimer's amyloid vasculopathy: synergism or antagonism? Front Physiol. (2015):6. doi: 10.3389/fphys.2015.00361
151. Miyamoto, R, Koike, S, Takano, Y, Shibuya, N, Kimura, Y, Hanaoka, K, et al. Polysulfides (H2Sn) produced from the interaction of hydrogen sulfide (H2S) and nitric oxide (NO) activate TRPA1 channels. Sci Rep. (2017) 7:45995.
152. Guo, F, Li, X, Liang, D, Li, T, Zhu, P, Guo, H, et al. Active and passive demethylation of male and female pronuclear DNA in the mammalian zygote. Cell Stem Cell. (2014) 15:447–59. doi: 10.1016/j.stem.2014.08.003
153. Yang, R, Qu, C, Zhou, Y, Konkel, J, Shi, S, Liu, Y, et al. Hydrogen sulfide promotes Tet1- and Tet2-mediated Foxp3 demethylation to drive regulatory T cell differentiation and maintain immune homeostasis. Immunity. (2015) 43:251–63. doi: 10.1016/j.immuni.2015.07.017
154. Li, L, Liu, D, Bu, D, Chen, S, Wu, J, Tang, C, et al. Brg1-dependent epigenetic control of vascular smooth muscle cell proliferation by hydrogen sulfide. Biochimica et Biophysica Acta (BBA) - molecular. Cell Res. (2013) 1833:1347–55. doi: 10.1016/j.bbamcr.2013.03.002
155. Gupta, S, Mochan, S, Arora, P, Rani, N, Luthra, K, Dwivedi, S, et al. Hydrogen sulfide promotes migration of trophoblast cells by a rho GTPase mediated actin cytoskeleton reorganization. Placenta. (2023) 142:135–46. doi: 10.1016/j.placenta.2023.09.004
156. Hess, R, Niu, Y, Garrud, T, Botting, K, Ford, S, and Giussani, D. Embryonic cardioprotection by hydrogen sulphide: studies of isolated cardiac function and ischaemia‐reperfusion injury in the chicken embryo. J Physiol. (2020) 598:4197–208. doi: 10.1113/JP279978
157. Postlethwait, J, Woods, I, Ngo-Hazelett, P, Yan, Y, Kelly, P, Chu, F, et al. Zebrafish comparative genomics and the origins of vertebrate chromosomes. Genome Res. (2000) 10:1890–902. doi: 10.1101/gr.164800
158. Prabhudesai, S, Koceja, C, Dey, A, Eisa-Beygi, S, Leigh, N, Bhattacharya, R, et al. Cystathionine β-synthase is necessary for Axis development in vivo. Front Cell Dev Biol. (2018):6. doi: 10.3389/fcell.2018.00014
159. Kumai, Y, Porteus, C, Kwong, R, and Perry, S. Hydrogen sulfide inhibits Na+ uptake in larval zebrafish, Danio rerio. Pflugers Arch - Eur J Physiol. (2015) 467:651–64. doi: 10.1007/s00424-014-1550-y
160. Mitidieri, E, Vanacore, D, Turnaturi, C, Sorrentino, R, di Villa, d’E, and Bianca, R. Uterine dysfunction in diabetic mice: the role of hydrogen sulfide. Antioxidants. (2020) 9:917. doi: 10.3390/antiox9100917
161. Bhattacharyya, S, Saha, S, Giri, K, Lanza, I, Nair, K, Jennings, N, et al. Cystathionine Beta-synthase (CBS) contributes to advanced ovarian Cancer progression and drug resistance. PLoS One. (2013) 8:e79167. doi: 10.1371/journal.pone.0079167
162. Wang, L, Shi, H, Liu, Y, Zhang, W, Duan, X, Li, M, et al. Cystathionine‑γ‑lyase promotes the metastasis of breast cancer via the VEGF signaling pathway. Int J Oncol. (2019) 55:473–87. doi: 10.3892/ijo.2019.4823
163. Cindrova-Davies, T, Herrera, E, Niu, Y, Kingdom, J, Giussani, D, and Burton, G. Reduced cystathionine γ-Lyase and increased miR-21 expression are associated with increased Vascular resistance in growth-restricted pregnancies. Am J Pathol. (2013) 182:1448–58. doi: 10.1016/j.ajpath.2013.01.001
Keywords: hydrogen sulfide, female reproduction, cystathionine beta synthase, cystathionine gamma lyase, oocyte physiology, early embryo development, uterus, gravidity
Citation: Pilsova A, Pilsova Z, Klusackova B, Zelenkova N, Chmelikova E, Postlerova P and Sedmikova M (2024) Hydrogen sulfide and its role in female reproduction. Front. Vet. Sci. 11:1378435. doi: 10.3389/fvets.2024.1378435
Edited by:
Wei Cui, University of Massachusetts Amherst, United StatesReviewed by:
Mohammed Ahmed Elmetwally, Mansoura University, EgyptCaroline Gomes Lucas, University of Missouri, United States
Copyright © 2024 Pilsova, Pilsova, Klusackova, Zelenkova, Chmelikova, Postlerova and Sedmikova. This is an open-access article distributed under the terms of the Creative Commons Attribution License (CC BY). The use, distribution or reproduction in other forums is permitted, provided the original author(s) and the copyright owner(s) are credited and that the original publication in this journal is cited, in accordance with accepted academic practice. No use, distribution or reproduction is permitted which does not comply with these terms.
*Correspondence: Aneta Pilsova, cGlsc292YUBhZi5jenUuY3o=