- 1Department of Parasitology, Faculty of Medicine, Assiut University, Assiut, Egypt
- 2Department of Parasitology, School of Veterinary Medicine, Badr University in Assiut, New Nasser City, Assiut, Egypt
- 3Department of Parasitology, Faculty of Veterinary Medicine, Assiut University, Assiut, Egypt
- 4Department of Parasitology, Faculty of Veterinary Medicine, Aswan University, Aswan, Egypt
- 5New Valley Lab, Department of Parasitology, Animal Health Research Institute, Agriculture Research Center (ARC), New Valley province, Egypt
- 6Department of Hygiene and Zoonoses, Faculty of Veterinary Medicine, Mansoura University, Mansoura, Egypt
- 7Department of Biology, College of Science and Humanities in Alkharj, Prince Sattam Bin Abdulaziz University, Alkharj, Saudi Arabia
- 8Department of Zoology, Faculty of Science, Suez Canal University, El-Sheikh Zayed, Ismailia, Egypt
- 9Department of Biology, College of Science in Al-Zulfi, Majmaah University, Al-Majmaah, Saudi Arabia
- 10Department of Biology, College of Science, Princess Nourah Bint Abdulrahman University, Riyadh, Saudi Arabia
- 11Department of Natural Resources, Faculty of African Postgraduate Studies, Cairo University, Giza, Egypt
- 12Departamento de Sanidad Animal, Grupo de Investigación en Sanidad Animal y Zoonosis (GISAZ), Universidad de Córdoba, Córdoba, Spain
- 13Department of Zoonoses, Faculty of Veterinary Medicine, Sohag University, Sohag, Egypt
Introduction: Blood parasites pose a significant threat to livestock production in southern Egypt, yet there is a scarcity of information regarding their circulation and epidemiology in sheep in this region. This study aimed to investigate the seroprevalence of blood parasite infections in sheep in Assiut governorate, Upper Egypt.
Methods: A total of 400 blood samples were collected from sheep of varying ages and genders. The preliminary screening for the presence of piroplasms, mainly Babesia and Theileria spp., via microscopic examination, followed by investigation of the potential risk factors linked with the exposure to infection. Moreover, molecular identification of both parasites on some of positive samples was performed using PCR targeting Babesia 18S rRNA and Theileria annulata Tams1 gene.
Results: The microscopic examination revealed that among the examined sheep, there was an overall prevalence of blood parasites at 44% (176 out of 400), with Babesia spp. observed in 14% (56 out of 400) and Theileria spp. in 30% (120 out of 400). Furthermore, the infection rate was non-significantly higher in young animals (50%) compared to adults (38.5%) (P = 0.246). Male sheep exhibited a significantly higher vulnerability to both parasites' infection (63.3%) compared to females (35.7%) (P = 0.011). Interestingly, the prevalence of both blood parasites was significantly higher during the cold season (66.1%) compared to the hot season (15.9%) (P = <0.001). The molecular analysis identified the presence of Babesia ovis and Theileria annulata among a subsample of the positive sheep's bloods films. The identified species were recorded in the GenBank™ databases and assigned specific accession numbers (OQ360720 and OQ360719 for B. ovis), and (OP991838 for T. annulata).
Conclusions: Taken together, this study confirms a high prevalence of piroplasmosis and offers epidemiological and molecular insights into blood parasites in sheep from Upper Egypt, highlighting the importance of detecting these parasites in various hosts and their competent vectors (ticks).
1 Introduction
Sheep industry is a significant economic asset due to the animals' resilience to food shortages and weather fluctuations (1). In Egypt, sheep and goats play a vital role in providing meat to humans, along with serving as sources for wool production and utilizing their manure as a fertilizer for soil (2). Nevertheless, sheep are vulnerable to numerous haemoprotozoan diseases that can impact their health and productivity. Among various others, Theileria spp. and Babesia spp., responsible for theileriosis and babesiosis, respectively, stand out as highly economically significant tick-borne diseases transmitted to sheep (3). These haemoprotozoa are part of the broader classification belonging to the Class Sporozoa, Order Piroplasmida, and families Babesiidae and Theileriidae (4). In terms of transmission, Theileria and Babesia are biologically transmitted by hard ticks in tropical and subtropical regions globally (5). Both diseases frequently occur between May and September, coinciding with the periodic movement of ticks (6). Regarding the life cycle of Babesia (7), the organism undergoes asexual division within a mammalian red blood cell (RBC), after which the daughter merozoites rupture the host cell to infect new erythrocytes. Ticks become infected by consuming erythrocytes containing merozoites. Sexual reproduction (gametogony) takes place in the tick gut, followed by asexual sporogony in its salivary glands. Subsequently, the development of infective stages (sporozoites) occurs in the salivary glands of infected ticks. This cycle can persist throughout the animal's lifespan depending on the host's immunity. Regarding their transmission, two distinct routes of transmission are involved: transstadial transmission from one stage of the tick's life cycle to the next and transovarial transmission (8–10). The life cycle of Theileria closely resembles that of Babesia, involving sexual gametogony followed by asexual sporogony in the tick gut. Ultimately, the inoculated sporozoites invade lymphocytes in the infected host, prompting continuous division as lymphoblasts through a process known as cellular transformation. This results in parasite replication, and the infected cells become widely distributed throughout the host's lymphoid system. After 2–3 weeks, cytotoxic T-cells damage parasitized lymphoblasts. Simultaneously, the parasites initiate an attack on erythrocytes, reproducing asexually within them. Ticks become infected through the ingestion of these infected erythrocytes. Transstadial transmission is the only established mode of transmission in Theileria spp., with no evidence of discernible transovarial transmission (11). Among others, Rhipicephalus (Boophilus) annulatus and other members genus Hyalomma, such as H. marginatum and H. excavatum, are considered the most predominant ticks responsible for transmittion of piroplasmosis to sheep in Egypt (12, 13).
Ovine babesiosis is a significant tick-borne disease affecting small ruminants, caused by various species such as B. ovis, B. motasi, B. crassa, B. taylori, and B. aktasi, among others (6, 7, 10–12, 14–16). Taken into account, the most pathogenic species in sheep is B. ovis that causes malignant ovine babesiosis with fever, hemolytic anemia, hemoglobinuria, icterus and possible death others (6, 7, 10–12, 17, 18). Hemoglobinuria is uncommon, but it can occur in the advanced stages of the disease, leading to potential abortion in pregnant animals. Herds at risk can experience a significant mortality rate (19). Sheep with chronic infections typically display no symptoms, except for the presence of parasitemia and a lack of thriftiness. Recovered animals with latent infections usually possess immunity for a specific period, and there is no cross-immunity between the parasites (20). Theileriosis in small ruminants is caused by protozoan parasites of the Theileria, specifically including species such as Theileria lestoquardi, T. ovis, T. recondita, T. annulata, T. separata, T. luwenshuni, T. uilenbergi, Theileria sp. OT1 and Theileria sp. OT3 (7, 21). Common symptoms of theileriosis in sheep include cough, fever, lymphadenopathy, fatigue, and weight loss (22). Among others, T. lestoquardi is considered the most pathogenic species, leading to a malignant ovine theileriosis with a great morbidity and mortality proportion in sheep (23). Also, several previous investigations revealed that Theileria infection can result in abortion (24, 25). The diagnosis of ovine babesiosis and theileriosis can be achieved through clinical symptoms observation and microscopic examination of lymph or thin blood smears stained with Giemsa which remains the gold standard for diagnosis of the infection. However, it should be noted that relying solely on morphological characteristics for blood parasite detection (26, 27). On the other hand, molecular identification of these parasites offers greater sensitivity and specificity compared to alternative diagnostic methods (15). However, the cost of these later techniques poses a significant challenge, particularly in countries with limited resources.
It should be stressed that blood parasites pose a significant economic challenge in sheep production in Egypt, leading to losses in meat and milk production due to high parasitemia and mortality rates in infected animals (28, 29). Clearly, routine surveillance of these parasites among small ruminants in a particular area is essential for understanding the prevalence of these diseases and implementing effective control measures. While some prior studies have been conducted on ovine babesiosis and theileriosis in Egypt [Table 1; references (4, 30–32, 34–39)], there is a notable scarcity of research, especially the molecular work in the southern region of the country, namely Upper Egypt. Considering the importance of understanding factors affecting infection exposure within natural populations, it is crucial for comprehending host-parasite dynamics, predicting infection susceptibility, and maintaining biological equilibrium (40). While several factors elucidated differences in occurrence of the parasite and exposure to infection among host species, the relationship between hosts, ticks, and pathogens undergoes continuous changes, largely driven by ecological, climatic, and anthropogenic alterations. Various factors, including animal sex, age, herd management practices, seasonal variations, tick infestation, and herd size, have been associated with infection by blood parasite in animals. Additionally, spatio-temporal factors such as vector habitats, animal feeding systems, sanitation measures, and management practices significantly influence the epidemiology of these infections (41). As mentioned above, theileriosis and babesiosis are parasitic diseases of significant commercial importance, thereby playing a crucial role in the global trade of animals and animal products. The precise documentation of these parasites in Egypt is essential for understanding their epidemiology and classification, enabling effective control measures to mitigate the damage caused by their infections. Given the aforementioned details, the present study aims to investigate the prevalence and epidemiology of ovine babesiosis and theileriosis in Assiut Governorate, Upper Egypt, while also considering the potential associated risk factors. This assessment utilizes a combination of microscopic methods, followed by molecular identification of the parasites.
2 Materials and methods
2.1 Study area and sample collection
The research comprised the random sampling of 400 blood samples of sheep from small-scale stakeholders participating in veterinary campaigns conducted between February 2022 and January 2023 across different locations in Assiut Governorate, Upper Egypt (Figure 1). The climate of the studied area exhibits notable temperature extremes, with June seeing the highest monthly average at 37.1z°C, while January experiences the lowest at 4.7°C. Additionally, humidity levels fluctuate considerably, ranging from a low of 25% in May to a peak of 52% in January. The sample size was determined based on a 95% confidence level to detect exposure to the parasites, assuming a maximum individual prevalence of 52% in Egypt (30, 42). The sampled sheep encompassed both sexes and various age groups. A 5 ml blood sample was drawn from the jugular vein of each animal into sterile vacutainer tube with ethylene-diamine tetra acetic acid (EDTA) (BD Vacutainer®, Franklin Lakes, NJ, USA, EUA). All the samples were refrigerated at 4–8°C. Once in the laboratory, we proceeded the preparation of blood films for examination under an ordinary microscope.
2.2 Microscopic examination
To prepare thin blood films, a drop of blood was placed on one end of a clean slide, and a spreader was slid at a 45° angle to touch the blood sample. The spreader was then gently and firmly moved, allowing the blood to follow behind and form a feathered edge. Subsequently, the thin film was air-dried, fixed in methanol for 5 min, and stained with a freshly prepared 10% Giemsa stain for 30 min. After staining, the film was washed with water and left to dry. Finally, the blood film was examined under a light microscope at × 100 (oil immersion) to detect blood parasites (43). The infected erythrocytes containing piroplasms or schizonts were identified as described in the literature (44, 45).
2.3 Molecular detection
2.3.1 DNA extraction
The genomic DNA extraction was performed from some blood samples (n = 40), which tested positive for each parasite during microscopic examination, indicating a higher concentration of parasites per field, using a commercial DNA isolation kit (QIAamp DNA Mini Kit, 51304) following the manufacturer's instructions.
2.3.2 PCR amplification and phylogenetic analysis
The amplification of Babesia through 18S rRNA gene and Theileria (Tams1 gene) species was conducted following established protocols from previous studies (34, 46, 47). Preparation of PCR Master Mix for cPCR according to Emerald Amp GT PCR master mix (Takara), Code No. RR310Akit. The reaction mixture (25 μL) included 12.5 μL of Emerald Amp GT PCR mastermix (2x premix) (Code No. RR310A), 0.2 μM of each primer, 5 μL of DNA template and Millipore water was added to achieve a final volume of 25 μL. The set of primers and temperature and time conditions during PCR are shown in Supplementary Tables 1, 2 (48, 49), respectively. PCR amplicons were examined through 1.5% agarose gel electrophoresis, and for each 100 ml gel, 6 μL of GreenSafe stain (10 mg/mL) was applied. Additionally, a 100 bp ladder was utilized as a reference to identify target amplicon sizes. Gel documentation system captured images of the gel, and the data were subsequently analyzed using computer software. PCR products from some of the positive samples exhibited distinct and sharp bands were purified using the QIAquick PCR Product Extraction Kit (ID: 51304) from Qiagen, Valencia. For the sequencing reaction, the BigDye Terminator V3.1 Cycle Sequencing Kit from Perkinelmer was employed, followed by purification using a Centrisep spin column. PCR products with positive amplifications were purified and sequenced using the Applied Biosystems 3130 Genetic Analyzer. To achieve species-level identity, a score of >99% identity was considered using both BLASTn (https://blast.ncbi.nlm.nih.gov/Blast.cgi; Mega-BLASTn option) (34, 50). Multiple alignments were carried out with MAFFT version 7 (51). For maximum likelihood (ML) phylogenetic analyses, the choice of the best-fitting evolutionary model was based on those defined using JModeltest2 on the basis of the Akaike information criterion (51). Tree reconstruction was carried out with Mega 11 (52). The evolutionary history was deduced using the maximum likelihood method and the Tamura 3-parameter model (53). The percentage of trees in which the associated taxa clustered together is shown next to the branches. The tree is drawn to scale, with branch lengths measured in the number of substitutions per site. The phylogenetic trees were manipulated for display using FigTree v.1.4.2 (54).
2.4 Statistical analysis
The data collected in this study was analyzed using the Statistical Package for Social Sciences (SPSS) software program, version 26. The chi-square test was employed to examine and compare qualitative variables. Quantitative measures were expressed as means ± standard deviation (SD) and Median (Interquartile range). The prevalences were estimated as the ratio of positives from the total number of samples analyses, with the exact binomial confidence intervals of 95% (95% CI) based on the score method (55). Logistic regression analyses were conducted to evaluate both unadjusted and adjusted odds ratios (OR) along with their corresponding 95% confidence intervals (95% CI) to identify factors associated with infection. A significance level of P < 0.05 was considered significant, while P < 0.01 was deemed highly significant.
3 Results
3.1 Prevalence of blood parasites in surveyed sheep and associated risk factors
In the present work, out of the 400 blood samples examined by microscopic examination, 176 tested positive for blood parasites, resulting in an overall prevalence of 44%. The individual infection rates for Babesia spp. and Theileria spp. were 14% and 30%, respectively. Clinically the positive sheep were anemic, emaciated, having lacrimation, decreased appetite, and temperature may be normal or slightly increased. Furthermore, corneal opacity, enlargement of lymph nodes, and hemoglobinuria were recorded in some cases. In terms of age as potential risk factor, which is shown in Table 2, the overall prevalence of detected blood parasites was higher in young animals (50%) compared to adults (38.5%), although a significant association was not found (OR: 160; P-value: 0.246). As depicted in Table 2, the infection rate of Babesia spp. was 14% (16.7% in animals younger than 2 years and 11.5% in animals older than 2 years), while for Theileria spp., it was 30% (33.3% in animals younger than 2 years and 26.9% in animals older than 2 years). Concerning sex (Table 2), the overall prevalence of blood parasites in males was 63.3% (OR: 3.11, P = 0.01) which was higher than in females 35.7%. The significance of these findings is highlighted by the results observed in Babesia, revealing an odds ratio (OR) of 8.25 between males and females (Table 2). In contrast, Table 2 showed that Theileria had an OR of 0.46, indicating a different pattern of association between gender and infection rates in these two parasites. Table 2 also depicts a significant relationship between blood parasitic infection in relation to sex (P = 0.011). In terms of seasonality, a significant association was identified between blood parasitic infections and the seasons (P < 0.01) (OR: 10.29; P < 0.001) (Table 2). This pattern was notably consistent during the cold season for both Babesia spp. and Theileria spp., with odds ratios of 5.73 and 6.29, respectively (Table 2).
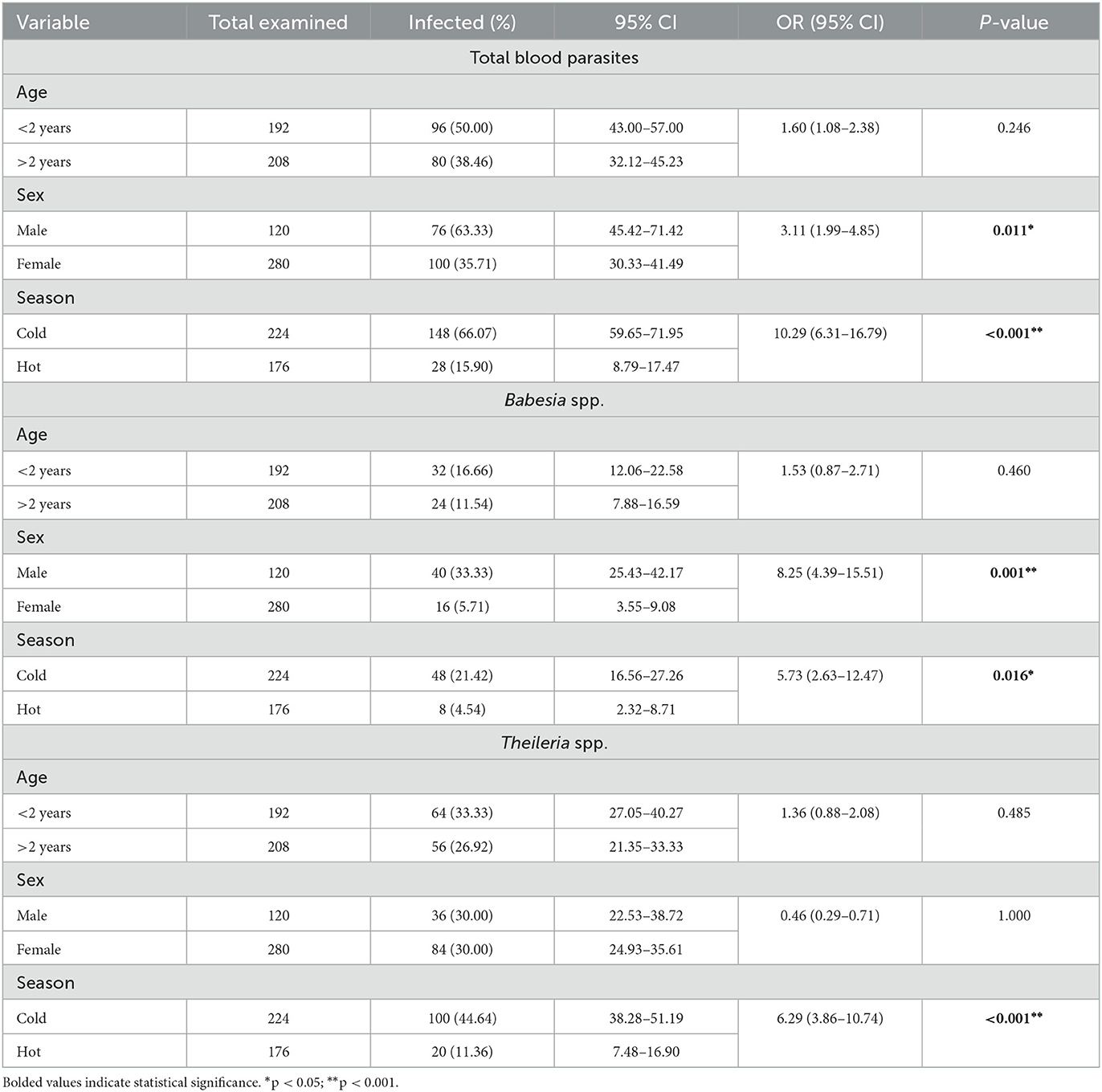
Table 2. Distribution of the overall prevalence of blood parasites, including Babesia and Theileria, infections according to the age group, sex, and sampling season of the surveyed sheep population (n = 400).
3.2 Morphological characteristics of blood parasites detected in sheep
Giemsa-stained blood smears of Babesia spp. showed erythrocytic stages in which intra-erythrocytic piroplasms are rounded or double pear-shaped typically located at the periphery of the infected host erythrocytes (Figure 2). Meanwhile, Giemsa-stained blood smears of Theileria spp. show erythrocytic stages, merozoites, schizonts and multiple microshizonts, which were intra-erythrocyte piroplasms of the small rod-shaped, ring, and rounded shaped forms present within lymphocytes (Figure 3). The infected red blood cells (RBCs) also displayed echinocyte-like protrusions on their surface, a common feature observed during Theileria infection.
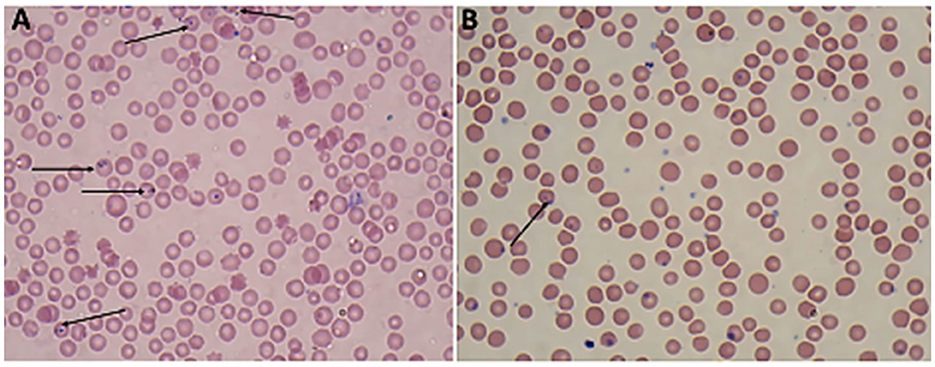
Figure 2. Thin blood film stained with Giemsa magnified × 100 (oil immersion lens) showing the blood protozoan parasites found in sheep (A, B): paired Trophozoites of Babesia spp. (arrow).
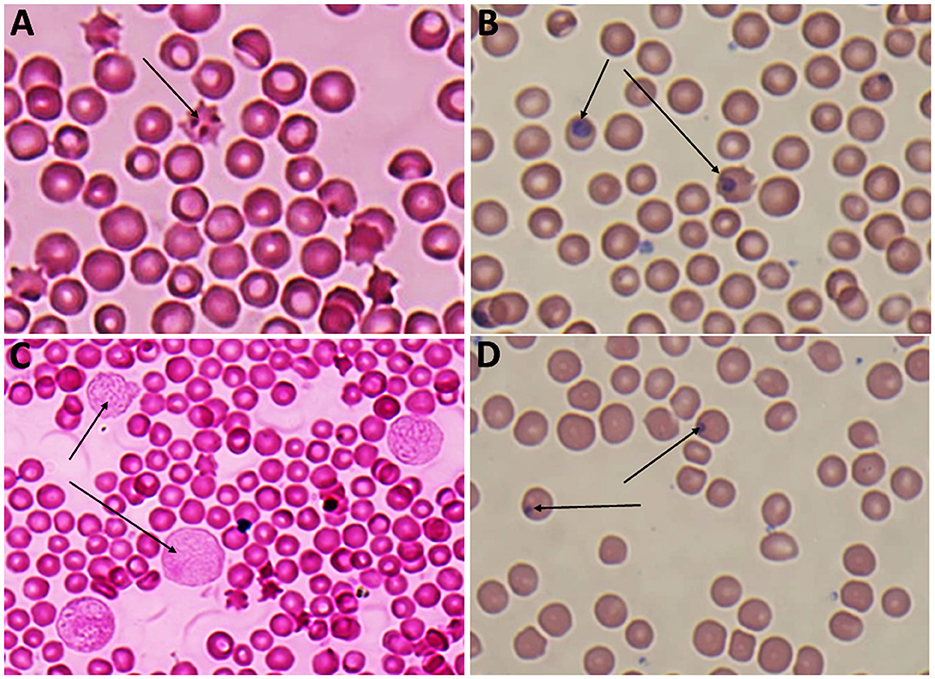
Figure 3. (A) Echincyte containing trophozoites of Theileria spp., x100 (oil immersion lens), (B) ring stage of Theileria spp., x100 (oil immersion lens), (C) multiple microshizonts of Theileria spp. x100 (oil immersion lens) (arrow), (D) small rod shape trophozoite of Theileria spp., x100 (oil immersion lens) (thin arrow), rounded shape trophozoite of Theileria spp., x 100 (oil immersion lens) (thick arrow).
3.3 PCR analysis
The molecular analysis of the 18S rRNA gene confirmed the presence of B. ovis (OQ360720 and OQ360719) with 100% homology to other sequences available in GenBank™. Using the Tams1 gene for Theileria, the presence of T. annulata (OP991838) was also confirmed with 100% homology to other sequences accessible in GenBank™. Additionally, the phylogenetic results of this study showed that the presence of B. ovis sequences clearly formed a single monophyletic cluster with other sequences of the same species, as depicted in Figures 4. On the other hand, the obtained sequence OP991838 in the present research is clearly differentiated from the rest of the finalized species (T. parva, T. yokoyama and T. lestoquardi) and integrated into the T. annulata cluster (Figure 5). The analysis of intraspecies genetic diversity revealed intriguing findings. For B. ovis, the 18S rRNA gene displayed a relatively low level of divergence among sequences (Figure 6). However, the Tams1 gene for T. annulata species exhibited notable intergenetic variability, surpassing the sequences found worldwide, as depicted in Figure 7.
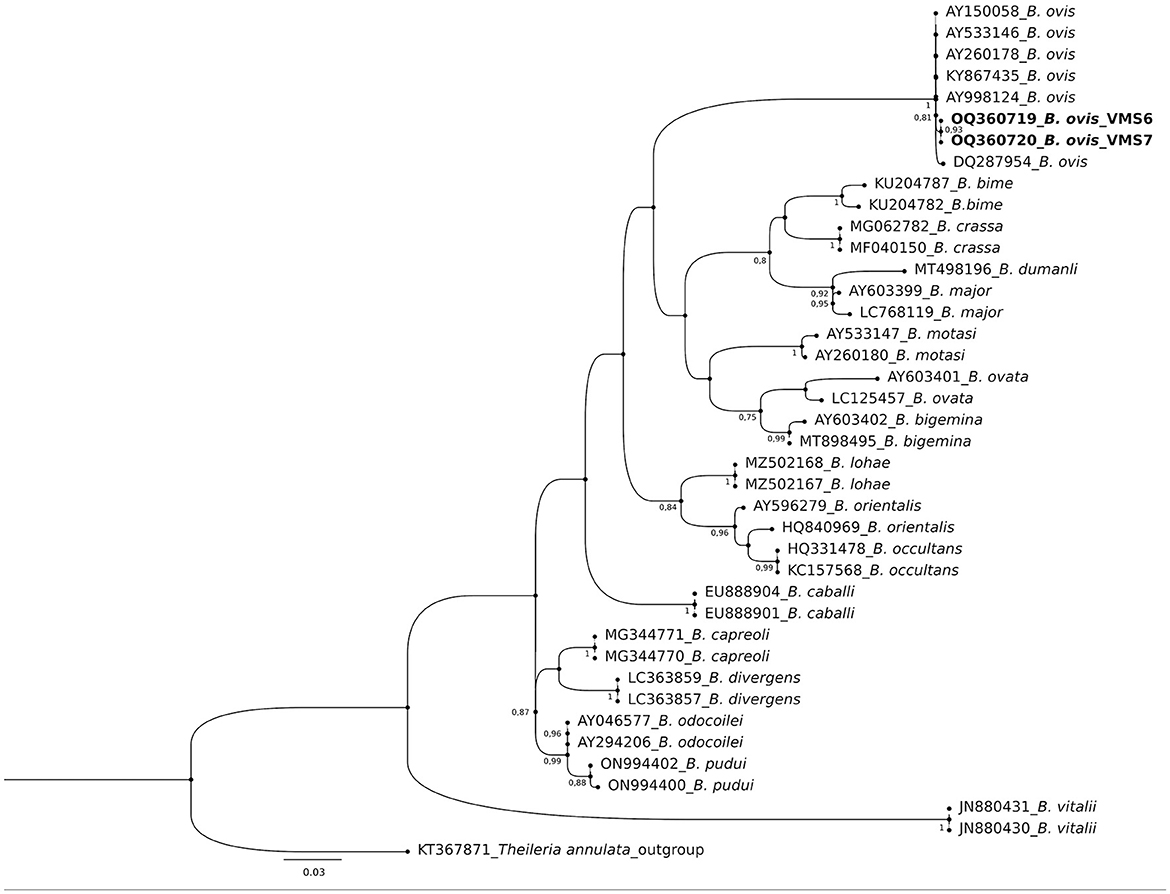
Figure 4. Phylogenetic relationships of Babesia ovis were inferred based on the analysis of 40 nucleotide 18S rRNA gene sequences using the Maximum Likelihood method and Kimura 2-parameter model. The tree with the highest log likelihood (−1656.70) is shown. A discrete Gamma distribution was used to model evolutionary rate differences among sites [5 categories (+G, parameter = 0.3354)]. The rate variation model allowed for some sites to be evolutionarily invariable ([+I], 51.51% sites). Bootstrap values >75% in 1000 repetitions are indicated at specific branch nodes. The bar indicates the average number of substitutions per site. The tree was rooted using Theileria annulata (KT367871) as the outgroup. Sequences obtained during this study are shown in boldface.
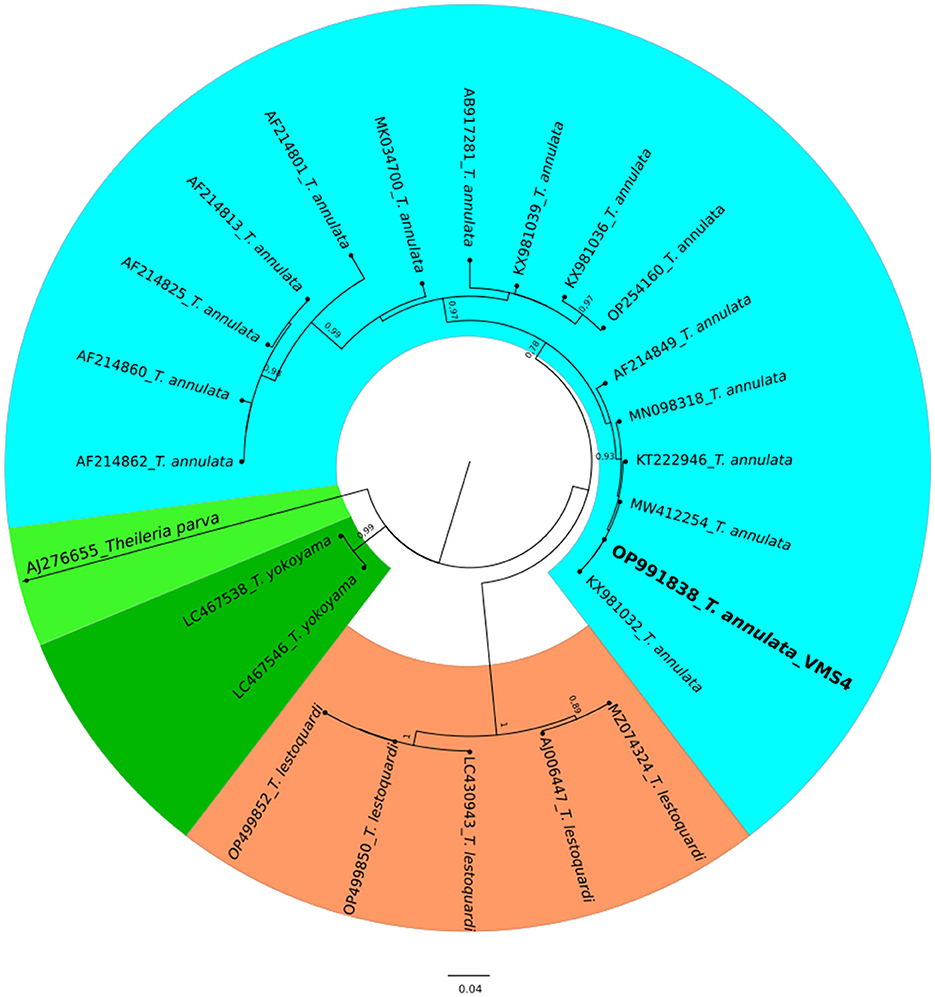
Figure 5. A circular phylogenetic tree of Theileria annulata based on the analysis of 24 nucleotide tams1 gene sequences using the Maximum Likelihood method and the Tamura 3-parameter model. The tree depicts the highest log likelihood (−10113.12). Evolutionary rate differences among sites were modeled using a discrete Gamma distribution with 5 categories (+G, parameter = 0.4271). Bootstrap values greater than 75% in 1000 repetitions are indicated at specific branch nodes. The bar represents the average number of substitutions per site. Sequences obtained during this study are highlighted in boldface.
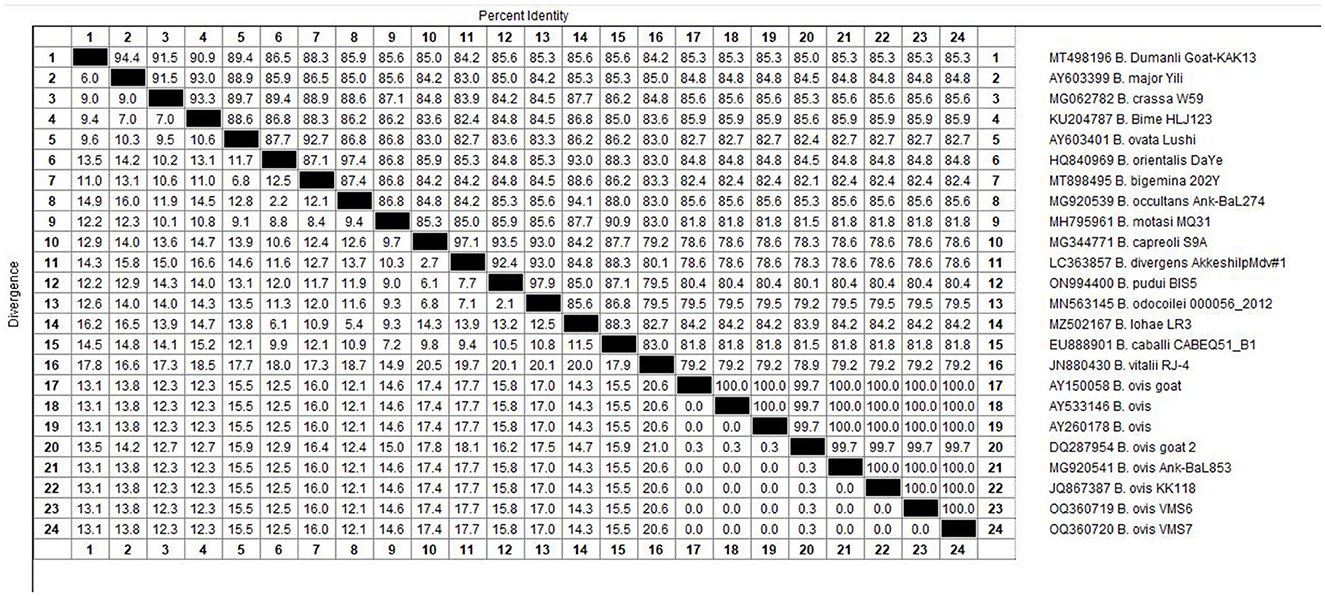
Figure 6. The percentages of identity for the studied isolates of B. ovis in sheep related to other isolates worldwide depend on B. ovis 18S rRNA gene.
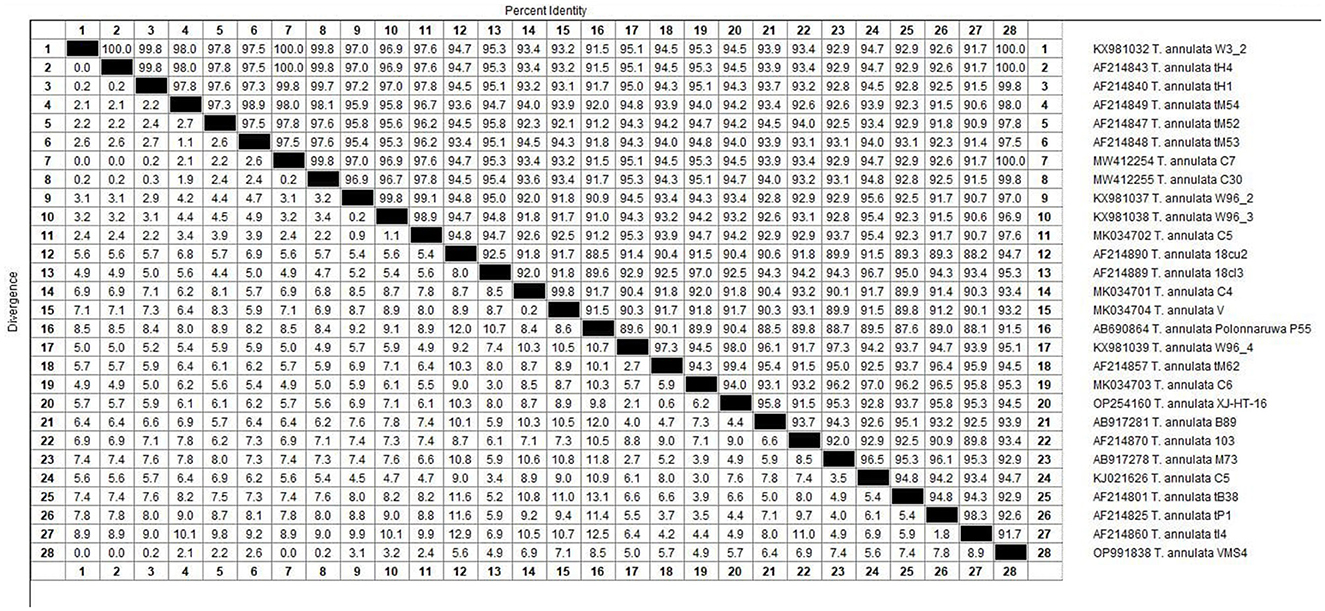
Figure 7. The percentages of identity for the studied isolates of Theileria annulata in sheep related to other isolates worldwide depend on Theileria annulata tams1 gene.
4 Discussion
Piroplasmid infection poses a significant threat to sheep populations globally. Considering their veterinary significance and substantial economic implications, understanding the epidemiological pattern of piroplasm's infection is essential for implementing targeted control strategies, including tick control measures, vaccination programs, and improved management practices to reduce the burden of the disease in sheep populations. The current study delves into the exposure and epidemiology of these parasites specifically among sheep populations from Upper Egypt, together with molecular characterization of both parasites. In addition, the study reviewed the prevalence data of Babesia spp. and Theileria spp. among sheep in Egypt (Table 1). As illustrated, the current study revealed that 176 out of the tested samples tested positive for piroplasmosis using microscopical detection methods, resulting in an overall prevalence of 44%. At national level, a previous study conducted by Hussein et al. (31) in Qena governorate, Upper Egypt, reported an overall prevalence of blood parasites at 38.46% which is slightly lower than our present findings. Conversely, another previous research (33) in the North Coast of Egypt showed a higher prevalence rate of blood parasites (84.80%), surpassing the present reported findings. Regarding the individual prevalence rates reported, the infection rates detected for Babesia spp. and Theileria spp. were 14% and 30%, respectively. A previous study conducted (4) in Behera governorate (Lower Egypt) reported prevalence rates of 17% for Babesia and 20% for Theileria, respectively. Another previous study (29) documented presence of Babesia spp., with prevalence rates of 23.7%, 23.1%, 21.2%, and 20.6% in the governorates of Beni Suef, Kafr Elsheikh, El-Fayoum, and El-Sharkia, respectively, which surpasses the prevalence rates observed in the current study. Conversely, another study conducted on sheep from Kalubyia governorate (Lower Egypt) revealed a lower incidence of 8.5% for Babesia spp. (28). A previous research (56) conducted on sheep from Egypt reported a higher prevalence rate of 50.7% for Theileria spp. by Giemsa stained blood film (24–26, 38, 46, 48, 49). Variations in infection prevalence rates may stem from the intricate interactions of factors such as the presence of infected ticks, sanitary and hygienic practices, procedural techniques, immunological status of infected animals, and the absence of veterinary supervision (34, 57, 58). Abiotic factors, particularly temperature and humidity, have a significant impact on the epidemiology of these infections (59). The climatic conditions in the studied area are characterized by mean temperatures throughout the year ranging from 18°C to 40°C, establishing an optimal habitat for the enduring presence of hard ticks. This environment not only facilitates the proliferation of their hosts and the spread of associated blood parasites but also likely contributes to the elevated prevalence rates (60, 61). Concerning the reported clinical signs, the infected sheep exhibited symptoms such as anorexia, anemia, and emaciation. Additionally, hemoglobinuria recorded in sheep infected with Babesia spp. Those infected with Theileria spp. displayed additional clinical signs such as lacrimation, corneal opacity, and enlarged lymph nodes, consistent with observations reported in the literature (22).
Concerning age, which was explored as a potential risk factor associated with the exposure to those parasites, this study unveiled that the overall prevalence of blood parasites in sheep was non-significantly higher in young animals under 2 years, compared to adult ones over 2 years. In this concern, the study found that Babesia spp. infection rates were higher in animals under 2 years compared to those over 2 years, while Theileria spp. infection rates were also more prevalent in the younger age group. However, the statistical difference were not significant. In a prior study by Fadly, 2012 (4) in Behera, Upper Egypt, a higher prevalence of both Babesia spp. and Theileria spp. was higher in sheep above 3 years of age, which contrasts with our findings. Furthermore, Elsayed et al. (33) in North Coast of Egypt concluded that the infection with blood parasites was higher in sheep more than 3 years. Another study (62) in Egypt recorded a higher infection rate in animals aged ≤ 1 year. The discrepancies observed between the current findings and other studies could be attributed to variations in sanitary and hygienic practices, the number of examined animals, variations in immunological resistance, and the impact of local climatic conditions, which may influence the spread of ticks (32).
Regarding sex as a studied individual variable factor, this study found that the overall prevalence of blood parasites in male sheep was significantly higher compared to females, which aligns with earlier studies conducted nationally or internationally (63–66). However, the present findings are in contrast with another reports in Egypt (31, 33, 62). This variation may stem from a notable difference in the sample size of males and females included in our study which could influence the prevalence rates (67). Another contributing factor is management practices in Egypt, where farms typically have few breeding males but a high percentage of young male lambs (68) and the increased susceptibility to infection among females grazing together outdoors (31). It has been demonstrated that the contact of healthy livestock with infected livestock during free grazing is a critical risk factor for increasing the burden and spread of Theileria infection (69–71). Furthermore, other previous reports revealed higher occurrence of blood parasites in female as compared to males due to various physiological factors such as pregnancy, parturition, and milk production, may experience increased stress, rendering them more susceptible to infection than males (72). In addition, farm management, microclimatic patterns, tick distribution, host breed, and sampling conditions may account for the variation in prevalence rates of tick-borne pathogens (73, 74). In this study, the prevalence of blood parasites was significantly higher in the cold season 66.1% than in the hot season 15.9%. The infection rate of Theileria spp. and Babesia spp. was higher in cold season (44.6% and 21.4%, respectively) than in hot season (11.4% and 4.5% respectively). Similarly, another previous study detected higher prevalence of blood parasites in winter when compared to summer (63). The obtained result disagreed with those data recorded (4) in Behera governorate (Lower Egypt), who reported higher infection rate in summer months as compared to winter season. Other studies have documented that there were no differences in the prevalence rate of blood parasites across the analyzed seasons (36). This may be attributed to the presence of ticks and can be explained by the hypothesis that the average temperatures of the studied area during cold months, which are known to provide favorable conditions for the rapid proliferation of ticks and facilitate their easy transmission among susceptible animals (75). These variations can be attributed to geographic and climatic conditions, vector activity, the parasitic status of the investigated area, and the immunological status of the examined animals (4, 76). In this regard, the studied area provides a conducive environment for the development of hard ticks (39). Specifically, the average temperatures in this region during winter might reach 21°C (77, 78), providing optimal conditions for the year-round spread of host vectors and accompanying blood parasites (60, 61).
Regarding the phylogenetic analysis, this study unequivocally identified two bloods parasites species, B. ovis through 18S rRNA gene and T. annulata by tams1 gene. According to previous studies, a conserved gene of 18S rRNA is a specific target for Babesia and Theileria species detection by using PCR assay (12, 79, 80), being a sensitive diagnostic tool for ovine piroplasmids. On the other hand, the Tams1 gene has proven to be a tool to detect new related Theileria species (81). Concerning Theileria, while blood smear examination remains the primary method for diagnosing, it falls short in species differentiation due to morphological similarities (82). In this work, PCR technique allowed us to identify T. annulata in this study. Similarly, a previous work (32) in Egypt detected various Theilaria species (T. ovis, T. annulata and T. lestoquardi). Experimental infections of sheep and goats with T. annulata, the causative agent of tropical theileriosis in cattle, have been documented to induce mild symptoms without piroplasm development. However, previous findings suggest that T. annulata can cause mild clinical symptoms in sheep post-experimental infection, and T. lestoquardi can similarly affect cattle (83). Precisely, T. lestoquardi is considered the primary pathogenic species responsible for malignant ovine theileriosis (84, 85), with many positive samples likely attributed to this species taking into account its mix detection with other species in different studies (9, 76, 86). Nonetheless, another study (87) also identified natural infections of sheep with various species of Theileria, known as T. lestoquardi, T. ovis, and T. annulate, by nested PCR-RFLP. Both infections are transmitted by the same vector, H. anatolicum, which is found in Egypt (76) and in many areas, cattle and sheep are raised together, potentially leading to cross-infestation (88, 89). Due to logistical constraints, our study was unable to conduct molecular determinations on all samples. The findings reported here raise the possibility of sheep acting as reservoirs for T. annulata, provided both parasites and a competent vector coexist in the same area. Despite this challenge, this study is considered one of the few molecular reports in Egypt confirming B. ovis, although confirmation could not be achieved in all samples, highlighting the need for further studies in this area to accurately determine the prevalence of this species in Egypt.
The current study faces several methodological limitations that necessitate careful consideration when interpreting the findings. Initially, screening for both blood parasites involved examining only a single blood film sample per animal using microscopy. While this method remains the gold standard technique for large-scale screening of piroplasmosis, particularly in resource-constrained settings, its accuracy in detection may be compromised, potentially leading to an underestimation of the true infection rate. Secondly, the cross-sectional design of the study is inadequate for monitoring the progression of piroplasmosis and for capturing seasonal variations in infection rates. Thirdly, the findings obtained may not be generalizable to other epidemiological contexts or geographic regions within the same country. Finally, the relatively limited number of samples subjected to molecular characterization may have impacted the precision of estimating the true genetic diversity and frequency of parasite species circulating among the examined sheep.
5 Conclusions
The current study has provided significant insights into the prevalence and impact of Babesia and Theileria, reaffirming their ongoing importance as blood parasites affecting Egyptian sheep in upper Egypt. This investigation emphasizes the critical role of utilizing both traditional microscopy and cutting-edge molecular methods for the precise detection and characterization of piroplasmosis. Given the above-mentioned findings, of particular note is the apparent rise in the circulation of piroplasmosis, a trend likely worsened by the expanding populations of the tick vectors. Furthermore, the study sheds light on the current epidemiological landscape of piroplasmosis in the Egyptian environment. By addressing the spread of these parasites, informed actions can be taken to mitigate the associated diseases, safeguarding the health and wellbeing of both livestock and the population at large. These findings serve as a call to action, emphasizing the importance of ongoing surveillance and strategic interventions to manage and minimize the impact of Babesia and Theileria infections in Egypt. This underscores the urgent necessity for additional investigations centered on these vectors, signaling a critical domain for future research endeavors and intervention initiatives. Further studies with larger sample sizes, diverse small ruminants, and additional epidemiological data are also necessary to comprehensively evaluate the associated risk factors for infection. Such knowledge would be helpful for governmental authorities to develop and implement effective control measures.
Data availability statement
The original contributions presented in the study are publicly available. This data can be found at: https://www.ncbi.nlm.nih.gov/nuccore/; OP984151.1.
Ethics statement
The animal studies were approved by Research Ethics Committee of the Faculty of Veterinary Medicine, Assiut University, under the designation 06/2023/0130. The studies were conducted in accordance with the local legislation and institutional requirements. Written informed consent was obtained from the owners for the participation of their animals in this study.
Author contributions
AD: Investigation, Methodology, Project administration, Supervision, Validation, Visualization, Writing—original draft, Writing—review & editing. FA-A: Conceptualization, Data curation, Formal analysis, Investigation, Methodology, Software, Validation, Writing—original draft, Writing—review & editing. SM: Conceptualization, Methodology, Software, Supervision, Validation, Visualization, Writing—original draft, Writing—review & editing. AG: Conceptualization, Data curation, Formal analysis, Investigation, Software, Supervision, Validation, Visualization, Writing—original draft, Writing—review & editing. FO: Conceptualization, Data curation, Investigation, Methodology, Software, Supervision, Validation, Visualization, Writing—original draft, Writing—review & editing. FE: Data curation, Formal analysis, Investigation, Software, Validation, Writing—review & editing. EH: Data curation, Formal analysis, Software, Validation, Visualization, Writing—review & editing. NA: Conceptualization, Data curation, Formal analysis, Software, Validation, Writing—review & editing. HA: Data curation, Formal analysis, Funding acquisition, Investigation, Software, Validation, Writing—review & editing. AA: Data curation, Formal analysis, Software, Validation, Writing—review & editing. DB-B: Data curation, Formal analysis, Software, Writing—review & editing. EE: Conceptualization, Data curation, Formal analysis, Funding acquisition, Investigation, Methodology, Resources, Validation, Writing—original draft, Writing—review & editing.
Funding
The author(s) declare financial support was received for the research, authorship, and/or publication of this article. This study was supported by Princess Nourah Bint Abdulrahman University Researchers Supporting Project No. (PNURSP2024R401), Princess Nourah Bint Abdulrahman University, Riyadh, Saudi Arabia. EE received support through a postdoctoral fellowship from the María Zambrano Program at the University of Córdoba, funded by the Program of Requalification of the Spanish University System, sponsored by the Spanish Ministry of Universities and financed by the European Union-NextGenerationEU.
Conflict of interest
The authors declare that the research was conducted in the absence of any commercial or financial relationships that could be construed as a potential conflict of interest.
The reviewer MR declared a shared affiliation with the author FE to the handling editor at the time of review.
Publisher's note
All claims expressed in this article are solely those of the authors and do not necessarily represent those of their affiliated organizations, or those of the publisher, the editors and the reviewers. Any product that may be evaluated in this article, or claim that may be made by its manufacturer, is not guaranteed or endorsed by the publisher.
Supplementary material
The Supplementary Material for this article can be found online at: https://www.frontiersin.org/articles/10.3389/fvets.2024.1373842/full#supplementary-material
References
1. Tanveer M, Farooq M, Amjad M, Asif M, Kashif M, Latif M, et al. Molecular prevalence, associated risk factors and phylogeny of Anaplasma marginale, Theileria ovis and T. lestoquardi in sheep from Pakistan. Comp Immunol Microbiol Infect Dis. (2022) 86:101822. doi: 10.1016/j.cimid.2022.101822
2. Abdelazeem AG, Dyab AK, Mohamed YM. Helminthic infection in digestive system of goats in slaughterhouse, Manfalout, Assiut governorate, Egypt. J Egypt Soc Parasitol. (2020) 50:649–55. doi: 10.21608/jesp.2020.131101
3. Aouadi A, Leulmi H, Boucheikhchoukh M, Benakhla A, Raoult D, Parola P. Molecular evidence of tick-borne hemoprotozoan-parasites (Theileria ovis and Babesia ovis) and bacteria in ticks and blood from small ruminants in Northern Algeria. Comp Immunol Microbiol Infect Dis. (2017) 50:34–9. doi: 10.1016/j.cimid.2016.11.008
4. Fadly SR. Prevalence of blood parasites of some farm animals at Behera province. Assiut Vet Med J. (2012) 58:1–7. doi: 10.21608/avmj.2012.173780
5. Uilenberg G. Babesia—A historical overview. Vet Parasitol. (2006) 138:3–10. doi: 10.1016/j.vetpar.2006.01.035
6. Sayin F, Karaer Z, Dincer S, Cakmak A, Inci A, Yukari BA, et al. A comparison of susceptibilities to infection of four species of Hyalomma ticks with Theileria annulata. Vet Parasitol. (2003) 113:115–21. doi: 10.1016/S0304-4017(03)00045-1
7. Schnittger L, Ganzinelli S, Bhoora R, Omondi D, Nijhof AM, Florin-Christensen M. The Piroplasmida Babesia, Cytauxzoon, and Theileria in farm and companion animals: species compilation, molecular phylogeny, and evolutionary insights. Parasitol Res. (2022) 121:1207–45. doi: 10.1007/s00436-022-07424-8
8. Scott I. Principles of veterinary parasitology, Dennis Jacobs, Mark Fox, Lynda Gibbons, Carlos Hermosilla. Vet Parasitol. (2016) 220:12. doi: 10.1016/j.vetpar.2016.02.012
9. Villanueva-Saz S, Borobia M, Fernández A, Jiménez C, Yzuel A, Verde MT, et al. Anaemia in sheep caused by Babesia and Theileria Haemoparasites. Animals (Basel). (2022) 12:341. doi: 10.3390/ani12233341
10. Ravindran R, Hembram PK, Kumar GS, Kumar KGA, Deepa CK, Varghese A. Transovarial transmission of pathogenic protozoa and rickettsial organisms in ticks. Parasitol Res. (2023) 122:691–704. doi: 10.1007/s00436-023-07792-9
11. Almazán C, Scimeca RC, Reichard M V, Mosqueda J. Babesiosis and Theileriosis in North America. Pathogens. (2022) 11:168. doi: 10.3390/pathogens11020168
12. El Imam AH, Hassan SM, Gameel AA, El Hussein AM, Taha KM, Oosthuizen MC. Molecular identification of different Theileria and Babesia species infecting sheep in Sudan. Ann Parasitol. (2016) 62:47–54. doi: 10.17420/ap6201.31
13. Friedhoff KT. Tick-borne diseases of sheep and goats caused by Babesia, Theileria or Anaplasma spp. Parassitologia. (1997) 39:99–109.
14. Kirman R, Guven E. Molecular detection of Babesia and Theileria species/genotypes in sheep and ixodid ticks in Erzurum, Northeastern Turkey: First report of Babesia canis in sheep. Res Vet Sci. (2023) 157:40–9. doi: 10.1016/j.rvsc.2023.02.012
15. Schnittger L, Yin H, Qi B, Gubbels MJ, Beyer D, Niemann S, et al. Simultaneous detection and differentiation of Theileria and Babesia parasites infecting small ruminants by reverse line blotting. Parasitol Res. (2004) 92:189–96. doi: 10.1007/s00436-003-0980-9
16. Schnittger L, Yin H, Gubbels MJ, Beyer D, Niemann S, Jongejan F, et al. Phylogeny of sheep and goat Theileria and Babesia parasites. Parasitol Res. (2003) 91:398–406. doi: 10.1007/s00436-003-0979-2
17. Stevanović O, Radalj A, Subić I, Jovanović NM, Sladojević Ž, Amović M, et al. The presence of malignant ovine babesiosis in Bosnia and Herzegovina indicates a possible emerging risk for Balkan region. Comp Immunol Microbiol Infect Dis.(2022) 90–91:101893. doi: 10.1016/j.cimid.2022.101893
18. Zhou M, Cao S, Sevinc F, Sevinc M, Ceylan O, Ekici S, et al. Molecular detection and genetic characterization of Babesia, Theileria and Anaplasma amongst apparently healthy sheep and goats in the central region of Turkey. Ticks Tick Borne Dis. (2017) 8:246–52. doi: 10.1016/j.ttbdis.2016.11.006
19. Zangana IK, Naqid IA. Prevalence of piroplasmosis (Theileriosis and Babesiosis) among goats in Duhok Governorate. Al-Anbar J Vet Sci. (2011) 4:50–7.
20. Ijaz M, Rehman A, Ali MM, Umair M, Khalid S, Mehmood K, et al. Clinico-epidemiology and therapeutical trials on babesiosis in sheep and goats in Lahore, Pakistan. The J Anim Plant Sci. (2013) 23:666–9.
21. Stuen S. Haemoparasites-challenging and wasting infections in small ruminants: a review. Animals. (2020) 10:2179. doi: 10.3390/ani10112179
22. Hassan MA, Raoofi A, Lotfollahzadeh S, Javanbakht J. Clinical and cytological characteristics and prognostic implications on sheep and goat Theileria infection in north of Iran. J Parasit Dis. (2015) 39:190–3. doi: 10.1007/s12639-013-0318-1
23. Naz S, Maqbool A, Ahmed S, Ashraf K, Ahmed N, Saeed K, et al. Prevalence of theileriosis in small ruminants in Lahore-Pakistan. J Vet Anim Sci. (2012) 2:16–20.
24. Harfoush MA. Studies on Some Parasitic Diseases in Sheep (Ph.D Thesis). Tanta: Tanta University, Faculty of Veterinary Medicine, Animal Diseases Department (1998).
25. Forshaw D, Alex SM, Palmer DG, Cotter J, Roberts WD, Jenkins C, et al. Theileria orientalis Ikeda genotype infection associated with anaemia, abortion and death in beef cattle in Western Australia. Aust Vet J. (2020) 98:290–7. doi: 10.1111/avj.12937
26. Criado-Fornelio A, Buling A, Pingret JL, Etievant M, Boucraut-Baralon C, Alongi A, et al. Hemoprotozoa of domestic animals in France: prevalence and molecular characterization. Vet Parasitol. (2009) 159:73–6. doi: 10.1016/j.vetpar.2008.10.012
27. Hassan S, Skilton RA, Pelle R, Odongo D, Bishop RP, Ahmed J, et al. Assessment of the prevalence of Theileria lestoquardi in sheep from the Sudan using serological and molecular methods. Prev Vet Med. (2019) 169:104697. doi: 10.1016/j.prevetmed.2019.104697
28. Ramadan MY, El-Akabawy ML. Studies on sheep Babesiosis in Kalubyia governorate, Egypt. Minufiya Vet J. (2000) 1:147–55.
29. Mahmoud MS. Some Serological Studies on Babesia Species Infecting Sheep in Egypt (M.V. Sc. Thesis). Giza: Cairo University (1992).
30. El-Seify MA, Mahran OM ABD EL AAL AMI. Epidemiological studies on hard ticks and tick borne parasites, in Shalatin City, red sea governorate, Egypt. Assiut Vet Med J. (2011) 57:1–28. doi: 10.21608/avmj.2011.176883
31. Hussein NM, Mohammed ES, Hassan AA, El-Dakhly KM. Distribution pattern of Babesia and Theileria species in Sheep in Qena Province, Upper Egypt. Arch Parasitol. (2017) 1:1–4.
32. Hegab AA, Fahmy MM, Mahdy OA, Wahba A. Parasitological and molecular identification of Theileria species by PCR-RFLP Method in Sheep, Egypt. Int J Adv Res Biol Sci. (2016) 3:48–55. Available online at: http://s-o-i.org/1.15/ijarbs-2016-3-7-7
33. Elsayed M-AA, Bessat MN, Ezzat SF, Felefel WI. The prevalence and associated infection risk factors of the blood protozoan parasites among the small ruminants in the north coast of Egypt. Matrouh J Vet Med. (2021) 1:19–23. doi: 10.21608/mjvm.2021.231054
34. Barghash SM. Molecular prevalence and phylogeny of some tick-borne parasites in ruminants in Sinai Peninsula, Egypt. Eur J Biomed Pharm Sci. (2022) 9:15–25.
35. Abdelsalam MA, Felefel W, Fadl S, Bessat M. Molecular prevalence and associated infection risk factors of tick-borne protozoan and rickettsial blood pathogens in small ruminants. BMC Vet Res. (2023) 19:138. doi: 10.1186/s12917-023-03702-4
36. Hosein HI, Ahmed SA, Ibrahim FA, Abou-Elnaga TR, Gebely MA, Mahmoud MA. Seroprevalence of Babesia ovis in small ruminants in Siwa Oasis, Egypt. Journal of Veterinary Medical Research. (2007) 17:19–24. doi: 10.21608/jvmr.2007.77888
37. Elsify A, Sivakumar T, Nayel M, Salama A, Elkhtam A, Rizk M, et al. An epidemiological survey of bovine Babesia and Theileria parasites in cattle, buffaloes, and sheep in Egypt. Parasitol Int. (2015) 64:79–85. doi: 10.1016/j.parint.2014.10.002
38. Eliwa M, Mahran KMA, Mousa WA, Hagag N, Shaalan MI, Bashandy MM. Ovine theileriosis: clinical, pathological and molecular investigations. Adv Anim Vet Sci. (2021) 9:462–72. doi: 10.17582/journal.aavs/2021/9.4.462.472
39. Al-Hosary AA, ElSify A, Salama AA, Nayel M, Elkhtam A, Elmajdoub LO, et al. Phylogenetic study of Theileria ovis and Theileria lestoquardi in sheep from Egypt: Molecular evidence and genetic characterization. Vet World. (2021) 14:634–9. doi: 10.14202/vetworld.2021.634-639
40. Smith KF, Acevedo-Whitehouse K, Pedersen AB. The role of infectious diseases in biological conservation. Anim Conserv. (2009) 12:1–12. doi: 10.1111/j.1469-1795.2008.00228.x
41. Kocan KM, Busby AT, Allison RW, Breshears MA, Coburn L, Galindo RC, et al. Sheep experimentally infected with a human isolate of Anaplasma phagocytophilum serve as a host for infection of Ixodes scapularis ticks. Ticks Tick Borne Dis. (2012) 3:147–53. doi: 10.1016/j.ttbdis.2012.01.004
43. Abdullah DA, Ali MS, Omer SG, Ola-Fadunsin SD, Ali FF, Gimba FI. Prevalence and climatic influence on hemoparasites of cattle and sheep in Mosul, Iraq. J Adv Vet Anim Res. (2019) 6:492. doi: 10.5455/javar.2019.f373
44. Levine ND. Protozoan Parasites of Domestic Animals and of Man, 1st Edn. Iowa: Iowa State University Press (1985). p. 414.
45. Bell-Sakyi L, Koney EBM, Dogbey O, Walker AR. Incidence and prevalence of tick-borne haemoparasites in domestic ruminants in Ghana. Vet Parasitol. (2004) 124:25–42. doi: 10.1016/j.vetpar.2004.05.027
46. Aydin MF, Aktas M, Dumanli N. Molecular identification of Theileria and Babesia in ticks collected from sheep and goats in the Black Sea region of Turkey. Parasitol Res. (2015) 114:65–9. doi: 10.1007/s00436-014-4160-x
47. Kumar B, Maharana BR, Thakre B, Brahmbhatt NN, Joseph JP. 18S rRNA gene-based piroplasmid PCR: an assay for rapid and precise molecular screening of Theileria and Babesia species in animals. Acta Parasitol. (2022) 67:1697–707. doi: 10.1007/s11686-022-00625-2
48. Nourollahi-Fard SR, Khalili M, Ghalekhani N. Detection of Theileria annulata in blood samples of native cattle by PCR and smear method in Southeast of Iran. J Parasit Dis. (2015) 39:249–52. doi: 10.1007/s12639-013-0333-2
49. Benson DA, Karsch-Mizrachi I, Lipman DJ, Ostell J, Rapp BA, Wheeler DL. GenBank. Nucleic Acids Res. (2002) 30:17–20. doi: 10.1093/nar/30.1.17
50. Altschul SF, Gish W, Miller W, Myers EW, Lipman DJ. Basic local alignment search tool. J Mol Biol. (1990) 215:403–10. doi: 10.1016/S0022-2836(05)80360-2
51. Katoh K, Standley DM. MAFFT multiple sequence alignment software version 7: improvements in performance and usability. Mol Biol Evol. (2013) 30:772–80. doi: 10.1093/molbev/mst010
52. Tamura K, Stecher G, Kumar S. MEGA11: molecular evolutionary genetics analysis version 11. Mol Biol Evol. (2021) 38:3022–7. doi: 10.1093/molbev/msab120
53. Nei M, Kumar S. Molecular Evolution and Phylogenetics. New York, NY: Oxford University Press (2000).
54. Rambaut A. FigTree v1.4.4. (2018). Available online at: http://tree.bio.ed.ac.uk/software/figtree/ (accessed April 10, 2024).
55. Wilson EB. Probable inference, the law of succession, and statistical inference. J Am Stat Assoc. (1927) 22:209–12. doi: 10.1080/01621459.1927.10502953
56. Ibrahim MS, El Seify MA, Hafez AM, Deghidy NS, Harfoush MA. Studies on Blood Parasites in Sheep. In: The 1st Scientific Congress for Provincial Laboratonries. (2000). p. 15–17.
57. Ferrer D, Castellà J, Gutiérrez JF. Seroprevalence of Babesia ovis in sheep in Catalonia, northeastern Spain. Vet Parasitol. (1998) 79:275–81. doi: 10.1016/S0304-4017(98)00175-7
58. Ziapour SP, Esfandiari B, Youssefi MR. Study of prevalence of Babesiosis in domesticated animals with suspected signs in Mazandaran province, North of Iran, during 2008. J Anim Vet Adv. (2011) 10:712–4. doi: 10.3923/javaa.2011.712.714
59. Foughali AA, Jedidi M, Dhibi M, Mhadhbi M, Sassi L, Berber A, et al. Infection by haemopathogens and tick infestation of sheep during summer season in Constantine region, Northeast Algeria. Vet Med Sci. (2021) 7:1769–77. doi: 10.1002/vms3.551
60. Fieler AM, Rosendale AJ, Farrow DW, Dunlevy MD, Davies B, Oyen K, et al. Larval thermal characteristics of multiple ixodid ticks HHS public access author manuscript. Comp Biochem Physiol A Mol Integr Physiol. (2021) 257:110939. doi: 10.1016/j.cbpa.2021.110939
61. Cable J, Barber I, Boag B, Ellison AR, Morgan ER, Murray K, et al. Global change, parasite transmission and disease control: lessons from ecology. Philos Trans R Soc Lond B Biol Sci. (2017) 372:88. doi: 10.1098/rstb.2016.0088
62. Abdel-Shafy S, Abdullah HHAM, Elbayoumy MK, Elsawy BSM, Hassan MR, Mahmoud MS, et al. Molecular epidemiological investigation of Piroplasms and Anaplasmataceae bacteria in Egyptian domestic animals and associated ticks. Pathogens. (2022) 11:1194. doi: 10.3390/pathogens11101194
63. Magzoub A, El Ghali A, Hussien MO, Juma Y, Mohammed SB. Prevalence of ticks (Acari: Ixodidae) and Theileria lestoquardi in sheep at El Huda and El Nuhud animals production research stations, Sudan. J Parasit Dis. (2021) 45:146. doi: 10.1007/s12639-020-01284-8
64. Osman T, Ali A, Hussein M, Ghali E, Salih D. Investigation on Theileria lestoquardi infection among sheep and goats in Nyala, South Darfur State, Sudan. Insights Vet Sci. (2017) 1:17–23. doi: 10.29328/journal.hvsr.1001002
65. Taha HA, Shoman SA, Alhadlag NM. Molecular and serological survey of some haemoprotozoan, rickettsial and viral diseases of small ruminants from Al-Madinah Al Munawarah, KSA. Trop Biomed. (2015) 32:511–23.
66. Iqbal F, Khattak R, Ozubek S, Khattak M, Rasul A, Aktas M. Application of the reverse line blot assay for the molecular detection of Theileria and Babesia sp. in Sheep and Goat blood samples from Pakistan Iran. J Parasitol. (2013) 8:289–95.
67. Miller IF, Schneider-Crease I, Nunn CL, Muehlenbein MP. Estimating infection prevalence: best practices and their theoretical underpinnings. Ecol Evol. (2018) 8:6738. doi: 10.1002/ece3.4179
68. Abd-Allah S. Assessment of the village sheep production systems under the prevalent management practices in the Nile Delta of Egypt. Egypt J Vet Sci. (2024) 55:517–30. doi: 10.21608/ejvs.2023.230657.1570
69. Swai ES, Karimuribo ED, Kambarage DM, Moshy WE, Mbise AN. A comparison of seroprevalence and risk factors for Theileria parva and T. mutans in smallholder dairy cattle in the Tanga and Iringa regions of Tanzania. Vet J. (2007) 174:390–6. doi: 10.1016/j.tvjl.2006.08.004
70. El-Ashker M, Hotzel H, Gwida M, El-Beskawy M, Silaghi C, Tomaso H. Molecular biological identification of Babesia, Theileria, and Anaplasma species in cattle in Egypt using PCR assays, gene sequence analysis and a novel DNA microarray. Vet Parasitol. (2015) 207:329–34. doi: 10.1016/j.vetpar.2014.12.025
71. Ullah K, Numan M, Alouffi A, Almutairi MM, Zahid H, Khan M, et al. Molecular characterization and assessment of risk factors associated with Theileria annulata Infection. Microorganisms. (2022) 10:1614. doi: 10.3390/microorganisms10081614
72. Fereig RM, Mohamed SGA, Mahmoud H, AbouLaila MR, Guswanto A, Nguyen TT, et al. Seroprevalence of Babesia bovis, B. bigemina, Trypanosoma evansi, and Anaplasma marginale antibodies in cattle in southern Egypt Ticks Tick. Borne Dis. (2017) 8:125–31. doi: 10.1016/j.ttbdis.2016.10.008
73. Cuervo PF, Artigas P, Lorenzo-Morales J, Bargues MD, Mas-Coma S. Ecological niche modelling approaches: challenges and applications in vector-borne diseases. Trop Med Infect Dis. (2023) 8:187. doi: 10.3390/tropicalmed8040187
74. Lihou K, Rose Vineer H, Wall R. Distribution and prevalence of ticks and tick-borne disease on sheep and cattle farms in Great Britain. Parasit Vectors. (2020) 13:406. doi: 10.1186/s13071-020-04287-9
75. Voyiatzaki C, Papailia SI, Venetikou MS, Pouris J, Tsoumani ME, Papageorgiou EG. Climate changes exacerbate the spread of Ixodes ricinus and the occurrence of Lyme borreliosis and tick-borne encephalitis in Europe—how climate models are used as a risk assessment approach for tick-borne diseases. Int J Environ Res Public Health. (2022) 19:6516. doi: 10.3390/ijerph19116516
76. Yakhchali M, Hosseine A. Prevalence and ectoparasites fauna of sheep and goats flocks in Urmia suburb, Iran. Vet Arhiv. (2006) 76:431–42.
77. Gelaro R, McCarty W, Suárez MJ, Todling R, Molod A, Takacs L, et al. The modern-era retrospective analysis for research and applications, version 2 (MERRA-2). J Clim. (2017) 30:5419–54. doi: 10.1175/JCLI-D-16-0758.1
78. San Francisco US Cedar LV Average Weather for TNorwayA. (2024). Available online at: https://weatherspark.com/y/84211/Average-W-T-N-Y-RoundAA, Weatherspark.com (accessed April 10, 2024).
79. Mans BJ, Pienaar R, Latif AA. A review of Theileria diagnostics and epidemiology. Int J Parasitol Parasites Wildl. (2015) 4:104–18. doi: 10.1016/j.ijppaw.2014.12.006
80. Tuli A, Singla L, Sharma A, Bal M, Filia G. Molecular epidemiology, risk factors and hematochemical alterations induced by Theileria annulata in bovines of Punjab (India). Acta Parasitol. (2015) 60:378–90. doi: 10.1515/ap-2015-0053
81. Sivakumar T, Fujita S, Tuvshintulga B, Kothalawala H, Silva SSP, Yokoyama N. Discovery of a new Theileria sp. closely related to Theileria annulata in cattle from Sri Lanka. Sci Rep. (2019) 9:1–10. doi: 10.1038/s41598-019-52512-y
82. Salih DA, El Hussein AM, Singla LD. Diagnostic approaches for tick-borne haemoparasitic diseases in livestock. J Vet Med Anim Health. (2015) 7:45–56. doi: 10.5897/JVMAH2014.0345
83. Taha KM, Salih DA, Ali AM, Omer RA, El Hussein AM. Naturally occurring infections of cattle with Theileria lestoquardi and sheep with Theileria annulata in the Sudan. Vet Parasitol. (2013) 191:143–5. doi: 10.1016/j.vetpar.2012.08.003
84. Luo J, Yin H. Theileriosis of sheep and goats in China. Trop Anim Health Prod. (1997) 29:2907. doi: 10.1007/BF02632907
86. Hashemi-Fesharki R. Tick-borne diseases of sheep and goats and their related vectors in Iran. Parassitologia. (1997) 39:115–7.
87. Zaeemi M, Haddadzadeh H, Khazraiinia P, Kazemi B, Bandehpour M. Identification of different Theileria species (Theileria lestoquardi, Theileria ovis, and Theileria annulata) in naturally infected sheep using nested PCR-RFLP. Parasitol Res. (2011) 108:837–43. doi: 10.1007/s00436-010-2119-0
88. Leemans I, Brown D, Hooshmand-Rad P, Kirvar E, Uggla A. Infectivity and cross-immunity studies of Theileria lestoquardi and Theileria annulata in sheep and cattle: I In vivo responses. Vet Parasitol. (1999) 82:179–92. doi: 10.1016/S0304-4017(99)00013-8
Keywords: sheep, piroplasm, Babesia, Theileria, microscopic, molecular, prevalence, Egypt
Citation: Dyab AK, Mohamed SA-A, Abdel-Aziz FM, Gareh A, Osman F, Elgohary FA, Hassan EA, Alsowayeh N, Alzaylaee H, Ahmed AA-RS, Bravo-Barriga D and Elmahallawy EK (2024) Microscopic and molecular detection of piroplasms among sheep in Upper Egypt. Front. Vet. Sci. 11:1373842. doi: 10.3389/fvets.2024.1373842
Received: 20 January 2024; Accepted: 07 May 2024;
Published: 27 May 2024.
Edited by:
Vikrant Sudan, Guru Angad Dev Veterinary and Animal Sciences University, IndiaReviewed by:
Mohamed Abdo Rizk, Mansoura University, EgyptKashif Hussain, University of Agriculture, Faisalabad, Pakistan
Copyright © 2024 Dyab, Mohamed, Abdel-Aziz, Gareh, Osman, Elgohary, Hassan, Alsowayeh, Alzaylaee, Ahmed, Bravo-Barriga and Elmahallawy. This is an open-access article distributed under the terms of the Creative Commons Attribution License (CC BY). The use, distribution or reproduction in other forums is permitted, provided the original author(s) and the copyright owner(s) are credited and that the original publication in this journal is cited, in accordance with accepted academic practice. No use, distribution or reproduction is permitted which does not comply with these terms.
*Correspondence: Ehab Kotb Elmahallawy, sa2elele@uco.es
†These authors have contributed equally to this work