- 1REQUIMTE, LAQV, ICBAS, School of Medicine and Biomedical Sciences, University of Porto, Porto, Portugal
- 2SORGAL, Sociedade de Óleos e Rações, S.A., Lugar da Pardala, S. João Ovar, Portugal
- 3SEBOL, Comércio e Indústria de Sebo, S.A., Santo Antão do Tojal, Portugal
- 4Indústria Transformadora de Subprodutos, S.A., Herdade da Palmeira—Olheiros do Meio—São José da Lamarosa Agolada Coruche, Coruche, Portugal
The increased fish consumption by the growing human population in the world translates into an increase in fish waste. The reintroduction of these fish by-products into food and feed chains presents economic benefits and contributes to counteracting their negative environmental impact. Under this context, the present study aimed to evaluate the effects of the dietary inclusion of fish hydrolysate and oil obtained from fish waste (experimental diet) in substitution of shrimp hydrolysate and salmon oil (control diet) mainly imported from third countries on palatability, apparent total tract digestibility, fecal characteristics and metabolites, blood fatty acid profile, flatulence, and coat quality of adult dogs. A two-bowl test was performed to evaluate palatability by the pairwise comparison between the two diets. A feeding trial was conducted according to a crossover design with two diets (control and experimental diets), six adult Beagle dogs per diet, and two periods of 6 weeks each. The replacement of shrimp hydrolysate and salmon oil with fish hydrolysate and oil did not affect the first diet approach and taste, as well as the intake ratio. Generally, the digestibility of dry matter, nutrients, and energy was not affected by diet, but the intake of digestible crude protein (CP) and ether extract was higher, respectively, with the control and the experimental diet. The higher intake of eicosapentaenoic acid and docosahexaenoic acid with the experimental diet was reflected in a higher content of these long-chain polyunsaturated fatty acids and the omega-3 index of red blood cells, but it did not affect coat quality. The significantly higher intake of digestible CP with the control diet might have contributed to the higher fecal ammonia-N and valerate concentrations. Daily fecal output and characteristics were similar between diets. Overall, results suggest that fish hydrolysate and oil from the agrifood industry might constitute sustainable functional ingredients for dog feeding while adding value for wild fisheries, aquaculture, and fish farming under a circular economy approach and reducing dependence on imports from third countries with a high carbon footprint.
1 Introduction
In the last decades, the increase in the world human population, which is increasingly consuming more fish and fish products due to their role as part of a healthy diet, has been the main driver for the growth in total fisheries and aquaculture (1). Indeed, recent estimates set an expected world fish production of 201 million tons by 2030, comprising an overall increase of 12.8% from 2018 to 2020, with an increasing share from aquaculture (2). The observed increase in fish consumption is translated into an increase in fish waste, being the majority of lost or unused biomass (e.g., skin, bones, heads, viscera, and trimmings) found during filleting and preparation of products for the final consumer (3). However, these by-products have the potential to originate high-value products that can be reintroduced into food and feed chains due to their richness in nutrients and bioactive compounds, such as proteins, bioactive peptides, polyunsaturated fatty acids (PUFAs), minerals, and enzymes (4, 5). Taking advantage of fish by-products has not only economic benefits but also counteracts the negative environmental impact of improper fish waste disposal, namely through the release of organic compounds into aquatic environments (5, 6). Indeed, the valorization of fish by-products is commonly considered the preferred option to manage fish waste, whereas when important pollutants are present, waste management processes should determine the fate of fish by-products (7). The application of the circular economy concept to the fishery field increases the rate of reuse and decreases the underutilization of fish waste, thus contributing to a reduction of the pressure on natural resources (3, 8), which is aligned with the Circular Economy Action Plan (9) launched by the European Union as part of the European Green Deal. Protein hydrolysates and oils rich in omega-3 PUFA, such as eicosapentaenoic (C20:5 n-3, EPA) and docosahexaenoic (C22:6 n-3, DHA) acids, are among the most common functional feed ingredients obtained from fish by- and co-products (BCPs) with great interest, among others, in companion animal feeding due to the current trends observed in this sector (6). Indeed, along with a growing worldwide pet population (10), owners are increasingly favoring sustainable functional ingredients that not only ensure energy and nutrient requirements but also benefit the health and wellbeing of their animals, contributing to a decrease in the risk of chronic disease development and an increase in life expectancy (11).
In this context, in vitro and in vivo studies, namely with dogs, have shown that protein hydrolysates provide amino acids more easily absorbed in the small intestine (12), with numerous biological activities such as hypoallergenic (13), anti-stress (14, 15), satiety (16), antihypertensive, antithrombotic, immunomodulatory, and antioxidative effects (17), as well as acting as palatability enhancers (13, 18). However, despite the high interest in protein hydrolysates and their use in commercial diets, studies on companion animals are scarce, especially with fish hydrolysates.
Regarding the long-chain omega-3 PUFA, EPA and DHA are daily recommended for fully-grown (19), growing, and reproductive dogs (19–21), being also considered to play a role in disease prevention and used in several therapeutic diets (e.g., cardiovascular, renal, gastrointestinal, orthopedic, and dermatological) at higher doses (22–26). Fish oil is considered the primary source of EPA and DHA (27), but due to sustainability reasons and also taking into consideration the Sustainable Development Goal of the United Nations Agenda 2030 to prevent overfishing, alternative sources of fish oil obtained from farmed and wild fish are required. Although several marine sources [e.g., microalgae, macroalgae, krill, copepods, single-cell organisms; (28, 29)] and genetically modified plant oilseeds [e.g., rapeseed, thale cress, and camelina; (30–33)] have been evaluated as EPA and DHA sources, the extraction of oil from fish waste originated from the processing stage might constitute a sustainable approach that also has the advantage of being able to be applied locally, contributing to a lower environmental impact. Therefore, the present study aimed to evaluate the effects of the inclusion of locally produced fish hydrolysate and oil obtained from fish waste in the substitution of imported shrimp hydrolysate (mainly from Ecuador, the major producer of shrimp) and salmon oil (mainly from the North of Europe, Norway, and Scotland, the main producers of salmon), on palatability, apparent total tract digestibility, fecal characteristics and metabolites, blood fatty acid profile, and coat quality of adult dogs.
2 Materials and methods
Trials were approved by the Animal Ethics Committee of the School of Medicine and Biomedical Sciences, University of Porto, and licensed by the Portuguese General Directorate of Food and Veterinary Medicine (Permit No 0421/000/000/2021). Trained scientists in laboratory animal science (FELASA, category C) conducted the experiments in line with good animal welfare practices (European Union Directive 2010/63/EU).
2.1 Animals and housing
Twelve healthy adult Beagle dogs (2 intact males, 4 neutered males, and 6 spayed females), 5.4 ± 0.57 years old, weighing 11.8 ± 2.20 kg with a body condition score of 4.3 ± 0.69 (assessed according to a 9-point scale; (34)), were used in the experimental trials. Dogs were clinically examined before the start of the trials to ensure their suitability to participate in the studies. At the kennel of the School of Medicine and Biomedical Sciences, University of Porto, animals were housed in pairs in environmentally enriched and communicating boxes comprising an interior and an exterior area of 1.8 and 3.5 m2, respectively, with sliding doors for their individual feeding. Animals were allowed to exercise and socialize in an outdoor park area under supervision and were leash-walked once a day for at least 30 min. To ensure the collection of individual feces, animals were housed individually during the collection period, and supervised access to the outdoor park was maintained between daily meals.
2.2 Diets and feeding
Fish hydrolysate and oil were supplied by a company group (Empresa Transformadora de Subprodutos Animais S.A., ETSA, Loures, Portugal) dedicated to recycling in the food sector and were obtained from fish by-products comprising heads, tails, skin, slices, and whole fish mainly of salmon, sea bream, sea bass, and redfish. These category 3 animal by-products are, according to Regulation European Commission 1069/2009 (35), identified as the lowest risk group since they come from healthy slaughtered animals and, despite not being fit for human consumption, present no risk to animals or the environment. Enzymatic hydrolysis was carried out on minced fish by-products with the non-specific serine endopeptidase from Bacillus licheniformis Alcalase 2.4 L (Novozymes®, Bagsvaerd, Denmark) in a ratio enzyme/substrate of 0.2%, during 4 h at 65 ± 5°C at pH ± 8, ensured by adjustment with 1 N NaOH. Once completion of the hydrolysis, heat treatment was applied at approximately 90°C for 10 to 15 min to deactivate the enzyme, and the liquid was filtered through a 450 μm sieve (200 mm diameter, VWR International, Oregon, United States) to separate residues such as scales and bones that had not been hydrolyzed from the aqueous fraction. The hydrolyzed liquid was centrifuged for 15 min at 5,394 × g at room temperature in a Gyrozen 1,248 centrifuge (Gyrozen® Co., Ltd., South Korea) to remove impurities, resulting in three layers: (i) the lipid fraction, from which fish oil was obtained; (ii) the mineral fraction; and (iii) the protein fraction that was further concentrated (four times) in a KNF RC900 Rotary Evaporator (KNF®, Breisgau, Germany). After this concentration phase, the protein hydrolysate was centrifuged again for 5 min at 5,394 × g at room temperature to ensure that it was free of possible suspended solids (such as the presence of some minerals and residues) and then subjected to the spray drying process (Mini Spray Dryer B-290, Büchi, Switzerland) at 130°C, 95% aspiration, and 13% system flow rate.
A commercial diet for adult medium-size dogs (Sorgal Pet Food, Ovar, Portugal) with the inclusion of 5% shrimp hydrolysate (Symrise Aqua, Equator) and 3% salmon oil (Symrise Aqua, Norway) was used as the control diet. An experimental diet was formulated by Sorgal (Ovar, Portugal) according to the current formulation matrixes to be iso-energetic, iso-lipidic, and iso-nitrogenous, using the same ingredients of the control diet, with slight adjustments on wheat grain (6.0 and 7.5% for the control and experimental diet), pea concentrate (7.0 and 5.0%), and poultry fat (5.0 and 5.3%), and the replacement of 5% shrimp hydrolysate with 5% fish hydrolysate, and 3% of salmon oil with 3.2% of fish oil.
Animals were individually fed the daily ration in two equal meals, at 8:30 a.m. and 17:00 p.m., with free access to fresh water. Both control and experimental diets were fed as an extruded kibble. Daily food allowance was calculated according to the body condition score (34) and the ideal body weight to meet the metabolizable energy (ME) requirements (19).
2.3 Palatability trial
A two-bowl test (36) was performed to evaluate palatability by pairwise comparison between the two diets. Animals (n = 12) were given the choice between the two diets in two different bowls (45 cm apart) on both meals for two consecutive days. The sample size and the duration of the trial were defined according to an earlier study (37) that found significant differences in intake ratio among diets without or with the inclusion of shrimp hydrolysate. The position of the bowls was switched between meals to eliminate any bowl-placement bias. The amount of food offered in each bowl was calculated as half of the daily ration needed to ensure the ME requirements of dogs (19). Diet first approached and diet first tasted were recorded. The test ended after 30 min or until animals consumed all the food available in one bowl. To calculate the intake ratio of both diets, food offered and food residues were weighed.
2.4 Feeding trial design and sample collection
Dogs were divided into two groups, blocked for sex and whether the males were intact or neutered, and received the control and experimental diets in two consecutive experimental periods following a crossover arrangement (six dogs per diet and period, following the recommendation of FEDIAF (19) for digestibility trials). Each experimental period lasted for 6 weeks. Daily food intake was recorded throughout the trial. Dogs were weighed, and their body condition was assessed every 2 weeks to adjust the daily food allowance. Measurements and sample collection were performed in the last week of each period. Dogs were subjected to a physical examination, including urinalysis, performed on urine samples collected directly into sterilized containers by voluntary urination before the morning meal. On one day of the last week of each period, blood was collected from each dog via jugular into clot activator gel tubes (EDTA BD Vacutainer; VWR International, Carnaxide, Portugal) for the analysis of the fatty acid profile of red blood cells (RBCs). Dogs were fasted overnight prior to blood collection but had free access to water at all times. In 5 days of the last week of each experimental week, total feces excreted by dogs were collected to determine the apparent total tract digestibility of dry matter (DM), nutrients, energy, and dietary ME content. During these days, the number of defecations was recorded every day, and individual fresh samples, collected within 15 min after defecation, were scored using a 5-point scale from 1 (watery diarrhea) to 5 (powdery hard mass pellets) (38). Samples were weighed, mixed, subsampled at different locations, and immediately frozen at −20°C in individual containers for later analysis of the chemical composition, fecal pH, ammonia-N, and volatile fatty acid concentrations carried out in mixed feces composited by period and dog.
2.5 Analytical procedures
2.5.1 Diets and feces
Diets and fecal samples were dried in a forced-air oven at 65°C until constant weight. Ground (1 mm) diet and feces samples were analyzed in duplicate according to official methods (39), determining the contents of DM (ID 934.01), ash (ID 942.05), Kjeldahl nitrogen (N) (in dried diet samples and fresh feces samples; ID 990.03), and ether extract (EE, ID 920.39). Crude protein (CP) was calculated as Kjeldahl N × 6.25. For diet and feces samples, neutral detergent fiber (with α-amylase and without sodium sulfite, NDF) was determined and expressed exclusive of residual ash (40), starch content was analyzed in 0.5 mm samples according to Salomonsson and Theander (41), and gross energy (GE) was determined using an adiabatic bomb calorimeter (Werke C2000, IKA, Staufen, Germany).
Fatty acids of the control and experimental diets were converted to fatty acid methyl esters by acid-catalyzed transesterification with methanolic HCl (42) and analyzed by gas chromatography, as reported by Maia, Fonseca (43). Nonadecanoic acid (Matreya LLC, Pleasant Gap, PA) was used as an internal standard, and the identification of fatty acids was performed by comparing retention times to commercially available standards (Supelco 37 Component FAME Mix, PUFA No.1, PUFA No.2, PUFA No.3, Sigma-Aldrich Co. LLC, St. Louis, MO, United States of America).
For amino acid analysis, determined as described by Aragão, Cabano (44), samples of the control and experimental diets were hydrolyzed with 6 M HCL solution at 116°C for 48 h and precolumn derivatized with Waters AccQ Fluor Reagent (6-aminoquinolyl-N-hydroxysuccinimidyl carbamate) flowing the AccQ Tag method (Waters, Milford, MA). Analyses were performed in ultra-high-performance liquid chromatography on a Waters reversed-phase amino acid analysis system. Norvaline was used as an internal standard, and the resulting peaks were analyzed with EMPOWER software (Waters).
2.5.2 Urine
Color and turbidity were evaluated as recommended by Alleman and Wamsley (45). Urinalysis was performed with a dipstick, and pH and density, respectively, determined with a potentiometer (pH and Ion-Meter GLP 22, Crison, Barcelona, Spain) and a refractometer (URIVET hand refractometer, HPM003, Zuzi).
2.5.3 Blood
RBCs were separated from plasma by centrifugation at 3,000 rpm for 15 min at 4°C and fatty acids transesterified as previously described by Harris, Pottala (46). The resulting fatty acid methyl esters were analyzed by gas chromatography using a Shimadzu GC-2010 Plus (Shimadzu Corporation, Kyoto, Japan) equipped with a capillary column (SP-2560, 100 m × 0.25 mm × 0.20 μm; Supelco, Bellefonte, PA, United States of America) and a flame-ionization detector. The oven’s initial temperature of 50°C was held for 1 min, then increased at a rate of 50°C/min to 150°C and held for 20 min, then increased at 1°C/min to 190°C and held for 3 min, and further increased at 1°C/min to 220°C and held for 20 min. Injector and detector temperatures were set at 250 and 280°C, respectively. Hydrogen was the carrier gas at a linear velocity of 17.2 cm/s. Fatty acids were identified by comparing retention times to standards (Supelco 37 Component FAME Mix, BAME Mix, PUFA No.1, PUFA No.2, PUFA No.3, Sigma-Aldrich, St. Louis, MO, United States of America; GLC-110 Mixture, Matreya, Pleasant Gap, PA, United States of America). The omega-3 index was calculated as the sum of EPA and DHA expressed as a proportion of total identified fatty acids.
2.6 Fecal pH and metabolites
Feces were thawed, and pH was determined using a potentiometer (pH and Ion-Meter GLP 22, Crison, Barcelona, Spain). Ammonia-N concentration was determined according to the method of Smith and Murphy (47) adapted to dog feces as described by Cabrita, Guilherme-Fernandes (48). Briefly, 1 g of feces were solubilized in 10 mL of KCl 2 M, centrifuged for 60 min at 5200 × g at 4°C, and the supernatant filtered using a 0.45 μm pore size polyethersulfone syringe filter (FILTER-LAB, Barcelona, Spain). Forty μL of supernatant were then mixed with 40 μL of water, 2.5 mL of phenol solution, and 2 mL of alkaline hypochlorite solution. After incubation for 10 min at 37°C and 40 min in the dark at 22°C, the absorbance of samples was read at 550 nm in a SynergyTM HT Multimode plate reader (BioTek® Instruments Inc., Winooski, VT). An ammonia solution (32 mg/dL) was used as a standard. Volatile fatty acid analysis was performed by gas chromatography in the supernatant from feces acidified with an orthophosphoric acid solution and centrifuged for 15 min at 2,360 × g at 4°C as described by Pereira, Maia (49).
2.7 Evaluation of flatulence
The assessment of dogs’ flatulence was performed for 5 h (from 10:30 h until 15:30 h) as described by Pereira, Guedes (50), using a device resembling the one described by Collins and Perez-Camargo (51). Briefly, a dog vest was developed to hold the device hardware, which consists of an air pump fitted with an H2S sensor connected to a microcontroller powered by a power bank. Gases were collected from the anal area through a 55 cm-long plastic tube (inner diameter 0.5 cm) connected to the pump-sensor box and an O-ring placed at the base of the tail. The H2S concentrations were presented in parts per million and recorded every 2 s. The number of flatulence episodes, maximum, and average intensity of H2S detected in each episode were recorded. Due to the sensitivity of the sensor and the possibility of cross-reactivity with other gases, blank data were collected in the room where the measures took place for 5 h to distinguish flatulence episodes from noise. Hydrogen sulfide values higher than 5 ppm and lasting at least for 10 s were considered the threshold for flatulence detection.
2.8 Evaluation of coat quality
Taking as reference the number of elements (7) used in the study of Hester (52), and in order to represent both the average dog owners and individuals involved in pet health, a naïve panel of eight dog owners (two kennel staff, one animal science post-doctoral researcher, one laboratory technician, two animal science students, and two veterinarians), blind to the treatments, performed a sensory assessment of the coat condition. A day before the evaluation, all dogs were washed using a hypoallergenic dog shampoo. The panel assessed glossiness, greasiness, softness, and scale as defined by Marsh, Ruedisueli (53), using the 5-point scale proposed by Hester (52), in which 5 is the best and 1 is the worst score (Table 1).
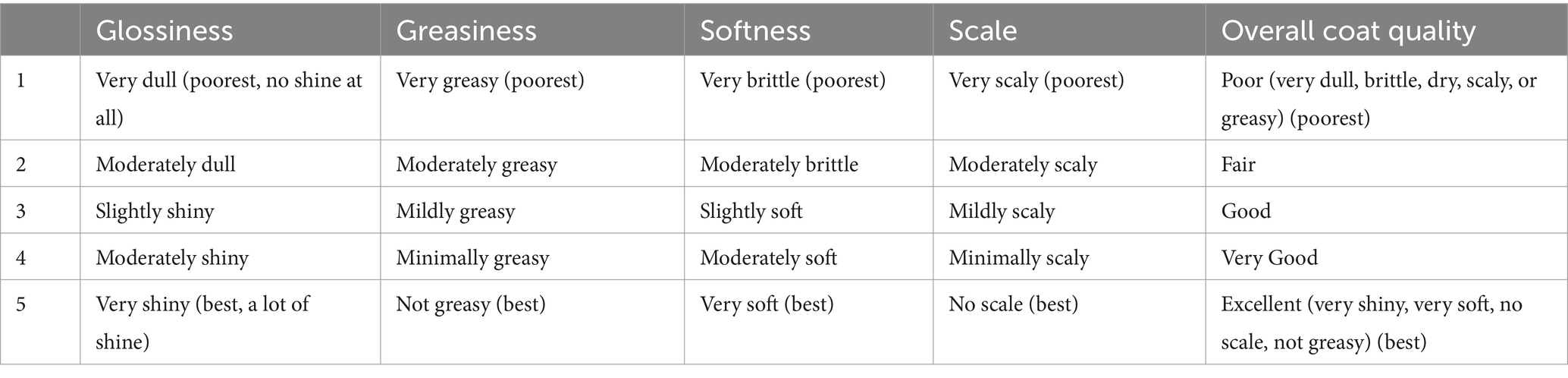
Table 1. Five-point scale used for coat scoring, adapted from Hester (52).
2.9 Calculations and statistical analysis
First-approach and first taste results from the palatability trial were submitted to the chi-square test and the intake ratio to the Student’s t-test, both at a 5% probability level.
Fecal production (%) was calculated as:
Diet apparent total tract digestibility (%) was calculated as follows:
The diet ME content was calculated according to Hall, Melendez (54) using the following equation:
Data from the feeding trial were analyzed using the mixed procedure of the Statistical Analysis Systems software package (SAS 2021, release 3.1.0., SAS Institute, Cary, NC, United States of America). The model included diet, period, and dietary sequence as fixed effects and animal within dietary sequence as a random effect. When differences were significant (p < 0.05), the Tukey test was used to compare means. The level of significance was set at a p-value of <0.05, and a trend was considered for a p-value of <0.10.
3 Results
3.1 Chemical composition of diets
The proximate composition of the studied diets is presented in Table 2. The control diet presented higher CP and slightly lower EE contents than the experimental diet, with NDF, starch, and GE contents similar between diets. The experimental diet presented a higher percentage of n-3 PUFAs, particularly EPA, docosapentaenoic acid (DPA, C22:5 n-3), and DHA, and a lower percentage of n-6 PUFA than the control diet, thus presenting a lower n-6:n-3 ratio (Table 3). Total amino acid content and profile were similar between diets (Table 4).
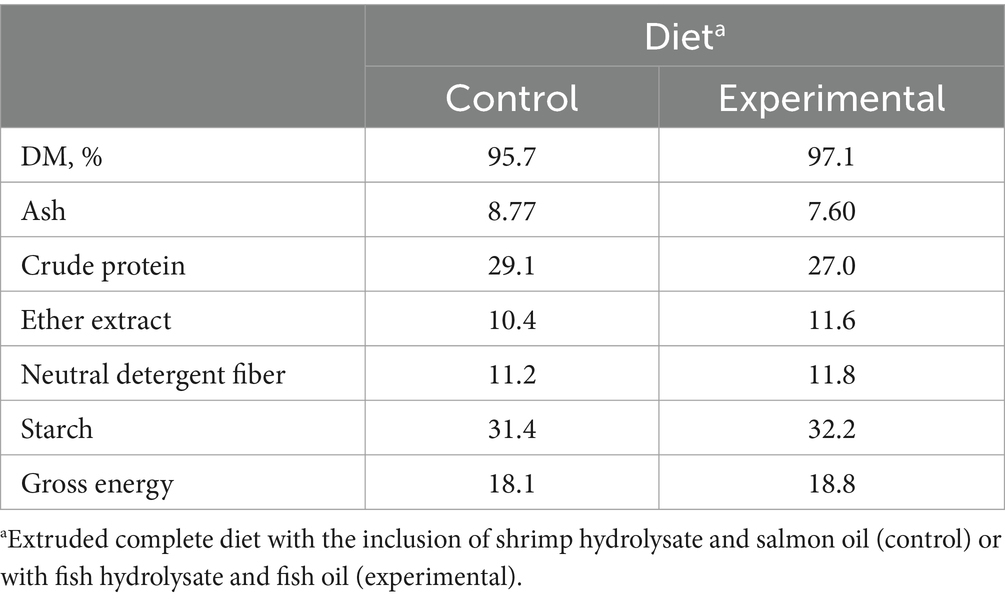
Table 2. Proximate composition (g 100 g−1 dry matter, DM) and gross energy (MJ kg−1 DM) of the control and experimental diets.
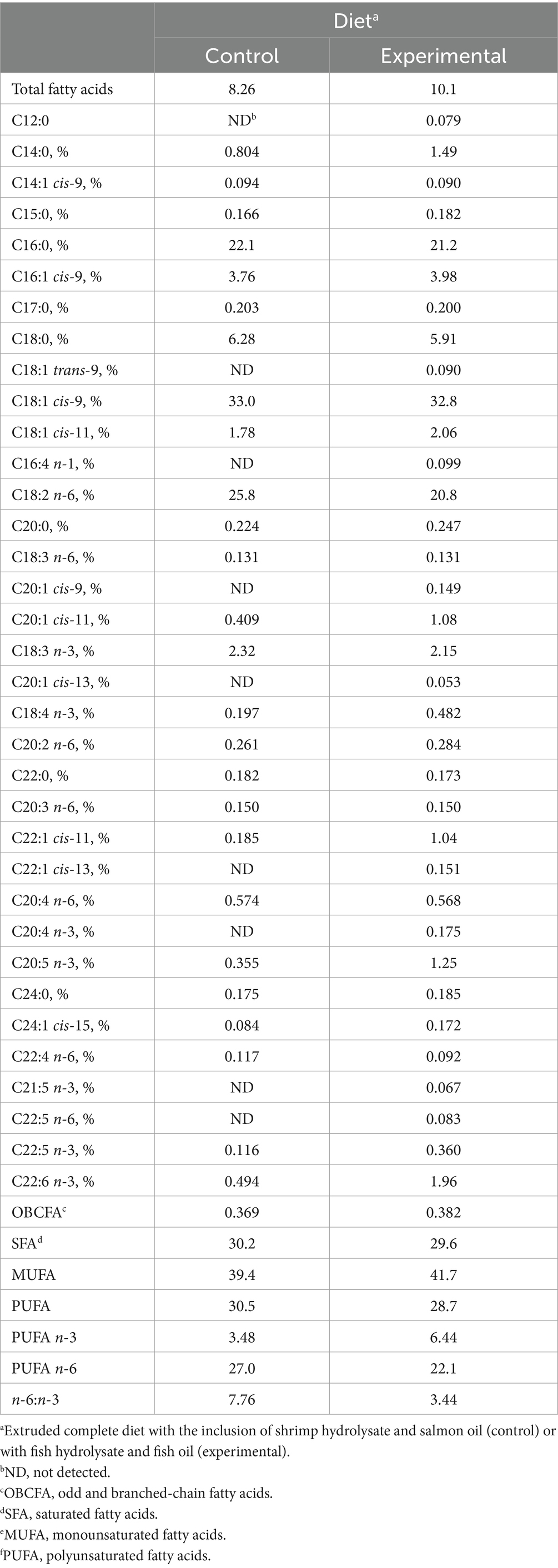
Table 3. Fatty acid content (g 100 g−1 dry matter) and profile (g 100 g−1 total fatty acids) of the control and experimental diets.
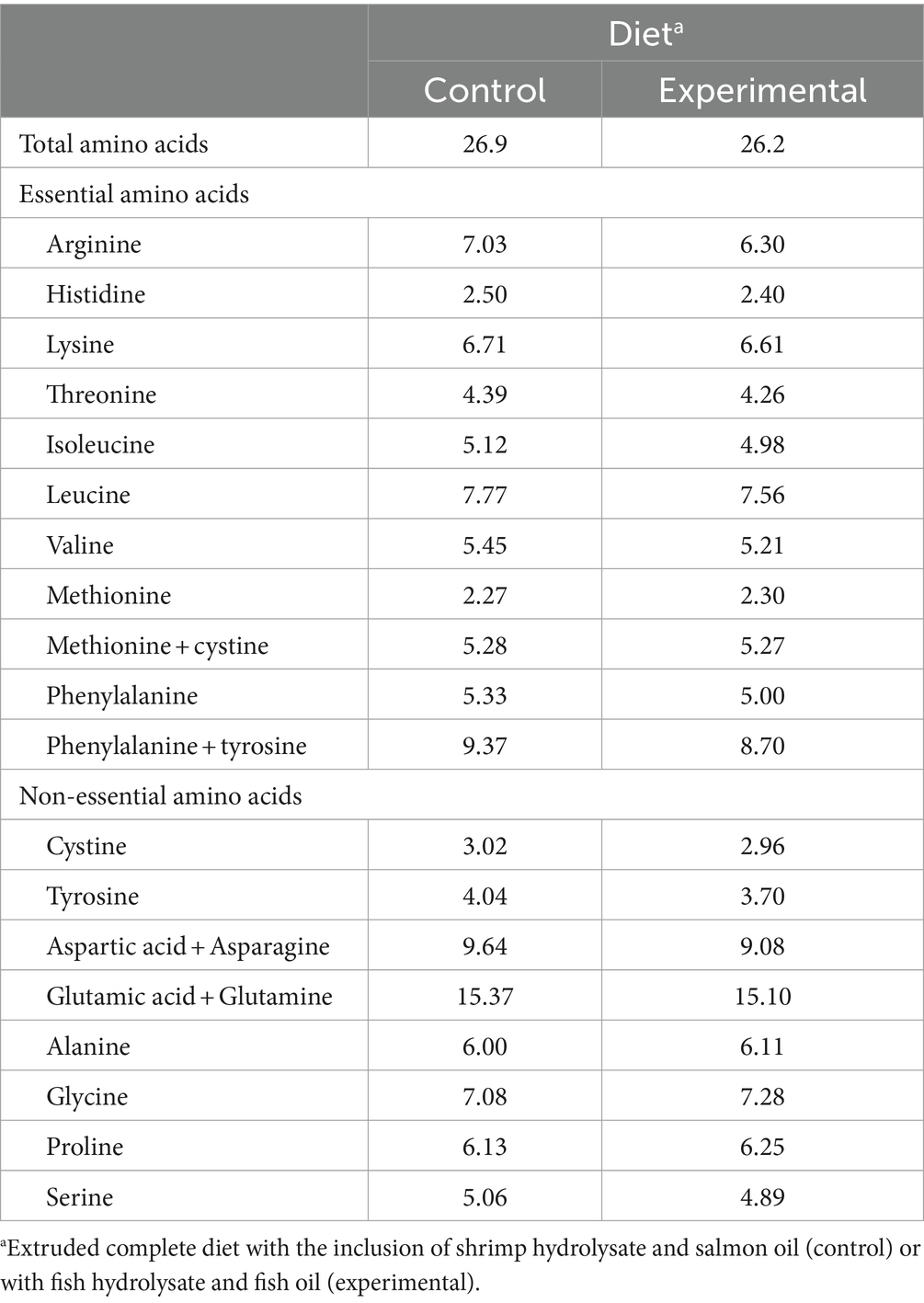
Table 4. Amino acid content (g 100 g−1 dry matter) and profile (g 100 g−1 total amino acids) of the control and experimental diets.
3.2 Palatability trial
The replacement of shrimp hydrolysate and salmon oil with fish hydrolysate and oil did not affect the first diet approach (p = 0.773) or taste (p = 0.386), as well as the intake ratio (p = 0.971; Table 5).
3.3 Feeding trial
3.3.1 Body weight, food intake, and fecal output
None of the dogs refused to eat any of the diets, and all remained healthy through the trial, with no signs of illness or maldigestion, with urine pH, density, and urinalysis results within the reference values for healthy animals (45) and not differing between diets (data not shown). Table 6 presents the body weight, body condition score, food and nutrient intake, and fecal output of dogs fed the control and experimental diets. Body weight and body condition score were not affected by diet (p > 0.05). Similarly, diet did not affect DM, nutrient, and energy intake (p > 0.05) with the exceptions of higher EE, EPA, DHA, and arachidonic acid (ARA, C20:4 n-6) intake with the experimental diet (p < 0.05) and a higher CP (p = 0.006) intake with the control diet. Daily fecal output was similar between diets (p > 0.05).
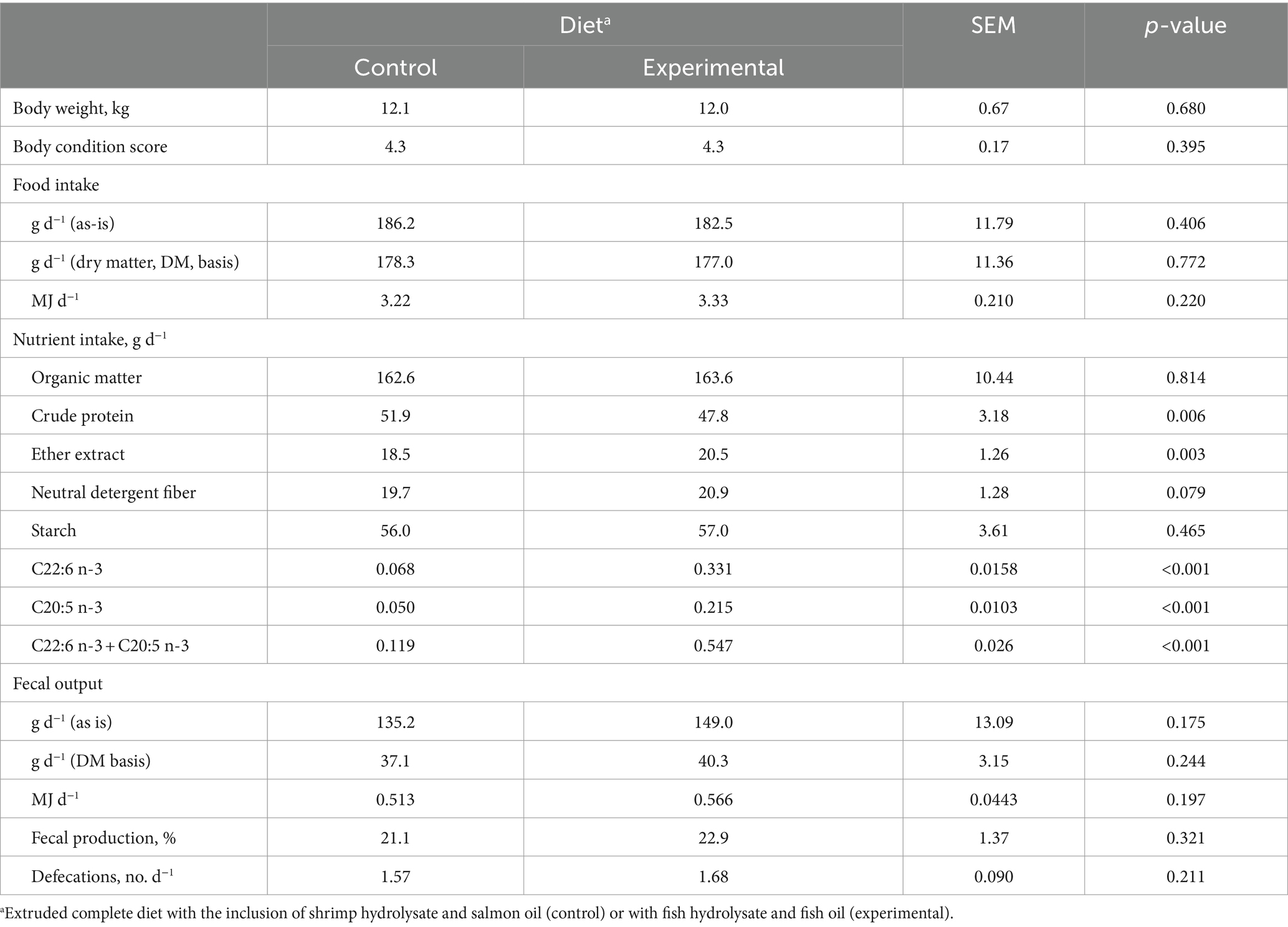
Table 6. Body weight, body condition score, food and nutrient intake, and fecal output of dogs fed the control and experimental diets.
3.3.2 Apparent total tract macronutrient digestibility and intake of digestible nutrients
Apparent total tract digestibility of DM, nutrients, and energy was not affected by diet (p > 0.05), except for NDF (p = 0.009), which was higher in the control diet (Table 7). Conversely, dietary ME content was higher in the experimental diet (p = 0.034). The intake of digestible DM and macronutrients was similar between diets, with the only exception of the intake of digestible CP and EE, which were higher in the control and the experimental diet (p < 0.001).
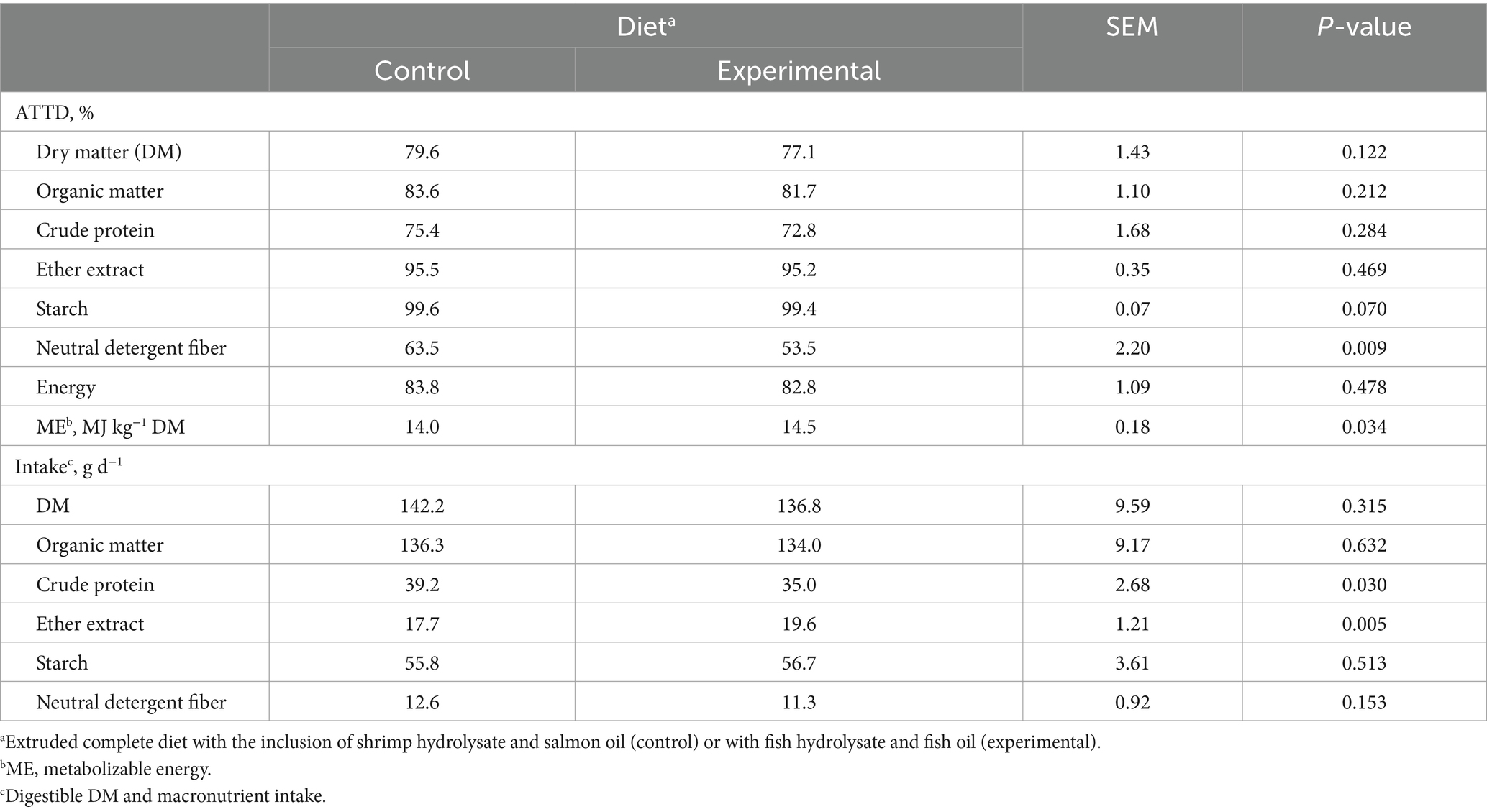
Table 7. Apparent total tract macronutrient digestibility (ATTD), estimated dietary metabolizable energy content and digestible dry matter, and nutrient intake of dogs fed the control and experimental diets.
3.3.3 Fecal characteristics and metabolites
Fecal characteristics, including pH, scores, DM, and metabolite concentrations, are presented in Table 8. None of the measured parameters were affected by diet (p > 0.05) except for ammonia-N (p = 0.042) and valerate concentration, which were higher (p = 0.007) in feces from dogs fed the control diet (Table 8).
3.3.4 Flatulence
The flatulence assessment is presented in Table 9. The number of episodes detected in 5 h averaged four, regardless of the diet. The average and maximum intensity of episodes (H2S ppm detected) ranged from 8.98 to 12.07 ppm and from 14.0 to 28.6 ppm, respectively, with no significant differences being detected between diets (p > 0.05).

Table 9. Number of episodes, average, and maximum intensity (H2S detected) of flatus of dogs fed the control and experimental diets.
3.3.5 Fatty acid profile of red blood cells
The experimental diet promoted the contents of EPA (p = 0.037) and DHA (p = 0.007), and, consequently, the omega-3 index (p = 0.005; Table 10) of the RBCs.
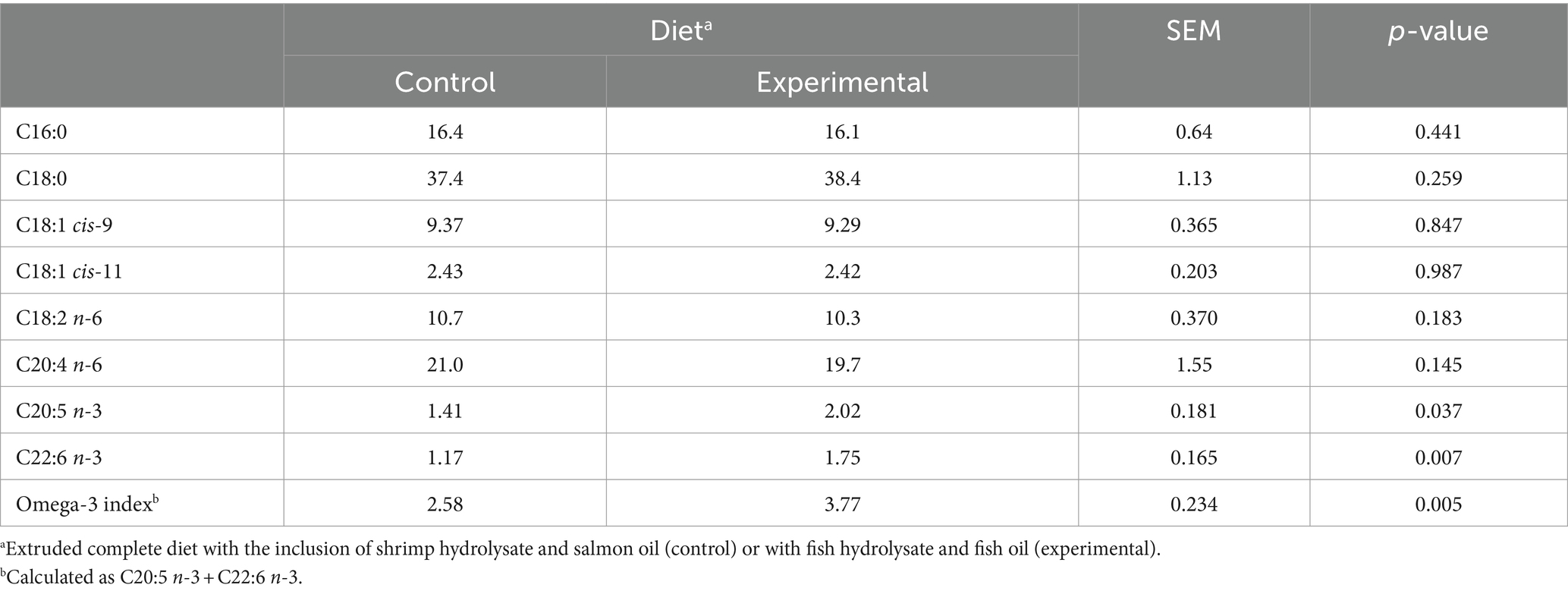
Table 10. Fatty acids’ profile (g 100 g-1) and omega-3 index of red blood cells from dogs fed the control and experimental diets.
3.3.6 Coat quality
The effect of diet on visual and sensorial coat evaluation is presented in Table 11. Scores were 3.99 vs. 3.83, 4.04 vs. 4.08, 4.04 vs. 3.98, 4.06 vs. 3.88, and 4.08 vs. 3.99 for glossiness, greasiness, softness, scale, and overall coat quality for the control and experimental diets. None of the parameters were affected by diet (p > 0.05).
4 Discussion
The use of fish BCPs from the agrifood sector for the extraction of oil rich in omega-3 fatty acids and protein hydrolysates constitutes a sustainable solution to the increasing problem of fish waste disposal. If applied locally, this circular economy further contributes to lowering dependency on third countries and mitigating the environmental impact of the food sector. For the full exploitation of these potential high-value products, in vivo studies are needed. Therefore, this current study aimed to investigate the effects of fish hydrolysate and oil from fish wastes during the processing stage on palatability, apparent total tract digestibility, fecal characteristics and metabolites, blood fatty acid profile, and coat quality of adult dogs. The experimental diet containing fish hydrolysate and oil was compared to a commercial diet including shrimp hydrolysate, commonly used in fish diets (55, 56), but recently shown to have the potential to be included in dog diets (37), and salmon oil, a common oil source used in dog food. With shrimp hydrolysate and salmon oil mainly imported from third countries, the use of fish hydrolysate and oil locally produced might contribute to the increased sustainability of the pet food sector.
Both diets used in the current study ensured the energy and nutrient requirements of adult dogs, according to FEDIAF (19). The chemical composition of the diets reflected the composition of the hydrolysates and oils used, with the main differences being observed in ash, CP, and EE contents, and fatty acid profiles. The lower ash content of the experimental diet might be a favorable aspect of the selection of the studied sources for addition in high-digestibility diets, as a high ash content may compromise protein digestibility (57). Regarding the EE content and fatty acid profile, it is well known that these parameters can be affected by fish species, size, age, sex, fish parts, diet, extraction method, and commercial source. The contents of omega-3 long-chain PUFA, namely EPA, DPA, and DHA, are generally higher in oil from marine than freshwater fish species (58–60). In vitro and in vivo studies with dogs showed that these conditionally essential fatty acids (19) play important metabolic roles in cell membrane fluidity, neural development, cognitive status, visual acuity, and inflammatory and immunologic responses (61–63). Similarly, the CP content and amino acid profile of seafood and crustacean hydrolysates might differ depending on the primary source of protein (species and part of the animal), enzyme source, and hydrolysis conditions (17, 64). An earlier study (37) showed that in shrimp hydrolysate obtained from the enzymatic hydrolysis of heads and cephalothoraxes of Litopenaeus vannamei, as the one used in the current study, lysine and leucine were the essential amino acids found in the highest amounts, and glutamic acid plus glutamine were the main non-essential amino acids. For fish hydrolysates, the review from Chalamaiah, Kumar (65) reported aspartic acid and glutamic acid to be found in high amounts in most of the fish protein hydrolysates, and that muscle, head, skin, and visceral hydrolysates contain all the essential and non-essential amino acids, whereas in fish frame protein hydrolysates, aromatic amino acids were not reported.
In the present study, diet palatability was evaluated using the two-bowl test, considered effective when evaluating intentional product enhancements but recognized to be unable to detect eventual flavor fatigue, longer-term nutritional feedback on taste and satiety, and dependent on the reference diet (36). Additionally, the number of animals included in the test is an important consideration when interpreting the results. Although using 20 animals over 2 or 4 days is considered to increase the test resolution (36), the number of animals used in palatability tests greatly differs in the literature, with studies reporting the use of 8 (66), 10 (67), and 16 (68) animals. In the current study, despite the sample size (12 animals) being defined according to an earlier study that evaluated the dietary supplementation with shrimp hydrolysate (37), the number of animals used was lower than the generally recommended (20 animals), so some caution should be taken when interpreting the results obtained. Animals accepted well both the control and the experimental diet, with no differences in the first approach, taste, or intake ratio. It is well known that factors such as food flavor, texture, ingredients, chemical composition, temperature, previous experience of the animal, and size and shape of the kibble can affect palatability (69), and this constitutes an important aspect when formulating diets as palatability might affect both food intake and the provision of nutrients and strongly determine food repurchase potential. In the current study, both diets presented the same kibble size, shape, and texture. Although animal protein hydrolysates are among the most common palatability enhancers used in commercial pet food, scientific studies are scarce (70). Sensorial characteristics of hydrolyzed proteins are associated with the raw protein source and mixture of peptides (71), with short peptides and amino acids such as taurine, glycine, arginine, glutamic acid, and alanine acting as feeding stimulants for companion animals (13). Moreover, bitterness was earlier associated with the molecular weight of peptides, with reports of increased bitterness in soy protein hydrolysates when the molecular weight of the peptides ranged from 4 to 2 kDa and decreased bitterness with peptides <1 kDa (72). Despite these reports, several studies showed the high palatability of protein hydrolysates, such as fish (18) and chicken (73) hydrolysates for dogs.
Dietary fat content is also known to affect palatability, with dogs preferring high-fat diets (74, 75). Being unsaturated fatty acids, particularly EPA and DHA, highly susceptible to deterioration (76), the free radical formation and breakdown of hydroperoxides release organic substances such as short-chain aldehydes, ketones, and alcohols, seen as off-flavors, which might promote the rejection of the food (77, 78). Although not measured in the current study, the stability of the oils used might not have constituted an issue, as no differences were observed on the palatability test, and both diets were well accepted during the feeding trial.
The daily food allowance was determined to ensure energy requirements and adjusted according to the ideal body weight and body condition score, and thus these parameters remained constant during the study. As diets presented similar energy content and digestibility, the daily amount of food required to ensure the requirements did not vary between diets. The differences found in the intake of CP, EE, and some fatty acids reflect the different chemical compositions of the diets. These differences were not reflected in the fecal output and stool quality that were adequate for dogs fed both diets and constitute important parameters for pet owners. An earlier study with dogs found higher fecal volume and lower fecal DM content with the dietary inclusion of 258 g/kg of hydrolyzed chicken liver when compared to a control diet with poultry byproduct, bovine meat, and bone meal, that might be explained by the high osmolarity of that attracts water to the intestinal lumen, but fecal DM output and fecal scores were not affected (79).
Digestibility of DM, nutrients, and energy was similar between diets except for NDF digestibility, which was higher in the control diet but did not affect the intake of digestible NDF, fecal pH, total VFA production, acetate concentration, or ammonia-N concentration. The differences in the dietary EE content and fatty acid profile might contribute to explaining the observed effects on NDF digestibility among diets, namely through the modulation of fecal microbial populations (80, 81). Although no significant effects were observed on CP digestibility, the significantly higher intake of digestible CP observed with the control diet might have contributed to the higher fecal ammonia-N and valerate concentrations (and a tendency for a higher iso-valerate concentration), suggesting that a higher amount of undigested protein has reached the intestine with this diet. Indeed, ammonia-N is generated by the deamination of amino acids, being toxic at high concentrations, and branched-chain fatty acids originate from the fermentation of branched-chain amino acids (82, 83). Although not herein measured, the results obtained might have affected fecal odor that results from the formation of several putrefactive substances from the colonic fermentation of endogenous and undigested amino acids, such as ammonia, aliphatic amines, branched-chain fatty acids, indoles, phenols, and volatile sulfur-containing compounds, that may also exert adverse effects on colonic health (84, 85). While large individual variation inhibited the significance of differences in H2S emissions, the higher values obtained with the control diet might suggest a higher availability of sulfur-amino acids in the colon of dogs fed the control diet. Using diets not differing on CP digestibility (70.1 to 73.7%), but differing on Zn source (Zn proteinate and Zn sulfate), and without or with the addition of a solid-state fermentation product of Aspergillus niger with residual enzymatic activity, Pereira, Guedes (50) also found a high individual variation on the number of episodes (3.3–10.3), average (11.0–17.0 ppm), and maximum intensity (20.4–33.8 ppm) of adult Beagle dogs. Further studies are needed to confirm the effect observed as H2S inhibits the utilization of butyrate by colonocytes caused by mitochondrial metalloproteins and is associated with ulcerative colitis (86) as well as the severity of flatus malodor (51). Additionally, despite the scarcity of information available, the study performed by Zhao and McCamish (87) with intact and hydrolyzed soy protein intestinally perfused in increasing amounts in dogs with duodenal and midintestinal fistulas has shown that intestinal transit is slowed in a load-dependent fashion regardless of the source of protein, but it is more pronounced with intact protein, and that a greater amount is absorbed in the proximal half of the small intestine when protein is delivered in a hydrolyzed form, also concluding that as digestion is the rate-limiting step of assimilation in the small intestine, a longer residence time might be needed with a higher protein load. Despite these observations, no effects were observed on fecal output or characteristics.
As the digestibility of EE did not differ between diets, the higher intake of digestible EE reflected the higher EE intake. Differences in EPA and DHA intake were further reflected in the EPA and DHA of RBC and the omega-3 index. Indeed, the RBC omega-3 index has been reported as a biomarker of long-term EPA and DHA intake in humans (88, 89), being inversely correlated with neutrophil–lymphocyte ratio (a systemic inflammation biomarker, 90), cardiovascular disease risk, and acute death (90). Recently, Harris, Jackson (91) suggested its use as a health status biomarker in dogs. Although no range has yet been established for optimal health status in dogs, a 0.3 to 7.0% omega-3 index was determined in routine veterinary dog blood samples, with higher values being associated with improved health (91). An increased omega-3 index was also reported in the RBC of dogs fed a diet supplemented with krill meal or fish meal plus fish oil for 4 weeks (92) and krill oil for 6 weeks (93). The highest values were reported for krill oil (2.70%) and krill meal (2.36%), in the range of those reported for the control diet (2.58%). Moreover, the experimental diet promoted an increase in the omega-3 index by approximately 1.5-fold compared with the control diet, suggesting a potential health-promoting effect of fish BCP’s inclusion in pet food, namely on promoting cardiovascular function and anti-inflammatory activity.
The increased absorption and metabolization of EPA and DHA by dogs fed the experimental diet were hypothesized to promote the coat quality of dogs, as suggested by Combarros and Castilla-Castano (94), but no differences were observed by a näive panel in the present study. The lack of effects may be due to the similarity of the oils used, both rich in omega-3 PUFA, and the use of healthy dogs. Indeed, Logas and Kunkle (95) reported an improvement in the coat quality of dogs with skin diseases and poor coat quality after 6 weeks of supplementation with EPA-rich marine oil (180 mg EPA/120 mg DHA) compared with corn oil supplement; no differences were observed after 3 weeks. More recently, Combarros, Castilla-Castano (94) investigated the effect of a fish oil supplement (110 mg EPA/68 mg DHA) for 3 months on dogs with poor hair coats and observed a reduced clinical score after 2 months of EPA and DHA supplementation when compared to placebo-supplemented dogs, but no differences after 4 weeks. These results support the need for longer studies to fully unveil the potential of fish by-product hydrolysate and oil supplementation on the coat quality of healthy dogs.
5 Conclusion
The present study shows that a diet with the inclusion of locally produced fish oil and hydrolysate from the agrifood industry in place of imported salmon oil and shrimp hydrolysate was well accepted by dogs, not affecting food intake, digestibility, or fecal characteristics. The lower fecal ammonia-N and valerate concentrations suggest a lower amount of undigested protein reaching the small intestine or a higher protein absorption. Conversely, fish hydrolysate and oil promoted blood EPA, DHA, and omega-3 index, suggesting a potential health-promoting effect. Overall, the results support the potential of fish hydrolysate and oil from the agrifood industry to be used in dog food, contributing to increasing the sustainability of the pet food sector. Further research is needed to evaluate the functional role of these fish by-products.
Data availability statement
The raw data supporting the conclusions of this article will be made available by the authors, without undue reservation.
Ethics statement
The animal study was approved by Animal Ethics Committee of School of Medicine and Biomedical Sciences, University of Porto, and licensed by the Portuguese General Directorate of Food and Veterinary Medicine (Permit No 0421/000/000/2021). The study was conducted in accordance with the local legislation and institutional requirements.
Author contributions
AC: Supervision, Writing – original draft, Resources, Project administration, Investigation, Funding acquisition, Formal analysis, Conceptualization. MM: Writing – review & editing, Investigation, Formal analysis, Conceptualization. APA: Writing – review & editing, Investigation. TA: Writing – review & editing, Resources. AR: Writing – review & editing, Resources. AA: Writing – review & editing, Resources. RM: Writing – review & editing, Resources. AF: Writing – review & editing, Resources, Investigation, Funding acquisition, Formal analysis, Conceptualization.
Funding
The author(s) declare financial support was received for the research, authorship, and/or publication of this article. This work received financial support from the Portuguese Foundation for Science and Technology (FCT/MCTES; UIDB/50006/2020 DOI: 10.54499/UIDB/50006/2020) through national funds.
Acknowledgments
Authors acknowledge project Healthy Pet Food (POCI-01-0247-FEDER-047073) supported by Portugal 2020 program through the European Regional Development Fund. The contract of MRGM was funded through DL 57/2016–Norma transitória (SFRH/BPD/70176/2010) by FCT. The authors also acknowledge, from the School of Medicine and Biomedical Sciences, University of Porto, Portugal, Sílvia Azevedo for the technical assistance and analytical determinations, Margarida Guedes for the technical assistance throughout in-vivo trials collection, and the staff of the kennel for assistance and maintenance of the dogs.
Conflict of interest
TA was employed by SORGAL, Sociedade de Óleos e Rações S.A. AR was employed by SEBOL, Comércio e Indústria de Sebo, S.A., AA and RM were employed by Indústria Transformadora de Subprodutos, S.A.
The remaining authors declare that the research was conducted in the absence of any commercial or financial relationships that could be construed as a potential conflict of interest.
Publisher’s note
All claims expressed in this article are solely those of the authors and do not necessarily represent those of their affiliated organizations, or those of the publisher, the editors and the reviewers. Any product that may be evaluated in this article, or claim that may be made by its manufacturer, is not guaranteed or endorsed by the publisher.
References
1. Regueiro, L, Newton, R, Soula, M, Mendez, D, Kok, B, Little, DC, et al. Opportunities and limitations for the introduction of circular economy principles in EU aquaculture based on the regulatory framework. J Ind Ecol. (2022) 26:2033–44. doi: 10.1111/jiec.13188
3. Cooney, R, de Sousa, DB, Fernández-Ríos, A, Mellett, S, Rowan, N, Morse, AP, et al. A circular economy framework for seafood waste valorisation to meet challenges and opportunities for intensive production and sustainability. J Clean Prod. (2023) 392:136283. doi: 10.1016/j.jclepro.2023.136283
4. Kandyliari, A, Mallouchos, A, Papandroulakis, N, Golla, JP, Lam, TT, Sakellari, A, et al. Nutrient composition and fatty acid and protein profiles of selected fish by-products. Food Secur. (2020) 9:190. doi: 10.3390/foods9020190
5. Sahana, MD, Balange, AK, Layana, P, and Naidu, BC. Chapter Five - Harnessing value and sustainability: fish waste valorization and the production of valuable byproducts. E Capanoglu, MD Navarro-Hortal, TY Forbes-Hernández, and M Battino, (Eds.), Advances in food and nutrition research. 107: Academic Press; (2023). 175–192
6. Coppola, D, Lauritano, C, Palma Esposito, F, Riccio, G, Rizzo, C, and de Pascale, D. Fish waste: from problem to valuable resource. Mar Drugs. (2021) 19:20116. doi: 10.3390/md19020116
7. Lopes, C, Antelo, LT, Franco-Uría, A, Alonso, AA, and Pérez-Martín, R. Valorisation of fish by-products against waste management treatments – comparison of environmental impacts. Waste Manag. (2015) 46:103–12. doi: 10.1016/j.wasman.2015.08.017
8. Caruso, G, Floris, R, Serangeli, C, and Di Paola, L. Fishery wastes as a yet undiscovered treasure from the sea: biomolecules sources, extraction methods and valorization. Mar Drugs. (2020) 18:622. doi: 10.3390/md18120622
9. European Commission. Communication from the commission to the European parliament, the European council, the council, the European economic and social committee and the committee of the regions. A new circular economy action plan. For a cleaner and more competitive Europe. European Commission Brussels Cf, editor. (2020). 1–19.
11. Schleicher, M, Cash, SB, and Freeman, LM. Determinants of pet food purchasing decisions. The Canadian veterinary journal = La revue veterinaire canadienne. (2019) 60:644–50.
12. Tjernsbekk, MT, Tauson, AH, Kraugerud OF,, and Ahlstrøm, Ø. Raw mechanically separated chicken meat and salmon protein hydrolysate as protein sources in extruded dog food: effect on protein and amino acid digestibility. J Animal Physiol Animal Nutr. (2017) 101:e323–31. doi: 10.1111/jpn.12608
13. Martínez-Alvarez, O, Chamorro, S, and Brenes, A. Protein hydrolysates from animal processing by-products as a source of bioactive molecules with interest in animal feeding: a review. Food Res Int. (2015) 73:204–12. doi: 10.1016/j.foodres.2015.04.005
14. Landsberg, GM, Mougeot, I, Kelly, S, and Milgram, NW. Assessment of noise-induced fear and anxiety in dogs: modification by a novel fish hydrolysate supplemented diet. J Vet Behav. (2015) 10:391–8. doi: 10.1016/j.jveb.2015.05.007
15. Titeux, E, Padilla, S, Paragon, BM, and Gilbert, C. Effects of a new dietary supplement on behavioural responses of dogs exposed to mild stressors. Vet Med Science. (2021) 7:1469–82. doi: 10.1002/vms3.560
16. Theysgeur, S, Cudennec, B, Deracinois, B, Perrin, C, Guiller, I, Lepoudère, A, et al. New bioactive peptides identified from a Tilapia byproduct hydrolysate exerting effects on DPP-IV activity and intestinal hormones regulation after canine gastrointestinal simulated digestion. Molecules. (2020) 26:10136. doi: 10.3390/molecules26010136
17. Anal, AK, Noomhorm, A, and Vongsawasdi, P. Protein hydrolysates and bioactive peptides from seafood and crustacean waste: their extraction, bioactive properties and industrial perspectives. Marine Proteins Peptides. (2013):709–35. doi: 10.1002/9781118375082.ch36
18. Folador, JF, Karr-Lilienthal, LK, Parsons, CM, Bauer, LL, Utterback, PL, Schasteen, CS, et al. Fish meals, fish components, and fish protein hydrolysates as potential ingredients in pet foods. J Anim Sci. (2006) 84:2752–65. doi: 10.2527/jas.2005-560
19. FEDIAF. The European pet food industry. Nutritional guidelines for complete and complementary pet food for cats and dogs. Bruxelles, Belgium: (2021).
20. NRC. Nutrient requirements for dogs and cat. 2nd rev. ed. Washington, DC, USA: The National Academies Press (2006).
21. AAFCO. Association of American Feed Control Officials, Methods for substantiating nutritional adquacy of dogs and cat foods, AAFCO dog and cat food nutrient profiles 2014, Available at: https://www.aafco.org/wp-content/uploads/2023/01/Model_Bills_and_Regulations_Agenda_Midyear_2015_Final_Attachment_A.__Proposed_revisions_to_AAFCO_Nutrient_Profiles_PFC_Final_070214.pdf
22. Freeman, LM, Rush, JE, Kehayias, JJ, Ross, JN, Meydani, SN, Brown, DJ, et al. Nutritional alterations and the effect of fish oil supplementation in dogs with heart failure. J Vet Intern Med. (1998) 12:440–8. doi: 10.1111/j.1939-1676.1998.tb02148.x
23. Neumayer, HH, Heinrich, M, Schmissas, M, Haller, H, Wagner, K, and Luft, FC. Amelioration of ischemic acute renal failure by dietary fish oil administration in conscious dogs. J Am Soc Nephrol. (1992) 3:1312–20. doi: 10.1681/ASN.V361312
24. Roush, JK, Cross, AR, Renberg, WC, Dodd, CE, Sixby, KA, Fritsch, DA, et al. Evaluation of the effects of dietary supplementation with fish oil omega-3 fatty acids on weight bearing in dogs with osteoarthritis. J Am Vet Med Assoc. (2010) 236:67–73. doi: 10.2460/javma.236.1.67
25. Blaskovic, M, Rosenkrantz, W, Neuber, A, Sauter-Louis, C, and Mueller, RS. The effect of a spot-on formulation containing polyunsaturated fatty acids and essential oils on dogs with atopic dermatitis. Vet J. (2014) 199:39–43. doi: 10.1016/j.tvjl.2013.10.024
26. Simpson, KW, Miller, ML, Loftus, JP, Rishniw, M, Frederick, CE, and Wakshlag, JJ. Randomized controlled trial of hydrolyzed fish diets in dogs with chronic enteropathy. J Vet Intern Med. (2023) 37:2334–43. doi: 10.1111/jvim.16844
27. Ganesan, B, Brothersen, C, and McMahon, DJ. Fortification of foods with omega-3 polyunsaturated fatty acids. Crit Rev Food Sci Nutr. (2014) 54:98–114. doi: 10.1080/10408398.2011.578221
28. Cottrell, RS, Blanchard, JL, Halpern, BS, Metian, M, and Froehlich, HE. Global adoption of novel aquaculture feeds could substantially reduce forage fish demand by 2030. Nat Food. (2020) 1:301–8. doi: 10.1038/s43016-020-0078-x
29. Orozco Colonia, BS, de Melo, V, Pereira, G, and Soccol, CR. Omega-3 microbial oils from marine thraustochytrids as a sustainable and technological solution: a review and patent landscape. Trends Food Sci Technol. (2020) 99:244–56. doi: 10.1016/j.tifs.2020.03.007
30. Gill, I, and Valivety, R. Polyunsaturated fatty acids, part 1: occurrence, biological activities and applications. Trends Biotechnol. (1997) 15:401–9. doi: 10.1016/S0167-7799(97)01076-7
31. Tocher, DR . Omega-3 long-chain polyunsaturated fatty acids and aquaculture in perspective. Aquaculture. (2015) 449:94–107. doi: 10.1016/j.aquaculture.2015.01.010
32. Patel, A, Desai, SS, Mane, VK, Enman, J, Rova, U, Christakopoulos, P, et al. Futuristic food fortification with a balanced ratio of dietary ω-3/ω-6 omega fatty acids for the prevention of lifestyle diseases. Trends Food Sci Technol. (2022) 120:140–53. doi: 10.1016/j.tifs.2022.01.006
33. Patel, AK, Singhania, RR, Awasthi, MK, Varjani, S, Bhatia, SK, Tsai, M-L, et al. Emerging prospects of macro- and microalgae as prebiotic. Microb Cell Factories. (2021) 20:112. doi: 10.1186/s12934-021-01601-7
34. Laflamme, D . Development and validation of a body condition score system for dogs. Canine Practice. (1997) 22:10–5.
35. Regulation European Commission, 1069/2009 E. Regulation (EC) No 1069/2009 of the European parliament and of the council of 21 October 2009 laying down health rules as regards animal by-products and derived products not intended for human consumption and repealing regulation (EC) no 1774/2002 (animal by-products regulation). Off J Eur Union; (2009). 1–33.
36. Aldrich, GC, and Koppel, K. Pet food palatability evaluation: a review of standard assay techniques and interpretation of results with a primary focus on limitations. Animals. (2015) 5:43–55. doi: 10.3390/ani5010043
37. Guilherme-Fernandes, J, Aires, T, Fonseca, AJM, Yergaliyev, T, Camarinha-Silva, A, Lima, SAC, et al. Squid meal and shrimp hydrolysate as novel protein sources for dog food. Frontiers in veterinary. Science. (2024):11. doi: 10.3389/fvets.2024.1360939
38. Carciofi, AC, de-Oliveira, LD, Valério, AG, Borges, LL, de Carvalho, FM, Brunetto, MA, et al. Comparison of micronized whole soybeans to common protein sources in dry dog and cat diets. Anim Feed Sci Technol. (2009) 151:251–60. doi: 10.1016/j.anifeedsci.2009.01.002
39. AOAC In: W Horwitz , editor. Official methods of analysis of AOAC international. Gaithersburg, Md: AOAC International (2000)
40. Van Soest, PJ, Robertson, JB, and Lewis, BA. Methods for dietary fiber, neutral detergent fiber, and nonstarch polysaccharides in relation to animal nutrition. J Dairy Sci. (1991) 74:3583–97. doi: 10.3168/jds.S0022-0302(91)78551-2
41. Salomonsson, AC, Theander, O, and Westerlund, E. Chemical characterization of some swedish cereal whole meal and bran fractions. Swed J Agric Res. (1984) 14:111–7.
42. Lepage, G, and Roy, CC. Improved recovery of fatty acid through direct transesterification without prior extraction or purification. J Lipid Res. (1984) 25:1391–6. doi: 10.1016/S0022-2275(20)34457-6
43. Maia, MRG, Fonseca, AJM, Cortez, PP, and Cabrita, ARJ. In vitro evaluation of macroalgae as unconventional ingredients in ruminant animal feeds. Algal Res. (2019) 40:101481. doi: 10.1016/j.algal.2019.101481
44. Aragão, C, Cabano, M, Colen, R, Fuentes, J, and Dias, J. Alternative formulations for gilthead seabream diets: towards a more sustainable production. Aquac Nutr. (2020) 26:444–55. doi: 10.1111/anu.13007
45. Alleman, R, and Wamsley, H. Complete urinalysis. BSAVA manual of canine and feline nephrology and urology : BSAVA Library; (2017). 60–83
46. Harris, WS, Pottala, JV, Vasan, RS, Larson, MG, and Robins, SJ. Changes in erythrocyte membrane trans and marine fatty acids between 1999 and 2006 in older Americans. J Nutr. (2012) 142:1297–303. doi: 10.3945/jn.112.158295
47. Smith, F, and Murphy, T. Analysis of Rumen Ammonia & Blood Urea Nitrogen University of Nebraska-Lincoln (2013).
48. Cabrita, ARJ, Guilherme-Fernandes, J, Spínola, M, Maia, MRG, Yergaliyev, T, Camarinha-Silva, A, et al. Effects of microalgae as dietary supplement on palatability, digestibility, fecal metabolites, and microbiota in healthy dogs. Frontiers in veterinary. Science. (2023):10:1245790. doi: 10.3389/fvets.2023.1245790
49. Pereira, AM, Maia, MRG, Pinna, C, Biagi, G, Matos, E, Segundo, MA, et al. Effects of zinc source and enzyme addition on the fecal microbiota of dogs. Front Microbiol. (2021) 12:688392. doi: 10.3389/fmicb.2021.688392
50. Pereira, AM, Guedes, M, Matos, E, Pinto, E, Almeida, AA, Segundo, MA, et al. Effect of zinc source and exogenous enzymes supplementation on zinc status in dogs fed high phytate diets. Animals. (2020) 10:30400. doi: 10.3390/ani10030400
51. Collins, SB, Perez-Camargo, G, Gettinby, G, Butterwick, RF, Batt, RM, and Giffard, CJ. Development of a technique for the in vivo assessment of flatulence in dogs. Am J Vet Res. (2001) 62:1014–9. doi: 10.2460/ajvr.2001.62.1014
52. Hester, SL . Effect of dietary polyunsaturated fatty acid and related nutrients on plasma lipids, and skin and hair coat condition in canines [Master's thesis]. Texas, USA: Texas A&M University (2004).
53. Marsh, KA, Ruedisueli, FL, Coe, SL, and Watson, TGD. Effects of zinc and linoleic acid supplementation on the skin and coat quality of dogs receiving a complete and balanced diet. Vet Dermatol. (2000) 11:277–84. doi: 10.1046/j.1365-3164.2000.00202.x
54. Hall, JA, Melendez, LD, and Jewell, DE. Using gross energy improves metabolizable energy predictive equations for pet foods whereas undigested protein and fiber content predict stool quality. PLoS One. (2013) 8:e54405. doi: 10.1371/journal.pone.0054405
55. Li, S, Dai, M, Qiu, H, and Chen, N. Effects of fishmeal replacement with composite mixture of shrimp hydrolysate and plant proteins on growth performance, feed utilization, and target of rapamycin pathway in largemouth bass, Micropterus salmoides. Aquaculture. (2021) 533:736185. doi: 10.1016/j.aquaculture.2020.736185
56. Gunathilaka, BE, Khosravi, S, Herault, M, Fournier, V, Lee, C, Jeong, J-B, et al. Evaluation of shrimp or tilapia protein hydrolysate at graded dosages in low fish meal diet for olive flounder (Paralichthys olivaceus). Aquac Nutr. (2020) 26:1592–603. doi: 10.1111/anu.13105
57. El-Wahab, AA, Chuppava, B, Zeiger, AL, Visscher, C, and Kamphues, J. Nutrient digestibility and fecal quality in beagle dogs fed meat and bone meal added to dry food. Vet Sci. (2022) 9:164. doi: 10.3390/vetsci9040164
58. Hesta, M, Verbrugghe2, A, Gulbrandsen, KE, Christophe, A, Zentek, J, Hellweg, P, et al. Biological effects of short-term salmon oil administration, using distinct salmon oil sources in healthy dogs. J Small Anim Pract. (2012) 53:699–704. doi: 10.1111/j.1748-5827.2012.12000.x
59. Mgbechidinma, CL, Zheng, G, Baguya, EB, Zhou, H, Okon, SU, and Zhang, C. Fatty acid composition and nutritional analysis of waste crude fish oil obtained by optimized milder extraction methods. Env Eng Res. (2023) 28:220034. doi: 10.4491/eer.2022.034
60. Rahman, N, Hashem, S, Akther, S, and Jothi, JS. Impact of various extraction methods on fatty acid profile, physicochemical properties, and nutritional quality index of Pangus fish oil. Food Sci Nutr. (2023) 11:4688–99. doi: 10.1002/fsn3.3431
61. Bauer, JE . Therapeutic use of fish oils in companion animals. J Am Vet Med Assoc. (2011) 239:1441–51. doi: 10.2460/javma.239.11.1441
62. LeBlanc, CJ, Horohov, DW, Bauer, JE, Hosgood, G, and Mauldin, GE. Effects of dietary supplementation with fish oil on in vivo production of inflammatory mediators in clinically normal dogs. Am J Vet Res. (2008) 69:486–93. doi: 10.2460/ajvr.69.4.486
63. Rodrigues, M, Rosa, A, Almeida, A, Martins, R, Ribeiro, T, Pintado, M, et al. Omega-3 fatty acids from fish by-products: innovative extraction and application in food and feed. Food Bioprod Process. (2024) 145:32–41. doi: 10.1016/j.fbp.2024.02.007
64. Naik, AS, Whitaker, RD, Albrektsen, S, Solstad, RG, Thoresen, L, and Hayes, M. Mesopelagic fish protein hydrolysates and extracts: a source of novel anti-hypertensive and anti-diabetic peptides. Front. Mar. Sci. (2021) 8:719608. doi: 10.3389/fmars.2021.719608
65. Chalamaiah, M, kumar, BD, Hemalatha, R, and Jyothirmayi, T. Fish protein hydrolysates: proximate composition, amino acid composition, antioxidant activities and applications: a review. Food Chem. (2012) 135:3020–38. doi: 10.1016/j.foodchem.2012.06.100
66. Murakami, FY, Lima, DC, Souza, CMM, Kaele, GB, Oliveira, SG, and Félix, AP. Digestibility and palatability of isolated porcine protein in dogs. Ital J Anim Sci. (2018) 17:1070–6. doi: 10.1080/1828051X.2018.1443404
67. Kępińska-Pacelik, J, Biel, W, Mizielińska, M, and Iwański, R. Chemical composition and palatability of nutraceutical dog snacks. Appl Sci. (2023) 13:2806. doi: 10.3390/app13052806
68. Bill Kaelle, GC, Menezes Souza, CM, Bastos, TS, Vasconcellos, RS, Oliveira, SG, and Félix, AP. Diet digestibility and palatability and intestinal fermentative products in dogs fed yeast extract. Ital J Anim Sci. (2022) 21:802–10. doi: 10.1080/1828051X.2022.2054733
69. Case, LP, Daristotle, L, Hayek, MG, and Raasch, M. Canine and feline nutrition: A resource for companion animal professionals Elsevier Health Sciences (2010).
70. Samant, SS, Crandall, PG, Jarma Arroyo, SE, and Seo, HS. Dry pet food flavor enhancers and their impact on palatability: a review. Food Secur. (2021) 10:1–19. doi: 10.3390/foods10112599
71. Adler-Nissen, J. Enzymic hydrolysis of food proteins: Elsevier applied science publishers ; (1986).
72. Cho, MJ, Unklesbay, N, Hsieh, F-H, and Clarke, AD. Hydrophobicity of bitter peptides from soy protein hydrolysates. J Agric Food Chem. (2004) 52:5895–901. doi: 10.1021/jf0495035
73. Loeffler, A, Lloyd, D, Bond, R, Pfeiffer, D, and Kim, J. Dietary trials with a commercial chicken hydrolysate diet in 63 pruritic dogs. Vet Rec. (2004) 154:519–22. doi: 10.1136/vr.154.17.519
74. Hall, JA, Vondran, JC, Vanchina, MA, and Jewell, DE. When fed foods with similar palatability, healthy adult dogs and cats choose different macronutrient compositions. J Exp Biol. (2018) 221:1–11. doi: 10.1242/jeb.173450
75. Hewson-Hughes, AK, Hewson-Hughes, VL, Colyer, A, Miller, AT, McGrane, SJ, Hall, SR, et al. Geometric analysis of macronutrient selection in breeds of the domestic dog, Canis lupus familiaris. Behav Ecol. (2012) 24:293–304. doi: 10.1093/beheco/ars168
76. Arab-Tehrany, E, Jacquot, M, Gaiani, C, Imran, M, Desobry, S, and Linder, M. Beneficial effects and oxidative stability of omega-3 long-chain polyunsaturated fatty acids. Trends Food Sci Technol. (2012) 25:24–33. doi: 10.1016/j.tifs.2011.12.002
77. German, JB . Food processing and lipid oxidation. Adv Exp Med Biol. (1999) 459:23–50. doi: 10.1007/978-1-4615-4853-9_3
78. Turner, R, Mclean, CH, and Silvers, KM. Are the health benefits of fish oils limited by products of oxidation? Nutr Res Rev. (2006) 19:53–62. doi: 10.1079/NRR2006117
79. Pinto, CFD, Bortolo, M, Marx, FR, and Trevizan, L. Characterisation of spray dried hydrolysed chicken liver powder: effects on palatability and digestibility when included as single source of animal protein in dog diets. Ital J Anim Sci. (2021) 20:2086–94. doi: 10.1080/1828051X.2021.1993091
80. Vastolo, A, Riedmüller, J, Cutrignelli, MI, and Zentek, J. Evaluation of the effect of different dietary lipid sources on dogs’ faecal microbial population and activities. Animals. (2022) 12:111368. doi: 10.3390/ani12111368
81. Kilburn, LR, Koester, LR, Schmitz-Esser, S, Serão, NVL, and Rossoni Serão, MC. High-fat diets led to OTU-level shifts in fecal samples of healthy adult dogs. Front Microbiol. (2020) 11:564160. doi: 10.3389/fmicb.2020.564160
82. Smith, EA, and Macfarlane, GT. Enumeration of amino acid fermenting bacteria in the human large intestine: effects of pH and starch on peptide metabolism and dissimilation of amino acids. FEMS Microbiol Ecol. (1998) 25:355–68. doi: 10.1111/j.1574-6941.1998.tb00487.x
83. Aguirre, M, Eck, A, Koenen, ME, Savelkoul, PHM, Budding, AE, and Venema, K. Diet drives quick changes in the metabolic activity and composition of human gut microbiota in a validated in vitro gut model. Res Microbiol. (2016) 167:114–25. doi: 10.1016/j.resmic.2015.09.006
84. Hussein, HS, Flickinger, EA, and Fahey, GC Jr. Petfood applications of inulin and oligofructose. J Nutr. (1999) 129:1454S–6S. doi: 10.1093/jn/129.7.1454S
85. Soares, NMM, Bastos, TS, Kaelle, GCB, de Souza, RBMS, de Oliveira, SG, and Félix, AP. Digestibility and palatability of the diet and intestinal functionality of dogs fed a blend of yeast cell wall and oregano essential oil. Animals. (2023) 13:52527. doi: 10.3390/ani13152527
86. Roediger, W, Moore, J, and Babidge, W. Colonic sulfide in pathogenesis and treatment of ulcerative colitis. Dig Dis Sci. (1997) 42:1571–9. doi: 10.1023/A:1018851723920
87. Zhao, X-T, McCamish, MA, Miller, RH, Wang, L, and Lin, HC. Intestinal transit and absorption of soy protein in dogs depend on load and degree of protein hydrolysis. J Nutr. (1997) 127:2350–6. doi: 10.1093/jn/127.12.2350
88. Flock, MR, Skulas-Ray, AC, Harris, WS, Etherton, TD, Fleming, JA, and Kris-Etherton, PM. Determinants of erythrocyte omega-3 fatty acid content in response to fish oil supplementation: a dose–response randomized controlled trial. J Am Heart Assoc. (2013) 2:e000513. doi: 10.1161/JAHA.113.000513
89. Walker, RE, Jackson, KH, Tintle, NL, Shearer, GC, Bernasconi, A, Masson, S, et al. Predicting the effects of supplemental EPA and DHA on the omega-3 index. Am J Clin Nutr. (2019) 110:1034–40. doi: 10.1093/ajcn/nqz161
90. Harris, WS, Tintle, NL, Imamura, F, Qian, F, Korat, AVA, Marklund, M, et al. Blood n-3 fatty acid levels and total and cause-specific mortality from 17 prospective studies. Nat Commun. (2021) 12:2329. doi: 10.1038/s41467-021-22370-2
91. Harris, WS, Jackson, KH, Carlson, H, Hoem, N, Dominguez, TE, and Burri, L. Derivation of the omega-3 index from EPA and DHA analysis of dried blood spots from dogs and cats. Vet Sci. (2023) 10:13. doi: 10.3390/vetsci10010013
92. Lindqvist, H, Dominguez, T, Dragøy, R, Ding, Y, and Burri, L. Comparison of fish, krill and flaxseed as omega-3 sources to increase the omega-3 index in dogs. Vet Sci. (2023) 10:162. doi: 10.3390/vetsci10020162
93. Dominguez, TE, Kaur, K, and Burri, L. Enhanced omega-3 index after long-versus short-chain omega-3 fatty acid supplementation in dogs. Vet Med Sci. (2021) 7:370–7. doi: 10.1002/vms3.369
94. Combarros, D, Castilla-Castano, E, Lecru, LA, Pressanti, C, Amalric, N, and Cadiergues, MC. A prospective, randomized, double blind, placebo-controlled evaluation of the effects of an n-3 essential fatty acids supplement (Agepi (R) omega 3) on clinical signs, and fatty acid concentrations in the erythrocyte membrane, hair shafts and skin surface of dogs with poor quality coats. Prostaglandins Leukotr Essential Fatty Acids. (2020) 159:102140. doi: 10.1016/j.plefa.2020.102140
95. Logas, D, and Kunkle, GA. Double-blinded crossover study with marine oil supplementation containing high-dose eicosapentaenoic acid for the treatment of canine pruritic skin disease. Vet Dermatol. (1994) 5:99–104. doi: 10.1111/j.1365-3164.1994.tb00020.x
Keywords: fish hydrolysate, fish oil, functionality, nutrient digestion, palatability, pet food
Citation: Cabrita ARJ, Maia MRG, Alves AP, Aires T, Rosa A, Almeida A, Martins R and Fonseca AJM (2024) Protein hydrolysate and oil from fish waste reveal potential as dog food ingredients. Front. Vet. Sci. 11:1372023. doi: 10.3389/fvets.2024.1372023
Edited by:
Patricia Massae Oba, University of Illinois at Urbana-Champaign, United StatesReviewed by:
Alexandra Rankovic, University of Guelph, CanadaKarla Dos Santos Felssner, Federal University of Paraná, Brazil
Copyright © 2024 Cabrita, Maia, Alves, Aires, Rosa, Almeida, Martins and Fonseca. This is an open-access article distributed under the terms of the Creative Commons Attribution License (CC BY). The use, distribution or reproduction in other forums is permitted, provided the original author(s) and the copyright owner(s) are credited and that the original publication in this journal is cited, in accordance with accepted academic practice. No use, distribution or reproduction is permitted which does not comply with these terms.
*Correspondence: Ana R. J. Cabrita, arcabrita@icbas.up.pt