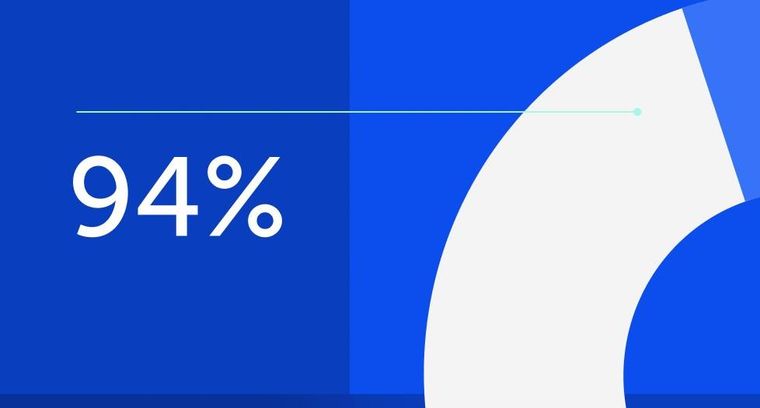
94% of researchers rate our articles as excellent or good
Learn more about the work of our research integrity team to safeguard the quality of each article we publish.
Find out more
ORIGINAL RESEARCH article
Front. Vet. Sci., 07 March 2024
Sec. Comparative and Clinical Medicine
Volume 11 - 2024 | https://doi.org/10.3389/fvets.2024.1365611
Introduction: Pancreatic islet isolation is essential for studying islet physiology, pathology, and transplantation, and feline islets could be an important model for human type II diabetes mellitus (T2D). Traditional isolation methods utilizing collagenases inflict damage and, in cats, may contribute to the difficulty in generating functional islets, as demonstrated by glucose-stimulated insulin secretion (GSIS). GLUT2 expression in β cells may allow for adaptation to hyperosmolar glucose solutions while exocrine tissue is selectively disrupted.
Methods: Here we developed a protocol for selective osmotic shock (SOS) for feline islet isolation and evaluated the effect of different hyperosmolar glucose concentrations (300 mmol/L and 600 mmol/L) and incubation times (20 min and 40 min) on purity, morphology, yield, and GSIS.
Results: Across protocol treatments, islet yield was moderate and morphology excellent. The treatment of 600 mmol/L glucose solution with 20 min incubation resulted in the highest stimulation index by GSIS.
Discussion: Glucose responsiveness was demonstrated, permitting future in vitro studies. This research opens avenues for understanding feline islet function and transplantation possibilities and enables an additional islet model for T2D.
In contrast to current rodent models, domestic cats naturally develop a form of diabetes mellitus (DM) that shares many similarities to type 2 diabetes (T2D) in humans, including obesity-induced insulin resistance and dyslipidemia, alterations in gut microbiota, impaired β-cell function, and spontaneous pancreatic amyloid deposition (1, 2). However, the utility of cats as a research model of type 2 diabetes has been hampered by the difficulty in studying feline islets in vitro. The ability to study islets in vitro is a critical component in the advancement of research into the prevention, targeted treatment, and etiology of DM.
The incidence of DM in cats is reported as approximately 1 in 100 cats presented to veterinary teaching hospitals (3) and approximately 1 in 200 cats presented to veterinary private practice (4). Like T2D in people, obesity, physical inactivity, and increased age are risk factors for DM in cats (5–7). Feline DM does not typically involve immune-mediated destruction of beta cells, but beta cell loss and dysfunction do occur (8–10), and unfortunately most cats are insulin-dependent upon diagnosis (11). Even with excellent owner compliance, daily insulin injections can be challenging, and DM can lead to a variety of complications such as infection, polyneuropathy, nephropathy, hypoglycemia, and diabetic ketoacidosis (8, 11). Therefore, islet transplantation might be worth exploring as a treatment option in feline DM. Whole pancreas transplantation allows for precise glycemic control but necessitates lifelong immunosuppression and is accompanied by high risk of organ rejection and perioperative complications (12–14). In contrast, islet isolation from exocrine pancreatic tissue enables targeted transplantation of only functionally necessary cells while minimizing host immune response and avoiding a surgical procedure. The site of clinical islet transplantation in people is the portal vein accessed via interventional radiology (15), but infusion into the peritoneal cavity and omentum have been reported in dogs, and may be more realistic transplant sites for the veterinary patient (16, 17). Additionally, isolated islets can be encapsulated, offering protection from the host immune system, eliminating the need for immunosuppression (15, 18). In humans, islet transplantation can result in 100% insulin independence at 1 year, and while this decreases to 30% at 5 years, recipients continue to have a 90% decrease in dangerous hypoglycemic episodes (15).
Protocols for islet isolation and culture have been developed and are routinely performed in other species, but these traditional methods involve enzymatic digestion and mechanical cell separation via density gradient centrifugation (19, 20). These methods can result in low islet yield and high rates of cell death (21–23). Consequently, most diabetic patients require multiple islet transplants to achieve satisfactory results (15). Collagenases are intended to disrupt connective tissue, but can also penetrate islet cell membranes, resulting in apoptosis, necrosis, and release of pro-inflammatory cytokines and free radicals, contributing to graft failure (24, 25). Atwater et al. (26) proposed an alternative method of islet isolation to circumvent some of these negative outcomes. This method exploits the GLUT 2 transporter to selectively spare beta cells from rapid changes in osmolality mediated by glucose concentration. In a high glucose hyperosmolar solution, glucose enters beta cells freely, preventing an osmolar gradient. Meanwhile, cells that do not express this membranal transporter are subjected to rapid changes in tonicity, cell membrane damage, and subsequent cell death. This selective osmotic shock technique has been successfully applied for islet isolation from porcine, canine, and human pancreata (26–28). Moreover, it is technically simpler and yields higher quantities compared to enzymatic digestions (26, 27).
Feline islet isolation has been described in a small number of studies with limited success utilizing traditional collagenase methods (29–32). To our knowledge, there is only one publication demonstrating yield of functional feline islets as determined by glucose-stimulated insulin secretion (GSIS) testing (32), which is the gold standard for assessing islet function (33). We hypothesized that use of selective osmotic shock (SOS) for isolation of feline islets will produce glucose-responsive beta cells. Our objectives in the current study were to (1) develop a selective osmotic shock protocol for feline islet isolation (2) describe purity and morphology and (3) compare the effects of osmolality and incubation time on islet yield and glucose responsiveness.
The study was approved by the Institutional Animal Care and Use Committee (protocol #202011101) at the University of Florida and conducted in accordance with all applicable regulations and guidelines, including the ARRIVE guidelines (34). Nine adult, aged 6–7 years, domestic shorthair cats from a laboratory colony housed at the University of Florida were used in this study. Body weight ranged from 3.9 to 7.2 kg (mean 5.3, SD 0.91). Four cats were female spayed, and five cats were male neutered. All animals were part of another study that involved partial pancreatectomy of the left pancreatic limb under general anesthesia. Anesthesia protocols were determined by the Animal Care and Use Veterinarian and consisted of premedication using Ketamine 2.5 mcg/kg, Dexmedetomidine 10 mcg/kg, and Butorphanol 0.2 mg/kg intramuscularly. Once sedated, cats underwent endotracheal intubation. Inhaled Isoflurane was administered if needed to facilitate intubation. Cats were maintained under anesthesia with inhaled Isoflurane in 100% oxygen. The resected tissue would have otherwise been discarded but instead was used in our study for islet isolation. Additional pancreatic tissue was obtained from a single cat that was euthanized for reasons unrelated to this study in accordance with AVMA guidelines for the Euthanasia of Animals (2020). This was a 2-year-old purpose-bred, male cat, and is cat 2 in Figure 1. All cats used in this study were healthy and without known pancreatic disease.
Figure 1. Islet yield among individual cats. Histogram (median) and interquartile range (25 and 75 percentiles) depicting islet yield for each cat, #1–10. For each cat, n = 4 (treatments A–D). Yield was compared between treatment groups and cats using a non-parametric ANOVA (Friedman’s) test (two tailed) and was found to be different among individual cats (p = 0.0025).
Following tissue harvest, the pancreatic tissue was submerged in RPMI 1640 media within a sterile specimen cup and covered in at least 1 inch of ice during transportation to the laboratory. Isolation was started within 15 min of harvest. Aseptic technique was used throughout the isolation method. The tissue in entirety was weighed using a gram scale and then divided into 4 comparable-sized pieces. These 4 pieces were randomly assigned to treatments A through D (Figure 2). Each segment was weighed individually using a gram scale so that yield could be standardized and reported per gram of tissue. Under a laminar flow hood at room temperature, the tissue segment was diced using a #10 surgical blade for approximately 10 min, or until fragments were < 3 mm. Each diced tissue segment was placed into 50 mL sterile centrifuge tubes with 30 mL Roswell Park Memorial Institute (RPMI) 1,640 zero glucose (Gibco™). Tissue homogenization was performed for approximately 30 s using a handheld tissue homogenizer (Tissue Tearor™, Biospec Products, Inc. Bartlesville, OK). The tubes were then centrifuged for 5 min at 180 relative centrifugal force (rcf) to produce a pellet. Media was decanted, and each homogenized tissue segment was resuspended in 30 mL of a hyperosmolar glucose solutions with the following protocols: treatment A; 300 mmol/L glucose for 20 min exposure time, treatment B; 300 mmol/L glucose for 40 min exposure time, treatment C; 600 mmol/L glucose for 20 min exposure time, and treatment D; 600 mmol/L glucose for 40 min exposure time. Experimental media were prepared prior to the experiments by adding appropriate amounts of glucose (Dextrose 50%, VetOne, Boise, ID) to glucose-free RPMI 1640 media.
Figure 2. Illustration of the division of the feline pancreas and randomized treatment group assignment for selective osmotic shock. Partial pancreatectomy is represented by the pancreas outlined in green while total pancreas extraction is represented by the pancreas outlined in red. The assignment of treatment groups A–D was random for each cat to avoid bias due to asymmetrical distribution of islets. Following treatment group assignment, the tissue was mechanically disrupted and then subjected to either 300 mmol/L glucose solution or 600 mmol/L glucose solution for either 20 min or 40 min. Illustration is not to scale.
The tissue fragments were periodically agitated during incubation at room temperature. After the respective treatment times, the tubes were centrifuged for 5 min at 180 rcf, media was decanted, and the pellet was resuspended in glucose-free RPMI media. Each sample was vigorously mixed and re-centrifuged for an additional 2 rinses using glucose-free RPMI for a total of 3 rinses. The tissue was then plated into 100 mm sterile polystyrene petri dishes and 3 mL of standard islet culture media composed of RPMI 1640 + 10% fetal bovine serum (Gibco™) + 1% Penicillin Streptomycin (Gibco™) and incubated at 37°C, 5% CO2. At 24 h after islet isolation, islets were hand-picked using a micropipette to increase purity and quantified per plate using an inverted light microscope (Olympus CKX53). Light microscopy imaging was performed with SPOT Idea CMOS microscope camera and SPOT Software 5.2 imaging software. Islet yield was then calculated by dividing the number of islets per treatment plate by the respective weight of the tissue segment. Purity (% islet tissue vs. % exocrine tissue) was estimated visually under light microscopy.
Islet β-cell function was measured with a modified version of a glucose-stimulated insulin secretion (GSIS) assay used in human islet transplant centers (Integrated Islet Distribution Program City of Hope, Standard Operating Procedure for GSIS).1 GSIS was performed 24 h after islet isolation on pancreata from cat 3–10 (n = 8) and was not performed on pancreata from cats 1 and 2 due to the lack of available consumables. Approximately 20 islets of similar size were handpicked with a micropipette from each treatment group and divided between 2 Eppendorf tubes (1.5 mL), each tube containing 500 μL of glucose-free RPMI 1640. The tubes were then pre-incubated for 2 h at 37°C. After incubation, the Eppendorf tubes were spun at low speed (180 rcf, 2 min), the glucose-free media was decanted, and each treatment group had 500 μL of a low glucose concentration RPMI (2.8 mmol/L) added to one tube and a high glucose concentration RPMI (28 mmol/L) added to the other tube. The researcher performing the experiment was blinded to the assignment of high (28 mmol/L) glucose concentration and low (2.8 mmol/L) glucose concentration during the islet handpicking as to minimize bias based on islet size and morphology. After 1 h incubation at 37°C, the tubes were again spun, and the media was removed and stored in a −20°C freezer for insulin determination. Insulin was measured using a commercially available feline insulin ELISA kit (Mercodia). Stimulation index was calculated using the standard formula:
The stimulation assay was performed in triplicates, for a total of 24 samples (approximately 60 islets per treatment group). In summary, three 10-islet Eppendorf tubes were tested at a low-glucose concentration and three 10-islet Eppendorf tubes were tested at a high-glucose concentration for each of the 4 treatment groups (treatment A–D) (Figure 3). Missing GSIS data for two pancreata in treatments B–D (n = 6 for these treatment groups) was due to limited sample and feline insulin ELISA kit.
Figure 3. Illustration of the glucose stimulated insulin secretion test. Treatment group B, represented by cell culture plate B, is used as an example to depict the procedure used for all treatment groups (A–D). For each treatment group, represented by cell culture plate A–D, 20 islets were hand-picked with a pipette and divided into two Eppendorf tubes for a 2-h pre-incubation in glucose-free RPMI 1640. Then, one group of 10 islets was exposed to a low glucose concentration (2.8 mmol/L) and the other group of islets was exposed to a high glucose concentration (28 mmol/L). After 1 h, the supernatant from each group was saved and analyzed for insulin concentration to determine stimulation index. This was performed in triplicate for each treatment group. Illustration is not to scale.
Data were analyzed using a commercial software package (GraphPad Prism, Version 10.0.2). Because of the small sample size, all data were analyzed with nonparametric tests and reported as median and range. For GSIS data, triplicates were first averaged into a single data point per treatment. Yield was compared between cats and between treatments using non-parametric ANOVA (Friedman’s) test (two-tailed). For GSIS, missing data precluded a repeated-measure analysis and treatments were compared using the Kruskal-Wallis test followed by Dunn’s test for multiple comparisons with adjusted p-values (two-tailed). Significance level was set to 0.05.
SOS produced isolated islets in all cats. Overall median (range) of islet yield was 690 (95–2,704) islets/g (n = 10). Treatment group did not have an effect on islet yield (p = 0.7) (Figure 4). Two outliers were identified in treatment group A, but even with removal of these outliers, there was no difference between treatment groups (p = 0.7). When evaluating the specific effects of osmolality of the glucose solution, islet/g median (range) for tissue exposed to 300 mmol/L glucose (611 [237–2,497], treatment groups A and B) was not different for tissue exposed to 600 mmol/L glucose (690 [95–2,704], treatment groups C and D). There was also no difference between exposure time on islet yield, in which tissue exposed for 20 min yielded 611 islets/g (95–2,497) and tissue exposed for 40 min yielded 666 islets/g (237–2,704). Islet yield differed among individual cats (p = 0.0025, Figure 1).
Figure 4. Islet yield among treatments. Histogram (median) and interquartile range (25 and 75 percentiles) depicting islet yield in treatment groups A (20 min incubation, 300 mmol/L glucose), B (40 min incubation, 300 mmol/L glucose), C (20 min incubation, 600 mmol/L glucose), and D (40 min incubation, 600 mmol/L glucose). For each treatment, n = 10. There was no difference in islet yield between treatment groups.
Islet purity (percentage of islets vs. acinar tissue) was estimated to be between 20 and 30% in all individuals and treatment groups, and there was no quantifiable difference between treatments. Visual assessment of morphology with light microscopy revealed islet sizes ranging from 50 to 150 μm. Observed islets were spherical, compact, and had well-rounded borders with congruent translucent capsular integrity (Figure 5). Subjectively, no differences in morphology were observed between treatments.
Figure 5. Isolated feline islets. Light microscopy photographs of feline islets isolated by SOS. Arrowheads (A) highlighting islets free of acinar cells. The surrounding floating amorphous material are acinar cells. Scale bars in both in panels (A, B): 100 μm. Note the intact peri-insular capsule, a marker of islet health.
Median (range) stimulation index (SI) for all islets in the study that underwent GSIS was 1.19 (0.06–3.61). Treatment group C (600 mmol/L glucose for 20 min) was found to have a higher (p = 0.017) median SI (2.03 [1.19–3.61]) compared to treatment group B (300 mmol/L glucose for 40 min) (0.69 [0.06–1.23] Figure 6). When osmolality was considered, median SI was higher (p = 0.0032) in islets incubated at 600 mmol/L glucose (1.95 [0.902–3.61]) compared to islets incubated at 300 mmol/L glucose (0.85 [0.06–2.56]). No difference (p = 0.25) was found between 20-min incubation (1.3 [0.48–3.61]) and 40-min incubation (0.92 [0.06–2.69]).
Figure 6. Stimulation index from glucose stimulated insulin secretion (GSIS). Histogram (median) and interquartile range (25 and 75 percentiles) depicting stimulation index (SI) in treatment groups A (20 min incubation, 300 mmol/L glucose) n = 8, B (40 min incubation, 300 mmol/L glucose) n = 6, C (20 min incubation, 600 mmol/L glucose) n = 6, and D (40 min incubation, 600 mmol/L glucose) n = 6. SI was significantly higher in treatment C compared to treatment B.
To our knowledge, this is the first description of SOS islet isolation in cats, and our results show that the SOS isolation protocol produces glucose-responsive β-cells, supporting our hypothesis. GSIS is a well-accepted measure of islet function, and healthy islets are expected to secrete increased insulin concentrations when moved from low to high concentrations of glucose (21, 35). In other species, healthy islets typically produce stimulation index >2 (35–37), but the standard for SI in human islet transplantation is an SI >1 (21). This is because clinical outcome of human islet transplant is not correlated with SI (18). Stimulation index can also be variable due to differences in protocols between institutions (37) and potentially because of intrinsic differences between species (35–37). There is only one study that has published on GSIS in isolated feline islets, and although this study used a different incubation time (30 min) and high glucose concentration (16.7 mM), the mean SI was approximately 2 (32), which is similar to our results in treatment group C. In vivo insulin stimulation testing suggests that glucose is a less effective stimulator of insulin secretion in cats compared to humans and that arginine may be superior to glucose for assessing insulin secretory capacity (38). Our results showed that islets isolated with 600 mmol/L glucose solution had higher SI than islets isolated with 300 mmol/L glucose solution. In canine islets, no significant difference was found between these two osmolalities, however, islets isolated with 300 mmol/L glucose solutions had higher trending SI than those isolated with 600 mmol/L glucose (27). It was suggested that higher glucose concentrations in dogs may affect islet function and viability, and although no difference in islet viability was found between osmolalites, there was a higher percentage of acinar cell death in the higher osmolality treatments (26). Unlike dogs, our results suggest that a higher osmolality could be optimal for isolation by SOS in cats. However, viability was not assessed, which is one of the limitations of this study.
Feline islets may be more sensitive to collagenases than islets of other species, and one proposed reason for this is that there is less basement membrane-like material and collagen surrounding feline islets and separating them from acinar tissue (30, 31). Higher amounts of collagen directly surrounding islets has been associated with higher purity (39) after enzymatic islet isolation procedures in other species, while higher amounts of total collagen in the pancreas are also associated with more difficult islet isolation. Thus, it is possible that traditional enzymatic islet isolation methods have a causal relationship with the limited success in producing glucose-responsive feline islets in the literature. The use of collagenases inherently cause damage to islets, injuring basement membranes and extracellular matrix, which causes a localized pro-inflammatory response via free radicals and cytokines (23–25, 40). Islets have even been shown to internalize these enzymes, resulting in decreased function and cell death (24). This results in the selection for resilience to collagenase-based procedures, which is unlikely to be uniform among islet size, functionality, and micro-architecture (41–43). Conversely, SOS likely selects for islets that have a higher density of GLUT 2 transporters, which has been identified as a favorable indicator of islet function and glucose responsiveness, (26, 44, 45) desirable traits for transplantation as well as in vitro studies.
The SOS isolation method has been shown to yield higher islets per gram of tissue (13,423 islets/g) (26) compared to Liberase-treated porcine pancreata (4,210 islets/g) (46) and compared to human cadaveric pancreata (2,279 islets/g) (19). In dogs, the SOS protocol had lower yield (28×103 islet-like equivalents) compared to traditional methods (49 to 234×103 islet-like equivalents) (47), however, delay in quantification and warm ischemia are suggested to have affected yield (27). There is minimal information on feline islet yield, with a single publication showing a mean yield of islet-like cell clusters (±SD) to be 2,200 ± 1,400 from a mean 3.8 ± 1.0 g of pancreas (30). Other publications have not reported yield(29, 31, 32). SOS isolation in feline donors, however, seems to have similar to increased yield when compared to traditional methods. Warm ischemia during islet isolation could have affected islet yield, and performing this step under cooled conditions is recommended in the future. Our treatment groups were the same as in other studies on SOS, and we found that neither osmolality nor incubation time had an effect on islet yield. Thompson et al. had a similar finding in canine donors (27), while Atwater et al. (26) found highest yield in 600 mmol/L incubated for 20 min in porcine donors. It has been proposed that higher osmolality could increase yield while longer incubation time may increase damage to islets. However, our findings did not support this outcome in cats. Additionally, there was marked variability in yield among individual cats, despite being free of known pancreatic and circulatory disease, which could also affect our ability to perceive treatment differences. The explanation for such wide ranges in islet yields is unclear, but some potential explanations could include varying degrees of warm ischemia after harvest and subclinical pancreatic, cardiovascular, or metabolic disease that may have affected islet concentration. Unfortunately, histopathology was not performed to assess and compare pancreatic architecture and islet concentration differences among individuals, which is a limitation to this study. There was significant variability in human islet yield until Ricordi described a method for a semi-automation of the enzymatic isolation process (15, 19). It is possible that a similar system for automation of the degree of mechanical and chemical exposure of the islets will be required before the selective osmotic shock procedure results in consistent islet yield, as well.
There are no standard morphological assessment criteria for isolated islets, and morphology differs among species (48). Morphology scoring systems proposed in human islet xenotransplantation have shown to correlate with outcome, where higher scores consist of spherical islets, well-rounded borders, compact cells, and larger borders (49, 50). Peri-insular capsule integrity has also been recognized as marker of islet health, and in recent years collagenase-based methods have aimed to preserved to aim capsular integrity (25, 26, 46, 51, 52). Feline islets isolated by SOS meet the majority of high-quality morphological characteristics described in isolated islets of other species, with a notably prominent and congruent peri-insular capsule that has not been described or demonstrated in feline islets isolated by other methods (29–31).
SOS is technically simple, allows for the successful isolation of clinical grade quality islets from feline donors, and is considerably less costly than traditional methods. While this protocol will be useful in obtaining feline islets for in vitro studies of feline islet physiology, it still requires significant improvements in islet purity and yield before clinical application of islet transplantation. Following SOS, Thompson et al. (27) incorporated the use of a sieve and cell strainer prior to plating canine islets for cell culture. We found that, in feline islet isolation, this drastically compromised yield, therefore the only purification step performed was hand picking islets to separate them from acinar tissue. Visual estimation of purity is also a limitation of this study, and in the future the use of an image analysis software for quantification of purity is suggested. Clinical islet transplant materials for humans have significantly higher purity (78.5%), (50, 53) but collagenase-based techniques require an additional step in purity, such density-gradient centrifugation, which separates acinar tissue from islets using dextran or ficoll (19, 20). An additional step to improve purity and further refinement and automation of mechanical tissue disruption is likely needed for the current SOS protocol. Nevertheless, this protocol enables access to an additional islet model for human type II diabetes mellitus.
The raw data supporting the conclusions of this article will be made available by the authors, without undue reservation.
The animal study was approved by the Institutional Animal Care and Use Committee at the University of Florida. The study was conducted in accordance with the local legislation and institutional requirements.
LP: Conceptualization, Data curation, Formal analysis, Funding acquisition, Investigation, Methodology, Project administration, Resources, Writing – original draft, Writing – review & editing. CA: Conceptualization, Investigation, Methodology, Project administration, Resources, Supervision, Writing – original draft, Writing – review & editing. CC: Data curation, Project administration, Writing – original draft, Writing – review & editing. JM: Data curation, Project administration, Writing – original draft, Writing – review & editing. CG: Conceptualization, Data curation, Formal analysis, Funding acquisition, Investigation, Methodology, Project administration, Resources, Supervision, Writing – original draft, Writing – review & editing.
The author(s) declare financial support was received for the research, authorship, and/or publication of this article. Funding was provided by the American College of Veterinary Internal Medicine Resident Research Grant sponsored by the Purina Institute (P0204319).
Thank you to Ann Chan and Oscar Hernandez of the 6-Pack Lab for technical assistance. Thank you to Brad Case and the Bolser lab for tissue donations.
The authors declare that the research was conducted in the absence of any commercial or financial relationships that could be construed as a potential conflict of interest.
All claims expressed in this article are solely those of the authors and do not necessarily represent those of their affiliated organizations, or those of the publisher, the editors and the reviewers. Any product that may be evaluated in this article, or claim that may be made by its manufacturer, is not guaranteed or endorsed by the publisher.
1. ^https://www.protocols.io/view/potency-test-glucose-stimulated-insulin-release-as-36wgq5odkgk5/v3?step=49
1. Kieler, IN, Osto, M, Hugentobler, L, Puetz, L, Gilbert, MTP, Hansen, T, et al. Diabetic cats have decreased gut microbial diversity and a lack of butyrate producing bacteria. Sci Rep. (2019) 9:4822. doi: 10.1038/s41598-019-41195-0
2. Osto, M, Zini, E, Franchini, M, Wolfrum, C, Guscetti, F, Hafner, M, et al. Subacute Endotoxemia induces adipose inflammation and changes in lipid and lipoprotein metabolism in cats. Endocrinology. (2011) 152:804–15. doi: 10.1210/en.2010-0999
3. Prahl, A, Guptill, L, Glickman, NW, Tetrick, M, and Glickman, LT. Time trends and risk factors for diabetes mellitus in cats presented to veterinary teaching hospitals. J Feline Med Surg. (2007) 9:351–8. doi: 10.1016/j.jfms.2007.02.004
4. O’Neill, DG, Gostelow, R, Orme, C, Church, DB, Niessen, SJM, Verheyen, K, et al. Epidemiology of diabetes mellitus among 193,435 cats attending primary-care veterinary practices in England. J Vet Intern Med. (2016) 30:964–72. doi: 10.1111/jvim.14365
5. Scarlett, JM, and Donoghue, S. Associations between body condition and disease in cats. J Am Vet Méd Assoc. (1998) 212:1725–31. doi: 10.2460/javma.1998.212.11.1725
6. McCann, TM, Simpson, KE, Shaw, DJ, Butt, JA, and Gunn-Moore, DA. Feline diabetes mellitus in the UK: the prevalence within an insured cat population and a questionnaire-based putative risk factor analysis. J Feline Med Surg. (2007) 9:289–99. doi: 10.1016/j.jfms.2007.02.001
7. Lederer, R, Rand, JS, Jonsson, NN, Hughes, IP, and Morton, JM. Frequency of feline diabetes mellitus and breed predisposition in domestic cats in Australia. Vet J. (2009) 179:254–8. doi: 10.1016/j.tvjl.2007.09.019
8. Henson, MS, and O’Brien, TD. Feline models of type 2 diabetes mellitus. ILAR J. (2006) 47:234–42. doi: 10.1093/ilar.47.3.234
9. Rand, JS. Pathogenesis of feline diabetes. Vet Clin North Am: Small Anim Pr. (2013) 43:221–31. doi: 10.1016/j.cvsm.2013.01.003
10. Zini, E, Lunardi, F, Zanetti, R, Heller, RS, Coppola, LM, Ferro, S, et al. Endocrine pancreas in cats with diabetes mellitus. Vet Pathol. (2016) 53:136–44. doi: 10.1177/0300985815591078
11. O’Brien, TD. Pathogenesis of feline diabetes mellitus. Mol Cell Endocrinol. (2002) 197:213–9. doi: 10.1016/S0303-7207(02)00265-4
12. Aref, A, Zayan, T, Pararajasingam, R, Sharma, A, and Halawa, A. Pancreatic transplantation: brief review of the current evidence. World J Transplant. (2019) 9:81–93. doi: 10.5500/wjt.v9.i4.81
13. Scalea, JR, Pettinato, L, Fiscella, B, Bartosic, A, Piedmonte, A, Paran, J, et al. Successful pancreas transplantation alone is associated with excellent self-identified health score and glucose control: a retrospective study from a high-volume center in the United States. Clin Transpl. (2018) 32:e13177. doi: 10.1111/ctr.13177
14. Mittal, S, Johnson, P, and Friend, P. Pancreas transplantation: solid organ and islet. Cold Spring Harb Perspect Med. (2014) 4:a015610. doi: 10.1101/cshperspect.a015610
15. Marfil-Garza, BA, Imes, S, Verhoeff, K, Hefler, J, Lam, A, Dajani, K, et al. Pancreatic islet transplantation in type 1 diabetes: 20-year experience from a single-Centre cohort in Canada. Lancet Diabetes Endocrinol. (2022) 10:519–32. doi: 10.1016/S2213-8587(22)00114-0
16. Ao, Z, Matayoshi, K, Lakey, JRT, Rajotte, RV, and Warnock, GL. Survival and function of purified islets in the omental pouch site of outbred dogs. Transplantation. (1993) 56:524–9. doi: 10.1097/00007890-199309000-00007
17. Wahoff, DC, Nelson, LA, Papalois, BE, Curtis, DA, Gores, PF, and Sutherland, DE. Decreased chronic failure of intraperitoneal impure islets versus intrahepatic pure islets in a canine autograft model. Transplant Proc. (1995) 27:3186.
18. Farney, AC, Sutherland, DER, and Opara, EC. Evolution of islet transplantation for the last 30 years. Pancreas. (2016) 45:8–20. doi: 10.1097/MPA.0000000000000391
19. Ricordi, C, Lacy, PE, and Scharp, DW. Automated islet isolation from human pancreas. Diabetes. (1989) 38:140–2.
20. Warnock, GL, Cattral, MS, Evans, MG, Kneteman, NM, and Rajotte, RV. Mass isolation of pure canine islets. Transplant Proc. (1989) 21:3371–2.
21. Papas, KK, Suszynski, TM, and Colton, CK. Islet assessment for transplantation. Curr Opin Organ Transplant. (2009) 14:674–82. doi: 10.1097/MOT.0b013e328332a489
22. Vrabelova, D, Adin, CA, Kenzig, A, Gilor, C, Xu, F, Buss, JL, et al. Evaluation of a high-yield technique for pancreatic islet isolation from deceased canine donors. Domest Anim Endocrinol. (2014) 47:119–26. doi: 10.1016/j.domaniend.2013.01.006
23. Rosenberg, L, Wang, R, Paraskevas, S, and Maysinger, D. Structural and functional changes resulting from islet isolation lead to islet cell death. Surgery. (1999) 126:393–8. doi: 10.1016/S0039-6060(99)70183-2
24. Barnett, MJ, Zhai, X, LeGatt, DF, Cheng, SB, Shapiro, AMJ, and Lakey, JRT. Quantitative assessment of collagenase blends for human islet isolation. Transplantation. (2005) 80:723–8. doi: 10.1097/01.TP.0000174133.96802.DE
25. Kin, T, Johnson, PRV, Shapiro, AMJ, and Lakey, JRT. Factors influencing the collagenase digestion phase of human islet isolation. Transplantation. (2007) 83:7–12. doi: 10.1097/01.tp.0000243169.09644.e6
26. Atwater, I, Guajardo, M, Caviedes, P, Jeffs, S, Parrau, D, Valencia, M, et al. Isolation of viable porcine islets by selective osmotic shock without enzymatic digestion. Transplant Proc. (2010) 42:381–6. doi: 10.1016/j.transproceed.2009.11.030
27. Thompson, EM, Sollinger, JL, Opara, EC, and Adin, CA. Selective osmotic shock for islet isolation in the cadaveric canine pancreas. Cell Transplant. (2017) 27:542–50. doi: 10.1177/0963689717752947
28. Enck, K, McQuilling, JP, Orlando, G, Tamburrini, R, Sivanandane, S, and Opara, EC. Cell microencapsulation, methods and protocols. Methods Mol Biol. (2016) 1479:191–8. doi: 10.1007/978-1-4939-6364-5_14
29. Brandão, IR, Zini, E, Reusch, CE, Lutz, TA, and Osto, M. Establishment of a protocol for the isolation of feline pancreatic islets. Physiol Behav. (2018) 186:79–81. doi: 10.1016/j.physbeh.2018.01.012
30. Maeno, T, Inoue, M, Embabi, SN, Miki, D, and Hatchell, DL. Islet-like cell clusters: viability, cell types, and subretinal transplantation in pancreatectomized cats. Lab Anim. (2006) 40:432–46. doi: 10.1258/002367706778476488
31. Zini, E, Franchini, M, Guscetti, F, Osto, M, Kaufmann, K, Ackermann, M, et al. Assessment of six different collagenase-based methods to isolate feline pancreatic islets. Res Vet Sci. (2009) 87:367–72. doi: 10.1016/j.rvsc.2009.03.017
32. Strage, EM, Ley, C, Westermark, GT, and Tengholm, A. Insulin release from isolated cat islets of Langerhans. Domest Anim Endocrinol. (2024) 87:106836. doi: 10.1016/j.domaniend.2023.106836
33. Corbin, KL, West, HL, Brodsky, S, Whitticar, NB, Koch, WJ, and Nunemaker, CS. A practical guide to rodent islet isolation and assessment revisited. Biol Proced Online. (2021) 23:7. doi: 10.1186/s12575-021-00143-x
34. Sert, NP du, Ahluwalia, A, Alam, S, Avey, MT, Baker, M, Browne, WJ, et al. Reporting animal research: explanation and elaboration for the ARRIVE guidelines 2.0. PLoS Biol. (2020) 18:e3000411. doi: 10.1371/journal.pbio.3000411
35. Carter, JD, Dula, SB, Corbin, KL, Wu, R, and Nunemaker, CS. A practical guide to rodent islet isolation and assessment. Biol Proced Online. (2009) 11:3–31. doi: 10.1007/s12575-009-9021-0
36. Woolcott, OO, Bergman, RN, Richey, JM, Kirkman, EL, Harrison, LN, Ionut, V, et al. Simplified method to isolate highly pure canine pancreatic islets. Pancreas. (2012) 41:31–8. doi: 10.1097/MPA.0b013e318221fd0e
37. Sakata, N, Egawa, S, Sumi, S, and Unno, M. Optimization of glucose level to determine the stimulation index of isolated rat islets. Suizo. (2008) 23:756–7. doi: 10.2958/suizo.23.756
38. Kitamura, T, Yasuda, J, and Hashimoto, A. Acute insulin response to intravenous arginine in nonobese healthy cats. J Vet Intern Med. (1999) 13:549–56. doi: 10.1111/j.1939-1676.1999.tb02208.x
39. van Suylichem, PTR, van Deijnen, J-EHM, Wolters, GHJ, and van Schilfgaarde, R. Amount and distribution of collagen in pancreatic tissue of different species in the perspective of islet isolation procedures. Cell Transplant. (1995) 4:609–14. doi: 10.1177/096368979500400610
40. O’Gorman, D, Kin, T, McGhee-Wilson, D, Shapiro, AMJ, and Lakey, JRT. Multi-lot analysis of custom collagenase enzyme blend in human islet isolations. Transplant Proc. (2005) 37:3417–9. doi: 10.1016/j.transproceed.2005.09.139
41. Cabrera, O, Berman, DM, Kenyon, NS, Ricordi, C, Berggren, PO, and Caicedo, A. The unique cytoarchitecture of human pancreatic islets has implications for islet cell function. Proc Natl Acad Sci. (2006) 103:2334–9. doi: 10.1073/pnas.0510790103
42. Vantyghem, MC, Kerr-Conte, J, Pattou, F, Gevaert, MH, Hober, C, Defossez, A, et al. Immunohistochemical and ultrastructural study of adult porcine endocrine pancreas during the different steps of islet isolation. Histochem Cell Biol. (1996) 106:511–9. doi: 10.1007/BF02473314
43. Mahler, R, Franke, FE, Hering, BJ, Brandhorst, D, Brandhorst, H, Brendel, MD, et al. Evidence for a significant correlation of donor pancreas morphology and the yield of isolated purified human islets. J Mol Med. (1999) 77:87–9. doi: 10.1007/s001090050308
44. Novelli, M, Tata, VD, Bombara, M, Bergamini, E, and Masiello, P. Age-dependent reduction in GLUT-2 levels is correlated with the impairment of the insulin secretory response in isolated islets of Spragueâ–Dawley rats. Exp Gerontol. (2000) 35:641–51. doi: 10.1016/S0531-5565(00)00100-5
45. Chen, L, Alam, T, Johnson, JH, Hughes, S, Newgard, CB, and Unger, RH. Regulation of beta-cell glucose transporter gene expression. Proc Natl Acad Sci. (1990) 87:4088–92. doi: 10.1073/pnas.87.11.4088
46. Brandhorst, H, Brandhorst, D, Hering, BJ, and Bretzel, RG. Significant progress in porcine islet mass isolation utilizing liberase HI for enzymatic low-temperature digestion. Transplantation. (1999) 68:355–61. doi: 10.1097/00007890-199908150-00006
47. Lakey, JRT, Cavanagh, TJ, Zieger, MAJ, Albertson, TE, Dwulet, F, Wright, MJ, et al. Evaluation of a purified enzyme blend for the recovery and in vitro function of isolated canine islets. Transplant Proc. (1998) 30:590–1. doi: 10.1016/S0041-1345(97)01419-X
48. Steiner, DJ, Kim, A, Miller, K, and Hara, M. Pancreatic islet plasticity: interspecies comparison of islet architecture and composition. Islets. (2010) 2:135–45. doi: 10.4161/isl.2.3.11815
49. Komatsu, H, Qi, M, Gonzalez, N, Salgado, M, Medrano, L, Rawson, J, et al. A multiparametric assessment of human islets predicts transplant outcomes in diabetic mice. Cell Transplant. (2021) 30:096368972110522. doi: 10.1177/09636897211052291
50. Salgado, M, Gonzalez, N, Medrano, L, Rawson, J, Omori, K, Qi, M, et al. Semi-automated assessment of human islet viability predicts transplantation outcomes in a diabetic mouse model. Cell Transplant. (2020) 29:096368972091944. doi: 10.1177/0963689720919444
51. Hughes, SJ, Clark, A, McShane, P, Contractor, HH, Gray, DWR, and Johnson, PRV. Characterisation of collagen VI within the islet-exocrine Interface of the human pancreas: implications for clinical islet isolation? Transplantation. (2006) 81:423–6. doi: 10.1097/01.tp.0000197482.91227.df
52. van Deijnen, JHM, Hulstaert, CE, Wolters, GHJ, and van Schilfgaarde, R. Significance of the peri-insular extracellular matrix for islet isolation from the pancreas of rat, dog, pig, and man. Cell Tissue Res. (1992) 267:139–46. doi: 10.1007/BF00318700
Keywords: diabetes mellitus, islet isolation, feline, GLUT2, selective osmotic shock, β-cell
Citation: Porter LT, Adin CA, Crews CD, Mott J and Gilor C (2024) Isolation of feline islets of Langerhans by selective osmotic shock produces glucose responsive islets. Front. Vet. Sci. 11:1365611. doi: 10.3389/fvets.2024.1365611
Received: 04 January 2024; Accepted: 26 February 2024;
Published: 07 March 2024.
Edited by:
Thomas Schermerhorn, Kansas State University, United StatesReviewed by:
John Lee Robertson, Virginia Tech, United StatesCopyright © 2024 Porter, Adin, Crews, Mott and Gilor. This is an open-access article distributed under the terms of the Creative Commons Attribution License (CC BY). The use, distribution or reproduction in other forums is permitted, provided the original author(s) and the copyright owner(s) are credited and that the original publication in this journal is cited, in accordance with accepted academic practice. No use, distribution or reproduction is permitted which does not comply with these terms.
*Correspondence: Chen Gilor, Y2dpbG9yQHVmbC5lZHU=
Disclaimer: All claims expressed in this article are solely those of the authors and do not necessarily represent those of their affiliated organizations, or those of the publisher, the editors and the reviewers. Any product that may be evaluated in this article or claim that may be made by its manufacturer is not guaranteed or endorsed by the publisher.
Research integrity at Frontiers
Learn more about the work of our research integrity team to safeguard the quality of each article we publish.