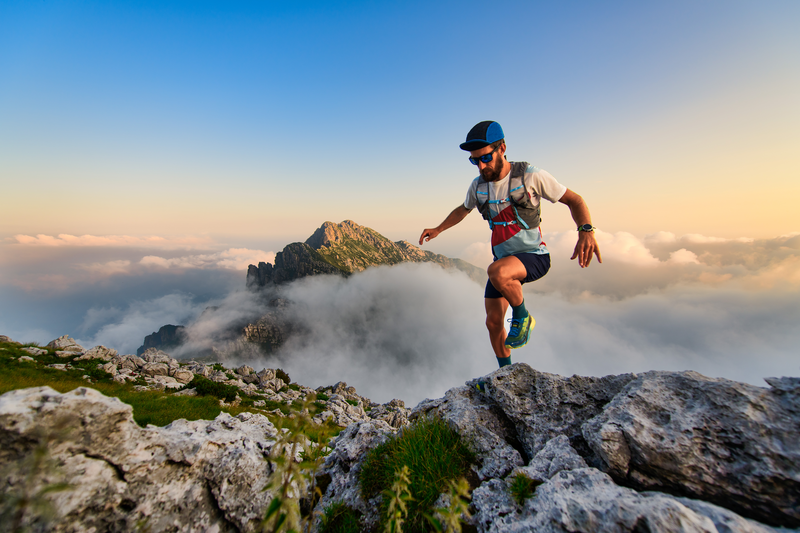
94% of researchers rate our articles as excellent or good
Learn more about the work of our research integrity team to safeguard the quality of each article we publish.
Find out more
ORIGINAL RESEARCH article
Front. Vet. Sci. , 09 May 2024
Sec. Veterinary Infectious Diseases
Volume 11 - 2024 | https://doi.org/10.3389/fvets.2024.1346713
Equine leptospirosis can result in abortion, stillbirth, neonatal death, placentitis, and uveitis. Horses can also act as subclinical reservoir hosts of infection, which are characterized as asymptomatic carriers that persistently excrete leptospires and transmit disease. In this study, PCR and culture were used to assess urinary shedding of pathogenic Leptospira from 37 asymptomatic mares. Three asymptomatic mares, designated as H2, H8, and H9, were PCR-positive for lipL32, a gene specific for pathogenic species of Leptospira. One asymptomatic mare, H9, was culture-positive, and the recovered isolate was classified as L. kirschneri serogroup Australis serovar Rushan. DNA capture and enrichment of Leptospira genomic DNA from PCR-positive, culture-negative samples determined that asymptomatic mare H8 was also shedding L. kirschneri serogroup Australis, whereas asymptomatic mare H2 was shedding L. interrogans serogroup Icterohaemorrhagiae. Sera from all asymptomatic mares were tested by the microscopic agglutination test (MAT) and 35 of 37 (94.6%) were seropositive with titers ranging from 1:100 to 1:3200. In contrast to asymptomatic mares, mare H44 presented with acute spontaneous abortion and a serum MAT titer of 1:102,400 to L. interrogans serogroup Pomona serovar Pomona. Comparison of L. kirschneri serogroup Australis strain H9 with that of L. interrogans serogroup Pomona strain H44 in the hamster model of leptospirosis corroborated differences in virulence of strains. Since lipopolysaccharide (LPS) is a protective antigen in bacterin vaccines, the LPS of strain H9 (associated with subclinical carriage) was compared with strain H44 (associated with spontaneous abortion). This revealed different LPS profiles and immunoreactivity with reference antisera. It is essential to know what species and serovars of Leptospira are circulating in equine populations to design efficacious vaccines and diagnostic tests. Our results demonstrate that horses in the US can act as reservoir hosts of leptospirosis and shed diverse pathogenic Leptospira species via urine. This report also details the detection of L. kirschneri serogroup Australis serovar Rushan, a species and serotype of Leptospira, not previously reported in the US.
Leptospirosis is a bacterial, zoonotic, and much neglected disease that causes significant morbidity and mortality in domestic animals. The causative agents, pathogenic species of the genus Leptospira, are excreted via urine or found in the genital tract of domestic livestock and can survive in suitable moist environmental conditions to facilitate additional disease transmission (1, 2). To date, 41 pathogenic species of Leptospira have been described comprising hundreds of serovars (2–6). Human leptospirosis is estimated to cause 1.03 million cases and 58,900 deaths each year (7).
Equine leptospirosis can result in abortion, stillbirth, neonatal death, placentitis, and uveitis (1, 8, 9). Diagnostic procedures for leptospirosis fall into two groups: (1) antibody detection and (2) direct demonstration of the presence of leptospires. The microscopic agglutination test (MAT) is the antibody diagnostic assay of choice. A rising antibody titer in paired acute and convalescent sera concurrent with clinical signs of acute disease is diagnostic (1). The presence of antibody in fetal serum is diagnostic of fetal infection. Direct detection of leptospires is facilitated by molecular assays and/or culture. Culture is definitive and provides an isolate that can be comprehensively characterized by genome sequencing and serotyping. Leptospira interrogans serogroup Pomona serovar Pomona type kennewicki was cultured from tissues and placenta associated with aborted equine fetuses in the US (10–14).
In Europe, serogroup Pomona has been identified as a cause of equine abortion as have the serogroups Australis, Hebdomadis, and Icterohaemorrhagiae (15). In Europe, equine recurrent uveitis is associated with serogroups Grippotyphosa, Australis, Sejroe, Pomona, and Javanica (16, 17). Horses in Europe have also been identified as reservoir hosts of Leptospira, asymptomatic carriers that persistently shed live pathogenic Leptospira via urine into the environment to maintain disease transmission (18). In Northern Ireland, it is hypothesized that horses act as a reservoir host for L. interrogans serogroup Australis serovar Bratislava, which has been cultured from equine kidneys (19). Seroprevalence studies on horses throughout the world demonstrate high levels of reactivity with serogroup Australis serovar Bratislava, which is used to further support the role of horses serving as reservoir hosts for L. interrogans serogroup Australis serovar Bratislava on a global level (20–25).
The MAT serological assay cannot diagnose horses acting as reservoir hosts of infection since seroprevalence studies in normal equine populations demonstrate exposure, not active clinical infection (1, 21, 26, 27). Direct detection of leptospires by culture or molecular methods is required to identify asymptomatic reservoir hosts of infection (1, 28, 29). However, culture is not routinely performed due to the fastidious growth requirements of Leptospira, the length of time, and the high levels of expertise required (30, 31). To design efficacious vaccination and diagnostic strategies for equine leptospirosis, it is essential to identify infected animals and determine both the genotype (species) and phenotype (serogroup/serovar) of Leptospira species associated with equine infections. Successful culture provides isolates that can be comprehensively characterized and used in bacterin vaccines. Since there are no published reports confirming that horses in the US act as reservoir hosts of leptospirosis, a population of asymptomatic thoroughbred mares was screened by molecular assays to determine if any were shedding Leptospira via urine. Samples from positive mares were then further examined by culture and Leptospira genome enrichment techniques to identify the species and serotype of Leptospira associated with subclinical carriage and for comparison with a strain of Leptospira commonly associated with overt clinical infection in horses.
A total of 37 asymptomatic thoroughbred mares from farm #1 in central Kentucky with no history of leptospirosis were sampled from December 2022 to February 2023. Blood was collected by jugular venipuncture into serum separator tubes (Vacutainer®, BD Diagnostics, Franklin Lakes, NJ, United States). Urine for PCR was collected by free catch and chilled on ice. In brief, the tail was wrapped, and sterile water used to remove all visible debris and thoroughly cleaned the vulva and surrounding area. Mid-stream urine was collected into sterile containers and shipped on ice packs for processing by PCR within 24 h. To collect urine for culture, if a mare was PCR-positive, the tail was wrapped and pulled away from the perineal area. Sterile water was used to remove all visible debris and thoroughly clean the vulva and surrounding area. The vulvar region was cleaned lightly with 70% ethyl alcohol and gently patted dry. The process was repeated if any contamination of the site occurred prior to urination. A diuretic was administered by intravenous injection (furosemide 5% injectable, 1 mg/kg based on estimated weight of mare), and midstream urine from successive voids was collected into sterile containers. The urine was passed to an assistant with clean gloves, and a sterile pipette was used to transfer 1 mL of urine from each voided sample to separate conical tubes containing 9 mL of HAN medium containing 5-fluorouracil (100 μg/μL) (30). Remaining urine from this second collection was transported on ice packs for a repeat PCR within 24 h.
Samples from farm #2 in central Kentucky, a farm with a very recent history of abortion by Leptospira as diagnosed by the University of Kentucky Veterinary Diagnostic Laboratory, were collected from a single mare suffering from an abortion in the final trimester of gestation (16 January 2023). Urine, chorioallantoic membrane, amnion, and allantoic fluid were immediately shipped on ice packs for processing within 24 h. A second set of samples collected 2 days later (18 January 2023), including urine, exudate, and a uterine swab, were inoculated directly into HAN medium and immediately shipped for processing within 24 h.
The MAT was performed using a panel of 18 antigens representative of 15 serogroups (Supplementary Table S1), as previously described (32, 33). A titer was considered positive at ≥1:100. Sera were also tested for reactivity in the MAT with the strain H9, an isolate recovered from a mare in this study.
The gene encoding the major outer membrane protein LipL32 discriminates pathogenic species of Leptospira from saprophytes. Before testing equine urine samples by lipL32 rtPCR (34, 35), preliminary studies were performed with spiked equine urine to optimize parameters for equine urine storage and pre-processing. In brief, 1 mL of L. borgpetersenii serovar Tarassovi strain Perepelitsin, at a density of 108 leptospires/mL, was inoculated into 9 mL of freshly collected equine urine. A 1 mL aliquot of this was serially diluted 10-fold to prepare spiked samples containing 107 Leptospira/mL to 100 Leptospira/mL urine. Intact motile leptospires were enumerated by dark-field microscopy as previously described (36). In total, 1 mL of each dilution was then processed for the extraction of DNA after storage in various conditions/time points, including: (A) that same day, (B) that same day after centrifugation at 900 × g for 10 min to remove “sludge,” (C) after storage for 24 h in a Styrofoam container with ice packs, (D) after storage for 24 h in a Styrofoam container with ice packs followed by centrifugation to remove “sludge,” (E) after storage for 48 h in a Styrofoam container with ice packs, (F) after storage for 48 h in a Styrofoam container with ice packs followed by centrifugation to remove “sludge,” (G) after freezing at −20°C for 24 h, and (H) after samples had been centrifuged at 900 × g to remove “sludge” and stored at −20°C for 24 h. Storage treatments A to H correlate with labels are shown in Supplementary Figure S1. Thereafter, urine was centrifuged at 12,000 × g for 30 min. The supernatant was removed, and the pellets were washed twice by resuspending in 1 mL phosphate buffered saline (PBS) and centrifuging at 12,000 × g for 15 min, leaving the final pellets in ~100 μL. DNA was extracted from the urinary pellet using the Maxwell RSC Purefood Purification Pathogen Kit (Promega Corporation, Madison, Wisconsin, United States), following the manufacturer’s instructions, except using a 1 h incubation with 200 μL lysis buffer A and a 100 μL elution volume (37). lipL32 rtPCR was performed using 10 μL of Perfect taq qPCR ToughMix low ROX™ (Quantabio, Beverly, MA, United States), 400 nmol/L of each primer, 132.5 nmol/L of probe, TaqMan™ Exogenous Internal Positive Control Reagents: 2 μL of 10X Exo IPC Mix, 0.4 μL of 50X Exo IPC DNA, and 5 μL of DNA extract from culture or sample. rtPCR cycling was conducted on a QuantStudio™ 7 (Thermo Fisher Scientific, United States) starting with an initial 3 min denaturation at 95°C for Taq polymerase activation, followed by 40 cycles of denaturation at 95°C for 15 s and primer annealing and extension at 60°C for 1 min. PCR of samples was performed in triplicate and considered positive by lipL32 rtPCR when duplicate or triplicates were positive with Ct values <40, as previously described (34, 35). DNA from L. borgpetersenii serovar Tarassovi strain Perepelitsin was used to prepare a standard curve as previously described (38).
A 45 mL aliquot of urine from each asymptomatic mare was centrifuged at 900 × g for 10 min at 4°C, and the supernatant was transferred to a clean 50 mL conical tube. Urine was then centrifuged at 12,000 × g for 30 min at 4°C. The supernatant was removed, and the pellet washed twice by resuspending in 1 mL of PBS and centrifuging at 12,000 × g for 10 min at 4°C. DNA was extracted as described above, and lipL32 rtPCR was performed as previously described (34, 35).
A 1 mL aliquot of freshly collected voided urine was immediately inoculated into 9 mL of HAN medium (30) and transported to the National Animal Disease Center (NADC), Ames, for culture of Leptospira, as previously described (37). Two 10-fold serial dilutions were made from the initial inoculum (i.e., 500 μL) into 5 mL of liquid HAN medium and incubated at 37°C in 5% CO2. Vulvar exudate and chorioallantois samples were vortexed with 9 mL of HAN medium and two 10-fold serial dilutions were made (i.e., 500 μL) into 5 mL of liquid HAN at 37°C in 5% CO2. Inoculated tubes were examined daily by darkfield microscopy for the first 2 weeks and then periodically for 6 months.
DNA was extracted from a 5 mL culture of the isolated strains using the Maxwell RSC Purefood Purification Pathogen Kit (Promega Corporation, Madison, WI), following the manufacturer’s instructions. The genomic DNA concentration was determined by Qubit (Qubit dsDNA Broad Range Assay Kit, Qubit 3.0 fluorometer, Invitrogen, Carlsbad, CA, United States). Illumina whole-genome sequence (WGS) was obtained (Nextera XT DNA Library Preparation Kit and the MiSeq Sequencer, 2 × 250 v2 paired-end chemistry, Illumina, San Diego, CA, United States), according to the manufacturer’s instructions. Illumina WGS reads were taxonomically identified using Kraken 2 version 2.1 (39). Reads were assembled with SPAdes 3.13 (40) and verified by comparing the expected genome size with the actual assembly size and verifying contigs as Leptospira by BLASTN (41) against the NCBI nucleotide (NT) database.
The serogroup of strains H9 and H44 were determined by the MAT method using a panel of polyclonal rabbit reference antisera representing 13 serogroups (Supplementary Table S2). The serovar of strains H9 and H44 was determined by performing MAT with panels of monoclonal antibodies (mAbs) that characteristically agglutinate serovars from the serogroups Australis and Pomona, respectively, as previously described (42).
DNA from two Leptospira PCR-positive culture-negative urine samples (designated as H2 and H8) was subjected to pan-pathogenic Leptospira DNA capture and enrichment, as previously described (43). The two samples were processed differently because DNA from sample H8 was highly fragmented and displayed low concentrations of nucleic acids (~0.5 ng/μL), whereas sample H2 had DNA that was more intact (average fragment size >6,000 bp) and concentrated (~4 ng/μL). For sample H2, the DNA was diluted to ~2 ng/μL in a volume of 40 μL and sonicated to an average size of 228 bp using a Q800R2 sonicator (QSonica, Newtown, CT, United States). For sample H8, 40 μL of undiluted DNA was subjected to brief sonication, and the final average fragment size was 103 bp. Short-read next-generation libraries were prepared separately using Agilent Sure-Select methodology. The libraries were then pooled together in equimolar amounts and were subjected to one round of DNA capture and enrichment and then sequenced on an Illumina MiSeq instrument using a MiSeq v3 600 cycle kit (2 × 300bp reads).
To estimate the percentage of Leptospira reads in the enriched sequences, reads were mapped against the standard Kraken database with Kraken v2.1.2 (39). Reads assigned as Leptospira were then extracted and assembled using SPAdes v3.13.0 (40) with default settings; assemblies were also generated for reads that were generated from isolates for samples H9 and H44. Assemblies for H2, H8, H9, and H44 were placed into a genus dendrogram containing 66 Leptospira reference genomes with Mashtree v1.2.046 (44) to confirm species identification. GenBank accession numbers for each genome are presented in figures.
Single nucleotide polymorphisms (SNPs) were identified among two enriched genomes (H2 and H8), two genomes from cultured isolates (H9 and H44), and 103 publicly available Leptospira genomes (GenBank accession numbers for each genome are presented in figures) by aligning reads against reference genomes Leptospira interrogans serogroup Canicola serovar Canicola strain LJ178 (GCA_008831445.1), Leptospira interrogans serogroup Icterohaemorrhagiae serovar Copenhageni strain Fiocruz_L1-130 (GCA_000007685.1), or Leptospira kirschneri serogroup Grippotyphosa serovar Grippotyphosa strain RedPanda1 (GCA_027563495.1) using minimap2 v2.22 (45) and calling SNPs from the BAM file with GATK v4.2.2 (46) using a depth of coverage ≥3× and a read proportion of 0.9. Based on a reference self-alignment with NUCmer v3.1 (47), SNPs that fell within duplicated regions were filtered from downstream analyses. All of these methods were wrapped by NASP v1.2.1 (48). Maximum likelihood phylogenies were then inferred on the concatenated SNP alignments using IQ-TREE v2.2.0.3 with default parameters (49), 1,000 bootstrap replicates, and the integrated ModelFinder method (50); the phylogenies were rooted with either Leptospira interrogans serovar Canicola strain LJ178, Leptospira interrogans serovar Copenhageni strain Fiocruz_L1-130, or Leptospira kirschneri serovar Grippotyphosa strain RedPanda1, as appropriate. To determine breadth of coverage for the enriched genomes, reads were aligned against Leptospira interrogans serovar Copenhageni strain Fiocruz_L1-130 for sample H2 and Leptospira kirschneri serovar Grippotyphosa strain RedPanda1 for sample H8 with minimap2, and the per base depth of coverage was calculated with Samtools v1.6 (51).
All animal experimentation was conducted in accordance with protocols as reviewed and approved by the Animal Care and Use Committee at the NADC and USDA institutional guidelines. Strains H9 and H44 were propagated in liquid HAN medium at 29°C and were evaluated for virulence by intraperitoneal injection of 108 leptospires in 500 μL into groups (n = 4 per group) of golden Syrian hamsters (Mesocricetus auratus). A negative control group (n = 4) received HAN medium alone. After 3 weeks of inoculation, animals were euthanized and exsanguinated by cardiac puncture, and whole blood smears were evaluated. Dried slides were submitted to the National Animal Disease Center Microscopy Services laboratory for Giemsa staining as reported previously (52), and Giemsa-stained slides were evaluated by counting the first 100 white blood cells to identify the portion of foamy macrophages. Differential cell counts were evaluated in R (53), where a linear regression model fitting challenge was used as a fixed effect to generate least square means and standard errors. Kidney and liver tissues were harvested for culture. qPCR for lipL32 was performed on liver and kidney samples, as previously described (54), except using DNA from L. interrogans serovar Canicola strain Hond Utrecht IV for a standard curve. Sera were collected for MAT, which was performed according to World Organisation for Animal Health (WOAH) guidelines (32), using a panel of 18 antigens representative of 15 serogroups (Supplementary Table S1), as well as strains H9 and H44. A titer was considered positive at ≥ 1:100.
In addition to strains H9 and H44, L. interrogans serogroup Icterohemorrhagiae serovar Copenhageni strain Fiocruz_L1-130 (55, 56) and L. interrogans serogroup Australis serovar Bratislava strain PigK151 (57) were propagated in HAN medium until mid-late log phase and harvested by centrifugation (10,000 × g, 4°C, 30 min), washed twice with PBS, and processed for one-dimensional (1-D) sodium dodecyl-sulfate polyacrylamide gel electrophoresis (SDS-PAGE) on 12% acrylamide gels (Bio-Rad, Hercules, CA, United States), according to the manufacturer’s guidelines. Proteins were visualized by staining with SYPRO Ruby (Invitrogen), and LPS was visualized by staining with Pro-Q Emerald 300 (Invitrogen), according to the manufacturer’s guidelines. For immunoblotting, samples were transferred by semi-dry transfer (Amersham TE77 PWR) to an Immobilon-P transfer membrane (Millipore, 220 Bedford, MA, United States) and blocked overnight at 4°C with Starting Block (PBS) blocking buffer (Thermo Fisher). Membranes were individually incubated with indicated antisera [anti-LipL32, anti-Loa22 or anti-LipL21 (58, 59)] or reference antisera [anti-Pomona, anti-Bratislava, anti-Copenhageni, or anti-Ramisi (NVSL, USDA, Ames, IA)] diluted in blocking buffer followed by incubation with horseradish-peroxidase anti-rabbit immunoglobulin G (Sigma, St. Louis, MO, United States) conjugate diluted 1:4,000 in blocking buffer. Bound conjugates were detected using Clarity Western ECL substrate (Bio-Rad), and images were acquired using a Bio-Rad ChemiDoc MP imaging system.
PCR of equine urine samples that were centrifuged at 900 × g for 10 min to remove “sludge” and processed on the same day or within 24 h of storage at 4°C provided the lowest limits of detection at 10 Leptospira/mL of urine (Supplementary Figure S1). Storage of samples at 4°C for 48 h, freezing, or not removing “sludge” by centrifugation at 900 × g for 10 min increased the limit of detection to 102 Leptospira/mL of urine. Freezing samples without removing “sludge” increased the limit of detection to 103 Leptospira/mL of urine.
Urine from 37 asymptomatic mares, designated as H1 to H37, was tested by rtPCR for lipL32 using optimized protocols as described above. Three urine samples (8%), designated as H2, H8, and H9 from three different pregnant mares, were PCR-positive with Ct values of 37, 38.2, and 36.9, respectively (Table 1). Repeat testing of urine collected 4 days later was PCR-positive for mares H8 and H9 but negative for mare H2. Urine from mare H9 was culture-positive for Leptospira. Complete data on all equine samples are shown in Supplementary Table S3.
Urine, chorioallantoic membrane, amnion, and allantoic fluid collected from a mare (designated as H44) showing clinical disease, and in the process of aborting at the time of sample collection, were positive by rtPCR for lipL32 with Ct values of 26, 31.8, 29.9, and 25.4, respectively (Supplementary Table S3). Chorioallantoic membrane was culture-positive for Leptospira. Samples collected from mare H44 after 2 days post-abortion, including urine, vulvar exudate, and uterine swabs, were all positive by rtPCR for lipL32, but all cultures were heavily contaminated (Supplementary Table S3).
An isolate of Leptospira cultured from urine of asymptomatic mare H9, designated as strain H9, was genotyped as L. kirschneri (Figure 1; Supplementary Figure S2). Serotyping of strain H9 with reference antisera identified the isolate as belonging to serogroup Australis (Supplementary Table S3). Additional serotyping with monoclonal antibodies to identify serovar confirmed that strain H9 was most similar to serovar Rushan due to similar reactivity patterns with the Rushan reference strain within the serogroup Australis (Supplementary Figure S3). Strain H9 is classified as L. kirschneri serogroup Australis serovar Rushan.
Figure 1. A maximum likelihood phylogeny of 36 L. kirschneri reference genomes together with Leptospira genomic DNA obtained from mares H8 and H9 (highlighted with blue text). The tree was inferred by IQ-TREE from a concatenated SNP alignment of 241,631 positions out of a core genome size of 2,978,905 nts. Leptospira from these two animals cluster together in a distinct L. kirschneri clade. GenBank accession numbers for reference genomes are included in the annotations along with strain name, host, geographic location, and year of collection when available. The phylogeny is rooted with L. interrogans strain Fiocruz_L1-130, and bootstrap values based on 1,000 replicates are indicated at major nodes.
An isolate of Leptospira cultured from chorioallantois of mare H44 presenting with abortion was genotyped as L. interrogans (Figure 2; Supplementary Figure S2). Serotyping of strain H44 with reference antisera identified the isolate as belonging to serogroup Pomona (Supplementary Table S3). Additional serotyping with monoclonal antibodies to identify serovar confirmed that strain H44 was most similar to serovar Pomona due to similar reactivity patterns with the Pomona reference strain within the serogroup Pomona (Supplementary Figure S4). Strain H44 is classified as L. interrogans serogroup Pomona serovar Pomona.
Figure 2. A maximum likelihood phylogeny of 16 L. interrogans reference genomes that fall within the “serovar Pomona” clade together with Leptospira strain H44 (highlighted with blue text). The tree was inferred by IQ-TREE from a concatenated SNP alignment of 10,732 positions out of a core genome size of 4,165,981 nts. Leptospira from this animal cluster among four other serovar Pomona genomes obtained from two sea lions, a fox, and a human from the US GenBank accession numbers for reference genomes are included in the annotations along with strain name, host, geographic location, and year of collection when available. The phylogeny is rooted with L. interrogans serovar Canicola strain LJ178, and bootstrap values based on 1,000 replicates are indicated at major nodes.
Given the fastidious growth requirements of pathogenic Leptospira and the inherent difficulties with their culture from urine of large animal species, urine samples that were PCR-positive for lipL32 but culture-negative for Leptospira were processed using a culture-independent DNA capture and enrichment system to obtain Leptospira genomic information directly from urine samples H2 and H8. Enriched samples H2 and H8 had very high and moderate proportions, respectively, of sequencing reads that assigned to Leptospira: 98.36% for H2 (1,529,676 of 1,555,190 reads) and 60.08% for H8 (562,885 of 936,958 total reads). Sample H2 revealed a breadth of coverage of 99.95% with an average depth of 77.5× (72–78×) against reference genome L. interrogans serogroup Icterohemorrhagiae serovar Copenhageni strain Fiocruz_L1-130. Sample H8 displayed a breadth and depth of coverage of 92.72% and 32.5× (3–176×) when aligned against Leptospira kirschneri serogroup Grippotyphosa serovar Grippotyphosa strain RedPanda1. The whole genome Leptospira dendrogram placed enriched and isolate genomes for strains H8 and H9 in the L. kirschneri clade, whereas enriched and isolate genomes for strains H2 and H44 grouped with L. interrogans (Supplementary Figure S2). To obtain higher resolution within L. interrogans, a core genome phylogeny was constructed containing enriched genome H2 and isolate genome H44 plus 51 diverse L. interrogans genomes (Supplementary Figure S5). This analysis placed H44 within the “serovar Pomona” clade and H2 within the “serovar Copenhageni” clade.
Three separate phylogenies were constructed based on the core genomes of: (1) enriched genome H8, isolate genome H9, and 36 L. kirschneri reference genomes (Figure 1); (2) isolate genome H44 and 16 L. interrogans reference genomes that assign to the “serovar Pomona” clade (Figure 2); and (3) enriched genome H2 and 9 L. interrogans reference genomes that fall within the “serovar Copenhageni” clade (Figure 3). Genomes for strains H8 and H9 clustered together in a clade within, yet distinct from, other L. kirschneri genomes (Figure 1). Genome H44 grouped with other genomes of L. interrogans serovar Pomona from US isolates of Leptospira derived from a fox, sea lions, and a human (Figure 2). Finally, enriched genome H2 was most like other L. interrogans serovar Copenhageni genomes (Supplementary Figure S5), yet still distinct by displaying 376 SNP differences to separate it from the “serovar Copenhageni” clade (Figure 3).
Figure 3. A maximum likelihood phylogeny of nine L. interrogans reference genomes that fall within the “serovar Copenhageni” clade together with enriched Leptospira genomic DNA from mare H2 (highlighted with blue text). The tree was inferred by IQ-TREE from a concatenated SNP alignment of 37,071 positions out of a core genome size of 4,247,835 nts. Leptospira from this animal is mostly similar to other genomes from the “serovar Copenhageni” clade, yet it is distinct. GenBank accession numbers for reference genomes are included in the annotations along with strain name, host, geographic location, and year of collection when available. The phylogeny is rooted with L. interrogans serovar Canicola strain LJ178, and bootstrap values based on 1,000 replicates are indicated at major nodes.
Sera from 37 asymptomatic mares were tested by MAT. Of these, 35 (94.6%) were seropositive (titer ≥1:100). Equivalent highest titers were observed for more than one serogroup in 10 samples (Supplementary Table S3). The most frequent highest-reacting MAT titer was with serogroup Australis (31.4%), followed by serogroups Pomona (20%), Djasiman (5.7%), Grippotyphosa (5.7%), and Icterohaemorrhagiae (2.8%). All equine sera were seronegative when tested by the MAT using strain H9.
Serum from the clinically infected mare (H44) presenting with abortion had a high MAT titer to serogroup Pomona (1:102,400).
Experimentally inoculated hamsters did not show any clinical signs of infection or weight loss after intraperitoneal inoculation with L. kirschneri serogroup Australis strain H9. Similarly, hamsters inoculated with L. interrogans serogroup Pomona strain H44 did not show clinical signs of disease, except for one hamster that was losing weight and euthanized at day 14 post-inoculation. After 3 weeks of infection, all remaining hamsters were euthanized. All kidney samples from each group tested positive by lipL32 qPCR (Table 2 and Figure 4). Livers from hamsters inoculated with strain H44 were also PCR-positive but livers from hamsters inoculated with strain H9 were PCR-negative. Kidneys and livers from hamsters inoculated with strain H44 were all culture-positive, but only two of four hamsters inoculated with strain H9 were kidney-culture positive (Table 2). Hamsters inoculated with L. kirschneri serogroup Australis strain H9 had positive MAT titers against strain H9 and reference strain L. interrogans serogroup Australis serovar Bratislava strain Jez Bratislava but were seronegative when tested against other reference antigens (Table 2). Hamsters inoculated with L. interrogans serogroup Pomona strain H44 had positive MAT titers against strain H44 and reference strain L. interrogans serogroup Pomona serovar Pomona strain Pomona but were seronegative when tested against other reference antigens (Table 2). Manual blood count differentials determined that all hamsters challenged with both H9 and H44 produced circulating foamy macrophages (1.50 ± 0.66 and 1.55 ± 0.66, respectively, foamy macrophages per 100 white blood cells evaluated), a marker associated with virulence and disease severity in the hamster model of leptospirosis (52, 60).
Table 2. Hamster challenge with L. kirschneri serogroup Australis strain H9 and L. interrogans serogroup Pomona strain H44.
Figure 4. Detection of Leptospira in the kidney and liver of hamsters after inoculation with L. kirschneri serogroup Australis strain H9 (associated with asymptomatic carriage in a mare) and L. interrogans serogroup Pomona strain H44 (associated with spontaneous equine abortion) by qPCR detection of lipL32. Numbers of genome copies (GC) of leptospires per gram (G) of tissue are shown in Table 2.
Total protein profiles of L. kirschneri serogroup Australis strain H9 and L. interrogans serogroup Pomona strain H44 were compared with those of L. interrogans serogroup Icterohemorrhagiae serovar Copenhageni strain Fiocruz_L1-130 and L. interrogans serogroup Australis serovar Bratislava strain PigK151 (Figure 5A). As expected for pathogenic Leptospira, similar protein profiles were detected across different species and serovars, and all strains were confirmed to express the pathogen-associated protein LipL32 and known virulence factors Loa22 and LipL21 (Figures 5B,C).
Figure 5. Total protein profiles (A) of 1: L. kirschneri serogroup Australis strain H9, 2: L. interrogans serogroup Pomona strain H44, 3: L. interrogans serogroup Icterohaemorrhagiae serovar Copenhageni strain Fiocruz_L1-130, and 4: L. interrogans serogroup Australis serovar Bratislava strain PigK151 and immunoblotting with (B) anti-LipL32/Loa22 or (C) anti-LipL32/LipL21. Molecular mass markers are indicated.
Total lipopolysaccharide (LPS) profiles of each strain were also compared (Figure 6A). The results confirm the unusual and atypical LPS profile of pathogenic Leptospira compared with that of E. coli and confirm different LPS profiles between serovars of Leptospira from different serogroups. Antigenic differences of LPS between serogroups and different serovars within the same serogroup were confirmed by immunoblotting with reference antisera specific for (1) serogroup Australis serovar Ramisi, (2) serogroup Pomona serovar Pomona, (3) serogroup Icterohemorrhagiae serovar Copenhageni, and (4) serogroup Australis serovar Bratislava (Figures 6B–E).
Figure 6. Lipopolysaccharide profiles of leptospires: total LPS profiles (A) of 1: L. kirschneri serogroup Australis strain H9, 2: L. interrogans serogroup Pomona strain H44, 3: L. interrogans serogroup Icterohaemorrhagiae serovar Copenhageni strain Fiocruz_L1-130, 4: L. interrogans serogroup Australis serovar Bratislava strain PigK151, and positive LPS control (+) comprising 10 μg of LPS from E. coli serotype 055:B5. Immunoblotting with reference antisera against (B) serogroup Australis serovar Ramisi, (C) serogroup Pomona serovar Pomona, (D) serogroup Icterohaemorrhagiae serovar Copenhageni, and (E) serogroup Australis serovar Bratislava. Molecular mass markers are indicated.
Sequencing data and accession numbers are available at NCBI under BioProject ID PRJNA994138.
The primary goal of this study was to determine whether asymptomatic mares can act as reservoir hosts of infection, and if so, what species and serovars of Leptospira are involved. Our approach was limited to the goodwill and cooperation of management and staff of a single commercial thoroughbred breeding farm that facilitated collection of samples from a limited number of available mares, with an incomplete vaccination history, on a single farm, within a relatively short timeframe. Our results demonstrate that asymptomatic mares can shed different species and serovars of Leptospira via urine. Unexpectedly, a single isolate recovered from an asymptomatic mare is classified as belonging to L. kirschneri serogroup Australis serovar Rushan, which has not previously been reported in the US or in horses. These findings justify more comprehensive studies on horses throughout the US to accurately determine the prevalence of equine leptospirosis, and species and serovars of Leptospira are associated with asymptomatic shedding.
The literature is replete with serological evidence that horses are exposed to serovars within the serogroup Australis and coupled with the isolation of L. interrogans serogroup Australis serovar Bratislava from horses in Ireland and Portugal (18, 19); it is hypothesized that horses act as reservoir hosts for serovar Bratislava. However, serology by MAT alone does not detect or reflect shedding of Leptospira in reservoir hosts of leptospirosis (1). Therefore, urine samples from asymptomatic mares were initially screened by rtPCR targeting lipL32, a gene present in pathogenic Leptospira species that discriminates from saprophytic species. Optimal detection of lipL32 in equine urine requires that they are processed for PCR within 24 h and shipped on icepacks (Supplementary Figure S1). Three PCR-positive mares (designated as H2, H8, and H9) were identified and targeted for culture and repeat rtPCR, of which two (H8 and H9) remained PCR-positive and one (H9) was culture-positive, validating initial results. Intermittent shedding of leptospires in urine of reservoir hosts is not uncommon, which may account for the negative rtPCR in mare H2 (38, 61). Because the farm’s veterinary clinician initiated antimicrobial therapy after PCR-positive results became available, additional rtPCR and culture were not performed. Culture of Leptospira from urine of large domestic animals is notoriously difficult (1), so a culture-independent approach for characterizing the genome of Leptospira in PCR-positive samples was used to genomically characterize those species associated with subclinical infection in mares H2 and H8 (43). Mare H2 was determined to be shedding L. interrogans, the genome of which aligned most closely with serogroup Icterohemorrhagiae serovar Copenhageni but still quite distinct, with 376 SNPs separating it from other genomes in this clade (Figure 3). Serogroup Icterohemorrhagiae is a leading cause of acute leptospirosis in human patients, and its classic reservoir host is the rat, not domestic animals. However, this serogroup has been isolated from equine kidney and aborted fetuses previously in Europe (15, 19) and more recently in urine from a US dairy cow at slaughter (Hamond and Nally, unpublished data). Mare H8 was determined to be shedding L. kirschneri, the genome of which aligned most closely with that of the cultured isolate L. kirschneri serogroup Australis serovar Rushan strain H9 (Figure 1). Of note, the genomes from H8 and H9 differed by only 16 SNPs when comparing 4,565,109 shared nucleotide positions.
Serogroup Australis contains at least 14 serovars including serovars Australis and Bratislava that are commonly used as representative serovars in MAT antigen panels and both of which belong to L. interrogans (Supplementary Table S1). Panels of reference monoclonal antibodies typed strain H9 as serovar Rushan. The reference strain for serovar Rushan was originally isolated from a toad (Bombina orientalis) in China and classified as L. noguchii (62, 63). Average nucleotide identity (ANI) later classified it as L. alstonii (64). Only one serovar within the serogroup Australis is assigned to L. kirschneri, the reference strain for serovar Ramisi, which was originally isolated in a human patient in Kenya, circa 1971 (65, 66). The efficacy of bacterin vaccines in animals is dependent on the inclusion of relevant serovars associated with animal infection. Serovar status is defined using reference-agglutinating antisera or monoclonal antibodies, which target LPS, a serovar specific and protective antigen. LPS from Leptospira has an atypical structure from that of other gram-negative bacteria and is much less toxic likely due to modified Lipid A (67). The LPS profile of L. kirschneri serogroup Australis strain H9 (a strain herein associated with subclinical carriage) was compared with that of L. interrogans serogroup Pomona strain H44 (a strain associated with spontaneous equine abortion), L. interrogans serogroup Icterohemorrhagiae serovar Copenhageni strain Fiocruz_L1-130 (a strain closely related to enriched genome H2), and L. interrogans serogroup Australis serovar Bratislava strain PigK151 [a strain recently used in an equine challenge study (68)] to highlight differences in LPS profiles (Figure 6A). More importantly, the use of polyclonal reference antisera in immunoblots confirms antigenic differences in expression of the LPS protective antigen (Figures 6B–E) between serovars, thus demonstrating their individual status and need for inclusion in bacterins as protective antigens or in MAT panels as diagnostic antigens. The reactivity of LPS derived from L. kirschneri serogroup Australis strain H9 with reference antiserum specific for L. kirschneri serogroup Australis serovar Ramisi confirms sufficient antigenic differences with L. interrogans serogroup Australis serovar Bratislava, demonstrating limited cross reactivity in bacterin vaccine or MAT diagnostic antigens (Figure 6E), as confirmed by MAT titers in experimentally infected hamsters (Table 2). In contrast to LPS, proteins are highly conserved among pathogenic species and serovars (Figure 5A). The expression of known virulence factors Loa22 and LipL21 was confirmed in all strains (Figures 5B,C) (59, 69–71).
Horses are hypothesized to act as a reservoir host for serovar Bratislava in the US, according to multiple seroprevalence studies (9). However, a serovar is used in an MAT panel as a representative serovar for the serogroup so that a positive reaction is indicative of exposure to a serogroup, not specifically the serovar used in the panel (2). No direct evidence has yet been provided that serovar Bratislava persistently infects US horses (72). Recent studies have attempted to force this hypothesis by experimental infection of horses with an isolate of serovar Bratislava which was recovered from a pig a few decades ago (57), but no evidence of established infection was reported (68). In the US, serogroup Australis serovar Bratislava has only ever been isolated from the kidneys or genital tract of pigs (73, 74) and is universally reported to be a significant cause of porcine reproductive loss (1). Cows act as reservoir hosts to serogroup Sejroe serovar Hardjo, which can also colonize kidneys and/or the genital tract. Evidence of bovine exposure to serogroup Sejroe is associated with an increase in median time from calving to conception, as well as being twice as likely to fail to conceive (75). In Brazil, Leptospira has been detected by PCR in endometrial biopsies or vaginal fluid from mares with reproductive issues including early embryonic death and endometritis (76); thus, it would be beneficial to screen US horses for the colonization of the genital tract by Leptospira, whether by L. kirschneri serogroup Australis or other strains.
Animal leptospirosis presents with an array of clinical signs that is dependent, in part, on both the serovar of Leptospira and specific animal host species (77). Pathogenic mechanisms of infection are neither clearly understood nor the reason why an animal host species acts as a reservoir host for one serovar but an incidental host for others. In this study, over 90% of horses were seropositive. However, no reactivity was detected when L. kirschneri serogroup Australis serovar Rushan strain H9 was included in the MAT antigen panel, even though two horses (H8 and H9) are reported here as active shedders. Similarly, horse H2 had no reactive titer against serogroup Icterohemorrhagiae though it was detected in urine. The identification of seronegative reservoir hosts is not uncommon when sera are tested at 1:100, and therefore, studies with subclinical animals may test sera at starting dilutions less than 1:100 (28, 78, 79). Incidental infection is characterized by acute dissemination of Leptospira with limited shedding compared with reservoir hosts, which have subclinical carriage and extended shedding (80). L. kirschneri serogroup Australis serovar Rushan strain H9 was not detected in the liver after experimental infection in hamsters but was detected at low levels in the kidney, whereas L. interrogans serogroup Pomona serovar Pomona strain H44 was detected at higher levels in all the livers and kidneys (Table 2). Serovar Pomona is associated with acute disease in many animal hosts, and strain H44 is closely related to other Pomona strains isolated from multiple animals geographically distributed throughout the US (81). A total of 195 SNPs differentiate the genome of strain H44 from California sea lion isolate strain CSL4002 when comparing 4,524,948 shared nucleotide positions (Figure 2). Unique genotypes of Pomona are reported to be associated with equine abortion (11), and the advent of high-resolution genome sequencing provides opportunities to explore these associations further. Similarly, comparative genomic analyses of strains associated with acute disease compared with strains associated with subclinical carriage may provide insights into the pathogenic mechanism of infection and host adaptation.
Previous studies on equine leptospirosis have relied on EMJH media to isolate Leptospira from aborted and stillborn horses (8, 82). However, pathogenic Leptospira comprise a diverse genus of highly fastidious bacteria that require alternative media formulations since many serovars will not grow in commercial EMJH media (2, 30). Reliance on EMJH to culture leptospires from mammalian hosts is selective for only those pathogenic leptospires capable of surviving in, or adapting to, the growth media and conditions used, so alternative media formulations such as HAN are recommended. Serogroup Australis serovar Bratislava was isolated from US sows using T80/40/LH medium, not EMJH (74, 83). Historically, the isolation of pathogenic Leptospira from mammalian hosts has been limited to cultures incubated at 28–30°C (31). However, the use of newer media formulations such as HAN allows the isolation from mammalian hosts at 28–30°C and 37°C, a temperature that more closely emulates that encountered during host infection (30). Temperature is an important environmental signal used by Leptospira to regulate gene and protein expression and antigens expressed by Leptospira that react with equine sera (84–86). Pathogenic Leptospira regulate gene and protein expression during acute and chronic infection (59, 69, 87, 88), so it is hypothesized that bacterins produced at temperatures more similar to that of a host will express higher levels of cross-protective protein antigens and virulence factors (89).
Leptospirosis is a global neglected disease and paradigm of one health (90). New species and serovars continue to be identified (91, 92) highlighting its complex epidemiology and need to determine species and serovars circulating within domestic animal populations. The use of novel media formulations for Leptospira has facilitated the characterization of a diverse range of a new species and serovars circulating in the environment (4) and US livestock (37, 38). Similarly, we report here the identification of a new species and serovar associated with asymptomatic carriage and shedding in urine of horses, L. kirschneri serogroup Australis serovar Rushan. In the absence of culture, real-time PCR of urine identifies asymptomatic carriers, while DNA capture and enrichment provide comprehensive Leptospira genomic information. For real-time PCR, equine urine should be chilled as soon as collected and processed within 24 h for optimal sensitivity. The identification and characterization of strains associated with equine leptospirosis is essential to design efficacious diagnostic, vaccination, prevention, and treatment strategies.
The datasets presented in this study can be found in online repositories. The names of the repository/repositories and accession number(s) can be found at: https://www.ncbi.nlm.nih.gov/genbank/, BioProject ID PRJNA994138.
The animal studies were approved by the Animal Care & Use Committee at the NADC and the USDA institutional guidelines. The studies were conducted in accordance with the local legislation and institutional requirements. Written informed consent was not obtained from the owners for the participation of their animals in this study because verbal consent was provided from the animals’ agent.
CH: Data curation, Formal analysis, Investigation, Methodology, Supervision, Validation, Visualization, Writing – original draft, Writing – review & editing. EA: Conceptualization, Data curation, Funding acquisition, Investigation, Methodology, Project administration, Resources, Writing – review & editing. NS: Data curation, Formal analysis, Investigation, Methodology, Software, Visualization, Writing – review & editing. KL: Data curation, Investigation, Methodology, Writing – review & editing. TA: Formal analysis, Investigation, Methodology, Writing – review & editing. EP: Data curation, Formal analysis, Investigation, Methodology, Writing – review & editing. PC: Data curation, Formal analysis, Investigation, Methodology, Writing – review & editing. JH: Data curation, Formal analysis, Investigation, Methodology, Writing – review & editing. TS: Data curation, Formal analysis, Investigation, Methodology, Writing – review & editing. HL: Data curation, Formal analysis, Investigation, Methodology, Writing – review & editing. DB: Data curation, Formal analysis, Investigation, Methodology, Writing – review & editing. JS: Data curation, Formal analysis, Investigation, Methodology, Supervision, Writing – review & editing. LS: Funding acquisition, Resources, Writing – review & editing. DW: Data curation, Formal analysis, Investigation, Methodology, Resources, Supervision, Writing – review & editing. JN: Conceptualization, Data curation, Formal analysis, Funding acquisition, Investigation, Methodology, Project administration, Resources, Supervision, Validation, Visualization, Writing – original draft, Writing – review & editing.
The author(s) declare that financial support was received for the research, authorship, and/or publication of this article. This research was supported by USDA and, in part, by an appointment to the Animal and Plant Health Inspection Service (APHIS) Research Participation Program administered by the Oak Ridge Institute for Science and Education (ORISE) through an interagency agreement between the US Department of Energy (DOE) and the US Department of Agriculture (USDA). ORISE is managed by ORAU under DOE contract number DE-SC0014664. USDA is an equal opportunity provider and employer. Mention of trade names or commercial products in this publication is solely for the purpose of providing specific information and does not imply recommendation or endorsement by the US Department of Agriculture.
The authors thank the farm clinician, management, and staff for their commitment, expert help, and time required to providing diagnostic samples for these studies.
The authors declare that the research was conducted in the absence of any commercial or financial relationships that could be construed as a potential conflict of interest.
The author(s) declared that they were an editorial board member of Frontiers, at the time of submission. This had no impact on the peer review process and the final decision.
All claims expressed in this article are solely those of the authors and do not necessarily represent those of their affiliated organizations, or those of the publisher, the editors and the reviewers. Any product that may be evaluated in this article, or claim that may be made by its manufacturer, is not guaranteed or endorsed by the publisher.
The Supplementary material for this article can be found online at: https://www.frontiersin.org/articles/10.3389/fvets.2024.1346713/full#supplementary-material
SUPPLEMENTARY FIGURE S1 | Lower limits of detection of Leptospira by lipL32 qPCR in equine urine that was processed fresh the day of collection (A,B), after storage for 24 h on ice packs (C,D), after storage for 48 h on ice packs (E,F), or after being frozen at −20oC (G,H). Samples B,D,F,H were centrifuged at 900 × g for 10 min to remove “sludge” prior to processing.
SUPPLEMENTARY FIGURE S2 | Whole-genome dendrogram based upon pairwise MASH distances of Leptospira genomes, showing mare sequences H2, H8, H9, and H44 within the Leptospira P1 clade (highlighted in blue) (3). Enriched and isolate genomes for strains H8 and H9 were placed in the L. kirschneri clade, whereas strains H2 and H44 grouped with L. interrogans. The dendrogram includes genomes of 66 Leptospira strains representing all known species from the P1, P2, S1 and S2 clades.
SUPPLEMENTARY FIGURE S3 | Serotyping of strain H9 with monoclonal antibodies (mAb) that characteristically agglutinate serovars from serogroup Australis. Titers of reactivity for each mAb are provided for L. kirschneri serogroup Australis strain H9 and reference strain L. noguchii serogroup Australis serovar Rushan strain 507. Reciprocal titers are shown on the y-axis; mAb number is shown on the x-axis.
SUPPLEMENTARY FIGURE S4 | Serotyping of strain H44 with monoclonal antibodies (mAb) that characteristically agglutinate serovars from serogroup Pomona. Titers of reactivity for each mAb are provided for L. interrogans serogroup Pomona strain H44 and reference strain L. interrogans serogroup Pomona serovar Pomona strain Pomona. Reciprocal titers are shown on the y-axis; mAb number is shown on the x-axis.
SUPPLEMENTARY FIGURE S5 | A maximum likelihood phylogeny of 51 L. interrogans reference genomes together with Leptospira genomic DNA from strain H2 and H44 (highlighted with blue text). The tree was inferred by IQ-TREE from a concatenated SNP alignment of 248,698 positions out of a core genome size of 2,688,796nts. Leptospira from strain H2 falls among other L. interrogans serovar Copenhageni genomes, whereas strain H44 groups among other L. interrogans serovar Pomona genomes. GenBank accession numbers for reference genomes are included in the annotations. The phylogeny is rooted with L. kirschneri strain RedPanda1.
1. Ellis, WA. Animal leptospirosis. Curr Top Microbiol Immunol. (2015) 387:99–137. doi: 10.1007/978-3-662-45059-8_6
2. Faine, S, Adler, B, Bolin, C, and Perolat, P. Leptospira and Leptospirosis. 2nd ed. Melbourne, Australia: MediSci (1999).
3. Vincent, AT, Schiettekatte, O, Goarant, C, Neela, VK, Bernet, E, Thibeaux, R, et al. Revisiting the taxonomy and evolution of pathogenicity of the genus leptospira through the prism of genomics. PLoS Negl Trop Dis. (2019) 13:e0007270. doi: 10.1371/journal.pntd.0007270
4. Fernandes, LGV, Stone, NE, Roe, CC, Goris, MGA, van der Linden, H, Sahl, JW, et al. Leptospira sanjuanensis sp. nov., a pathogenic species of the genus leptospira isolated from soil in Puerto Rico. Int J Syst Evol Microbiol. (2022) 72:5560. doi: 10.1099/ijsem.0.005560
5. Casanovas-Massana, A, Vincent, AT, Bourhy, P, Neela, VK, Veyrier, FJ, Picardeau, M, et al. Leptospira dzianensis and Leptospira putramalaysiae are later heterotypic synonyms of Leptospira yasudae and Leptospira stimsonii. Int J Syst Evol Microbiol. (2021) 71:4713. doi: 10.1099/ijsem.0.004713
6. Korba, AA, Lounici, H, Kainiu, M, Vincent, AT, Mariet, JF, Veyrier, FJ, et al. Leptospira abararensis sp. nov. and Leptospira chreensis sp. nov., four new species isolated from water sources in Algeria. Int J Syst Evol Microbiol. (2021) 71:5148. doi: 10.1099/ijsem.0.005148
7. Costa, F, Hagan, JE, Calcagno, J, Kane, M, Torgerson, P, Martinez-Silveira, MS, et al. Global morbidity and mortality of leptospirosis: a systematic review. PLoS Negl Trop Dis. (2015) 9:e0003898. doi: 10.1371/journal.pntd.0003898
8. Donahue, JM, Smith, BJ, Redmon, KJ, and Donahue, JK. Diagnosis and prevalence of Leptospira infection in aborted and stillborn horses. J Vet Diagn Invest. (1991) 3:148–51. doi: 10.1177/104063879100300208
9. Divers, TJ, Chang, YF, Irby, NL, Smith, JL, and Carter, CN. Leptospirosis: an important infectious disease in north American horses. Equine Vet J. (2019) 51:287–92. doi: 10.1111/evj.13069
10. Polle, F, Storey, E, Eades, S, Alt, D, Hornsby, R, Zuerner, R, et al. Role of intraocular Leptospira infections in the pathogenesis of equine recurrent uveitis in the southern United States. J Equine Vet. (2014) 34:1300–6. doi: 10.1016/j.jevs.2014.09.010
11. Timoney, JF, Kalimuthusamy, N, Velineni, S, Donahue, JM, Artiushin, SC, and Fettinger, M. A unique genotype of Leptospira interrogans serovar Pomona type kennewicki is associated with equine abortion. Vet Microbiol. (2011) 150:349–53. doi: 10.1016/j.vetmic.2011.02.049
12. Donahue, JM, Smith, BJ, Poonacha, KB, Donahoe, JK, and Rigsby, CL. Prevalence and serovars of leptospira involved in equine abortions in Central Kentucky during the 1991–1993 foaling seasons. J Vet Diagn Invest. (1995) 7:87–91. doi: 10.1177/104063879500700114
13. Poonacha, KB, Donahue, JM, Giles, RC, Hong, CB, Petrites-Murphy, MB, Smith, BJ, et al. Leptospirosis in equine fetuses, stillborn foals, and placentas. Vet Pathol. (1993) 30:362–9. doi: 10.1177/030098589303000405
14. Donahue, JM, Smith, BJ, Donahoe, JK, Rigsby, CL, Tramontin, RR, Poonacha, KB, et al. Prevalence and serovars of leptospira involved in equine abortions in Central Kentucky during the 1990 foaling season. J Vet Diagn Invest. (1992) 4:279–84. doi: 10.1177/104063879200400309
15. Ellis, W, Bryson, D, O’brien, J, and Neill, S. Leptospiral infection in aborted equine foetuses. Equine Vet J. (1983) 15:321–4. doi: 10.1111/j.2042-3306.1983.tb01811.x
16. Brem, S, Gerhards, H, Wollanke, B, Meyer, P, and Kopp, H. Demonstration of intraocular leptospira in 4 horses suffering from equine recurrent uveitis (ERU). Berliner und Munchener Tierarztliche Wochenschrift. (1998) 111:415–7.
17. Hartskeerl, R, Goris, M, Brem, S, Meyer, P, Kopp, H, Gerhards, H, et al. Classification of leptospira from the eyes of horses suffering from recurrent uveitis. J Veterinary Med Ser B. (2004) 51:110–5. doi: 10.1111/j.1439-0450.2004.00740.x
18. Rocha, T, Ellis, W, Montgomery, J, Gilmore, C, Regalla, J, and Brem, S. Microbiological and serological study of leptospirosis in horses at slaughter: first isolations. Res Vet Sci. (2004) 76:199–202. doi: 10.1016/j.rvsc.2003.12.003
19. Ellis, WA, O’Brien, JJ, Cassells, JA, and Montgomery, J. Leptospiral infection in horses in Northern Ireland: serological and microbiological findings. Equine Vet J. (1983) 15:317–20. doi: 10.1111/j.2042-3306.1983.tb01809.x
20. Lees, VW, and Gale, SP. Titers to Leptospira species in horses in Alberta. Can Vet J. (1994) 35:636–40.
21. Trimble, AC, Blevins, CA, Beard, LA, Deforno, AR, and Davis, EG. Seroprevalence, frequency of leptospiuria, and associated risk factors in horses in Kansas, Missouri, and Nebraska from 2016-2017. PLoS One. (2018) 13:e0206639. doi: 10.1371/journal.pone.0206639
22. Benvin, I, Perko, VM, Maljković, MM, Habuš, J, Štritof, Z, Hađina, S, et al. Serological surveillance of equine leptospirosis in Croatia in the period from 2012 to 2022: a key insight into the changing Epizootiology. J Equine Vet. (2023) 127:104844. doi: 10.1016/j.jevs.2023.104844
23. Odontsetseg, N, Boldbaatar, D, Mweene, AS, and Kida, H. Serological prevalence of Leptospira interrogans serovar Bratislava in horses in Mongolia. Vet Rec. (2005) 157:518–9. doi: 10.1136/vr.157.17.518
24. Simbizi, V, Saulez, MN, Potts, A, Lötter, C, and Gummow, B. A study of leptospirosis in south African horses and associated risk factors. Prev Vet Med. (2016) 134:6–15. doi: 10.1016/j.prevetmed.2016.09.019
25. Båverud, V, Gunnarsson, A, Engvall, EO, Franzén, P, and Egenvall, A. Leptospira seroprevalence and associations between seropositivity, clinical disease and host factors in horses. Acta Vet Scand. (2009) 51:1–10. doi: 10.1186/1751-0147-51-15
26. Williams, D, Smith, B, Donahue, J, and Poonacha, K. Serological and microbiological findings on 3 farms with equine leptospiral abortions. Equine Vet J. (1994) 26:105–8. doi: 10.1111/j.2042-3306.1994.tb04345.x
27. Fagre, AC, Mayo, CE, Pabilonia, KL, and Landolt, GA. Seroprevalence of Leptospira spp. in Colorado equids and association with clinical disease. J Vet Diagn Invest. (2020) 32:718–21. doi: 10.1177/1040638720943155
28. Nally, JE, Hornsby, RL, Alt, DP, Bayles, D, Wilson-Welder, JH, Palmquist, DE, et al. Isolation and characterization of pathogenic leptospires associated with cattle. Vet Microbiol. (2018) 218:25–30. doi: 10.1016/j.vetmic.2018.03.023
29. Nally, JE, Ahmed, AA, Putz, EJ, Palmquist, DE, and Goris, MG. Comparison of real-time PCR, bacteriologic culture and fluorescent antibody test for the detection of Leptospira borgpetersenii in urine of naturally infected cattle. Vet Sci. (2020) 7:66. doi: 10.3390/vetsci7020066
30. Hornsby, RL, Alt, DP, and Nally, JE. Isolation and propagation of leptospires at 37 degrees C directly from the mammalian host. Sci Rep. (2020) 10:9620. doi: 10.1038/s41598-020-66526-4
32. Cole, JR, Sulzer, CR, and Pursell, AR. Improved microtechnique for the leptospiral microscopic agglutination test. Appl Microbiol. (1973) 25:976–80. doi: 10.1128/am.25.6.976-980.1973
33. Goris, MG, and Hartskeerl, RA. Leptospirosis serodiagnosis by the microscopic agglutination test. Curr Protoc Microbiol. (2014) 32:5.1-E. doi: 10.1002/9780471729259.mc12e05s32
34. Galloway, RL, and Hoffmaster, AR. Optimization of LipL32 PCR assay for increased sensitivity in diagnosing leptospirosis. Diagn Microbiol Infect Dis. (2015) 82:199–200. doi: 10.1016/j.diagmicrobio.2015.03.024
35. Stoddard, RA, Gee, JE, Wilkins, PP, McCaustland, K, and Hoffmaster, AR. Detection of pathogenic Leptospira spp. through TaqMan polymerase chain reaction targeting the LipL32 gene. Diagn Microbiol Infect Dis. (2009) 64:247–55. doi: 10.1016/j.diagmicrobio.2009.03.014
36. Miller, JN. Spirochetes in body fluids and tissues. Springfield, Illinois, U.S.A.: Charles C. THomas (1971).
37. Hamond, C, Dirsmith, KL, LeCount, K, Soltero, FV, Rivera-Garcia, S, Camp, P, et al. Leptospira borgpetersenii serovar Hardjo and Leptospira santarosai serogroup Pyrogenes isolated from bovine dairy herds in Puerto Rico. Frontiers in veterinary. Science. (2022) 9:1795. doi: 10.3389/fvets.2022.1025282
38. Hamond, C, LeCount, K, Putz, EJ, Bayles, DO, Camp, P, Goris, MGA, et al. Bovine leptospirosis due to persistent renal carriage of Leptospira borgpetersenii Serovar Tarassovi. Front Vet Sci. (2022) 9:848664. doi: 10.3389/fvets.2022.848664
39. Wood, DE, Lu, J, and Langmead, B. Improved metagenomic analysis with kraken 2. Genome Biol. (2019) 20:1–13. doi: 10.1186/s13059-019-1891-0
40. Bankevich, A, Nurk, S, Antipov, D, Gurevich, AA, Dvorkin, M, Kulikov, AS, et al. SPAdes: a new genome assembly algorithm and its applications to single-cell sequencing. J Comput Biol. (2012) 19:455–77. doi: 10.1089/cmb.2012.0021
41. Altschul, SF, Gish, W, Miller, W, Myers, EW, and Lipman, DJ. Basic local alignment search tool. J Mol Biol. (1990) 215:403–10. doi: 10.1016/S0022-2836(05)80360-2
42. Hartskeerl, R, Smits, H, Korver, H, Goris, M, and Terpstra, W. Manual international course on laboratory methods for the diagnosis of leptospirosis. Amsterdam, The Netherlands: KIT (2006).
43. Stone, NE, McDonough, RF, Hamond, C, LeCount, K, Busch, JD, Dirsmith, KL, et al. DNA capture and enrichment: a culture-independent approach for characterizing the genomic diversity of pathogenic Leptospira species. Microorganisms. (2023) 11:51282. doi: 10.3390/microorganisms11051282
44. Katz, LS, Griswold, T, Morrison, SS, Caravas, JA, Zhang, S, den Bakker, HC, et al. Mashtree: a rapid comparison of whole genome sequence files. J Open Source Softw. (2019) 4:1762. doi: 10.21105/joss.01762
45. Li, H. Minimap2: pairwise alignment for nucleotide sequences. Bioinformatics. (2018) 34:3094–100. doi: 10.1093/bioinformatics/bty191
46. McKenna, A, Hanna, M, Banks, E, Sivachenko, A, Cibulskis, K, Kernytsky, A, et al. The genome analysis toolkit: a MapReduce framework for analyzing next-generation DNA sequencing data. Genome Res. (2010) 20:1297–303. doi: 10.1101/gr.107524.110
47. Delcher, AL, Salzberg, SL, and Phillippy, AM. Using MUMmer to identify similar regions in large sequence sets. Curr Protoc Bioinformatics. (2003) 10:s00. doi: 10.1002/0471250953.bi1003s00
48. Sahl, JW, Lemmer, D, Travis, J, Schupp, JM, Gillece, JD, Aziz, M, et al. NASP: an accurate, rapid method for the identification of SNPs in WGS datasets that supports flexible input and output formats. Microb Genom. (2016) 2:e000074. doi: 10.1099/mgen.0.000074
49. Nguyen, LT, Schmidt, HA, von Haeseler, A, and Minh, BQ. IQ-TREE: a fast and effective stochastic algorithm for estimating maximum-likelihood phylogenies. Mol Biol Evol. (2015) 32:268–74. doi: 10.1093/molbev/msu300
50. Kalyaanamoorthy, S, Minh, BQ, Wong, TKF, von Haeseler, A, and Jermiin, LS. ModelFinder: fast model selection for accurate phylogenetic estimates. Nat Methods. (2017) 14:587–9. doi: 10.1038/nmeth.4285
51. Li, H, Handsaker, B, Wysoker, A, Fennell, T, Ruan, J, Homer, N, et al. The sequence alignment/map format and SAMtools. Bioinformatics. (2009) 25:2078–9. doi: 10.1093/bioinformatics/btp352
52. Putz, EJ, Andreasen, CB, Stasko, JA, Fernandes, LG, Palmer, MV, Rauh, MJ, et al. Circulating foamy macrophages in the Golden Syrian Hamster (Mesocricetus auratus) model of leptospirosis. J Comp Pathol. (2021) 189:98–109. doi: 10.1016/j.jcpa.2021.10.004
53. R. Core Team. R: A language and environment for statistical computing. R Foundation for Statistical Computing, Vienna, Austria. Available at: https://www.R-project.org/. (2021)
54. Hamond, C, Browne, AS, de Wilde, LH, Hornsby, RL, LeCount, K, Anderson, T, et al. Assessing rodents as carriers of pathogenic Leptospira species in the U.S. Virgin Islands and their risk to animal and public health. Sci Rep. (2022) 12:4846. doi: 10.1038/s41598-022-04846-3
55. Nascimento, AL, Ko, AI, Martins, EA, Monteiro-Vitorello, CB, Ho, PL, Haake, DA, et al. Comparative genomics of two Leptospira interrogans serovars reveals novel insights into physiology and pathogenesis. J Bacteriol. (2004) 186:2164–72. doi: 10.1128/JB.186.7.2164-2172.2004
56. Nascimento, AL, Verjovski-Almeida, S, Van Sluys, MA, Monteiro-Vitorello, CB, Camargo, LE, Digiampietri, LA, et al. Genome features of Leptospira interrogans serovar Copenhageni. Braz J Med Biol Res. (2004) 37:459–77. doi: 10.1590/S0100-879X2004000400003
57. Alt, DP, Wilson-Welder, JH, Bayles, DO, Cameron, C, Adler, B, Bulach, DM, et al. Complete genome sequence of Leptospira interrogans Serovar Bratislava, strain PigK151. Genome Announc. (2015) 3:e15. doi: 10.1128/genomeA.00678-15
58. Nally, JE, Wilson-Welder, JH, Hornsby, RL, Palmer, MV, and Alt, DP. Inbred rats as a model to study persistent renal leptospirosis and associated cellular immune responsiveness. Front Cell Infect Microbiol. (2018) 8:66. doi: 10.3389/fcimb.2018.00066
59. Nally, JE, Whitelegge, JP, Bassilian, S, Blanco, DR, and Lovett, MA. Characterization of the outer membrane proteome of Leptospira interrogans expressed during acute lethal infection. Infect Immun. (2007) 75:766–73. doi: 10.1128/IAI.00741-06
60. Fernandes, LG, Putz, EJ, Stasko, J, Lippolis, JD, Nascimento, AL, and Nally, JE. Evaluation of LipL32 and LigA/LigB knockdown mutants in Leptospira interrogans Serovar Copenhageni: impacts to proteome and virulence. Front Microbiol. (2022) 12:799012. doi: 10.3389/fmicb.2021.799012
61. Monti, G, Montes, V, Tortosa, P, Tejeda, C, and Salgado, M. Urine shedding patterns of pathogenic Leptospira spp. in dairy cows. Vet Res. (2023) 54:1190. doi: 10.1186/s13567-023-01190-w
62. Stallman, ND. International committee on systematic bacteriology subcommittee on the taxonomy of Leptospira: minutes of the meeting, 5 and 6 September 1986, Manchester, England. Int J Syst Evol Microbiol. (1987) 37:472–3. doi: 10.1099/00207713-37-4-472
63. Brenner, DJ, Kaufmann, AF, Sulzer, KR, Steigerwalt, AG, Rogers, FC, and Weyant, RS. Further determination of DNA relatedness between serogroups and serovars in the family Leptospiraceae with a proposal for Leptospira alexanderi sp. nov. and four new Leptospira genomospecies. Int J Syst Evol Microbiol. (1999) 49:839–58. doi: 10.1099/00207713-49-2-839
64. Guglielmini, J, Bourhy, P, Schiettekatte, O, Zinini, F, Brisse, S, and Picardeau, M. Genus-wide Leptospira core genome multilocus sequence typing for strain taxonomy and global surveillance. PLoS Negl Trop Dis. (2019) 13:e0007374. doi: 10.1371/journal.pntd.0007374
66. Dikken, H, Kmety, E, De Geus, A, and Timmer, V. A new leptospiral serovar in the Australis serogroup. Trop Geogr Med. (1979) 31:263–8.
67. Que-Gewirth, NL, Ribeiro, AA, Kalb, SR, Cotter, RJ, Bulach, DM, Adler, B, et al. A methylated phosphate group and four amide-linked acyl chains in Leptospira interrogans lipid a. the membrane anchor of an unusual lipopolysaccharide that activates TLR2. J Biol Chem. (2004) 279:25420–9. doi: 10.1074/jbc.M400598200
68. Zilch, TJ, Lee, JJ, Saleem, MZ, Zhang, H, Cortese, V, Voris, N, et al. Equine leptospirosis: experimental challenge of Leptospira interrogans serovar Bratislava fails to establish infection in naïve horses. Equine Vet J. (2021) 53:845–54. doi: 10.1111/evj.13442
69. Nally, JE, Monahan, AM, Miller, IS, Bonilla-Santiago, R, Souda, P, and Whitelegge, JP. Comparative proteomic analysis of differentially expressed proteins in the urine of reservoir hosts of leptospirosis. PLoS One. (2011) 6:e26046. doi: 10.1371/journal.pone.0026046
70. Ristow, P, Bourhy, P, da Cruz McBride, FW, Figueira, CP, Huerre, M, Ave, P, et al. The OmpA-like protein Loa22 is essential for leptospiral virulence. PLoS Pathog. (2007) 3:e97. doi: 10.1371/journal.ppat.0030097
71. Fernandes, LGV, Teixeira, AF, and Nascimento, A. Evaluation of Leptospira interrogans knockdown mutants for LipL32, LipL41, LipL21, and OmpL1 proteins. Front Microbiol. (2023) 14:1199660. doi: 10.3389/fmicb.2023.1199660
72. Chang, YF. The reproductive syndrome in equine leptospirosis. Equine Vet J. (2021) 53:856. doi: 10.1111/evj.13447
73. Ellis, W, and Thiermann, A. Isolation of Leptospira interrogans serovar Bratislava from sows in Iowa. Am J Vet Res. (1986) 47:1458–60.
74. Bolin, CA, and Cassells, JA. Isolation of Leptospira interrogans serovars Bratislava and hardjo from swine at slaughter. J Vet Diagn Invest. (1992) 4:87–9. doi: 10.1177/104063879200400121
75. Guitian, J, Thurmond, MC, and Hietala, SK. Infertility and abortion among first-lactation dairy cows seropositive or seronegative for Leptospira interrogans serovar Hardjo. J Am Vet Med Assoc. (1999) 215:515–8. doi: 10.2460/javma.1999.215.04.515
76. Hamond, C, Pestana, CP, Rocha-de-Souza, CM, Cunha, LE, Brandão, FZ, Medeiros, MA, et al. Presence of leptospires on genital tract of mares with reproductive problems. Vet Microbiol. (2015) 179:264–9. doi: 10.1016/j.vetmic.2015.06.014
77. Putz, EJ, and Nally, JE. Investigating the immunological and biological equilibrium of reservoir hosts and pathogenic Leptospira: balancing the solution to an acute problem? Front Microbiol. (2020) 11:2005. doi: 10.3389/fmicb.2020.02005
78. Steven, EW, Rogers, GM, Ramachandran, S, Engelken, TJ, Epperson, WB, Larson, RL, et al. Herd prevalence and risk factors of Leptospira infection in beef cow/calf operations in the United States. The. Bovine Practitioner. (2007) 41:15–23. doi: 10.21423/bovine-vol41no1p15-23
79. Ellis, WA, O’Brien, JJ, and Cassells, J. Role of cattle in the maintenance of Leptospira interrogans serotype Hardjo infection in Northern Ireland. Vet Rec. (1981) 108:555–7. doi: 10.1136/vr.108.26.555
80. Babudieri, B. Animal reservoirs of leptospires. Ann N Y Acad Sci. (1958) 70:393–413. doi: 10.1111/j.1749-6632.1958.tb35398.x
81. Helman, SK, Tokuyama, AFN, Mummah, RO, Stone, NE, Gamble, MW, Snedden, CE, et al. Pathogenic Leptospira are widespread in the urban wildlife of southern California. Sci Rep. (2023) 13:14368. doi: 10.1038/s41598-023-40322-2
82. Johnson, RC, and Harris, VG. Differentiation of pathogenic and saprophytic leptospires I. Growth at low temperatures. J Bacteriol. (1967) 94:27–31. doi: 10.1128/jb.94.1.27-31.1967
83. Ellis, W, Montgomery, J, and Cassells, J. Dihydrostreptomycin treatment of bovine carriers of Leptospira interrogans serovar Hardjo. Res Vet Sci. (1985) 39:292–5. doi: 10.1016/S0034-5288(18)31716-8
84. Nally, JE, Artiushin, S, and Timoney, JF. Molecular characterization of thermoinduced immunogenic proteins Qlp42 and Hsp15 of Leptospira interrogans. Infect Immun. (2001) 69:7616–24. doi: 10.1128/IAI.69.12.7616-7624.2001
85. Nally, JE, Timoney, JF, and Stevenson, B. Temperature-regulated protein synthesis by Leptospira interrogans. Infect Immun. (2001) 69:400–4. doi: 10.1128/IAI.69.1.400-404.2001
86. Putz, EJ, Sivasankaran, SK, Fernandes, LGV, Brunelle, B, Lippolis, JD, Alt, DP, et al. Distinct transcriptional profiles of Leptospira borgpetersenii serovar Hardjo strains JB197 and HB203 cultured at different temperatures. PLoS Negl Trop Dis. (2021) 15:e0009320. doi: 10.1371/journal.pntd.0009320
87. Monahan, AM, Callanan, JJ, and Nally, JE. Proteomic analysis of Leptospira interrogans shed in urine of chronically infected hosts. Infect Immun. (2008) 76:4952–8. doi: 10.1128/IAI.00511-08
88. Nally, JE, Grassmann, AA, Planchon, S, Sergeant, K, Renaut, J, Seshu, J, et al. Pathogenic Leptospires modulate protein expression and post-translational modifications in response to mammalian host signals. Front Cell Infect Microbiol. (2017) 7:362. doi: 10.3389/fcimb.2017.00362
89. Putz, EJ, Fernandes, LG, Sivasankaran, SK, Bayles, DO, Alt, DP, Lippolis, JD, et al. Some like it hot, some like it cold; proteome comparison of Leptospira borgpetersenii serovar Hardjo strains propagated at different temperatures. J Proteome. (2022) 262:104602. doi: 10.1016/j.jprot.2022.104602
90. Sykes, JE, Haake, DA, Gamage, CD, Mills, WZ, and Nally, JE. A global one health perspective on leptospirosis in humans and animals. J Am Vet Med Assoc. (2022) 260:1589–96. doi: 10.2460/javma.22.06.0258
91. Hamond, C, LeCount, K, Anderson, T, Putz, EJ, Stuber, T, Hicks, J, et al. Isolation and characterization of saprophytic and pathogenic strains of Leptospira from water sources in the Midwestern United States. Frontiers in Water. (2024) 6:8088. doi: 10.3389/frwa.2024.1278088
92. Nally, JE, Arent, Z, Bayles, DO, Hornsby, RL, Gilmore, C, Regan, S, et al. Emerging infectious disease implications of invasive mammalian species: the greater white-toothed shrew (Crocidura russula) is associated with a novel Serovar of pathogenic Leptospira in Ireland. PLoS Negl Trop Dis. (2016) 10:e0005174. doi: 10.1371/journal.pntd.0005174
Keywords: Leptospira, leptospirosis, equine, Australis, kirschneri
Citation: Hamond C, Adam EN, Stone NE, LeCount K, Anderson T, Putz EJ, Camp P, Hicks J, Stuber T, van der Linden H, Bayles DO, Sahl JW, Schlater LK, Wagner DM and Nally JE (2024) Identification of equine mares as reservoir hosts for pathogenic species of Leptospira. Front. Vet. Sci. 11:1346713. doi: 10.3389/fvets.2024.1346713
Received: 29 November 2023; Accepted: 24 April 2024;
Published: 09 May 2024.
Edited by:
Valentina Virginia Ebani, University of Pisa, ItalyReviewed by:
Balamurugan Vinayagamurthy, National Institute of Veterinary Epidemiology and Disease Informatics (ICAR), IndiaCopyright © 2024 Hamond, Adam, Stone, LeCount, Anderson, Putz, Camp, Hicks, Stuber, van der Linden, Bayles, Sahl, Schlater, Wagner and Nally. This is an open-access article distributed under the terms of the Creative Commons Attribution License (CC BY). The use, distribution or reproduction in other forums is permitted, provided the original author(s) and the copyright owner(s) are credited and that the original publication in this journal is cited, in accordance with accepted academic practice. No use, distribution or reproduction is permitted which does not comply with these terms.
*Correspondence: Jarlath E. Nally, amFybGF0aC5uYWxseUB1c2RhLmdvdg==
Disclaimer: All claims expressed in this article are solely those of the authors and do not necessarily represent those of their affiliated organizations, or those of the publisher, the editors and the reviewers. Any product that may be evaluated in this article or claim that may be made by its manufacturer is not guaranteed or endorsed by the publisher.
Research integrity at Frontiers
Learn more about the work of our research integrity team to safeguard the quality of each article we publish.