- 1College of Animal Science and Technology, Southwest University, Chongqing Beef Cattle Engineering Technology Research Center, Chongqing, China
- 2Chongqing Academy of Animal Sciences, Chongqing, China
- 3Chongqing Animal Husbandry Technology Extension Station, Chongqing, China
The objective of this study was to determine the effects of heat stress (HS) on physiological, blood biochemical, and energy metabolism in Dazu black goats. Six wether adult Dazu black goats were subjected to 3 experimental periods: high HS (group H, temperature-humidity index [THI] > 88) for 15 d, moderate HS (group M, THI was 79-88) for 15 d, and no HS (group L, THI < 72) for 15 d. Rectal temperature (RT) and respiratory rate (RR) were determined on d 7 and 15 of each period, and blood samples were collected on d 15 of each period. All goats received glucose (GLU) tolerance test (GTT) and insulin (INS) tolerance test on d 7 and d 10 of each period. The results showed that HS decreased dry matter intake (DMI) and INS concentrations (p < 0.05), and increased RT, RR, non-esterified fatty acid (NEFA), cortisol (COR), and total protein (TP) concentrations (p < 0.05). Compared to group L, the urea nitrogen (BUN) concentration increased and GLU concentration decreased in group H (p < 0.05).During the GTT, the area under the curve (AUC) of GLU concentrations increased by 12.26% (p > 0.05) and 40.78% (p < 0.05), and AUC of INS concentrations decreased by 26.04% and 14.41% (p < 0.05) in groups H and M compared to group L, respectively. The INS concentrations were not significant among the three groups (p > 0.05) during the ITT. A total of 60 differentially expressed metabolites were identified in response to groups H and M. In HS, changes in metabolites related to carbohydrate metabolism and glycolysis were identified (p < 0.05). The metabolites related to fatty acid βoxidation accumulated, glycogenic and ketogenic amino acids were significantly increased, while glycerophospholipid metabolites were decreased in HS (p < 0.05). HS significantly increased 1-methylhistidine, creatinine, betaine, taurine, taurolithocholic acid, inosine, and hypoxanthine, while decreasing vitamin E in blood metabolites (p < 0.05). In summary, HS changed the metabolism of fat, protein, and energy, impaired GLU tolerance, and mainly increased amino acid metabolism to provide energy in Dazu black goats.
1 Introduction
Heat stress (HS) is the body’s nonspecific response under high temperature environment to produce a reaction combined. Animals with HS may experience a range of physiological and behavioral abnormalities that impair their ability to reproduce, grow, and produce (1, 2). According to a study by Hashem et al. (3), HS reduced the pH, cooking loss, water holding capacity, and shear force of Black Bengal goats’ meat. HS has been shown to decrease growth performance and milk production of dairy goats by 12 and 3–10%, respectively (4, 5). HS can result in total animal losses averaging $2.4 billion annually (6). In addition, HS may cause damage to animal proteins, fats, and carbohydrates metabolism (7). It was found that HS caused a significant decrease in the abundance of several polar lipids such as phosphatidylcholine, phosphatidylserine, lysophosphatidylcholine, and glucosylceramide, while significantly increased the activities of glycogen phosphorylase and pyruvate dehydrogenase in muscle, as well as increased protein degradation (8, 9). The temperature–humidity index (THI) is a common bioclimatic indicator to assess HS. Goats critical temperature of the HS in 35–40°C (5), and goats can occur when the THI exceeds 80 (10). Goats have a wide isothermal zone, are highly resistant to heat (11), and recover from HS via physiological, biochemical, and metabolic changes (12–14). However, HS and even death of goats may occur at high environmental temperature (ET) and relative humidity (RH), especially for goats with production needs. ET of 34–36°C are reported to reduce the conception rate of female goats (15) and HS was found to decrease the expression of genes related to reproductive efficiency in Malabari goats (16). The Dazu black goat is native to Chongqing, China, and is characterized by a short black coat. It has the characteristics of cold and drought tolerance, strong stress resistance, efficient disease resistance, and roughage-resistance, which is of great significance to the development of livestock farming. Notably, black coats absorb more solar radiation, and short-haired goats tolerate radiant heat less than hairy goats (5). In Chongqing, the ET exceeds 35°C for an average of 40.77 d during the summer months with an average annual RH of 80%, which belongs to the high humidity area. Moreover, the housing environments of goats can be even more humid, which is more likely to cause HS for Dazu black goats. To sum up, no HS occurs when THI is less than 77.33 (17), was alert and in danger between 80 and 90, with extreme danger beyond 90 for goats (10), which influenced the physiology, blood biochemical indices, metabolism, and even cause the death of goats. The performance of the Dazu black goat was greatly affected in the summer. Therefore, it is of great significance to study the changes in physiological indexes, blood biochemical indexes, and blood metabolites of Dazu black goats to improve the metabolic regulation, feed digestibility, and growth performance of goats during HS in order to prevent and control HS.
To date, relatively few studies have investigated the physiological and metabolic changes of Dazu black goats in response to high ET and RH. Therefore, the present study aimed to assess the effects of HS on the physiological and blood biochemical indices as well as endogenous metabolites of Dazu black goats. The results of this study will help to clarify the response mechanisms of goats to HS and provide basic parameters for efficient and healthy breeding under hot and humid conditions.
2 Materials and methods
2.1 Animals, diets, and experimental design
Six wether adult Dazu black goats (28.4 ± 3.2 kg of body weight) were subjected to 3 experimental periods with a single-factor self-controlled trial. The total length of the trial was 52 d, with the pre-feeding period was 7 d and the 3 experimental periods consisting of (1) 15 d of high HS (group H, THI > 88); (2) 15 d of moderate HS (group M, THI was 79–88); and (3) 15 d of no HS (group L, THI < 72). ET and RH were manually controlled for temperature and humidity with the use of 4 heaters, 2 humidifiers, and 2 air conditioners. Three temperature and humidity data logger devices (Testo, Inc., Sparta Township, NJ, United States), which located about 1.6 meters above the ground, were used to record the ET and RH every 30 min. The ET and RH were under control from 8:00 to 18:00 h and 18:01 to 7:59 h the next day during the three treatment phases. THI was calculated from ET and RH values which were recorded. Table 1 displays the ET, RH, and THI during the duration of the experiment. The THI was calculated according to the formula described in Hamzaoui et al. (18):
where Tdb is the dry bulb temperature (°C) and RH is the relative humidity (%).
All Dazu black goats were housed in single pens located in a barn on the Rongchang Campus of Southwest University and fed a total mixed ration (TMR) twice daily at 08:30 and 18:00 h with feed intake ad libitum and had free access to clean drinking water. It had displayed the composition and nutrient content of the daily TMR in Table 2. The ME of each raw material was calculated according to the Feeding Standard of Meat-Producing Sheep and Goats of Chinese Agricultural Industry Standards (HB, NY/T 816–2004). The quality of the TMR was checked periodically during the experiment to ensure the absence of mold and spores. The level of aflatoxin in the maize used, as determined by Total aflatoxin detection kit, was below the maximum tolerance threshold in the European Union. The results of this trial were not related to aflatoxins.
2.2 Temperature–humidity index
As can be seen in Figure 1, during the first period of the experiment, the THI was >88 at 15:00 h, indicating high HS. The THI varied daily from 79 to 88 throughout the experiment’s second period, indicating moderate HS. The THI was <72 in the third experiment period, which meant there was no HS.
2.3 Sample collection and analysis
2.3.1 Dry matter intake, rectal temperature, and respiratory rate
The average daily dry matter intake (DMI) of Dazu black goats was calculated as the difference between the initial amount of feed and the amount of feed leftover the following morning.
In accordance with the method described by Tucker et al. (19), rectal temperature (RT; obtained with a GLA 525/550 Hi-Performance Digital Thermometer, San Luis Obispo, CA) was measured by inserting a disinfected thermometer into the rectum of each goat at 08:00, 14:00, and 18:00 h on d 7 and 15 of each experimental period. The respiratory rate (RR) of each goat was recorded by calculating as breaths/min using a stopwatch for 1 min and the average value of three consecutive measurements was recorded.
2.3.2 Blood biochemistry
Blood samples were collected on d 15 of each experimental period before feeding at 8:00 h and used to prepare serum and plasma samples. After centrifugation at 3,000 rmp for 15 min, the supernatant was aspirated, and aliquots were stored in 1.5-mL centrifuge tubes at −20°C until assayed. Blood glucose (GLU), blood urea nitrogen (BUN), triglycerides (TG), cholesterol (CHO), high-density lipoprotein (HDL-C), and low density-lipoprotein (LDL-C) were measured with an automatic biochemical analyzer (model AU5800; Beckman Coulter, Inc., Brea, CA, United States). Commercial kits were used for the measurement of Non-esterified fatty acid (NEFA; Wako Chemicals GmbH, Neuss, Germany). Serum levels of total protein (TP), cortisol (COR), triiodothyronine (T3), thyroxin (T4), and insulin (INS) were measured with enzyme-linked immunosorbent assay (ELISA) kits (Nanjing Jiang Cheng Bioengineering Institute, Nanjing, China).
2.3.3 GLU tolerance test and INS tolerance test
At 08:00 h on d 7 and 10 of each period, GLU tolerance test (GTT) and INS tolerance test (ITT) of all goats was conducted after fasting for 12 h, respectively. A 50% dextrose (0.5 g/kg) solution (AGRIpharm Products, Grapevine, TX) was administered via the jugular catheter and immediately chased with 12 mL of sterile saline. GLU concentration was measured before insulin injection, which was administered at 0.75 U/Kg body weight. Blood samples were collected at 5 min before, at the time of GLU administration (0 min was used as a baseline parameter), and at 5, 10, 15, 20, 30, 45, 60, and 90 min after GLU load. Samples were collected into disposable glass culture tubes containing 250 U of sodium heparin and were immediately placed on ice. And then centrifuged at 3,000 rmp for 15 min. The plasma was divided into 2 aliquots, which were both frozen at −20°C; one aliquot was later analyzed for plasma glucose levels and the other for plasma insulin concentrations.
The GLU and INS responses to the GTT were measured as area under the curve (AUC). Mean GLU values from the samples obtained before the start of a challenge were used as the baseline metabolite concentrations, and the 0 time point sample was used for the INS baseline value. The GLU and INS AUC were calculated through the 90 min sample during the GTT. The blood GLU AUC was calculated through the 90 min sample during the ITT.
2.3.4 Blood metabolites
At 15:00 h, on the last day of all three experimental stages, 3 mL of blood were collected from each goat into tubes coated with heparin sodium as an anticoagulant. Following a 15-min centrifugation at 3,000 rmp, the serum was moved to a fresh tube and kept at −80°C for non-targeted metabolomics examination. Blood samples stored at −80°C were thawed slowly at 4°C for sample pretreatment and analyzed by the Agilent 1,290 Infinity LC ultra-high performance liquid chromatography system (UHPLC). The samples were separated by UHPLC and analyzed by a Triple TOF 5600 mass spectrometer (AB SCIEX). The positive ion (ESI+) and negative ion (ESI-) modes were used for detection, and the raw data were converted into.MZXML format by ProteoWizard for data processing.
Non-targeted metabolomics analysis included ultra-high performance liquid chromatography with quadrupole time-of-flight mass spectrometry and pathway enrichment analysis in reference to the Kyoto Encyclopedia of Genes and Genomes (KEGG) database.1 The KEGG database and MetPA software were used for pathway analysis of potential biomarkers to identify related metabolic pathways. The KEGG pathway enrichment analysis of differential metabolites was performed by Fisher’s exact test.
2.3.5 Chemical analysis
The TMR samples were dried in an oven at 65°C until a constant weight was achieved. Upon drying, the samples were ground and passed through a 1-mm sieve for further analysis. The chemical composition of dry matter (DM), crude protein (CP), ether extract (EE), ash, calcium (Ca), and phosphorus (P) were measured according to the Association of Official Analytical Chemists [(20); AOAC]. Neutral detergent fiber (NDF) and acid detergent fiber (ADF) were determined according to Vansoest et al. (21). The ingredients and chemical composition of the Dazu black goats’ TMR are presented in Table 2.
2.3.6 Statistical analysis
Excel 2016 (Microsoft Corporation, Redmond, WA, United States) was used to record the initial DMI, RT, RR, and blood biochemical data. IBM SPSS Statistics for Windows, version 26.0, was used to analyze the data using one-way analysis of variance or the paired sample t-test (IBM Corporation, Armonk, NY, United States). Graphpad Prism 8 software was used to plot DMI, RT, RR, and tolerance texts graphs (San Diego, CA, United States). Statistical analysis between multiple groups of repeated measurement samples was performed by Repeat ANOVA Measurement of variance and p value was calculated. Results were shown as the Mean ± SD. Probability (p) value <0.05 was considered significant. Metabolites with VIP > 1 and p < 0.05 that were applied to Student’s t-test at univariate level among the groups were considered statistically significant.
3 Results
3.1 Dry matter intake
The average DMI of Dazu black goats significantly increased as the THI decreased. Notably, DMI was significantly lower in groups H and M as compared to group L (Figure 2; p < 0.05).
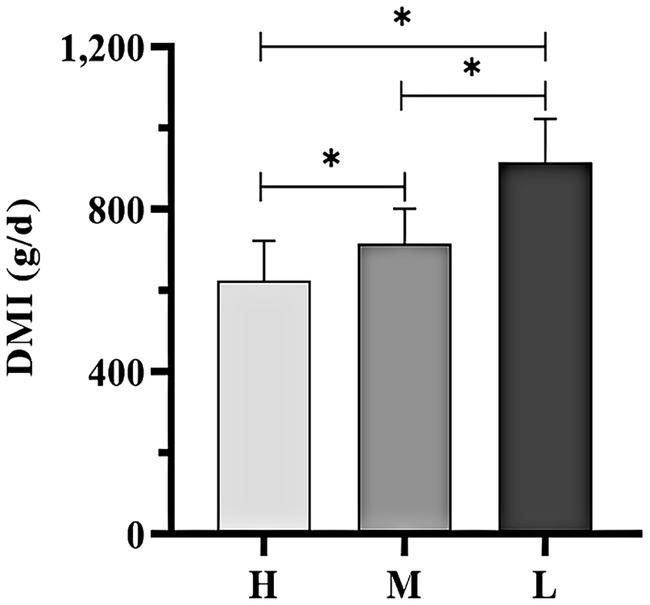
Figure 2. DMI of Dazu black goats of all experimental groups. *indicated significant difference (p < 0.05).
3.2 Rectal temperature and respiratory rate measurements
Table 3 displays the statistical data on RT and RR. Compared to group L HS increased the RT of Dazu black goats at all three time points in groups H and M (p < 0.05). But there was no significant difference in RT between groups H and M at 08:00 and 18:00 h (p > 0.05). HS significantly increased the RR of Dazu black goats at all three time points (p < 0.05). The highest measurements of both RT and RR occurred at 14:00 h.
3.3 Blood biochemical indices
3.3.1 Blood indices
Compared to group L, HS increased NEFA, COR, and TP concentrations and decreased INS concentration (p < 0.05). The BUN concentration increased and the GLU concentration decreased in group H compared to group L (p < 0.05). There were no significant differences in CHO, HDL-C, LDL-C, TG, T3, and T4 contents among the three groups (p > 0.05; Table 4).
3.3.2 GLU tolerance test
As shown in Figure 3A, during the GTT, the GLU concentration in group H was higher than that in group L (20.25–58.00%) during the 0–20 min period. The GLU concentration in group M was higher than that in group L (23.89–77.47%) during the 0–90 min period. Figure 3B shows that the INS concentrations in group H (18.19–42.73%) and group M (8.46–34.17%) were lower than those in group L during 5–60 min.
Figure 4A shows that the AUC of GLU concentrations in groups H and M were 12.26% (p > 0.05) and 40.78% (p < 0.05) higher than that in group L, respectively. Figure 4B shows that the AUC of INS concentrations in groups H and M were 26.04 and 14.41% (p < 0.05) lower than that in group L, respectively.
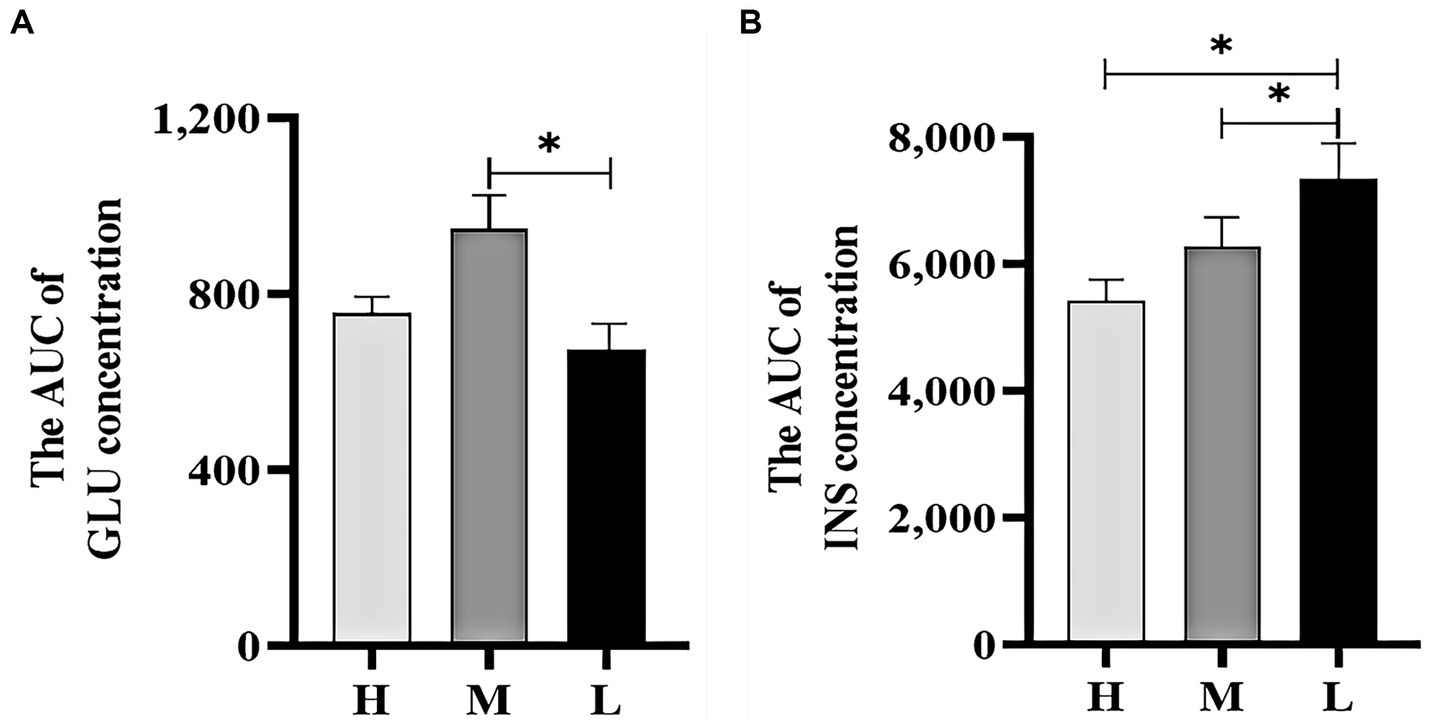
Figure 4. The AUC of GLU concentration (A) and INS concentration (B) during the GTT. *indicated significant difference (p < 0.05).
3.3.3 INS tolerance test
As shown in Figures 5, 6, the AUC of GLU concentrations were not significant among the three groups (p > 0.05).
3.4 The blood metabolome
In total, there were 75 differential metabolites between groups H and L, 77 between groups M and L, and 26 between groups H and M. As compared to group L, 60 significantly different metabolites were identified in groups H and M, which mainly included amino acids, organic amines, carbohydrates, organic acids, and esters (Table 5). As shown in Figure 7, serum levels of glycogenic amino acids and ketogenic amino acids were significantly increased in groups H and M compared to group L (p < 0.05). In addition, serum levels of taurine, creatinine, choline, indole-2-carboxylic acid, L-carnosine, diethanolamine, inosine, creatine, and carbohydrates were also significantly increased (p < 0.05), while the serum levels of phenylacetyl glycine, erucamide, alpha-tocopherol, 1-palmitoyl-sn-glycero-3-phosphocholine, creatine, PC (16:0/16:0), 1,2-dioleoyl-sn-glycero-3-phosphatidylcholine, glycerophosphocholine, thioetheramide-PC, indoxyl sulfate, prostaglandin F3α, arachidic acid, and perindopril were significantly decreased (p < 0.05) in groups H and M compared to group L.
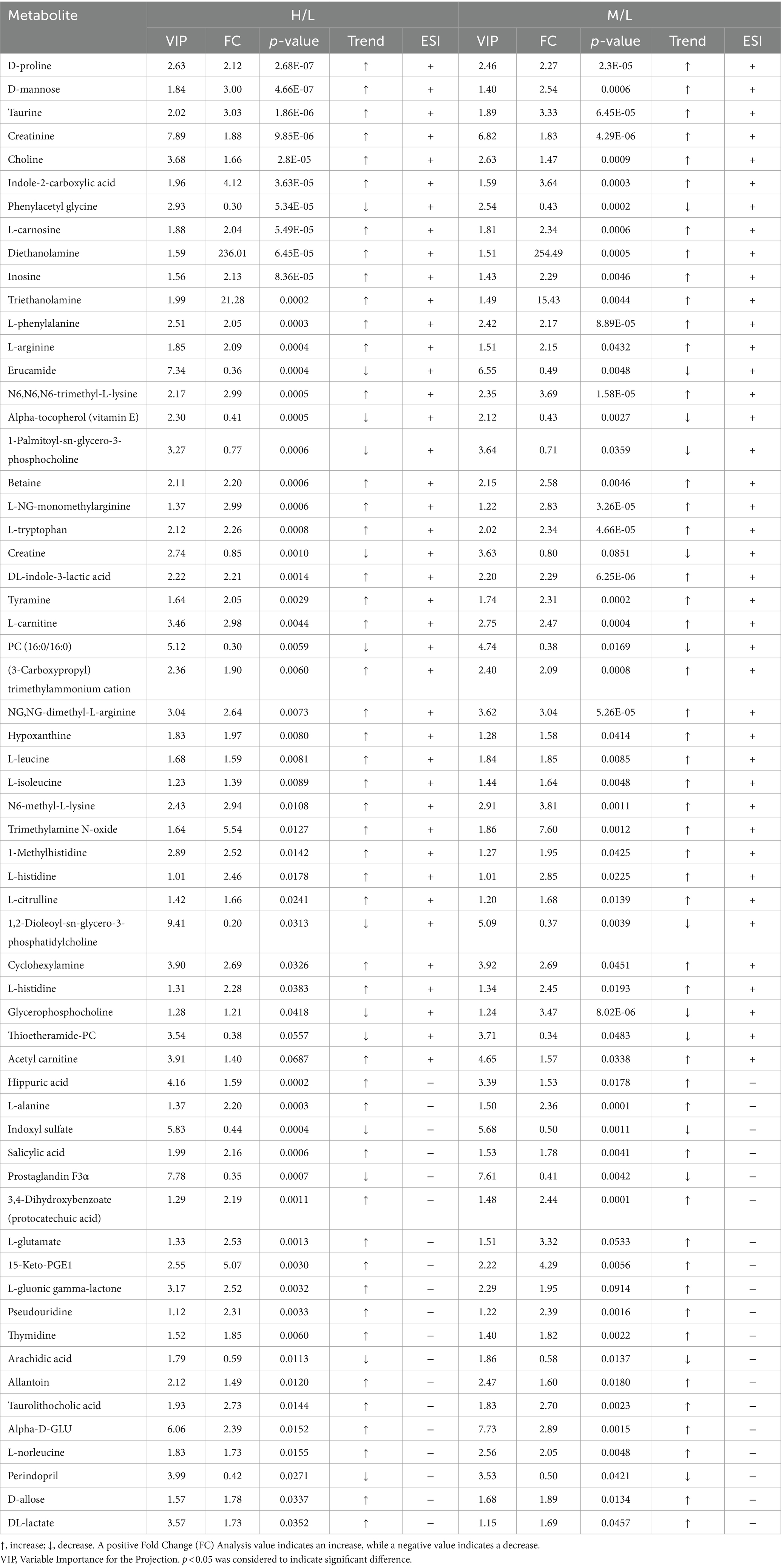
Table 5. Identified differential metabolites of the groups H, M, and L in positive and negative ion modes.
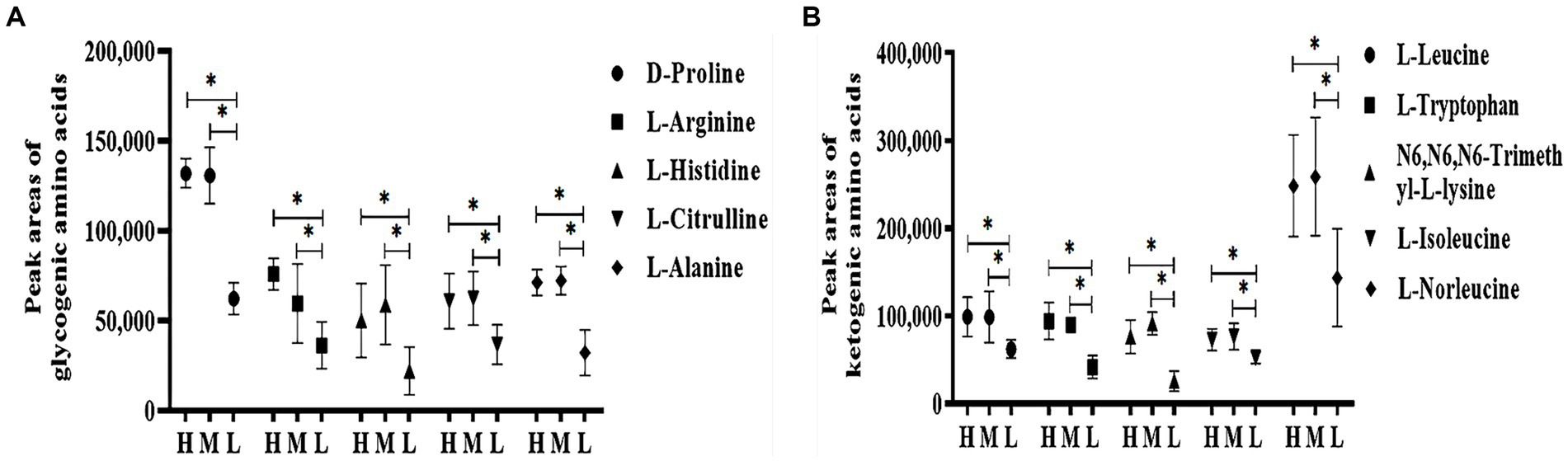
Figure 7. Effects of HS on peak areas of amino acid in Dazu black goats. (A) Glycogenic amino acids in the plasma; (B) Ketogenic amino acids in the plasma. *indicated significant difference (p < 0.05).
Comprehensive analysis of the differential metabolites obtained by comparisons of two pairs using KEGG metabolic pathways. The results showed that the differential metabolites in plasma were associated with “amino acid metabolism,” “lipid metabolism,” “carbohydrate metabolism,” “nucleotide metabolism,” “central carbon metabolism,” “aminoacyl-tRNA biosynthesis,” “ABC transporter” as well as digestion and absorption of proteins and minerals (Table 6).
4 Discussion
4.1 Physiological indicators
In this experiment, DMI increased as the THI decreased. In response to HS, animal secretion and expression of adiponectin and leptin will increase, adiponectin regulates feeding behavior by stimulating peripheral receptors to transmit nerve impulses to the hypothalamus, while leptin stimulates the hypothalamic appetitive center to reduce food intake (22–25), ultimately reducing metabolism and heat production. Reduced DMI and nutrient digestibility by HS can lead to undernutrition in animals and adversely affect their health (26). HS is reported to decrease DMI in sheep (27), dairy goats (18), and dairy cows (28).
RR and RT are the most common physiological indices of HS. Exposure to high ET inhibits the ability to dissipate heat, resulting in increased RT. Meanwhile, the RR is increased to enhance lung ventilation and dissipate heat (29). Under suitable environmental conditions, the RT of ewes fluctuates between 38.3°C and 39.0°C (30). Shilja et al. (31) found that the RR and RT of goats were significantly higher in the HS group than in the non-HS group (69.17 breaths/min and 39.08°C vs. 31.92 breaths/min and 38.70°C, respectively). Marai et al. (32) reported that the RR and RT of goats were significantly higher in the summer than the winter, but varied among different breeds, and physiological changes were greater in cold-adapted breeds during the summer than heat-adapted breeds. The results of the present study showed that the RR and RT of goats were significantly increased in response to HS, as RT increased to 39.8°C. Banerjee et al. (33) found that an increase in RT of ≤1°C decreased productivity and reproductive capacity.
4.2 Blood biochemistry
The hypothalamic–pituitary–adrenal axis of the neuroendocrine system is primarily involved in the stress response (34). HS-induced stimulation transmits nerve impulses through the cerebral cortex to the hypothalamus, which then releases hormones that promote the secretion of adrenocorticotropic hormone (ACTH) and inhibit the production of thyroid hormone (TSH). ACTH and TSH act on the adrenal and thyroid glands, respectively, thus increasing the secretion of COR by the adrenal glands while decreasing the production of T3 and T4 by the thyroid gland. In response to HS, serum levels of COR are increased (35). COR is a common biomarker of the stress response and can help maintain the internal environment and reduce HS-induced damage (36, 37). However, excessive production of COR in response to long-term HS can damage immune-related organs by inducing the release of inflammatory factors and promoting the aggregation and adhesion of leukocytes, thereby triggering an inflammatory response. Exposure to excessive heat for more than 2 h will elevate serum COR levels (38). Przemyslaw et al. (39) reported that exposure to ET of 50°C increased serum COR levels of Merino rams by nearly 10 fold. In addition, HS-induced increases in serum levels of COR and epinephrine generally inhibit the production of INS and TG while promoting glycolysis, gluconeogenesis, lipolysis, and the production of NEFA (40, 41).
Low serum GLU can promote lipid mobilization (42), resulting in the release of NEFA into the blood for energy production. Therefore, increased serum levels of NEFA can conserve GLU (28). The decrease in serum levels of GLU with increased concentrations of NEFA in response to HS supports this view. HS was confirmed to decrease serum levels of GLU in cows (43), goats (44), and calves (45). The results of GTT and ITT showed that HS impaired GLU tolerance, but did not change INS tolerance and sensitivity, so it is speculated that HS may change blood GLU concentration by affecting INS secretion rather than sensitivity. In the context of HS, the increase in blood GLU concentration during the GTT can be attributed to the altered metabolic responses induced by the stress condition. HS can lead to the release of stress hormones such as COR, which can promote gluconeogenesis and glycogenolysis, consequently elevating blood GLU concentration (46). Additionally, HS may reduce INS secretion, further contributing to the hyperglycemic response observed during the GTT under HS conditions (47).
HS can promote the production of free radicals and subsequent oxidative damage and apoptosis (48, 49). Oxidation helps to maintain cellular integrity and provides energy. Antioxidation works in tandem with aerobic metabolism to combat free radical-induced tissue damage. Moreover, reduced production of antioxidants can promote oxidative stress (48) and increase secretion of inflammatory cytokines (50). Excessive oxidation induces the body to produce excessive inflammatory response and immune response, which further aggravates the damage of tissues, organs and systems. Reactive oxygen species (ROS) can increase protein degradation while reducing protein synthesis (48). Finocchiaro et al. (51) found that the protein content in ewe milk was negatively correlated with the THI during HS. In the present study, the TP increased significantly under HS, indicating that goat body protein degradation increased at this time. A large number of reactive oxygen species can damage the protein structure, DNA structure, cell membrane structure and various organelles of cells, thereby causing systemic inflammation, such as fatty liver, laminitis, metritis and mastitis, and reducing the yield and quality of milk and meat.
4.3 Blood metabolite
In this study, there were notable changes to metabolites of carbohydrate metabolism, gluconeogenesis, and glycolysis (e.g., alpha-D-GLU, D-allose, Phenylacetyl glycine, hippuric acid, glycogenic amino acids, and DL-lactate; Figure 8), indicating that HS influences the energy metabolism of Dazu black goats. The increased contents of alpha-D-GLU and D-allose in response to HS indicate decreased energy expenditure with increasing ET, which could reduce heat production in goats. Serum levels of glycogenic amino acids, including D-proline, L-arginine, L-histidine, L-citrulline, and L-alanine, were increased in response to HS, indicating enhanced gluconeogenesis. In a state of HS, goats experience decreased DMI and energy supply, leading to insufficient nutrient intake, which results in the use of stored nutrients for energy, thereby increasing protein degradation and serum levels of amino acids. When the energy supply is low, glycogen reserves are limited, and carbohydrate transport occurs through gluconeogenesis (52). In addition, decreased production of TSH, T3, and T4 with increased production of ROS in response to HS will increase protein degradation and serum levels of amino acids. Guo et al. (53) found increased serum concentrations of total amino acids in cows in response to HS, especially glucogenic amino acids (alanine, aspartic acid, glutamic acid, and glycine). Cowley et al. (54) reported that low serum concentrations of GLU can increase the consumption of amino acids, thereby promoting gluconeogenesis in cows in response to HS. DL-lactate, the main metabolite of glycolysis, accumulated in the blood of Dazu black goats during HS. In addition to the amino acids involved in gluconeogenesis, those related to glycolysis are also increased by HS (55). L-alanine can regulate gluconeogenesis and glycolysis to ensure energy production when energy intake is insufficient (56). Increased serum levels of metabolites, such as alpha-D-GLU, glucogenic amino acids, and DL-lactate, indicate enhanced gluconeogenesis and glycolysis in response to HS to meet energy requirements. These changes were all observed in KEGG pathways (Table 6).
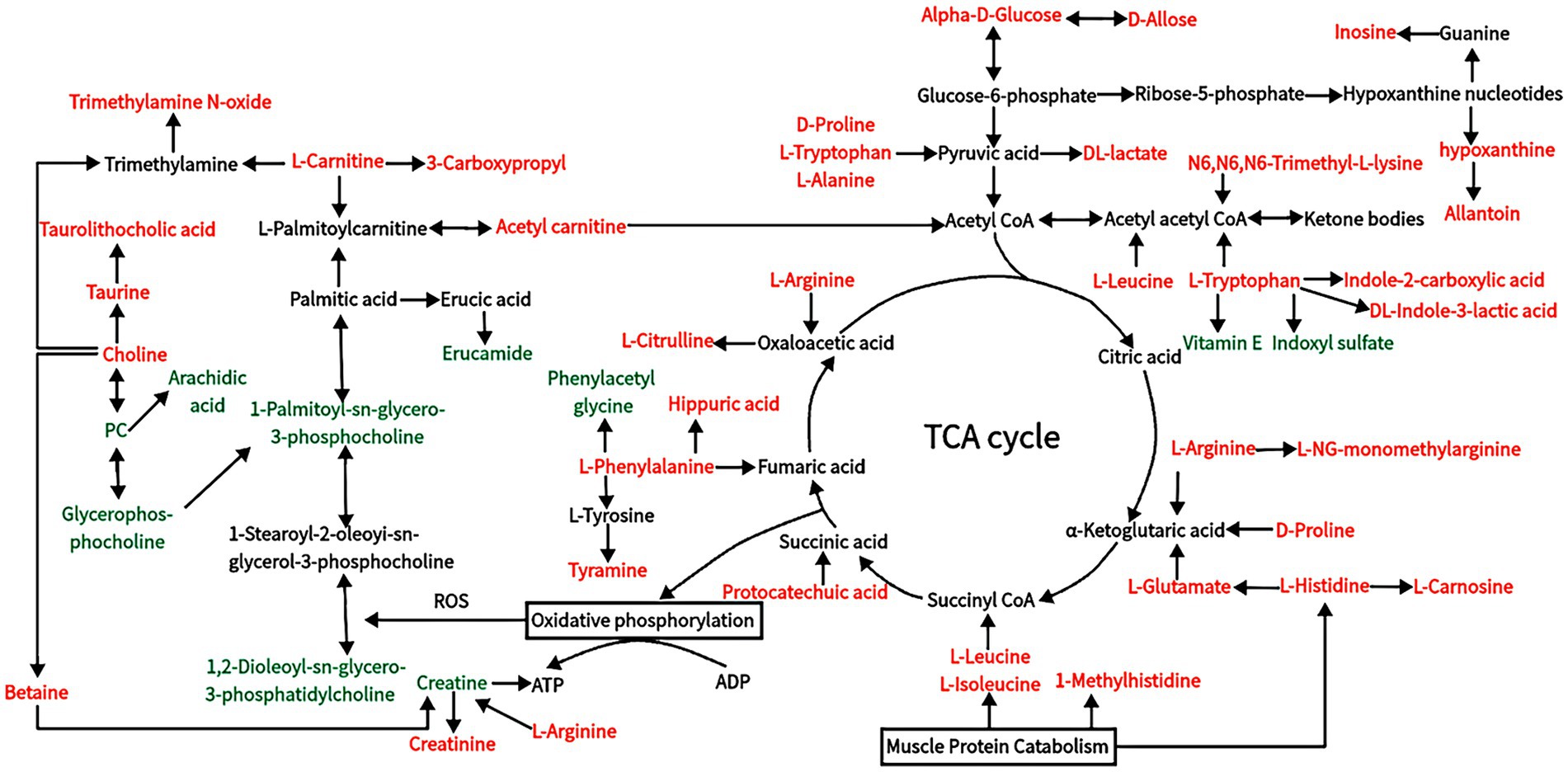
Figure 8. Changes in blood metabolic pathways of Dazu black goats under HS. The metabolites are colored according to the type of change in response to HS (black, no change; red, upregulation; green, downregulation).
L-carnitine and acetyl carnitine are metabolites of fatty acids (57) and act as carriers of long-chain fatty acids, such as palmitoylcarnitine, across the mitochondrial inner membrane for fatty acid β-oxidation. Acetyl carnitine is produced in the mitochondrial matrix by carnitine and acetyl coenzyme A (58). Metabolites of acetylcarnitine can undergo fatty acid oxidation and enter the tricarboxylic acid cycle. Accumulation of L-carnitine indicates inhibition of β-oxidation in goats in a state of HS. However, L-carnitine can be beneficial by inhibiting aerobic oxidation of lipids, thereby reducing oxidative stress (59). The main application of vitamin E is as an antioxidant to protect polyunsaturated lipids from damage brought on by free radicals (60). In this study, the content of vitamin E was significantly decreased in goats in response to HS, indicating that HS may reduce the production of antioxidants. Therefore, Dazu black goats may resist cellular oxidative stress by reducing fatty acid β-oxidation rather than antioxidant regulation. Under physiological conditions, energy is produced by the complete oxidation of fatty acids in the kidney, myocardium, and other tissues. However, incomplete oxidation of fatty acids in liver cells forms ketone bodies. Notably, serum levels of ketogenic amino acids (L-leucine, L-tryptophan, L-lysine, L-isoleucine, and L-norleucine) were relatively increased in the HS group as compared to the non-HS group. Low serum GLU indicates insufficient intake of exogenous nutrients and cellular energy production. Intake of amino acids by liver cells increases production of ketone bodies, which then enter the circulation and are oxidized in extrahepatic tissues to supply energy. The L-leucine and L-isoleucine not only participate in ketogenesis but also play roles in immune regulation and protein metabolism (61, 62).
In addition to influencing the metabolism of liver cells, HS also significantly impacts the kidneys, which are crucial for physiological functions (63, 64). Under the conditions of HS and decreased feed intake, amino acid utilization is increased, leading to increased methylhistidine production as a marker of muscle fibrinolysis and increased liver urea synthesis (52). Abdelnour et al. (8) and Kamiya et al. (65) found that HS could break down histamine, as evidenced by increased serum concentrations of methylhistidine and urea. In the present study, serum levels of methylhistidine were significantly increased in response to HS, indicating increased fibrin catabolism. HS also increases serum concentrations of catecholamine and COR, leading to increased resistance in the kidneys and visceral vessels, resulting in renal ischemia and the timely excretion of metabolites (66). Furthermore, HS causes vasodilation and evaporative water loss, leading to lower blood pressure, followed by increased water retention, resulting in decreased renal clearance (67, 68). These two conditions are the main reasons for elevated serum concentrations of methylhistidine and urea. In addition, creatinine levels are significantly increased under HS conditions (8). The serum content of creatinine, a metabolite of creatine, is a reliable indicator of muscle tissue degradation (69). The serum concentration of creatinine is dependent on glomerular filtration and increases with impaired renal function. Although the creatine content was reduced under HS conditions due to decreased renal clearance, serum creatinine eventually increased. Citrulline is a product of muscle metabolism and is mainly filtered by the kidneys. Therefore, the serum citrulline concentration is considered a marker of renal function (70). An abnormally high serum citrulline level is an indicator of impaired renal function.
Serum levels of choline, betaine, taurine, and taurocholic acid were significantly increased, suggesting that Dazu black goats mainly recover from HS by producing a series of metabolites. Phospholipids, including glycerophospholipids and sphingosine phospholipids, are the main components of biofilms. In animals, phospholipids are hydrolyzed into glycerol, phosphate, choline, and ethanolamine by a series of phospholipases. Glycerophospholipids are major lipids in cell membranes and play important roles in cell signaling, G protein-coupled receptors, and ion transport (71). In the present study, serum levels of glycerophosphocholine, 1,2-dioleoyl-sn-glycero-3-phosphatidylcholine, and 1-palmitoyl-sn-glycero-3-phosphocholine were decreased in Dazu black goats in response to HS (Figure 8). Decreased levels of glycerophospholipid metabolites, such as hotline, indicate changes to the cell membrane structure and function of Dazu black goats. In addition, a significant decrease in glycerophospholipids alters the permeability and fluidity of cell membranes, which can serve as a defense mechanism to protect against oxidative damage caused by ROS (72, 73). Choline is a key precursor for the synthesis of acetylcholine (74), which can be easily oxidized to betaine (75), and plays an important regulatory role in maintaining cellular structural integrity and reducing oxidative stress (76). Heat shock proteins (HSPs) gradually restore proteins denatured by heat damage to normal states by refolding and preventing protein aggregation. Betaine can effectively improve the folding rate and decomposition rate of HSPs, thus enhancing their ability to withstand HS (77, 78). Choline, which reduces oxidative stress and thermal injury through a series of enzymatic reactions, produces glutathione and taurine (79, 80). Taurine can neutralize cholic acid in the liver to synthesize taurocholate, which promotes the absorption of lipids and fat-soluble vitamins. Chronic HS has been shown to influence purine metabolism, RNA transport, and down-regulate the metabolism of L-arginine and D-proline in dairy goats, while activating pathways associated with apoptosis and inhibiting pathways associated with tissue repair (81). In this study, serum levels of inosine and hypoxanthine were increased in response to HS, indicating that HS can induce apoptosis. Therefore, the contents of choline, betaine, taurine, and taurocholic acid were increased to recover from heat injury, and the increased L-arginine and D-proline may be mainly used for energy metabolism.
5 Conclusion
HS decreased DMI and increased the RR and RT in Dazu black goats. HS changed blood hormone levels, increased protein degradation, increased plasma amino acid concentrations, elevated lipid levels, and impaired GLU tolerance. Gluconeogenesis, glycolysis, and ketogenic metabolism are increased under HS, thereby altering energy metabolic pathways in goats. Moreover, Dazu black goats primarily used an increase in amino acid metabolism as a source of energy in HS. In addition, in response to HS, a series of metabolites are produced to restore the heat damage of the organism.
Data availability statement
The original contributions presented in the study are included in the article/supplementary material, further inquiries can be directed to the corresponding authors.
Ethics statement
The animal study was approved by the Institutional Animal Care and Use Committee of Southwest University (Chongqing, China). The study was conducted in accordance with the local legislation and institutional requirements.
Author contributions
LW: Conceptualization, Data curation, Formal analysis, Investigation, Methodology, Validation, Writing – original draft, Writing – review & editing. PZ: Data curation, Investigation, Validation, Writing – original draft. YD: Investigation, Validation, Writing – original draft. CW: Investigation, Validation, Writing – original draft. LZ: Writing – review & editing. LY: Conceptualization, Data curation, Formal analysis, Investigation, Methodology, Validation, Writing – original draft. FZ: Conceptualization, Funding acquisition, Project administration, Resources, Writing – review & editing. WH: Conceptualization, Data curation, Formal analysis, Funding acquisition, Project administration, Resources, Writing – review & editing.
Funding
The author(s) declare that financial support was received for the research, authorship, and/or publication of this article. This study was supported by the Special Key Project of Chongqing Technology Innovation and Application Development (cstc2021jscx-tpyzxX0014) and the Key Project of Chong Qing Natural Science Foundation (Grant No. cstc2020jcyj-zdxmX0005).
Acknowledgments
The authors acknowledge FZ and WH for financing and organizing the feeding trial. The authors are thankful to the Rongchang Campus of Southwest University for running a feeding trial.
Conflict of interest
The authors declare that the research was conducted in the absence of any commercial or financial relationships that could be construed as a potential conflict of interest.
Publisher’s note
All claims expressed in this article are solely those of the authors and do not necessarily represent those of their affiliated organizations, or those of the publisher, the editors and the reviewers. Any product that may be evaluated in this article, or claim that may be made by its manufacturer, is not guaranteed or endorsed by the publisher.
Footnotes
References
1. Sejian, V, Maurya, VP, Kumar, K, and Naqvi, SMK. Effect of multiple stresses (thermal, nutritional, and walking stress) on the reproductive performance of malpura ewes. Vet Med Int. (2012) 2012:471760. doi: 10.1155/2012/471760
2. Summer, A, Lora, I, Formaggioni, P, and Gottardo, F. Impact of heat stress on milk and meat production. Anim Front. (2019) 9:39–46. doi: 10.1093/af/vfy026
3. Hashem, MA, Hossain, MM, Rana, MS, Hossain, MM, Islam, MS, and Saha, NG. Effect of heat stress on blood parameter, carcass and meat quality of black bengal goat. Bangladesh J Animal Sci. (2013) 42:57–61. doi: 10.3329/bjas.v42i1.15783
4. Pragna, P, Sejian, V, Bagath, M, Krishnan, G, Archana, PR, Soren, NM, et al. Comparative assessment of growth performance of three different indigenous goat breeds exposed to summer heat stress. J Anim Physiol Anim Nutr. (2018) 102:825–36. doi: 10.1111/jpn.12892
5. Salama, AAK, Caja, G, Hamzaoui, S, Badaoui, B, Castro-Costa, A, Facanha, DAE, et al. Different levels of response to heat stress in dairy goats. Small Rumin Res. (2014) 121:73–9. doi: 10.1016/j.smallrumres.2013.11.021
6. St-Pierre, NR, Cobanov, B, and Schnitkey, G. Economic losses from heat stress by us livestock industries. J Dairy Sci. (2003) 86:E52–77. doi: 10.3168/jds.S0022-0302(03)74040-5
7. Lima, ARC, Silveira, RMF, Castro, MSM, De Vecchi, LB, Fernandes, MHMR, and Resende, KT. Relationship between thermal environment, thermoregulatory responses and energy metabolism in goats: a comprehensive review. J Therm Biol. (2022) 109:103324. doi: 10.1016/j.jtherbio.2022.103324
8. Abdelnour, SA, Abd El-Hack, ME, Khafaga, AF, Arif, M, Taha, AE, and Noreldin, AE. Stress biomarkers and proteomics alteration to thermal stress in ruminants: a review. J Therm Biol. (2019) 79:120–34. doi: 10.1016/j.jtherbio.2018.12.013
9. Saunders, PU, Watt, MJ, Garnham, AP, Spriet, LL, Hargreaves, M, and Febbraio, MA. No effect of mild heat stress on the regulation of carbohydrate metabolism at the onset of exercise. J Appl Physiol. (2001) 91:2282–8. doi: 10.1152/jappl.2001.91.5.2282
10. Silanikove, N, and Koluman, N. Impact of climate change on the dairy industry in temperate zones: Predications on the overall negative impact and on the positive role of dairy goats in adaptation to earth warming. Small Rumin Res. (2015) 123:27–34. doi: 10.1016/j.smallrumres.2014.11.005
11. Sejian, V, and Srivastava, RS. Effects of melatonin on adrenal cortical functions of indian goats under thermal stress. Vet Med Int. (2010) 2010:348919. doi: 10.4061/2010/348919
12. Al-Dawood, A. Effect of heat stress on adipokines and some blood metabolites in goats from jordan. Anim Sci J. (2017) 88:356–63. doi: 10.1111/asj.12636
13. Hu, L, Ma, Y, Liu, L, Kang, L, Brito, LF, Wang, D, et al. Detection of functional polymorphisms in the hsp70 gene and association with cold stress response in inner-mongolia sanhe cattle. Cell Stress Chaperones. (2019) 24:409–18. doi: 10.1007/s12192-019-00973-5
14. Macias-Cruz, U, Lopez-Baca, MA, Vicente, R, Mejia, A, Alvarez, FD, Correa-Calderon, A, et al. Effects of seasonal ambient heat stress (spring vs. Summer) on physiological and metabolic variables in hair sheep located in an arid region. Int J Biometeorol. (2016) 60:1279–86. doi: 10.1007/s00484-015-1123-6
15. Mellado, M, and Meza-Herrera, CA. Influence of season and environment on fertility of goats in a hot-arid environment. J Agric Sci. (2002) 138:97–102. doi: 10.1017/s0021859601001630
16. Amitha, JP, Krishnan, G, Bagath, M, Sejian, V, and Bhatta, R. Heat stress impact on the expression patterns of different reproduction related genes in malabari goats. Theriogenology. (2019) 131:169–76. doi: 10.1016/j.theriogenology.2019.03.036
17. Giorgino, A, Raspa, F, Valle, E, Bergero, D, Cavallini, D, Gariglio, M, et al. Effect of dietary organic acids and botanicals on metabolic status and milk parameters in mid–late lactating goats. Animals. (2023) 13:797. doi: 10.3390/ani13050797
18. Hamzaoui, S, Salama, AA, Albanell, E, Such, X, and Caja, G. Physiological responses and lactational performances of late-lactation dairy goats under heat stress conditions. J Dairy Sci. (2013) 96:6355–65. doi: 10.3168/jds.2013-6665
19. Tucker, CB, Rogers, AR, and Schutz, KE. Effect of solar radiation on dairy cattle behaviour, use of shade and body temperature in a pasture-based system. Appl Anim Behav Sci. (2008) 109:141–54. doi: 10.1016/j.applanim.2007.03.015
20. AOAC. Gaithersburg, md: Association of official analytical chemists international. 18th ed. Arlington, Virginia, USA: Association of Official Analytical Chemists (2006).
21. Vansoest, PJ, Robertson, JB, and Lewis, BA. Methods for dietary fiber, neutral detergent fiber, and nonstarch polysaccharides in relation to animal nutrition. J Dairy Sci. (1991) 74:3583–97. doi: 10.3168/jds.S0022-0302(91)78551-2
22. Guo, Y, Guo, H, Qiu, L, Fu, Y, Zhu, X, Zhang, H, et al. Appetite suppression and interleukin 17 receptor signaling activation of colonic mycobiota dysbiosis induced by high temperature and high humidity conditions. Front Cell Infect Microbiol. (2021) 11:657807. doi: 10.3389/fcimb.2021.657807
23. Hoyda, TD, Samson, WK, and Ferguson, AV. Central nervous system roles for adiponectin in neuroendocrine and autonomic function In: Preedy VR and Hunter RJ, editors. Adipokines. USA: Crc Press-Taylor & Francis Group (2011). 167–84.
24. Morera, P, Basirico, L, Hosoda, K, and Bernabucci, U. Chronic heat stress up-regulates leptin and adiponectin secretion and expression and improves leptin, adiponectin and insulin sensitivity in mice. J Mol Endocrinol. (2012) 48:129–38. doi: 10.1530/jme-11-0054
25. Rabe, K, Lehrke, M, Parhofer, KG, and Broedl, UC. Adipokines and insulin resistance. Mol Med. (2008) 14:741–51. doi: 10.2119/2008-00058.Rabe
26. Yang, L, Zhang, L, Zhang, P, Zhou, Y, Huang, X, Yan, Q, et al. Alterations in nutrient digestibility and performance of heat-stressed dairy cows by dietary l-theanine supplementation. Anim Nutr. (2022) 11:350–8. doi: 10.1016/j.aninu.2022.08.002
27. Bernabucci, U, Lacetera, N, Danieli, PP, Bani, P, Nardone, A, and Ronchi, B. Influence of different periods of exposure to hot environment on rumen function and diet digestibility in sheep. Int J Biometeorol. (2009) 53:387–95. doi: 10.1007/s00484-009-0223-6
28. Wheelock, JB, Rhoads, RP, Vanbaale, MJ, Sanders, SR, and Baumgard, LH. Effects of heat stress on energetic metabolism in lactating holstein cows. J Dairy Sci. (2010) 93:644–55. doi: 10.3168/jds.2009-2295
29. Gonzalez-Rivas, PA, Chauhan, SS, Ha, M, Fegan, N, Dunshea, FR, and Warner, RD. Effects of heat stress on animal physiology, metabolism, and meat quality: A review. Meat Sci. (2020) 162:108025. doi: 10.1016/j.meatsci.2019.108025
30. Costa, WP, Façanha, DAE, Leite, JHGM, Silva, RCB, Souza, CH, Chaves, DF, et al. Thermoregulatory responses and blood parameters of locally adapted ewes under natural weather conditions of brazilian semiarid region. Semina: Ciências Agrárias. (2015) 36:4589–4600. doi: 10.5433/1679-0359.2015v36n6Supl2p4589
31. Shilja, S, Sejian, V, Bagath, M, Mech, A, David, CG, Kurien, EK, et al. Adaptive capability as indicated by behavioral and physiological responses, plasma hsp70 level, and pbmc hsp70 mrna expression in osmanabadi goats subjected to combined (heat and nutritional) stressors. Int J Biometeorol. (2015) 60:1311–23. doi: 10.1007/s00484-015-1124-5
32. Marai, IFM, El-Darawany, AA, Fadiel, A, and Abdel-Hafez, MAM. Physiological traits as affected by heat stress in sheep—a review. Small Rumin Res. (2007) 71:1–12. doi: 10.1016/j.smallrumres.2006.10.003
33. Banerjee, D, Upadhyay, RC, Chaudhary, UB, Kumar, R, and Singh, S. Seasonal variations in physio-biochemical profiles of indian goats in the paradigm of hot and cold climate. Biol Rhythm Res. (2014) 46:221–36. doi: 10.1080/09291016.2014.984999
34. Atkinson, HC, Wood, SA, Kershaw, YM, Bate, E, and Lightman, SL. Diurnal variation in the responsiveness of the hypothalamic-pituitary-adrenal axis of the male rat to noise stress. J Neuroendocrinol. (2006) 18:526–33. doi: 10.1111/j.1365-2826.2006.01444.x
35. Sharma, S, Ramesh, K, Hyder, I, Uniyal, S, Yadav, VP, Panda, RP, et al. Effect of melatonin administration on thyroid hormones, cortisol and expression profile of heat shock proteins in goats (capra hircus) exposed to heat stress. Small Rumin Res. (2013) 112:216–23. doi: 10.1016/j.smallrumres.2012.12.008
36. Ronchi, B, Stradaioli, G, Verini-Supplizi, A, Bernabucci, U, Lacetera, N, Accorsi, PA, et al. Influence of heat stress or feed restriction on plasma progesterone, oestradiol-17 beta, lh, fsh, prolactin and cortisol in holstein heifers. Livest Prod Sci. (2001) 68:231–41. doi: 10.1016/s0301-6226(00)00232-3
37. Sejian, V, Bhatta, R, Gaughan, JB, Dunshea, FR, and Lacetera, N. Review: Adaptation of animals to heat stress. Animal. (2018) 12:s431–44. doi: 10.1017/S1751731118001945
38. Silanikove, N. Effects of heat stress on the welfare of extensively managed domestic ruminants. (2000), 67, 1–18.
39. Przemyslaw, C, Roman, K, and Czerski, A. Effect of heat stress on physiological parameters and blood composition in polish merino rams. Berl Munch Tierarztl Wochenschr. (2014) 127:177. doi: 10.2376/0005-9366-127-177
40. Mayorga, EJ, Ross, JW, Keating, AF, Rhoads, RP, and Baumgard, LH. Biology of heat stress; the nexus between intestinal hyperpermeability and swine reproduction. Theriogenology. (2020) 154:73–83. doi: 10.1016/j.theriogenology.2020.05.023
41. Rhoads, ML, Rhoads, RP, VanBaale, MJ, Collier, RJ, Sanders, SR, Weber, WJ, et al. Effects of heat stress and plane of nutrition on lactating holstein cows: I. Production, metabolism, and aspects of circulating somatotropin. J Dairy Sci. (2009) 92:1986–97. doi: 10.3168/jds.2008-1641
42. Shehab-El-Deen, M, Fadel, MS, Van Soom, A, Saleh, SY, Maes, D, and Leroy, J. Circadian rhythm of metabolic changes associated with summer heat stress in high-producing dairy cattle. Trop Anim Health Prod. (2010) 42:1119–25. doi: 10.1007/s11250-010-9534-1
43. Settivari, RS, Spain, JN, and Ellersieck, MR. Relationship of thermal status to productivity in heat-stressed dairy cows given recombinant bovine somatotropin. J Dairy Sci. (2007) 90:1265–80. doi: 10.3168/jds.S0022-0302(07)71615-6
44. Ribeiro, NL, Germano Costa, R, Pimenta Filho, EC, Ribeiro, MN, and Bozzi, R. Effects of the dry and the rainy season on endocrine and physiologic profiles of goats in the brazilian semi-arid region. Ital J Anim Sci. (2017) 17:454–61. doi: 10.1080/1828051x.2017.1393320
45. Yazdi, M, Mirzaei-Alamouti, H, and Amanlou, H. Effects of heat stress on metabolism, digestibility, and rumen epithelial characteristics in growing holstein calves. J Anim Sci. (2016) 94:77–89. doi: 10.2527/jas2015-9364
46. Epel, ES, McEwen, B, Seeman, T, Matthews, K, Castellazzo, G, Brownell, KD, et al. Stress and body shape: Stress-induced cortisol secretion is consistently greater among women with central fat. Psychosom Med. (2000) 62:623–32. doi: 10.1097/00006842-200009000-00005
47. Kuo, T, McQueen, A, Chen, TC, and Wang, JC. Regulation of glucose homeostasis by glucocorticoids. Adv Exp Med Biol. (2015) 872:99–126. doi: 10.1007/978-1-4939-2895-8_5
48. Belhadj Slimen, I, Najar, T, Ghram, A, and Abdrrabba, M. Heat stress effects on livestock: Molecular, cellular and metabolic aspects, a review. J Anim Physiol Anim Nutr (Berl). (2016) 100:401–12. doi: 10.1111/jpn.12379
49. Chauhan, SS, Rashamol, VP, Bagath, M, Sejian, V, and Dunshea, FR. Impacts of heat stress on immune responses and oxidative stress in farm animals and nutritional strategies for amelioration. Int J Biometeorol. (2021) 65:1231–44. doi: 10.1007/s00484-021-02083-3
50. Dodd, SL, Gagnon, BJ, Senf, SM, Hain, BA, and Judge, AR. Ros-mediated activation of nf-kappa b and foxo during muscle disuse. Muscle Nerve. (2010) 41:110–3. doi: 10.1002/mus.21526
51. Finocchiaro, R, van Kaam, JBCHM, Sardina, MT, and Misztal, I. Effect of heat stress on production in mediterranean dairy sheep. Ital J Anim Sci. (2005) 4:70–2. doi: 10.4081/ijas.2005.2s.70
52. Lamp, O, Derno, M, Otten, W, Mielenz, M, Nurnberg, G, and Kuhla, B. Metabolic heat stress adaption in transition cows: Differences in macronutrient oxidation between late-gestating and early-lactating german holstein dairy cows. PLoS One. (2015) 10:24. doi: 10.1371/journal.pone.0125264
53. Guo, J, Gao, ST, Quan, SY, Zhang, YD, Bu, DP, and Wang, JQ. Blood amino acids profile responding to heat stress in dairy cows. Asian Australas J Anim Sci. (2018) 31:47–53. doi: 10.5713/ajas.16.0428
54. Cowley, FC, Barber, DG, Houlihan, AV, and Poppi, DP. Immediate and residual effects of heat stress and restricted intake on milk protein and casein composition and energy metabolism. J Dairy Sci. (2015) 98:2356–68. doi: 10.3168/jds.2014-8442
55. Cruzen, SM, Pearce, SC, Baumgard, LH, Gabler, NK, Huff-Lonergan, E, and Lonergan, SM. Proteomic changes to the sarcoplasmic fraction of predominantly red or white muscle following acute heat stress. J Proteome. (2015) 128:141–53. doi: 10.1016/j.jprot.2015.07.032
56. Meijer, AJ. Amino acids as regulators and components of nonproteinogenic pathways. J Nutr. (2003) 133:2057S–62S. doi: 10.1093/jn/133.6.2057S
57. Chen, Y, Liu, Y, Bai, YC, Xu, SG, Yang, XF, and Cheng, B. Intestinal metabolomics of juvenile lenok (brachymystax lenok) in response to heat stress. Fish Physiol Biochem. (2022) 48:1389–400. doi: 10.1007/s10695-022-01128-7
58. Lu, QZ, Zhang, YF, and Elisseeff, JH. Carnitine and acetylcarnitine modulate mesenchymal differentiation of adult stem cells. J Tissue Eng Regen Med. (2015) 9:1352–62. doi: 10.1002/term.1747
59. Li, JL, Wang, QY, Luan, HY, Kang, ZC, and Wang, CB. Effects of l-carnitine against oxidative stress in human hepatocytes: Involvement of peroxisome proliferator-activated receptor alpha. J Biomed Sci. (2012) 19:9. doi: 10.1186/1423-0127-19-32
60. Fukuzawa, K. Dynamics of lipid peroxidation and antioxidion of alpha-tocopherol in membranes. J Nutr Sci Vitaminol. (2008) 54:273–85. doi: 10.3177/jnsv.54.273
61. Escobar, J, Frank, JW, Suryawan, A, Nguyen, HV, Kimball, SR, Jefferson, LS, et al. Physiological rise in plasma leucine stimulates muscle protein synthesis in neonatal pigs by enhancing translation initiation factor activation. Am J Physiol-Endocrinol Metab. (2005) 288:E914–21. doi: 10.1152/ajpendo.00510.2004
62. Escobar, J, Frank, JW, Suryawan, A, Nguyen, HV, Kimball, SR, Jefferson, LS, et al. Regulation of cardiac and skeletal muscle protein synthesis by individual branched-chain amino acids in neonatal pigs. Am J Physiol-Endocrinol Metab. (2006) 290:E612–21. doi: 10.1152/ajpendo.00402.2005
63. Kozlov, AV, Duvigneau, JC, Hyatt, TC, Raju, R, Behling, T, Hartl, RT, et al. Effect of estrogen on mitochondrial function and intracellular stress markers in rat liver and kidney following trauma-hemorrhagic shock and prolonged hypotension. Mol Med. (2010) 16:254–61. doi: 10.2119/molmed.2009.00184
64. Marques, AT, Lecchi, C, Grilli, G, Giudice, C, Nodari, SR, Vinco, LJ, et al. The effect of transport stress on turkey (meleagris gallopavo) liver acute phase proteins gene expression. Res Vet Sci. (2016) 104:92–5. doi: 10.1016/j.rvsc.2015.11.014
65. Kamiya, M, Kamiya, Y, Tanaka, M, Oki, T, Nishiba, Y, and Shioya, S. Effects of high ambient temperature and restricted feed intake on urinary and plasma 3-methylhistidine in lactating holstein cows. Anim Sci J. (2006) 77:201–7. doi: 10.1111/j.1740-0929.2006.00338.x
66. Wilson, TE. Renal sympathetic nerve, blood flow, and epithelial transport responses to thermal stress. Auton Neurosci-Basic Clin. (2017) 204:25–34. doi: 10.1016/j.autneu.2016.12.007
67. Silanikove, N. Effects of water scarcity and hot environment on appetite and digestion in ruminants—a review. Livest Prod Sci. (1992) 30:175–94. doi: 10.1016/S0301-6226(06)80009-6
68. Silanikove, N. The struggle to maintain hydration and osmoregulation in animals experiencing severe dehydration and rapid rehydration—the story of ruminants. Exp Physiol. (1994) 79:281–300. doi: 10.1113/expphysiol.1994.sp003764
69. O'Brien, MD, Rhoads, RP, Sanders, SR, Duff, GC, and Baumgard, LH. Metabolic adaptations to heat stress in growing cattle. Domest Anim Endocrinol. (2010) 38:86–94. doi: 10.1016/j.domaniend.2009.08.005
70. Levillain, O, Parvy, P, and Hassler, C. Amino acid handling in uremic rats: Citrulline, a reliable marker of renal insufficiency and proximal tubular dysfunction. Metab-Clin Exp. (1997) 46:611–8. doi: 10.1016/s0026-0495(97)90002-0
71. Mukhopadhyay, TK, and Trauner, D. Concise synthesis of glycerophospholipids. J Organomet Chem. (2022) 88:11253–7. doi: 10.1021/acs.joc.2c02096
72. Braverman, NE, and Moser, AB. Functions of plasmalogen lipids in health and disease. Biochim Biophys Acta-Mol Basis Dis. (2012) 1822:1442–52. doi: 10.1016/j.bbadis.2012.05.008
73. Melvin, SD, Lanctot, CM, Doriean, NJC, Bennett, W, and Carroll, AR. Nmr-based lipidomics of fish from a metal(loid) contaminated wetland show differences consistent with effects on cellular membranes and energy storage. Sci Total Environ. (2019) 654:284–91. doi: 10.1016/j.scitotenv.2018.11.113
74. Dave, N, Judd, JM, Decker, A, Winslow, W, Sarette, P, Espinosa, OV, et al. Dietary choline intake is necessary to prevent systems-wide organ pathology and reduce alzheimer's disease hallmarks. Aging Cell. (2023) 22:e13775. doi: 10.1111/acel.13775
75. Craig, SAS. Betaine in human nutrition. Am J Clin Nutr. (2004) 80:539–49. doi: 10.1093/ajcn/80.3.539
76. Yang, M, Kuang, MQ, Wang, GL, Ali, I, Tang, YJ, Yang, CX, et al. Choline attenuates heat stress-induced oxidative injury and apoptosis in bovine mammary epithelial cells by modulating perk/nrf-2 signaling pathway. Mol Immunol. (2021) 135:388–97. doi: 10.1016/j.molimm.2021.05.002
77. Dangi, SS, Dangi, SK, Chouhan, VS, Verma, MR, Kumar, P, Singh, G, et al. Modulatory effect of betaine on expression dynamics of hsps during heat stress acclimation in goat (capra hircus). Gene. (2016) 575:543–50. doi: 10.1016/j.gene.2015.09.031
78. Diamant, S, Eliahu, N, Rosenthal, D, and Goloubinoff, P. Chemical chaperones regulate molecular chaperones in vitro and in cells under combined salt and heat stresses. J Biol Chem. (2001) 276:39586–91. doi: 10.1074/jbc.M103081200
79. Mehta, AK, Gaur, SN, Arora, N, and Singh, BP. Effect of choline chloride in allergen-induced mouse model of airway inflammation. Eur Respir J. (2007) 30:662–71. doi: 10.1183/09031936.00019307
80. Zhou, Z, Trevisi, E, Luchini, DN, and Loor, JJ. Differences in liver functionality indexes in peripartal dairy cows fed rumen-protected methionine or choline are associated with performance, oxidative stress status, and plasma amino acid profiles. J Dairy Sci. (2017) 100:6720–32. doi: 10.3168/jds.2016-12299
Keywords: heat stress, Dazu black goat, physiological indicators, hormonal, amino acids
Citation: Wang L, Zhang P, Du Y, Wang C, Zhang L, Yin L, Zuo F and Huang W (2024) Effect of heat stress on blood biochemistry and energy metabolite of the Dazu black goats. Front. Vet. Sci. 11:1338643. doi: 10.3389/fvets.2024.1338643
Edited by:
Rui Hu, Sichuan Agricultural University, ChinaReviewed by:
Jian Ma, Guangdong Ocean University, ChinaXianwen Dong, Chongqing Academy of Animal Science, China
Copyright © 2024 Wang, Zhang, Du, Wang, Zhang, Yin, Zuo and Huang. This is an open-access article distributed under the terms of the Creative Commons Attribution License (CC BY). The use, distribution or reproduction in other forums is permitted, provided the original author(s) and the copyright owner(s) are credited and that the original publication in this journal is cited, in accordance with accepted academic practice. No use, distribution or reproduction is permitted which does not comply with these terms.
*Correspondence: Wenming Huang, hwmyy@126.com; Fuyuan Zuo, zfuyuan@163.com