- Fujian Key Laboratory of Animal Genetics and Breeding, Institute of Animal Husbandry and Veterinary Medicine, Fujian Academy of Agricultural Sciences, Fuzhou, China
Gonadotropin-inhibitory hormone (GnIH) is a neurohormone that not only suppresses reproduction at the brain level but also regulates steroidogenesis and gametogenesis at the gonad level. However, its function in gonadal physiology has received little attention in rabbits. The main objective of this study was to evaluate the effects of GnIH on testicular development and function in prepubertal Minxinan Black rabbits (Oryctolagus cuniculus). In the present study, we investigated the serum reproductive hormone concentration, testicular parameters, morphology of seminiferous tubules, apoptosis of testicular cells, and expression of reproductive-related genes in male prepubertal Minxinan Black rabbits intraperitoneally administered with 0, 0.5, 5, or 50 μg quail GnIH-related peptides (qGnIH) for 10 days. Compared with the vehicle, administration with 5 μg of qGnIH downregulated the serum testosterone concentration and mRNA levels of spermatogenic genes (PCNA, FSHR, INHβA, HSF1, and AR) and upregulated the apoptosis rate of testicular cells; administration with 50 μg of qGnIH decreased the serum testosterone concentration and hypothalamic GnIH gene mRNA level and increased the serum LH concentration, pituitary LHβ gene mRNA level, testicular weight, gonadosomatic index (GSI), and spermatogenic cell layer thickness. It is concluded that GnIH could exert dual actions on testicular development depending on the male prepubertal rabbits receiving different intraperitoneal doses.
1 Introduction
Gonadotropin-inhibiting hormone (GnIH) is a hypothalamic neuropeptide with a C-terminal LPXRFamide (X = L or Q) motif that was originally isolated from the brain of Japanese quail as an inhibitor of gonadotropin-releasing hormone (GtH) (1). An abundance of studies have shown that peptides orthologous to quail GnIH shared identical C-terminal sequences act to control GtH release across vertebrates and are also named GnIH (2–5). Central administration of GnIH reduced gonadotrophin release hormone (GnRH)-stimulated luteinizing hormone β subunit (LHβ) or follicle-stimulating hormone β subunit (FSHβ) expression in the pituitary gland and decreased LH and FSH release into the hypophyseal portal systems in sea bass (6), ducks (7), Siberian hamsters (8, 9), and rats (10). Although most studies have shown an inhibitory effect of GnIH on the vertebrate HPG axis, several in vivo and in vitro studies have shown a stimulatory effect depending on the species, reproductive stages, higher doses or longer duration of GnIH administration (3, 8, 11, 12). For instance, the stimulatory effects of GnIH on GtH synthesis and secretion were reported in European eel (13), Catla catla (14), male chub mackerel (15), yellowtail kingfish (12), and hamster (8). Thus, these studies have demonstrated that GnIHs are highly conserved in the regulation of the hypothalamic–pituitary-gonadal (HPG) axis.
GnIH has also been reported to express in gonads and act directly on the gonads mediated by its receptor: G protein-coupled receptor 147 (GPR147) in vertebrates (1, 11, 16). Treatment with GnIH significantly decreased the number of G0/G1 cells and proliferative activity of type A spermatogonia. It inhibited human chorionic gonadotropin (hCG) and FSH-induced spermatogenesis in the ex-vivo culture of zebrafish testis (11). The injection of GnIH induced testicular apoptosis, decreased spermatogenic activity and suppressed normal testicular growth in quails (1). Furthermore, GnIH also decreased testicular activity in mice (16), mediated epididymal apoptosis and autophagy in rats (17), and inhibited granulosa cell (GC) proliferation in pigs (18). In vivo and in vitro studies have shown that GnIH is capable of influencing gonadal development and function.
In prepubertal Minxinan Black rabbits, the testis remains morphologically silent until the animal approaches puberty (approximately 90 days old). Administration of exogenous GnIH might influence pubertal onset and development, resulting in advanced or delayed puberty in rabbits. However, the effects of chronic infusion of GnIH on testicular development and function have never been evaluated in the male prepubertal rabbits. Therefore, in this study, we first examined the effects of GnIH on serum reproductive hormone concentrations and testicular parameters, then evaluated its effects on the morphology of seminiferous tubules and apoptosis rate of testicular cells, and finally detected its effect on the expression of reproductive-related genes in Minxinan Black rabbits aged 90 days.
2 Methods
2.1 Animals and drugs
In this study, quail GnIH-related peptide 1 (qGnIH) was purchased from Phoenix Pharmaceuticals (catalog No. 040–52, United States), stored at −80°C and dissolved in the vehicle for administration.
Eighty-day-aged Minxinan Black rabbits (n = 48 total) weighing 1,500 ± 100 g were used in this study. Based on the previous study (19), three different doses of qGnIH (0.5 μg, 5 μg, 50 μg; n = 12 per group) were dissolved in normal saline solution as different treatment groups. Rabbits in the control group (n = 12) received vehicle only. Rabbits received intraperitoneal injections of GnIH daily for 10 days. The care and use of rabbits fully complied with local animal welfare laws, guidelines, and policies. The rabbits were allowed free access to food and water at all times and maintained on a 12 h L/12 h D (light/dark) cycle and in an air-conditioned room.
2.2 Elisa
Blood samples were collected from the ear vein 24 h after the last administration. After centrifugation (1,000 g, 15 min) of the blood samples, the obtained serum samples were stored at −80°C until further analysis. Serum concentrations of GnRH, FSH, LH and testosterone were measured using ELISA kits following the manufacturer’s instructions (Shanghai Jining Industrial, China).
2.3 Measurements and tissue sampling
Rabbits were weighed (live weight defined as LW, kg) prior to sacrifice 24 h after the last administration. The testes were dissected out of the scrotum and the epididymides were removed. Testes were weighed (testicular weight defined as TW, g) and testicular length (defined as L, cm) and width (defined as W, cm) were also measured. Testicular volume (defined as TV, cm3) was calculated as TV = 4/3π × (1/2 L) × (1/2 W)2 (20) and gonadosomatic index (defined as GSI, g kg−1) was calculated as GSI = TW/LW (21, 22). Samples of the hypothalamus, pituitary gland, and left testis were collected and stored in liquid nitrogen until RNA extraction. The right testis was stored in 4% paraformaldehyde for morphometric analysis and TUNEL assay. At the time of tissue collection, none of the rabbits showed signs of disease.
2.4 qPCR
Total RNA was isolated from the hypothalamus, pituitary gland, and testis with Trizol™ Reagent (Invitrogen, United States). cDNAs were synthesized using Oligo (dT)18 and M-MLV reverse transcriptase (Promega, United States) according to the manufacturer’s instructions. qPCR was performed in triplicate using SYBR Green Master Mix (Applied Biosystems, United States) on a CFX384 Real-time PCR System (Bio-Rad, United States). Glyceraldehyde-3-phosphate dehydrogenase (GAPDH) was used as an internal control to normalize the data. The qPCR primers are presented in Table 1.
2.5 Morphometric analysis
After fixation in 4% paraformaldehyde, testicular tissues were dehydrated with graded 75, 85, 95, and 100% alcohol, infiltrated in xylene, embedded in paraffin blocks, sectioned into 4 μm-thick sections, mounted onto slides, and stained with hematoxylin and eosin (HE). The section images were acquired on a Pannoramic MIDI scanner (3DHISTECH, Hungary). Perimeters and cross-sectional areas of seminiferous tubules (μm and μm2) were measured using the liner measurement annotation of Caseviewer (3DHISTECH, Hungary). Thicknesses of spermatogenic cell layers (μm) were measured using liner measurement annotation of Caseviewer (3DHISTECH, Hungary). The thicknesses of each seminiferous tubule were measured 4 times and then averaged. On average, 40–50 tubules were analysed per rabbit.
2.6 TUNEL assay
The paraffin-embedded sections (4 μm) were dewaxed with xylene, hydrated using an ethanol gradient, and subsequently subjected to incubation with proteinase K. After performing a TUNEL assay kit containing TdT (Roche Diagnostics, Switzerland) to detect apoptosis-positive cells in rabbit testes, the sections were counterstained with DAPI and mounted onto slides. Fluorescent images were acquired on a Pannoramic MIDI scanner (3DHISTECH, Hungary) using two channels, including DAPI (blue) and TUNEL (green). Cell apoptosis analysis was performed by counting all cells with blue fluorescence (DAPI) and apoptotic cells with green fluorescence (TUNEL) of seminiferous tubules in the sections. The apoptotic rate was calculated as the ratio of the number of apoptotic cells to the total cell count in the testis. AIPATHWELL software (Servicebio, China) for automatic quantification of fluorescent markers was utilized to count the total and apoptotic cells of seminiferous tubules and calculate the apoptosis rate.
2.7 Statistical analysis
All data are presented as mean ± SEM. Statistical analysis was performed using one-way ANOVA using SPSS Statistics 17.0 (SPSS Inc., United States). Comparisons between the groups were made by analysing data with the least significant difference (LSD) method. p-values of <0.05 were considered to be statistically significant.
3 Results
3.1 Effects of qGnIH on hormone parameters
The serum concentrations of the reproductive hormones are shown in Table 2. The serum LH level under the high qGnIH-injected concentration (50 μg) was significantly higher than that of the vehicle (p < 0.05). Serum testosterone levels under the medium and high qGnIH-injected concentrations (5 and 50 μg) were both significantly lower compared to the vehicle (p < 0.05).
3.2 Effects of qGnIH on testicular parameters
To investigate whether qGnIH affects testicular development in male prepubertal rabbits, we measured and calculated the testicular parameters. The TW and GSI were increased by the infusion of 50 μg of qGnIH (p < 0.05), while the TV was not changed (p > 0.05). The results are shown in Figure 1.
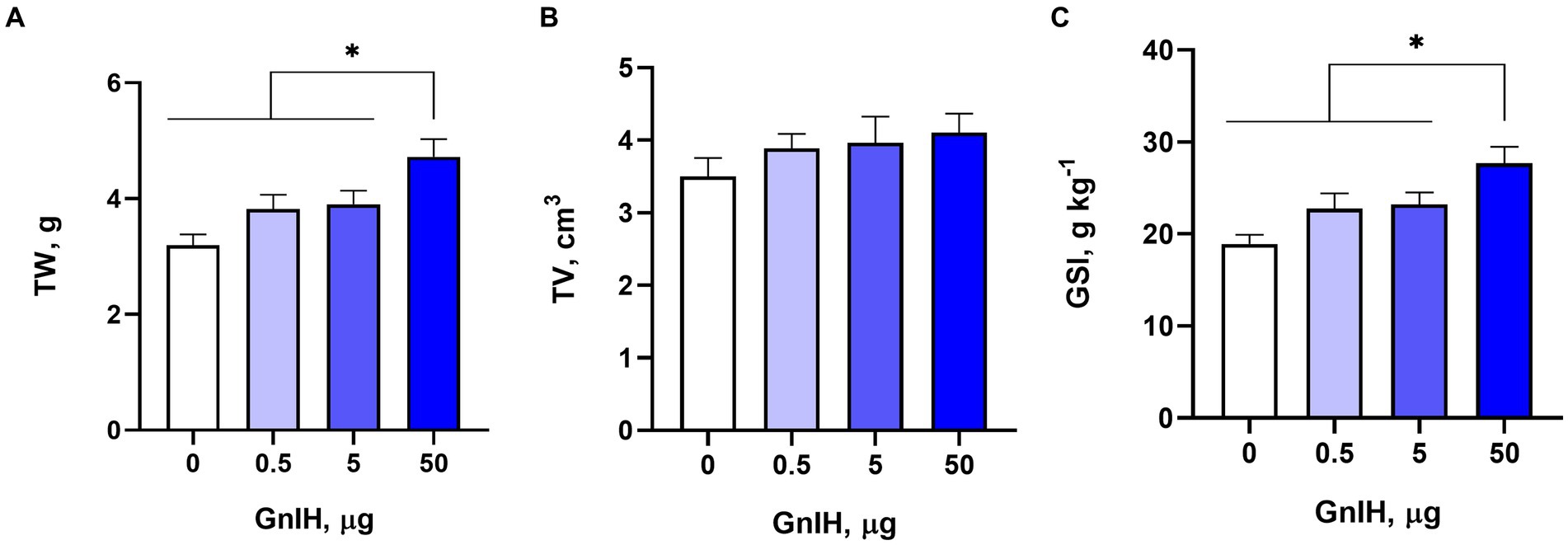
Figure 1. Effects of different injected doses of qGnIH on testicular parameters. (A) Effect on testicular weight (TW). (B) Effect on testicular volume (TV). (C) Effect on gonadosomatic index (GSI). Asterisks indicate significant differences * p < 0.05.
3.3 Effects of qGnIH on the morphology of seminiferous tubules
The intraperitoneal infusion of qGnIH into prepubertal rabbits had no effect on the perimeters or cross-sectional areas of seminiferous tubules (p > 0.05). However, there was a positive effect of 50 μg of qGnIH on the thickness of the spermatogenic cell layer (p < 0.01). The results are shown in Figure 2.
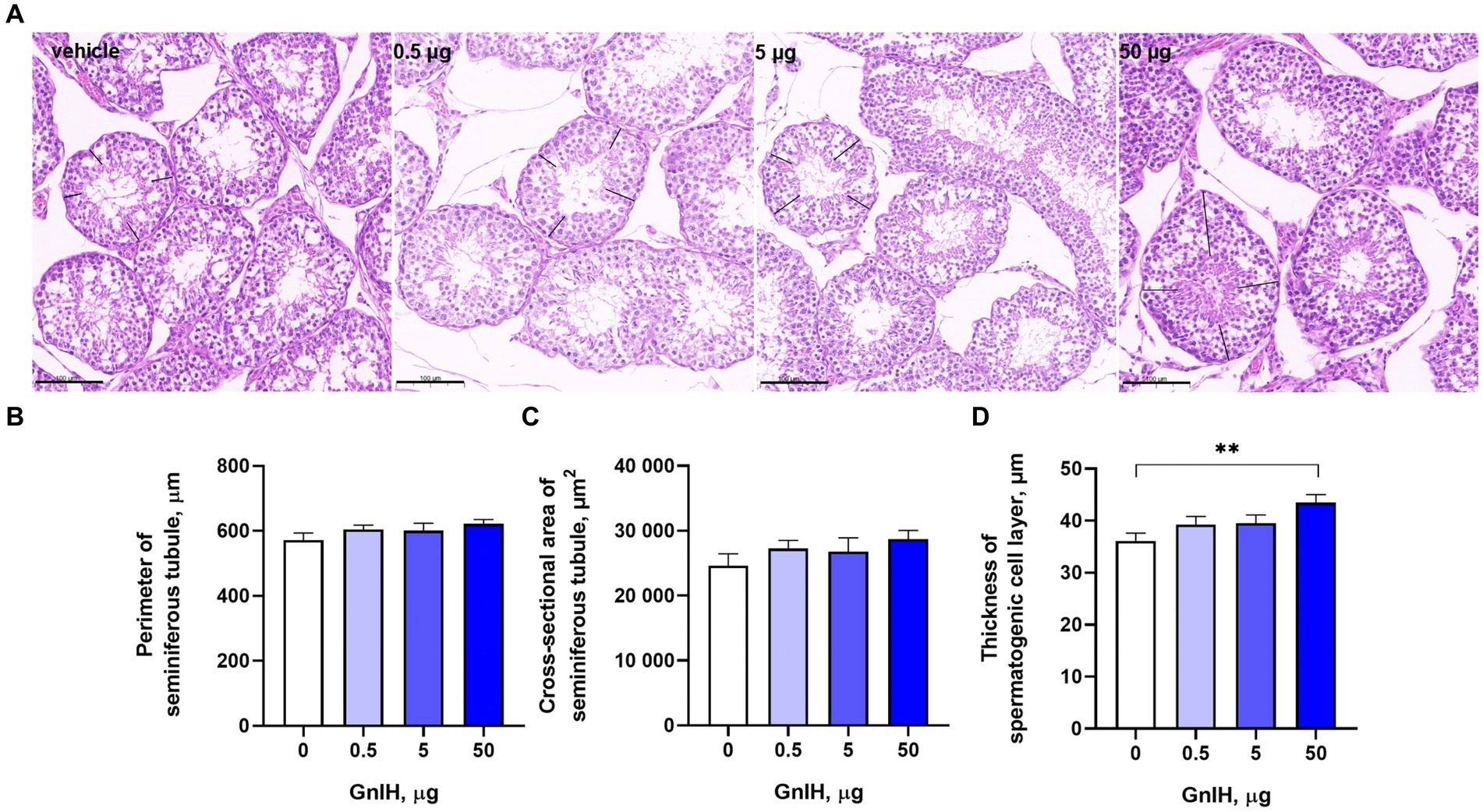
Figure 2. Effects of different injected doses of qGnIH on the morphology of Minxinan Black rabbit seminiferous tubules. (A) HE-stained seminiferous tubules (the spermatogenic cell layer thickness marked with the black line segment). Scale bar = 100 μm. (B) The perimeter of the seminiferous tubule meaured with the closed polygan annotation of Caseview soft (3DHISTECH, Hungary). (C) The cross-sectional area of the seminiferous tubule measured with the closed polygan annotation of Caseview soft (3DHISTECH, Hungary). (D) The spermatogenic cell layer thickness of seminiferous tubule measured with the liner measurement annotation of Caseview soft (3DHISTECH, Hungary). Asterisks indicate significant differences ** p < 0.01.
3.4 Effects of qGnIH on the apoptosis of testicular cells
The apoptosis rates of testicular cells were further detected by TUNEL assay. We observed that infusion of qGnIH at 5 μg increased the apoptosis rate of testicular cells, and neither infusion of GnIH at 0.5 or 50 μg affected the apoptosis rate of testicular cells (p > 0.05). Representative images of the TUNEL assay of the apoptosis rate are shown in Figure 3.
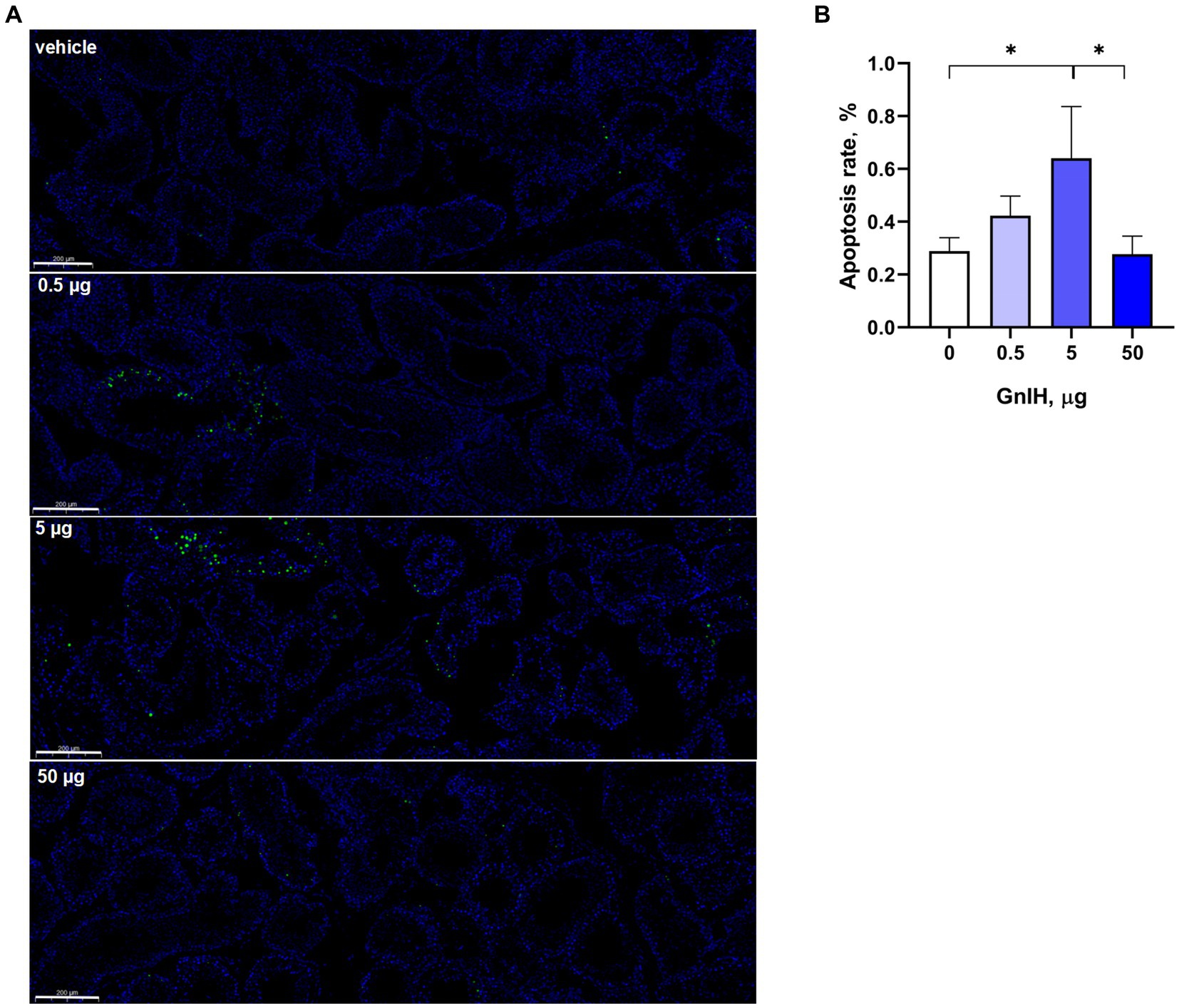
Figure 3. Effects of different injected doses of qGnIH on the apoptosis of Minxinan Black rabbit spermatogenic cells. (A) Testicular seminiferous tubules stained with TUNEL and DAPI, green fluorescence represents apoptotic cells (TUNEL staining), and blue fluorescence represents all cells in seminiferous tubules (DAPI staining). Scale bar = 200 μm. (B) Apoptotic rate of testicular cells. Asterisks indicate significant differences * p < 0.05.
3.5 Effects of qGnIH on the genes expressed in the HPG axis
3.5.1 Effects of qGnIH on the genes expressed in the hypothalamus
As shown in Figure 4A, the mRNA level of GPR147 was significantly increased (p < 0.05) and the mRNA level of GnIH gene was significantly decreased (p < 0.05) in the hypothalamus at a dose of 50 μg of qGnIH. The mRNA levels of GnRH, GnRH receptor (GnRHR), and estrogen receptor 1 (ESR1) in the hypothalamus were not changed after the injection of qGnIH (p > 0.05).
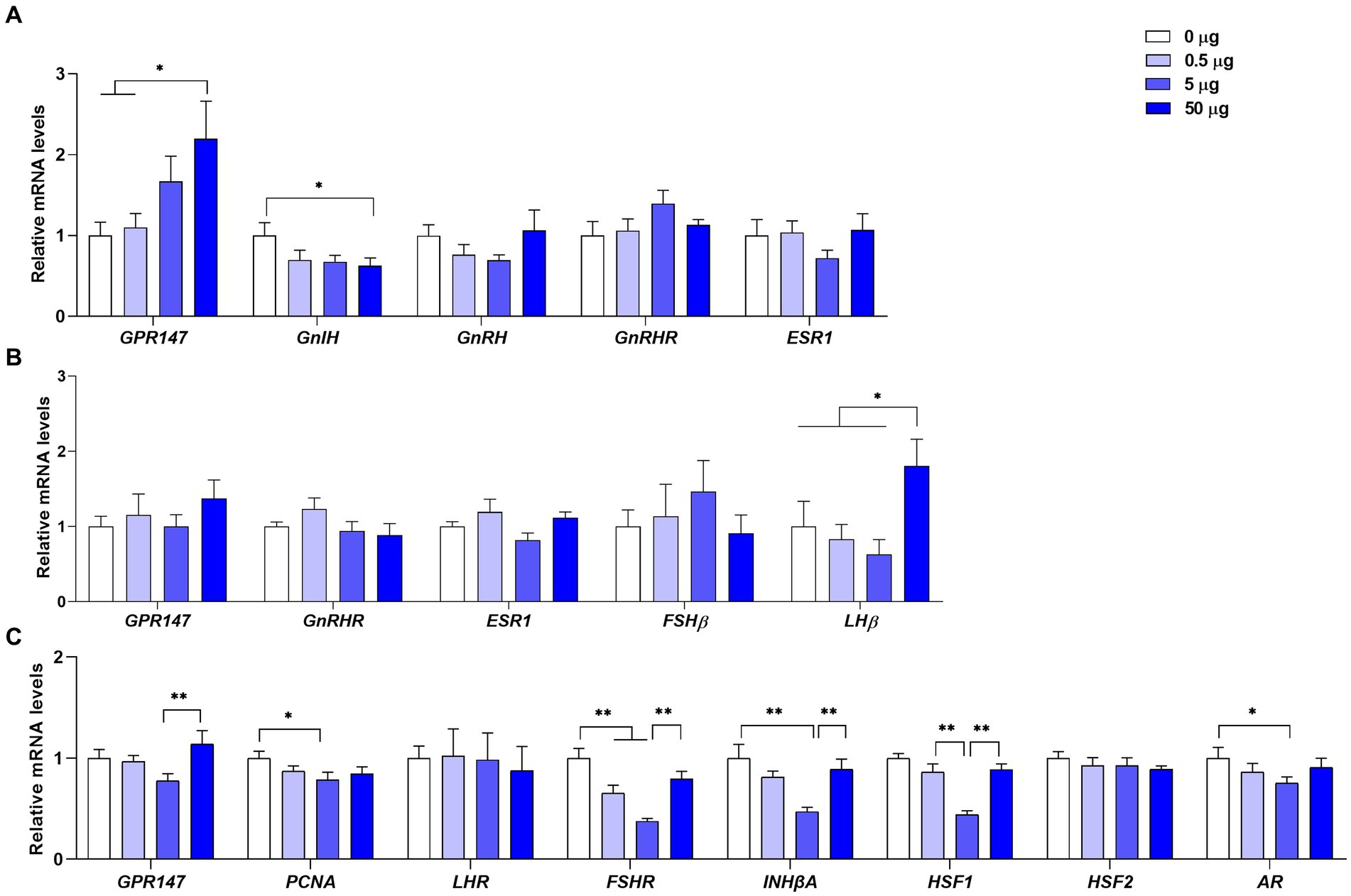
Figure 4. Effects of different injected doses of qGnIH on the mRNA levels of reproductive-related genes in the hypothalamic–pituitary-gonadal (HPG) axis. (A) The mRNA levels of reproductive-related genes in the hypothalamus. (B) The mRNA levels of reproductive-related genes in the pituitary gland. (C) The relative mRNA levels of spermatogenesis genes in testes. Asterisks indicate significant differences * p < 0.05; ** p < 0.01.
3.5.2 Effects of qGnIH on the genes expressed in the pituitary gland
As shown in Figure 4B, the mRNA level of LHβ in the pituitary gland was significantly increased at a dose of 50 μg of qGnIH compared to the vehicle (p < 0.05). Moreover, no significant differences were observed for the mRNA levels of GnRHR, FSHβ, and ESR genes after the injection of qGnIH (p > 0.05).
3.5.3 Effects of GnIH on the spermatogenic genes expressed in the testes
As shown in Figure 4C, the mRNA levels of proliferating cell nuclear antigen (PCNA, p < 0.05), FSH receptor (FSHR, p < 0.01), inhibin beta A (INHβA, p < 0.01), heat shock factor 1 (HSF1, p < 0.01), and androgen receptor (AR, p < 0.05) were lower under the medium qGnIH-injected concentration (5 μg) compared to the vehicle. In addition, although administration with 50 μg of qGnIH did not alter the mRNA levels of testicular spermatogenic genes compared to the vehicle (p > 0.05), it increased the mRNA levels of GPR147 (p < 0.01), FSHR (p < 0.01), INHβA (p < 0.01), and HSF1 (p < 0.01) compared to administration with 5 μg of qGnIH.
4 Discussion
A substantial body of primary research demonstrated that GnIH exerts its regulatory effects on the release or synthesis of GnRH by acting via its receptor-GPR147 in brains. The qGnIH used in the study and rabbit GnIH share a common C-terminal LPXRFamide motif, which plays a crucial role in binding GnIH to its receptor (23). Kriegsfeld et al. reported that the injection of avian GnIH into Syrian hamsters effectively suppressed LH release (24). In the present study, although the administration of 50 μg qGnIH to male rabbits did not alter the hypothalamic mRNA levels of GnRH, GnRHR, and ESR1 or the serum GnRH level, we also observed that it stimulated GPR147 expression, suppressed GnIH gene expression in the hypothalamus and increased LH synthesis and secretion in the pituitary gland. GnRH is released and transported to the anterior pituitary in an impulsive manner, which affects the synthesis and secretion of downstream hormones (25). Therefore, a single detection of GnRH concentration cannot reveal whether GnIH has the action on GnRH. Taken together, administration of qGnIH could regulate downstream genes in the HPG axis and resultant function via GPR147.
In addition to the distribution in brains of vertebrates, the expression at both the mRNA and protein levels of GnIH and its target receptor gene were also detected in gonads and accessory reproductive organs (26, 27). Moreover, GnIH was also reported to be immunolocalized in the spermatogenic cells, Leydig cells, and interstitial cells of testes in hamsters and pigs throughout sexual development (28, 29). Previous studies demonstrated that gonadal GnIH could directly disrupt the differentiation and maturation of stem cells, as well as the synthesis of sex steroids in gonads of several species in an autocrine or paracrine manner (12, 30). The results of our study indicate that the chronic infusion of qGnIH at a dose of 5 μg into male prepubertal rabbits decreased the concentration of testosterone and the mRNA levels of PCNA, FSHR, INHβA, HSF1, and AR genes and increased the resultant apoptosis rate of testicular cells. The expression of PCNA is initiated during the period of spermatogonial germ cell proliferation prior to entering meiosis and it regulates spermatogenesis in the control of DNA replication (31). HSF1 is involved in the reorganization of DNA during spermatid differentiation, and knockout of HSF1 gene results in meiosis arrest and spermatocyte apoptosis (32). The protein product of the INHβA gene, activin A, exerts an influence on germ cell maturation, Sertoli cell function, and the timing of fertility onset (33). Moreover, targeted knockdown of INHβA in fetal Leydig cells disrupts testicular cord elongation and expansion, ultimately leading to reduced spermatogenesis and testicular lesions in adulthood (34). FSHR involves Sertoli cell proliferation, spermatogenesis, and germ cell survival in testes and the FSH signal transduction pathway via FSHR plays a crucial role in regulating the spermatogenesis in mammalian testes (35, 36). AR is involved in the manifestation of secondary sexual characteristics and the initiation and maintenance of spermatogenesis (37). In addition, administration with 5 μg of qGnIH did not alter the concentrations of LH and FSH or the expression levels of GPR147, GnIH and other related genes expression in brains. Therefore, the findings support the hypothesis that GnIH can directly regulate the secretion of steroid hormones and spermatogenesis in mammalian gonads.
Although the majority of studies conducted in vertebrates have consistently demonstrated the inhibitory effect of GnIH on the HPG axis, several investigations carried out in mammals and fish have revealed its stimulatory action (2, 8, 38). In the present study, the serum LH level and its related gene expression level in the pituitary gland were increased by the intraperitoneal injection of 50 μg qGnIH in prepuberal male rabbits. On the other hand, treatment with qGnIH at 50 μg widened the thickness of the spermatogenic layers, resulting in heavier testicular weight and higher GSI. An electrophysiological study showed that GnIH stimulated 18% of GnRH neurons in proestrus female mice, but no stimulation of GnIH on GnRH neuronal firing was observed in female mice during estrus (39). Moussavi et al. (2) reported that intraperitoneal injection of GnIH in different gonadal stages of goldfish has different effects on the mRNA levels of pituitary LHβ and FSHβ and serum LH concentration. Peripheral sex-steroid levels of different estrus or gonadal development stages may modify the action of GnIH (38). Moreover, the internalization of GnIH receptors caused by chronic administration or a high concentration of GnIH and the antagonism of injected peptides may lead to the stimulation of GnIH on the HPG axis (38). The central chronic injection of GnIH to Syrian hamsters adapted to short days could increase testicular weight (40). The complex mechanism may be involved in stimulatory and inhibitory effects of GnIH on the HPG axis depending on different exogenous GnIH injected doses and developmental and reproductive stages.
In the present study, the medium dose (5 μg) of qGnIH reduced the serum testosterone level and the expression abundances of spermatogenic genes in the testes but did not alter the synthesis and secretion of reproductive hormone genes in the brain. Thus, at this dose, qGnIH only regulated testicular development and function at the gonadal level, as well as showed inhibitory action. The high dose (50 μg) of qGnIH increased the expression levels of hypothalamic GPR147 and pituitary LHβ genes and the serum LH level, whereas it decreased the serum testosterone level. This suggests that testicular development and function would be dually regulated both by the direct inhibitory action of qGnIH in testes and the stimulatory action of qGnIH-induced LH produced in brains. GnIH may control both GtH release and synthesis in brains to affect the downstream reproductive system (38). In addition, it directly acts on gonadal regulatory steroid hormone production and germ cell differentiation and maturation (38). Moreover, although the high dose (50 μg) of GnIH had no effects on the mRNA levels of spermatogenesis genes and apoptosis rate of testicular cells compared to the control, it increased the mRNA levels of INHβA, HSF1, and FSHR genes and decreased the apoptosis rate of testicular cells compared to the medium dose (5 μg) of GnIH. At a dose of 50 μg of qGnIH, the stimulatory effect of LH may reduce the direct inhibitory action of qGnIH on testicular germ cell differentiation and maturation and the qGnIH still showed a predominant stimulatory effect on testicular development and function in prepubertal male rabbits. Therefore, the heavier testicular weights, the higher GSI and the wider spermatogenic layer thicknesses were observed compared to the control.
5 Conclusion
In summary, the present study is the first to investigate the action of GnIH on testicular function and development in prepubertal male Minxinan Black rabbits. The medium and high intraperitoneal administration doses (5 and 50 μg) of qGnIH were found to show dual actions on the regulation of spermatogenesis and exhibit complex regulatory mechanisms in male rabbits. The overall results demonstrate that GnIH may show stimulatory or inhibitory activity on gonadal development and function depending on doses in the male prepubertal rabbits and give a reference to how to employ GnIH in mammals.
Data availability statement
The original contributions presented in the study are included in the article/supplementary material, further inquiries can be directed to the corresponding author.
Ethics statement
The animal study was approved by Institutional Animal Care and Use Committee and the Ethics Committee on Animal Experimentation, Fujian Academy of Agricultural Sciences. The study was conducted in accordance with the local legislation and institutional requirements.
Author contributions
LS: Conceptualization, Funding acquisition, Writing – original draft, Writing – review & editing, Project administration. SS: Project administration, Validation, Writing – review & editing. JW: Formal analysis, Project administration, Writing – review & editing. CG: Project administration, Writing – review & editing. DC: Project administration, Writing – review & editing. XX: Conceptualization, Funding acquisition, Writing – review & editing.
Funding
The author(s) declare financial support was received for the research, authorship, and/or publication of this article. This work was supported by the earmarked fund for the China Agriculture Research System (Grant number CARS-43-G-5), Natural Science Foundation of Fujian province (Grant number 2021J01486), and Fujian Public Welfare Project (Grant number 2021R10260014, 2022R1026003). The programs had no roles in the design of the study, the collection, analysis, and interpretation of the data, the writing of the report, or the decision to submit for publication.
Conflict of interest
The authors declare that the research was conducted in the absence of any commercial or financial relationships that could be construed as a potential conflict of interest.
Publisher’s note
All claims expressed in this article are solely those of the authors and do not necessarily represent those of their affiliated organizations, or those of the publisher, the editors and the reviewers. Any product that may be evaluated in this article, or claim that may be made by its manufacturer, is not guaranteed or endorsed by the publisher.
References
1. Ubuka, T, Ukena, K, Sharp, PJ, Bentley, GE, and Tsutsui, K. Gonadotropin-inhibitory hormone inhibits gonadal development and maintenance by decreasing gonadotropin synthesis and release in male quail. Endocrinology. (2006) 147:1187–94. doi: 10.1210/en.2005-1178
2. Moussavi, M, Wlasichuk, M, Chang, JP, and Habibi, HR. Seasonal effect of gonadotrophin inhibitory hormone on gonadotrophin-releasing hormone-induced gonadotroph functions in the goldfish pituitary. J Neuroendocrinol. (2013) 25:506–16. doi: 10.1111/jne.12024
3. Biran, J, Golan, M, Mizrahi, N, Ogawa, S, Parhar, IS, and Levavi-Sivan, B. LPXRFa, the piscine ortholog of GnIH, and LPXRF receptor positively regulate gonadotropin secretion in Tilapia (Oreochromis niloticus). Endocrinology. (2014) 155:4391–401. doi: 10.1210/en.2013-2047
4. Bulut, F, Kacar, E, Bilgin, B, Hekim, MG, Keleştemur, MM, Sahin, Z, et al. Crosstalk between kisspeptin and gonadotropin-inhibitory hormone in the silence of puberty: preclinical evidence from a calcium signaling study. J Recept Signal Transduct Res. (2022) 42:608–13. doi: 10.1080/10799893.2022.2125014
5. Choi, D . Expressions of gonadotropin subunit genes in the reproductively inactive Golden hamsters. Dev Reprod. (2022) 26:37–47. doi: 10.12717/DR.2022.26.2.37
6. Paullada-Salmerón, JA, Cowan, M, Aliaga-Guerrero, M, Morano, F, Zanuy, S, and Muñoz-Cueto, JA. Gonadotropin inhibitory hormone Down-regulates the brain-pituitary reproductive Axis of male European Sea bass (Dicentrarchus labrax). Biol Reprod. (2016) 94:121. doi: 10.1095/biolreprod.116.139022
7. Fraley, GS, Coombs, E, Gerometta, E, Colton, S, Sharp, PJ, Li, Q, et al. Distribution and sequence of gonadotropin-inhibitory hormone and its potential role as a molecular link between feeding and reproductive systems in the Pekin duck (Anas platyrhynchos domestica). Gen Comp Endocrinol. (2013) 184:103–10. doi: 10.1016/j.ygcen.2012.11.026
8. Ubuka, T, Inoue, K, Fukuda, Y, Mizuno, T, Ukena, K, Kriegsfeld, LJ, et al. Identification, expression, and physiological functions of Siberian hamster gonadotropin-inhibitory hormone. Endocrinology. (2012) 153:373–85. doi: 10.1210/en.2011-1110
9. Gotlieb, N, Baker, CN, Moeller, J, and Kriegsfeld, LJ. Time-of-day-dependent sensitivity of the reproductive axis to RFamide-related peptide-3 inhibition in female Syrian hamsters. J Neuroendocrinol. (2019) 31:e12798. doi: 10.1111/jne.12798
10. Pineda, R, Garcia-Galiano, D, Sanchez-Garrido, MA, Romero, M, Ruiz-Pino, F, Aguilar, E, et al. Characterization of the inhibitory roles of RFRP3, the mammalian ortholog of GnIH, in the control of gonadotropin secretion in the rat: in vivo and in vitro studies. Am J Physiol Endocrinol Metab. (2010) 299:E39–46. doi: 10.1152/ajpendo.00108.2010
11. Fallah, HP, Rodrigues, MS, Zanardini, M, Nóbrega, RH, and Habibi, HR. Effects of gonadotropin-inhibitory hormone on early and late stages of spermatogenesis in ex-vivo culture of zebrafish testis. Mol Cell Endocrinol. (2021) 520:111087. doi: 10.1016/j.mce.2020.111087
12. Wang, B, Zhang, Y, Cui, A, Xu, Y, Jiang, Y, Wang, L, et al. LPXRFa and its receptor in yellowtail kingfish (Seriola lalandi): molecular cloning, ontogenetic expression profiles, and stimulatory effects on growth hormone and gonadotropin gene expression. Gen Comp Endocrinol. (2021) 312:113872. doi: 10.1016/j.ygcen.2021.113872
13. Maugars, G, Pasquier, J, Atkinson, C, Lafont, AG, Campo, A, Kamech, N, et al. Gonadotropin-inhibitory hormone in teleosts: new insights from a basal representative, the eel. Gen Comp Endocrinol. (2020) 287:113350. doi: 10.1016/j.ygcen.2019.113350
14. Kumar, P, Wisdom, KS, Bhat, IA, Pathakota, GB, Nayak, SK, Reang, D, et al. Molecular characterization of gonadotropin-inhibitory hormone (GnIH) gene and effect of intramuscular injection of GnIH peptide on the reproductive axis in Catla catla. Anim Biotechnol. (2020) 31:335–49. doi: 10.1080/10495398.2019.1597730
15. Ohga, H, and Matsuyama, M. Effects of LPXRFamide peptides on chub mackerel gonadotropin secretion. Biol Reprod. (2021) 105:1179–88. doi: 10.1093/biolre/ioab130
16. Anjum, S, Krishna, A, and Tsutsui, K. Possible role of GnIH as a mediator between adiposity and impaired testicular function. Front Endocrinol (Lausanne). (2016) 7:6. doi: 10.3389/fendo.2016.00006
17. Wang, X, Guo, G, Zhang, X, Li, M, Xiao, K, Hu, C, et al. Effect of RFRP-3, the mammalian ortholog of GnIH, on the epididymis of male rats. Theriogenology. (2018) 118:196–202. doi: 10.1016/j.theriogenology.2018.05.029
18. Zhang, X, Li, M, Huang, M, Peng, H, Song, X, Chen, L, et al. Effect of RFRP-3, the mammalian ortholog of GnIH, on apoptosis and autophagy in porcine ovarian granulosa cells via the p38MAPK pathway. Theriogenology. (2022) 180:137–45. doi: 10.1016/j.theriogenology.2021.12.024
19. Howard, JG, Brown, JL, Bush, M, and Wildt, DE. Teratospermic and normospermic domestic cats: ejaculate traits, pituitary-gonadal hormones, and improvement of spermatozoal motility and morphology after swim-up processing. J Androl. (1990) 11:204–15. doi: 10.1002/j.1939-4640.1990.tb03229.x
20. Sang, L, Chen, DJ, Sun, SK, Gao, CF, Wang, JX, Chen, YF, et al. Cloning and expression of GnIH gene and its effect on reproductive hormones secretion of young male rabbits. Acta Vet Zootech Sin. (2023) 54:201–2. doi: 10.11843/j.issn.0366-6964.2023.01.019
21. Melo, RMC, Cruz, CKF, Weber, AA, Luz, RK, Bazzoli, N, and Rizzo, E. Effects of temperature manipulation on gamete development and reproductive activity in the farmed catfish Lophiosilurus alexandri. Anim Reprod Sci. (2022) 247:107100. doi: 10.1016/j.anireprosci.2022.107100
22. Zhou, X, Wang, S, Zhou, R, Zhang, T, Wang, Y, Zhang, Q, et al. Erectile dysfunction in hypospadiac male adult rats induced by maternal exposure to di-n-butyl phthalate. Toxicology. (2022) 475:153227. doi: 10.1016/j.tox.2022.153227
23. Yin, H, Ukena, K, Ubuka, T, and Tsutsui, K. A novel G protein-coupled receptor for gonadotropin-inhibitory hormone in the Japanese quail (Coturnix japonica): identification, expression and binding activity. J Endocrinol. (2005) 184:257–66. doi: 10.1677/joe.1.05926
24. Kriegsfeld, LJ, Mei, DF, Bentley, GE, Ubuka, T, Mason, AO, Inoue, K, et al. Identification and characterization of a gonadotropin-inhibitory system in the brains of mammals. Proc Natl Acad Sci U S A. (2006) 103:2410–5. doi: 10.1073/pnas.0511003103
25. Okamura, H, Tsukamura, H, Ohkura, S, Uenoyama, Y, Wakabayashi, Y, and Maeda, K. Kisspeptin and GnRH pulse generation. Adv Exp Med Biol. (2013) 784:297–323. doi: 10.1007/978-1-4614-6199-9_14
26. Fallah, HP, and Habibi, HR. Role of GnRH and GnIH in paracrine/autocrine control of final oocyte maturation. Gen Comp Endocrinol. (2020) 299:113619. doi: 10.1016/j.ygcen.2020.113619
27. Alonge, MM, Greville, LJS, Faure, PA, and Bentley, GE. Immunoreactive distribution of gonadotropin-inhibitory hormone precursor, RFRP, in a temperate bat species (Eptesicus fuscus). J Comp Neurol. (2022) 530:1459–69. doi: 10.1002/cne.25291
28. Zhao, S, Zhu, E, Yang, C, Bentley, GE, Tsutsui, K, and Kriegsfeld, LJ. RFamide-related peptide and messenger ribonucleic acid expression in mammalian testis: association with the spermatogenic cycle. Endocrinology. (2010) 151:617–27. doi: 10.1210/en.2009-0978
29. Zheng, L, Su, J, Fang, R, Jin, M, Lei, Z, Hou, Y, et al. Developmental changes in the role of gonadotropin-inhibitory hormone (GnIH) and its receptors in the reproductive axis of male Xiaomeishan pigs. Anim Reprod Sci. (2015) 154:113–20. doi: 10.1016/j.anireprosci.2015.01.004
30. Rodrigues, MS, Fallah, HP, Zanardini, M, Malafaia, G, Habibi, HR, and Nóbrega, RH. Interaction between thyroid hormones and gonadotropin inhibitory hormone in ex vivo culture of zebrafish testis: an approach to study multifactorial control of spermatogenesis. Mol Cell Endocrinol. (2021) 532:111331. doi: 10.1016/j.mce.2021.111331
31. Miura, C, Miura, T, and Yamashita, M. PCNA protein expression during spermatogenesis of the Japanese eel (Anguilla japonica). Zool Sci. (2002) 19:87–91. doi: 10.2108/zsj.19.87
32. Widlak, W, and Vydra, N. The role of heat shock factors in mammalian spermatogenesis. Adv Anat Embryol Cell Biol. (2017) 222:45–65. doi: 10.1007/978-3-319-51409-3_3
33. Archambeault, DR, Tomaszewski, J, Childs, AJ, Anderson, RA, and Yao, HH. Testicular somatic cells, not gonocytes, are the major source of functional activin a during testis morphogenesis. Endocrinology. (2011) 152:4358–67. doi: 10.1210/en.2011-1288
34. Archambeault, DR, and Yao, HH. Activin a, a product of fetal Leydig cells, is a unique paracrine regulator of Sertoli cell proliferation and fetal testis cord expansion. Proc Natl Acad Sci U S A. (2010) 107:10526–31. doi: 10.1073/pnas.1000318107
35. Bhartiya, D, and Patel, H. An overview of FSH-FSHR biology and explaining the existing conundrums. J Ovarian Res. (2021) 14:144. doi: 10.1186/s13048-021-00880-3
36. Hermann, BP, and Heckert, LL. Transcriptional regulation of the FSH receptor: new perspectives. Mol Cell Endocrinol. (2007) 260-262:100–8. doi: 10.1016/j.mce.2006.09.005
37. Collins, LL, Lee, HJ, Chen, YT, Chang, M, Hsu, HY, Yeh, S, et al. The androgen receptor in spermatogenesis. Cytogenet Genome Res. (2003) 103:299–301. doi: 10.1159/000076816
38. Ubuka, T, and Parhar, I. Dual actions of mammalian and piscine gonadotropin-inhibitory hormones, RFamide-related peptides and LPXRFamide peptides, in the hypothalamic-pituitary-gonadal Axis. Front Endocrinol (Lausanne). (2018) 8:377. doi: 10.3389/fendo.2017.00377
39. Ducret, E, Anderson, GM, and Herbison, AE. RFamide-related peptide-3, a mammalian gonadotropin-inhibitory hormone ortholog, regulates gonadotropin-releasing hormone neuron firing in the mouse. Endocrinology. (2009) 150:2799–804. doi: 10.1210/en.2008-1623
Keywords: GnIH, dual action, testicular development, spermatogenesis, Minxinan Black rabbit
Citation: Sang L, Sun S, Wang J, Gao C, Chen D and Xie X (2024) Dual effects of gonadotropin-inhibitory hormone on testicular development in prepubertal Minxinan Black rabbits (Oryctolagus cuniculus). Front. Vet. Sci. 11:1320452. doi: 10.3389/fvets.2024.1320452
Edited by:
Rosa Maria Garcia-Garcia, Complutense University of Madrid, SpainReviewed by:
Ana Sanchez-Rodriguez, Spanish National Research Council (CSIC), SpainMarco Berland, Temuco Catholic University, Chile
Copyright © 2024 Sang, Sun, Wang, Gao, Chen and Xie. This is an open-access article distributed under the terms of the Creative Commons Attribution License (CC BY). The use, distribution or reproduction in other forums is permitted, provided the original author(s) and the copyright owner(s) are credited and that the original publication in this journal is cited, in accordance with accepted academic practice. No use, distribution or reproduction is permitted which does not comply with these terms.
*Correspondence: Xiping Xie, eHhwNzAyQDE2My5jb20=