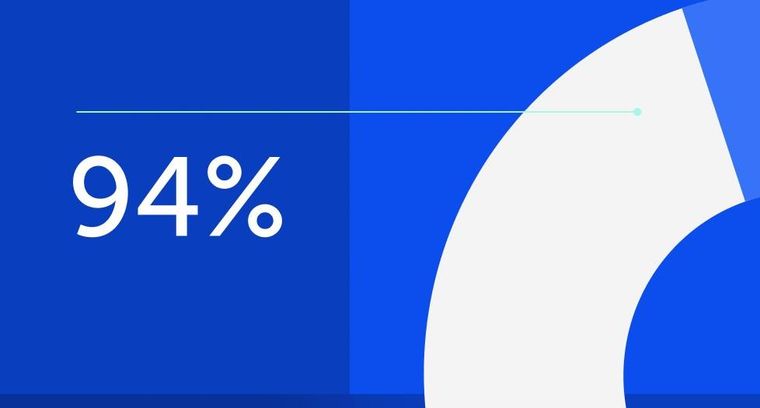
94% of researchers rate our articles as excellent or good
Learn more about the work of our research integrity team to safeguard the quality of each article we publish.
Find out more
ORIGINAL RESEARCH article
Front. Vet. Sci., 05 January 2024
Sec. Animal Nutrition and Metabolism
Volume 10 - 2023 | https://doi.org/10.3389/fvets.2023.1342747
This article is part of the Research TopicNutricines and Derivatives of Nutrients in Animal Health and Disease PreventionView all 15 articles
Dark tea, rich in nutricines including tea polyphenols and free amino acids, is a kind of post-fermented tea. The potential application of nutricines against oxidative damage and senescence, which drives animal health maintenance and disease prevention, has attracted considerable interest. In this study, the effect of dark tea and its effects on longevity and defense against oxidative stress was investigated in the Caenorhabditis elegans (C. elegans) model. Under normal conditions, dark tea extended the lifespan without significant impairment of propagation. It also improved the motility, alleviated the fat accumulation and apoptosis. Additionally, orally administered dark tea could significantly decrease the level of reactive oxygen species (ROS) and resulted in a superior lifespan in H2O2-induced oxidative stressed C. elegans. In antioxidant assays in vitro, dark tea was found to be rich in strong hydroxyl, DPPH and ABTS+ free radical scavenging capacity. Interestingly, mRNA sequence analyses further revealed that dark tea may catalyze intracellular relevant oxidative substrates and synthesize antioxidants through synthetic and metabolic pathways. These results suggest that dark tea is worth further exploration as a potential dietary supplement for the maintenance of animal health and the prevention of related diseases.
The health of farm animals is critical to production and profitability. Alterations in energy metabolism can adversely affect their health. Elevated metabolic demands can lead to a significantly increased oxygen demand which can result in an upsurge in reactive oxygen species (ROS) (1). This, in turn, disrupts the equilibrium between the production and elimination of free radicals, contributing to a heightened inflammatory response (2). Disruptions in the redox balance have been associated with common diseases such as enteritis and mastitis in pigs and cattle, and recurrent airway obstruction in horses (3). However, the currently used chemically synthesized antioxidants have been under suspicion for their association with organic damage (4, 5). The efficient production of foods at a low cost is of great importance for good health. Therefore, researchers have developed a keen interest on the potential application of natural nutrients against oxidative damage and for longevity.
Tea is a commonly consumed functional beverage. It has high levels of safety and significant therapeutic effects, together with low toxicity and minimal side effects. According to the different production methods, tea can be classified into white tea, green tea, yellow tea, oolong tea, black tea, and dark tea. The quality characteristics of tea are associated with a combination of various active substances, including polyphenolic compounds for astringency, free amino acids for the sense of newness, and volatile substances for aromas (6, 7). Tea has a variety of health properties, such as antioxidant, anti-inflammatory, immunomodulatory, anti-cancer, cardio-protective, anti-diabetic, weight loss and hepatoprotective effects (8). The available data suggest that green tea polyphenol supplementation at postpartum improved the milk yield and health status in cows with hyperketonemia during early lactation (9). Green tea extracts could improve intestinal microflora balance, contributing to the prevention of digestive and respiratory organ diseases in calves (10). The catechins components in green tea have been found to relieve oxidative stress and fatty liver disease in dairy cows during the periparturient phase and transition period (11, 12). Hence, tea can be used as a functional substance in livestock feed.
Dark tea is a tree belonging to the Camellia sinensis family, distinguished by the oily black or black-brown color of its leaves (13). The process of production of dark tea involves microorganisms; hence, dark tea is the only post-fermented tea among the six major tea types (14). Compared to the widely studied green, black, and oolong teas, dark tea is often overlooked as a unique post-fermented tea. Dark tea contains phytochemicals and macronutrients, also believed to be beneficial to animals (15). As a natural and harmless nutraceutical, it can be used as a nutritional supplement, food additive and medicinal ingredient (16, 17).
Caenorhabditis elegans (C. elegans) is the first multicellular organism to have its genome sequenced fully. The conservation of illnesses-related pathways between C. elegans and higher organisms, along with the advantages of its short life cycle, simple culture and high reproduction capacity (18, 19), has made C. elegans a favorable in vivo non-rodent model organism for mechanistic explanations and high-throughput screening of drug candidates, being screened for a range of oxidative stress, toxicity and related conditions or diseases (20).
Therefore, in this study, three types of Chinese dark tea, all of which are commonly available in the market and have high sales volumes, were selected as raw materials, namely Brick tea, Pu'er tea, and Liubao tea, to investigate the effects of dark tea on the lifespan, propagation, motility, fat deposition, apoptosis, and resistance to oxidative stress in C. elegans. Additionally, the differences in the expression of genes were explored by the mRNA sequence analyses.
Brick tea (Anhua, China), Pu'er tea (Xishuangbanna, China), or Liubao tea (Wuzhou, China), were mixed with water in a 1:15 ratio of tea to water, and heated in a water bath at 85°C for 1 h, cooled and filtered, and then subjected to rotary evaporation at 58°C for 1 h, respectively (21).
The total polyphenol contents of dark tea were determined through the iron tartrate colorimetric method (22). Free amino acids were detected through ninhydrin colorimetry (23). The active ingredients in dark tea extract are listed in Supplementary Table 1.
Determination of the free radical scavenging ability of Brick, Pu'er, and Liubao teas at concentrations of 200, 400, 600 and 800 μg/mL, respectively.
A sample solution (0.5 mL) of the dark tea extracts was added to 0.5 mL of salicylic acid ethanol solution (9 mmol/L; LABGO, China), 0.5 mL of H2O2 (9 mmol/L; Guangfu, China) solution, and 0.5 mL of FeSO4 (9 mmol/L; BEIJINGSHIJI, China) solution. The mixtures were allowed to react for 30 min in a 37°C water bath, and the absorbance of the resulting solution was measured at 510 nm, and the values were assessed against a blank (24). The hydroxyl radical scavenging ability was calculated by the following formula:
where A1 is the absorbance of the reaction solution with the sample, A2 is the absorbance of the solution without salicylic acid and A0 is the control group where the sample was replaced with distilled water.
Dark tea extracts (2 mL) were added to 2 mL of DPPH (Aladdn, China) ethanol solution (5 mg/mL). The mixtures were allowed to react for 30 min in a dark place, and the absorbance of the resulting solution was measured at 517 nm, and the values were calculated against a blank (25). The DPPH radical scavenging ability was calculated by the following formula:
where A1 is the absorbance of the reaction solution with the sample, A2 is the absorbance of anhydrous ethanol in place of DPPH and A0 is the absorbance of anhydrous ethanol in place of sample.
Dark tea extracts (3 mL) were added to 1 mL of 2,2′-azino-bis(3-ethylbenzthiazoline-6-sulphonate) (ABTS; Rhawn, Chian) solution. The mixtures were allowed to react for 6 min in a 30°C water bath, and the absorbance of the resulting solution was measured at 734 nm, and the values were assessed against a blank (26). The ABTS+ radical scavenging ability was calculated by the following formula:
where A1 is the absorbance of the reaction solution with the sample and A2 is the control group where the sample was replaced with distilled water.
In this research, the wild-type N2 strain of C. elegans was used. All C. elegans strains were grown in the nematode growth medium (NGM) at 20°C, and all C. elegans feed on Escherichia coli OP50. All C. elegans strains and the Escherichia coli OP50 were purchased from the Caenorhabditis Genetics Centre, University of Minnesota (Minneapolis, MN, USA). C. elegans was transferred by cutting a small portion of the nematode-containing NGM using a sterile scalpel, placing it upside down in OP50-coated NGM, and incubating it at 20°C to allow C. elegans to crawl onto the new NGM. At the time of synchronization during the spawning period, C. elegans were washed down with M9 buffer, the lysate was added, and the precipitate was taken by centrifugation at low speed.
The synchronized N2 C. elegans were placed on NGM containing Brick tea extract (600, 700, 800 μg/mL), Pu’er tea extract (600, 700, 800 μg/mL), and Liubao tea extract (600, 700, 800 μg/mL) for incubation, which was set up as the experimental group, and the one with no dark tea extract as the control group. To block C. elegans reproduction, 5-Fluorouracil (FUDR; Rhawn, China) was added. For each strain, at least 100 egg-synchronized C. elegans were grown at 20°C and transferred daily to a fresh plate (27). The number of C. elegans surviving, dying and lost was recorded every day until all C. elegans were dead.
The synchronized N2 C. elegans were placed on NGM containing 600 μg/mL Brick tea extract, 700 μg/mL Pu’er tea extract, and 700 μg/mL Liubao tea extract for cultivation. They were allowed to grow until reaching the L4 stage after which cultivation was continued for an additional 4 days and compared with the control group. Oxidative damage to C. elegans was induced by adding 200 μL of H2O2 (0.5 mmol/L, Rhawn, China) solution to a 96-well plate (28). Then 15 C. elegans were placed into each well, and the number of C. elegans that died was observed at hourly intervals until all of the C. elegans were dead, and the survival rate was estimated.
The method was the same as mentioned in Section 2.6. During the reproductive period (approximately days 1–6), C. elegans were transferred every day to new NGM plates and allowed to deposit progeny. Record the number of eggs laid per day (29).
The same method as mentioned in Section 2.6 was followed. FUDR (Rhawn, China) was added to block C. elegans reproduction and then incubated at 20°C until days 2, 4, 8, 12, and 14. C. elegans were then picked and placed onto agar spacers coated with OP50. The frequency of head bobbing in 1 min and the number of body bending within 20 s were observed using a body microscope (30).
For this, the protocol mentioned in Section 2.6 was followed. Synchronized C. elegans were grown for 4 days after which they were washed with M9 buffer, and the precipitate was collected after low-speed centrifugation. Subsequently, 100 μL of 1% paraformaldehyde (Skyho, China) was added, and the sample was kept at 4°C for 15 min before being snap-frozen and stored at −80°C. After 1 h, the samples were removed, thawed, centrifuged, and rinsed with M9 buffer. Next, a mixture of equal volumes of 2% Triton X-100 (Applygen, China) and 1% Oil Red O (Solarbio, China) was prepared, and 100 μL of this mixture was added for precipitation. The mixture was then incubated in a 37°C temperature-controlled shaker for 30 min (31). Finally, C. elegans were picked and placed on an agar spacer using a picker, and images were captured under a fluorescence microscope.
The DCFH-DA method (32) was used to detect the level of ROS in vivo. ROS levels in C. elegans were measured under both normal conditions and following induction of oxidative damage by H2O2. Same method as Section 2.6. Subsequently, the C. elegans were exposed to H2O2 (0.5 mmol/L) for 2 h at 20°C. The OP50 around C. elegans was washed using M9 buffer; from this, 100 μL of precipitate was taken and 1 μL of 10 mM H2DCF-DA (Chemstan, China) was added, and placed in a constant temperature shaker at 37°C in dark for 30 min. C. elegans was then transferred to a 2% agar spacer, and observed and photographed using a fluorescence microscope at the excitation wavelength of 485 nm and the emission wavelength of 528 nm.
Apoptosis was experimentally detected in vivo using acridine orange staining (33), following the same method mentioned in Section 2.6. After three rinses C. elegans with M9 buffer, the supernatant was aspirated, and 100 μL of acridine orange (25 μg/mL, Klamar, China) staining solution was added and placed on a constant temperature shaker at 37°C, in the dark for 2 h. Subsequently, C. elegans were placed in blank NGM for 10 min to allow recovery from the staining. Finally, they were transferred to 2% agar pads and observed under a fluorescence microscope at an excitation wavelength of 488 nm and an emission wavelength of 515 nm.
After 4 days of feeding on dark tea, C. elegans was rinsed, placed in microfuge tubes, and left to settle, after which the precipitate was taken. RNA-seq was performed on a sequencing platform at Beijing BMK Biotechnology Co., Ltd. (Beijing, China), to obtain insights into aggregate gene transcription in subject C. elegans cells.
Statistical analysis was performed using GraphPad Prism 8, and expressed as mean ± standard deviation (SD). One-way ANOVA was used for multiple group comparisons. Values with p < 0.05 were considered statistically significant. The intensity of quantified fluorograms was analyzed using ImageJ software. Each experiment was repeated three times.
The C. elegans were successively treated with Brick, Pu'er, and Liubao teas under normal culture conditions. As shown in Figure 1 and Table 1, all these treatments affected the normal C. elegans lifespan. The mean lifespan of control C. elegans was 14 days. Treatment with the different teas was found to prolong the lifespan of C. elegans, resulting in increased average lifespan of 21.4, 14.3, and 14.3% after treatment with Brick tea at concentrations of 600, 700, and 800 μg/mL, respectively, 7.1, 21.4, and 14.3% after exposure to the same concentrations of Pu’er tea, and 21.4, 28.4, and 21.4% for Liubao tea, respectively, all relative to the control group. In addition, 600 μg/mL Brick tea, 700 μg/mL Pu'er tea, and 700 μg/mL Liubao tea had the most significant effect on the increase in average and maximum lifespan (Table 1). Therefore, the above-mentioned concentrations were selected for subsequent experiments.
Figure 1. Effects of various concentrations of dark tea (A, Brick Tea; B, Pu’er Tea; C, Liubao Tea) on the lifespan in C. elegans.
Lifespan involves the process of gradual aging, and the oxidative damage pathway accelerates aging (34). Under oxidative stress, the lifespan of dark tea-treated C. elegans increased significantly, especially in those treated with Brick tea or Liubao tea (Figure 2).
Under both normal conditions and oxidative stress, the control group had the highest ROS level and this effect was minimized by dark tea treatments (Figure 3). The fluorescence intensity decreased by 41, 68, and 37% after treatment with Brick, Pu’er, and Liubao teas, respectively (Figure 3C), without H2O2 treatment, and decreased by 28.4, 35.4, and 22.2%, respectively (Figure 3D), in the presence of H2O2 compared with the control group. These results demonstrated that dark tea markedly reduced the levels of ROS in C. elegans, indicating that dark tea has strong antioxidant activity in vivo.
Figure 3. Effects of dark tea on the ROS levels in C. elegans. (A) ROS fluorescence plot in the normal state. (B) ROS fluorescence plot in the oxidative damage state. (C) Quantitative analysis plot of ROS fluorescence intensity in the normal state. (D) Quantitative analysis plot of ROS fluorescence intensity in the oxidative damage state. **** indicates p < 0.0001.
To further assess the effects of dark tea on rendering stress resistance in C. elegans, the free radicals scavenging capacity of dark tea was investigated. As shown in Figure 4, the scavenging capacity of 600 μg/mL of Brick tea for hydroxyl, DPPH, and ABTS+ radicals was maximized. The scavenging ability of Pu’er tea and Liubao tea for DPPH, and ABTS+ radicals increased with increasing concentration; however, the scavenging ability for hydroxyl radicals reached a maximum at 600 μg/mL. These results suggest the strong free radicals scavenging capacity of dark tea.
Figure 4. Effects of dark tea on the free radicals scavenging capacity. (A) -OH. (B) DPPH. (C) ABTS+.
The spawning period of C. elegans was about 6–7 days, and mainly concentrated in the first 3 days (Figure 5A). There was no significant difference between the experimental groups in terms of the total number of offspring (Figure 5B). The results indicated that the reproductive capacity of C. elegans is not impaired by dark tea and provided evidence for the safety of dark tea.
Figure 5. Effects of dark tea on the reproductive capacity in C. elegans. (A) The number of offspring daily in each groups in the pawning period. (B) The total of number of offspring in each group in the pawning period.
With aging, muscle function gradually declines, which in turn slows down mobility. The effect of dark tea on motility was recorded on days 2, 4, 8, 12 and 14 post-dosing, and the results are presented in Figure 6. On day 12, the motility of C. elegans decreased substantially. The dark tea significantly affected the frequency of head bobbing in C. elegans in the pre-preliminary stage, among these, Liubao tea had the most significant effect (Figure 6A). Dark tea substantially enhanced the body bending frequency of C. elegans, whereas the effect of Pu’er tea on C. elegans in the later stages of the process was not significant (Figure 6B). Thus, dark tea can alleviate the aging-related decrease in the energy transportation capacity of C. elegans.
Figure 6. Effects of dark tea on the motility in C. elegans. (A) Frequency of head bobbing in 1 min. (B) Degree of body bending in 20 s. * indicates p < 0.05, ** indicates p < 0.01, *** indicates p < 0.001.
Fat is the root cause of inflammation due to aging (35). Staining with Oil Red O (Solarbio, China) showed a significantly lower number of fat cells in the experimental group than those in the control group (Figure 7A), and C. elegans fed Brick tea had the lowest body fat content. Further analysis revealed that the body fat content of C. elegans fed with Brick tea, Pu’er tea, or Liubao tea was reduced by 9, 4, and 8%, respectively (Figure 7B). These results suggest that dark tea significantly ameliorated age-associated physiological characteristics by reducing fat deposition in C. elegans by reducing fat accumulation.
Figure 7. Effects of dark tea on the fat content in C. elegans. (A) Plot of fat staining. (B) Plot of quantitative analysis of staining intensity. ** indicates p < 0.01, **** indicates p < 0.0001.
Caenorhabditis elegans treated with Brick tea, Pu'er tea, and Liubao tea had significantly lower apoptosis than the control group (Figure 8A). Quantitative analysis of the fluorescence intensity in C. elegans fed Brick tea, Pu’er tea, and Liubao tea decreased by 15, 24, and 14%, respectively (Figure 8B). The above results show that dark tea could achieve anti-aging effects by inhibiting the degree of apoptosis.
Figure 8. Effects of dark tea on the apoptosis in C. elegans. (A) Apoptosis fluorescence graph. (B) Quantitative analysis graph of apoptosis fluorescence intensity. **** indicates p < 0.0001.
Genetic data of dark tea-treated three groups are shown in Figure 9. The volcano plot (red, up-regulated; blue, down-regulated) revealed that the number of down-regulated or up-regulated genes in the Brick tea, Pu’er tea, and Liubao tea groups overlapped, while each group also exhibited unique genes (Figure 9A). Interestingly, the number of down-regulated genes was the highest in the Liubao tea group, while the number of up-regulated genes was the most in the Brick tea group was the most (Figure 9B). Compared to the control group, the same 344 genes were identified in the Brick tea, Pu’er tea, and Liubao tea groups (Figure 9C).
Figure 9. Distribution of genes identified in dark tea. (A) Volcano plot of differentially expressed genes. The horizontal coordinate indicates the logarithmic value of the fold difference in expression of a gene in the two samples and the vertical coordinate indicates the negative logarithmic value of the statistical significance of the change in gene expression. Red dots indicate up-regulation and blue dots indicate down-regulation. (B) Up-regulated and down-regulated genes. (C) Venn diagram of the set of differential genes (fold change≥2).
The gene products were analyzed for GO enrichment in terms of biological process (BP), cellular component (CC), and molecular function (MF), as shown in Figure 10. A few of the same up-regulated and down-regulated genes with functional annotations were identified in the Brick tea, Pu’er tea, and Liubao tea groups, which included cellular process and metabolic process in BP, cellular anatomical entity in CC, and catalytic activity and binding in MF. The alterations in the above-mentioned biological functions have all been shown to affect lifespan (36). Thus, dark tea mainly affects endocrine system-related pathways, especially catalysis-related bio-metabolism within cells.
Figure 10. GO annotation categorization statistical chart. (A) Brick Tea. (B) Pu’er Tea (C) Liubao Tea. The horizontal coordinate is the GO classification, the vertical coordinate is the number of genes, and different colors represent the different primary classifications to which they belong.
To further explore the regulation pathways of dark tea, a KEGG pathway enrichment analysis was conducted (Figure 11). In the Brick tea, Pu’er tea, and Liubao tea groups, the ubiquitin mediated proteolysis, spliceosome and mannose type O-glycan biosynthesis pathways were significantly up-regulated, while fatty acid metabolism and fatty acid degradation pathways were significantly down-regulated. Among the annotated pathways, a majority were closely linked to glucose and lipid metabolism. These pathways suggested the metabolism and biosynthesis are significantly involved in the redox reaction, which were important to improve antioxidant defense system.
Figure 11. Bubble chart of genes enriched in KEGG pathways. (A) Brick Tea. (B) Pu’er Tea (C) Liubao Tea. The bubble size represents the number of genes in the enriched pathway terms, and the bubble color represents the p value.
A total of 2, 1, and 2 genes, respectively, were significantly up-regulated (p < 0.05) in the ubiquitin mediated proteolysis, mannose type O-glycan biosynthesis, and fatty acid metabolism and degradation pathways, respectively (Table 2). Specifically, the expression of PRKN and SKP1 in the ubiquitin mediated proteolysis was significantly up-regulated (p < 0.05). After dark tea treatment, the expression of K12H6.3 in the mannose type O-glycan biosynthesis was significantly up-regulated (p < 0.05); in addition, the expression of ACADM, CPT1 in the fatty acid metabolism and degradation pathways was significantly up-regulated (p < 0.05).
Table 2. Genes after dark tea intervention in the ubiquitin mediated proteolysis, mannose type O-glycan biosynthesis, fatty acid metabolism and fatty acid degradation pathways in C. elegans.
As the only post-fermented tea, many biochemical reactions of the fermentation process in the production of dark tea result in differences that set dark tea and other five types of tea. Twenty-two macronutrient chemical composition indicators in 65 Chinese dark teas have been reported, with the richer ones being polyphenols and free amino acids (9). In the present study, we first determined the content of the main two nutrients in the three represented dark teas. The contents of tea polyphenols and L-theanine in dark tea were maintained at around 10 and 1.5% (Supplementary Table 1).
Free radical production is essential in normal metabolism, and excessive free radical formation may lead to oxidative stress and other related diseases (37). Xu et al. (38) found that tea polyphenols increased SOD, CAT, T-AOC and GSH-Px contents along with a reduction in MAD and ROS in crows. In this study, we found that orally supplied dark tea could significantly decrease the ROS levels and exhibited a superior lifespan in H2O2-induced oxidative stress C. elegans. Similarly, we observed an improvement in-OH, DPPH, and ABTS+ free radicals scavenging capacity in antioxidant assays in vitro, indicating the scavenging ability of dark tea.
The relationship between the activity of antioxidants and longevity promotion has long been noticed. Although many chemically synthesized antioxidants are available, long-term use may have potential side effects (2, 3). We found that dark tea extended the lifespan and motility without significantly impairing propagation in C. elegans. Obesity due to excessive fat accumulation is associated with accelerated onset of diseases occurring in old age, while fat ablation increases life span (39). Apoptosis is also considered an effective immune defense mechanism when the body is subjected to various harmful stimuli (40, 41). Our results indicated that the lipid oxidation products and apoptosis in C. elegans could be reduced after dark tea treatment. Therefore, several conclusions and speculations can be drawn, which suggest that the longevity extension of dark tea might be attributed to the inhibition of lipid oxidation and the extent of generation of apoptosis-related products to protect the health of the body. Noticeably, no significant toxic effects of dark tea were observed in our study.
GO analysis allows for a standardized description of the gene products in terms of the biological pathways involved and cellular localization (42). To elucidate the roles of the identified differentially expressed genes, GO analysis revealed significant enrichment of BP, CC, and MF, namely, cellular process, metabolic process, catalytic activity, and binding, which play independent roles in antioxidant and anti-aging traits. According to KEGG enrichment analysis the number of genes annotated to a metabolic pathway in a differentially expressed gene is significantly greater than the proportion of background genes among all such genes (43). Among the annotated pathways, most are related to glucose and lipid metabolism. Four common enriched signaling pathways identified in three dark tea groups included mannose type O-glycan biosynthesis, fatty acid metabolism, fatty acid degradation, ubiquitin mediated proteolysis, and spliceosome. Further comparison of the differentially expressed genes with the functional annotations of the genes in the database, revealed that most of the genes with more significant changes in gene up-regulation and down-regulation because of dark tea treatment were regulated by SKP1, K12H6.3, ACADM, and CPT1. SKP1 was up-regulated in the ubiquitin mediated proteolysis pathway, which connects cell cycle regulators to the ubiquitin proteolysis machinery to thus improve cellular growth (44). K12H6.3 was found to be involved in the mannose type O-glycan biosynthesis pathway; the gene encodes the relevant core α1,3-fucosyltransferase, whose expression is promoted during development (45). ACADM and CPT1 are key genes catalyzing mitochondrial fatty acid metabolism and degradation. They catalyze the rate-limiting step of the conversion of acyl-coenzyme. As into acyl-carnitines, which can cross membranes to enter the mitochondria, and elevate triglyceride, phospholipid, and droplet levels of cellular lipids to regulate genetic, epigenetic, physiological, and nutritional modulators (46).
Overall, it may be safely concluded that dark tea can independently reduce oxidative stress, thus preventing oxidative injury. As a natural and non-toxic antioxidant, dark tea can be used as a nutritional supplement for livestock and as a functional component of livestock feed, thus acting as a defense against various diseases caused by oxidative damage. It thus has great promise for animal health and disease prevention. Further exploration of dark tea as a potential dietary supplement for animal health maintenance and disease prevention is worth exploring.
In conclusion, the safety of dark tea was demonstrated in C. elegans and dark tea was found to mitigate oxidative stress-induced damage by promoting the clearance of free radicals. Additionally, it was observed that dark tea modulated metabolic processes, including carbohydrates, lipids, and proteins, catalyzing the synthesis of antioxidants from specific oxidative substrates within the cell.
The datasets presented in this study can be found in online repositories. The names of the repository/repositories and accession number(s) can be found at: https://figshare.com/, 10.6084/m9.figshare.24609774.
The animal study was approved by Animal Care and Use Committee, Changchun University of Science and Technology. The study was conducted in accordance with the local legislation and institutional requirements.
JW: Writing – original draft, Writing – review & editing. KZ: Writing – original draft. YZ: Writing – original draft. SG: Writing – review & editing. SZ: Writing – review & editing.
The author(s) declare financial support was received for the research, authorship, and/or publication of this article. This research was supported by the Science and Technology Development Program Project of Jilin Provincial Science and Technology Department (grant number 20220204127YY).
We would like to acknowledge all study participants for their time, effort, and contributions.
The authors declare that the research was conducted in the absence of any commercial or financial relationships that could be construed as a potential conflict of interest.
All claims expressed in this article are solely those of the authors and do not necessarily represent those of their affiliated organizations, or those of the publisher, the editors and the reviewers. Any product that may be evaluated in this article, or claim that may be made by its manufacturer, is not guaranteed or endorsed by the publisher.
The Supplementary material for this article can be found online at: https://www.frontiersin.org/articles/10.3389/fvets.2023.1342747/full#supplementary-material
1. Wu, S, Liu, X, Cheng, L, Wang, D, Qin, G, Zhang, X, et al. Protective mechanism of leucine and isoleucine against H2O2-induced oxidative damage in bovine mammary epithelial cells. Oxidative Med Cell Longev. (2022) 2022:1–22. doi: 10.1155/2022/4013575
2. Erdemli, Z, Erdemli, ME, Gul, M, Altinoz, E, Gul, S, Kocaman, G, et al. Ameliorative effects of crocin on the inflammation and oxidative stress-induced kidney damages by experimental periodontitis in rat. Iran J Basic Med Sci. (2021) 24:825–32. doi: 10.22038/ijbms.2021.55875.12499
3. Lykkesfeldt, J, and Svendsen, O. Oxidants and antioxidants in disease: oxidative stress in farm animals. Vet J. (2007) 173:502–11. doi: 10.1016/j.tvjl.2006.06.005
4. Poljsak, B, Šuput, D, and Milisav, I. Achieving the balance between ROS and antioxidants: when to use the synthetic antioxidants. Oxidative Med Cell Longev. (2013) 2013:1–11. doi: 10.1155/2013/956792
5. Gianeti, MD, Mercurio, DG, and Maia Campos, PM. The use of green tea extract in cosmetic formulations: not only an antioxidant active ingredient. Dermatol Ther. (2013) 26:267–71. doi: 10.1111/j.1529-8019.2013.01552.x
6. Zhang, L, Cao, QQ, Granato, D, Xu, YQ, and Ho, CT. Association between chemistry and taste of tea: a review. Trends Food Sci Technol. (2020) 101:139–49. doi: 10.1016/j.tifs.2020.05.015
7. Chen, GH, Yang, CY, Lee, SJ, Wu, CC, and Tzen, JT. Catechin content and the degree of its galloylation in oolong tea are inversely correlated with cultivation altitude. J Food Drug Anal. (2014) 22:303–9. doi: 10.1016/j.jfda.2013.12.001
8. Tang, GY, Meng, X, Gan, RY, Zhao, CN, Liu, Q, Feng, YB, et al. Health functions and related molecular mechanisms of tea components: an update review. Int J Mol Sci. (2019) 20:6196. doi: 10.3390/ijms20246196
9. Ma, Y, Feng, Y, Song, L, Li, M, Dai, H, Bao, H, et al. Green tea polyphenols supplementation alters immunometabolism and oxidative stress in dairy cows with hyperketonemia. Anim Nutr. (2021) 7:206–15. doi: 10.1016/j.aninu.2020.06.005
10. Ishihara, N, Chu, DC, Akachi, S, and Juneja, LR. Improvement of intestinal microflora balance and prevention of digestive and respiratory organ diseases in calves by green tea extracts. Livest Prod Sci. (2001) 68:217–29. doi: 10.1016/S0301-6226(00)00233-5
11. Wein, S, Beyer, B, Gohlke, A, Blank, R, Metges, CC, and Wolffram, S. Systemic absorption of catechins after intraruminal or intraduodenal application of a green tea extract in cows. PLoS One. (2016) 11:e0159428. doi: 10.1371/journal.pone.0159428
12. Vizzotto, EF, Stivanin, SC, de Paris, M, Passos, LT, Werncke, D, Klein, CP, et al. Supplementation with green tea and oregano extracts on productive characteristics, blood metabolites, and antioxidant status of Jersey cows during the transition period. Animal. (2021) 15:100032. doi: 10.1016/j.animal.2020.100032
13. Kong, YS, Ren, HY, Liu, R, da Silva, RR, Aksenov, AA, Melnik, AV, et al. Microbial and nonvolatile chemical diversities of Chinese dark teas are differed by latitude and pile fermentation. J Agric Food Chem. (2022) 70:5701–14. doi: 10.1021/acs.jafc.2c01005
14. Yue, Y, Chu, GX, Liu, XS, Tang, X, Wang, W, Liu, GJ, et al. TMDB: a literature-curated database for small molecular compounds found from tea. BMC Plant Biol. (2014) 14:243–8. doi: 10.1186/s12870-014-0243-1
15. Hamer, M. The beneficial effects of tea on immune function and inflammation: a review of evidence from in vitro, animal, and human research. Nutr Res. (2007) 27:373–9. doi: 10.1016/j.nutres.2007.05.008
16. Lin, FJ, Wei, XL, Liu, HY, Li, H, Xia, Y, Wu, DT, et al. State-of-the-art review of dark tea: from chemistry to health benefits. Trends Food Sci Technol. (2021) 109:126–38. doi: 10.1016/j.tifs.2021.01.030
17. Deng, H, Liu, J, Xiao, Y, Wu, JL, and Jiao, R. Possible mechanisms of dark tea in cancer prevention and management: a comprehensive review. Nutrients. (2023) 15:3903. doi: 10.3390/nu15183903
18. Hunt, PR. The C. elegans model in toxicity testing. J Appl Toxicol. (2017) 37:50–9. doi: 10.1002/jat.3357
19. Lin, C, Zhang, X, Su, Z, Xiao, J, Lv, M, Cao, Y, et al. Carnosol improved lifespan and healthspan by promoting antioxidant capacity in Caenorhabditis elegans. Oxidative Med Cell Longev. (2019) 2019:1–13. doi: 10.1155/2019/5958043
20. Shen, P, Yue, Y, Zheng, J, and Park, Y. Caenorhabditis elegans: a convenient in vivo model for assessing the impact of food bioactive compounds on obesity, aging, and Alzheimer's disease. Annu Rev Food Sci Technol. (2018) 9:1–22. doi: 10.1146/annurev-food-030117-012709
21. Wang, BS, Yu, HM, Chang, LW, Yen, WJ, and Duh, PD. Protective effects of pu-erh tea on LDL oxidation and nitric oxide generation in macrophage cells. LWT Food Sci Technol. (2008) 41:1122–32. doi: 10.1016/j.lwt.2007.07.002
22. Qadir, M, Muhammad, T, Bakri, M, and Gao, F. Determination of total polyphenols in tea by a flow injection-fiber optic spectrophotometric system. Instrument Sci Technol. (2018) 46:185–93. doi: 10.1080/10739149.2017.1346515
23. Sui, H, Chen, L, Han, XX, Zhang, X, Wang, X, and Zhao, B. Quantitative determination of total amino acids based on surface-enhanced raman scattering and ninhydrin derivatization. Anal Sci. (2017) 33:53–7. doi: 10.2116/analsci.33.53
24. Romulo, A. The principle of some in vitro antioxidant activity methods. InIOP Conf Ser Earth Environ Sci. (2020) 426:012177. doi: 10.1088/1755-1315/426/1/012177
25. Zhang, L, Wang, D, Chen, W, Tan, X, and Wang, P. Impact of fermentation degree on the antioxidant activity of pu-erh tea in vitro. J Food Biochem. (2012) 36:262–7. doi: 10.1111/j.1745-4514.2010.00536.x
26. Kına, E, Uysal, İ, Mohammed, FS, Doğan, M, and Sevindik, M. In-vitro antioxidant and oxidant properties of Centaurea rigida. Turkish J Agric Food Sci Technol. (2021) 9:1905–7. doi: 10.24925/turjaf.v9i10.1905-1907.4603
27. Reigada, I, Moliner, C, Valero, MS, Weinkove, D, Langa, E, and Gómez, RC. Antioxidant and antiaging effects of licorice on the Caenorhabditis elegans model. J Med Food. (2020) 23:72–8. doi: 10.1089/jmf.2019.0081
28. Lin, Q, Song, B, Zhong, Y, Yin, H, Li, Z, Wang, Z, et al. Effect of sodium hyaluronate on antioxidant and anti-ageing activities in Caenorhabditis elegans. Foods. (2023) 12:1400. doi: 10.3390/foods12071400
29. Wang, X, Zhang, Z, Zhou, H, Sun, X, Chen, X, and Xu, N. The anti-aging effects of Gracilaria lemaneiformis polysaccharide in Caenorhabditis elegans. Int J Biol Macromol. (2019) 140:600–4. doi: 10.1016/j.ijbiomac.2019.08.186
30. Lin, C, Xiao, J, Xi, Y, Zhang, X, Zhong, Q, Zheng, H, et al. Rosmarinic acid improved antioxidant properties and healthspan via the IIS and MAPK pathways in Caenorhabditis elegans. Biofactors. (2019) 45:774–87. doi: 10.1002/biof.1536
31. Qin, X, Wang, W, and Chu, W. Antioxidant and reducing lipid accumulation effects of rutin in Caenorhabditis elegans. Biofactors. (2021) 47:686–93. doi: 10.1002/biof.1755
32. Huang, RT, Huang, Q, Wu, GL, Chen, CG, and Li, ZJ. Evaluation of the antioxidant property and effects in Caenorhabditis elegans of Xiangxi flavor vinegar, a Hunan local traditional vinegar. J Zhejiang Univ Sci B. (2017) 18:324–33. doi: 10.1631/jzus.B1600088
34. Argyropoulou, A, Aligiannis, N, Trougakos, IP, and Skaltsounis, AL. Natural compounds with anti-ageing activity. Nat Prod Rep. (2013) 30:1412–37. doi: 10.1039/c3np70031c
35. Bhuyan, LP, Sabhapondit, S, Baruah, BD, Bordoloi, C, Gogoi, R, and Bhattacharyya, P. Polyphenolic compounds and antioxidant activity of CTC black tea of North-East India. Food Chem. (2013) 141:3744–51. doi: 10.1016/j.foodchem.2013.06.086
36. Lam, WY, Jash, A, Yao, CH, D’Souza, L, Wong, R, Nunley, RM, et al. Metabolic and transcriptional modules independently diversify plasma cell lifespan and function. Cell Rep. (2018) 24:2479–2492.e6. doi: 10.1016/j.celrep.2018.07.084
37. Wu, JQ, Kosten, TR, and Zhang, XY. Free radicals, antioxidant defense systems, and schizophrenia. Prog Neuro Psychopharmacol Biol Psychiatry. (2013) 46:200–6. doi: 10.1016/j.pnpbp.2013.02.015
38. Xu, R, Zhu, M, Cao, J, and Guo, M. Tea polyphenols protect the mammary gland of dairy cows by enhancing antioxidant capacity and regulating the TGF-β1/p38/JNK pathway. Meta. (2022) 12:1009. doi: 10.3390/metabo12111009
39. Long, W, Zhang, G, Dong, Y, and Li, D. Dark tea extract mitigates hematopoietic radiation injury with antioxidative activity. J Radiat Res. (2018) 59:387–94. doi: 10.1093/jrr/rrx072
40. Pfeffer, CM, and Singh, AT. Apoptosis: a target for anticancer therapy. Int J Mol Sci. (2018) 19:448. doi: 10.3390/ijms19020448
41. Salminen, A, Ojala, J, and Kaarniranta, K. Apoptosis and aging: increased resistance to apoptosis enhances the aging process. Cell Mol Life Sci. (2011) 68:1021–31. doi: 10.1007/s00018-010-0597-y
42. Ashburner, M, Ball, CA, Blake, JA, Botstein, D, Butler, H, Cherry, JM, et al. Gene ontology: tool for the unification of biology. Nat Genet. (2000) 25:25–9. doi: 10.1038/75556
43. Kanehisa, M, Furumichi, M, Sato, Y, Kawashima, M, and Ishiguro-Watanabe, M. KEGG for taxonomy-based analysis of pathways and genomes. Nucleic Acids Res. (2023) 51:D587–92. doi: 10.1093/nar/gkac963
44. Guan, Y, Leu, NA, Ma, J, Chmátal, L, Ruthel, G, Bloom, JC, et al. SKP1 drives the prophase I to metaphase I transition during male meiosis. Sci Adv. (2020) 6:eaaz2129. doi: 10.1126/sciadv.aaz2129
45. Wilson, IB. Glycosylation of proteins in plants and invertebrates. Curr Opin Struct Biol. (2002) 12:569–77. doi: 10.1016/S0959-440X(02)00367-6
Keywords: dark tea, oxidative stress, longevity, mRNA sequencing analysis, Caenorhabditis elegans
Citation: Wang J, Zhang K, Zhang Y, Ge S and Zhang S (2024) Defense against oxidative stress in Caenorhabditis elegans by dark tea. Front. Vet. Sci. 10:1342747. doi: 10.3389/fvets.2023.1342747
Received: 22 November 2023; Accepted: 14 December 2023;
Published: 05 January 2024.
Edited by:
Tao Wang, Jilin Agricultural University, ChinaReviewed by:
Yonggang Liu, Beijing University of Chinese Medicine, ChinaCopyright © 2024 Wang, Zhang, Zhang, Ge and Zhang. This is an open-access article distributed under the terms of the Creative Commons Attribution License (CC BY). The use, distribution or reproduction in other forums is permitted, provided the original author(s) and the copyright owner(s) are credited and that the original publication in this journal is cited, in accordance with accepted academic practice. No use, distribution or reproduction is permitted which does not comply with these terms.
*Correspondence: Shumin Ge, Z2VzaHVtaW4yMDEzQDE2My5jb20=; Shuhua Zhang, emhhbmdzaDEwMThAMTI2LmNvbQ==
Disclaimer: All claims expressed in this article are solely those of the authors and do not necessarily represent those of their affiliated organizations, or those of the publisher, the editors and the reviewers. Any product that may be evaluated in this article or claim that may be made by its manufacturer is not guaranteed or endorsed by the publisher.
Research integrity at Frontiers
Learn more about the work of our research integrity team to safeguard the quality of each article we publish.