- 1College of Animal Science, Shanxi Agricultural University, Jinzhong, China
- 2DSM Nutritional Products Animal Nutrition & Health, Shanghai, China
This study evaluated the impacts of α-amylase (AM) and coated α-amylase (CAM) on bull performance, nutrient digestibility, and ruminal fermentation. This study randomized 60 Holstein bulls of 365 ± 11.5 days of age and 457.5 ± 9.35 kg body weight into three groups: without AM addition, adding AM 0.6 g/kg dry matter (DM), and adding CAM 0.6 g AM/kg DM, separately. This whole experimental period was 80 days, including a 20-day adaptation period and a 60-day data and sample acquisition period. In comparison with the unsupplemented control, dry matter intake (DMI) was unaltered; however, average daily gain (ADG) and feed efficiency (FE) were greater for AM or CAM addition. Bulls receiving AM or CAM supply had greater total-tract nutrient digestibility, ruminal total volatile fatty acids (VFA) content, propionate molar proportion, cellulolytic enzyme and AM activities, and the number of microorganisms. In addition, the activities of AM and trypsin in the jejunum and ileum and glucose, albumin, and total protein concentrations in serum were greater for AM or CAM addition compared to the control. When comparing the supplementation mode of AM, bulls receiving CAM addition had greater ADG and FE. The crude protein and starch digestibility and intestinal AM and trypsin activity were higher, while acid detergent fiber (ADF) digestibility was lower for CAM addition than for AM addition. The lower propionate molar proportion and cellobiase and carboxymethyl cellulase activities, together with Ruminococcus albus, Ruminococcus flavefaciens, and Fibrobacter succinogenes populations were observed for CAM addition compared with AM addition. However, there were greater glucose, albumin, and total protein concentrations in serum after adding CAM. According to the data, the supply of AM improved ADG, nutrient digestion, and rumen fermentation. Notably, the optimum supplementation mode was in the form of CAM in bulls.
1 Introduction
Dietary starch is the main energy source for rumen microorganisms and ruminants. Starch in the diet is degraded to propionate and hydrolyzed to glucose in the rumen and small intestine, respectively (1, 2). Approximately 80% of rumen propionate is used to synthesize glucose by gluconeogenesis (1). Therefore, increasing rumen propionate production and/or intestine starch digestibility can improve dietary energy utilization efficiency and cause an increase in bull performance. It was reported that supplementing α-amylase (AM) in diets increased average daily gain (ADG) in finishing beef (3) and improved lactation performance in dairy cows (4, 5). Moreover, some studies found that AM addition increased rumen butyrate molar proportion (6), stimulated B. fibrisolvens D1 growth in vitro (7), and increased AM activity and rumen propionate molar proportion of cows (8). Others reported that supplementation with AM increased total-tract digestibility of organic matter (OM), dry matter (DM), neutral detergent fiber (NDF), and crude protein (CP) in cows (4, 9). The synergistic effect of exogenous enzymes and ruminal enzymes increased nutrient degradation (10, 11). These findings showed that supplementing AM in diets could potentially improve nutrient digestibility in the rumen. The improvement was correlated with the stimulating effects of exogenous enzymes on ruminal microbial growth. Nevertheless, no studies evaluated the impact of AM supplements on ruminal fermentation and microflora in bulls. Furthermore, starch digestibility in the small intestine was only 40%–62% in ruminants (12, 13); thus, the exogenous AM supply was required. Studies in weaned pigs and broilers found that exogenous AM addition increased the activities of AM and trypsin in the small intestine (14, 15). However, dietary AM would be destroyed and inactivated in the abomasum (10). Noziere et al. (8) discovered that AM supplementation increased starch degradability in the rumen but did not alter total-tract starch digestion in cows. The coated AM (CAM) supplement can avoid the negative influence of abomasum, releasing 25.3% of AM in the rumen and 69.5% of AM in the intestine. Hence, supplementation with CAM might have a greater increase in ADG than AM addition in bulls.
Based on the research studies above, it is necessary to define the regulatory characteristics of exogenous AM on rumen fermentation and microflora and to find out the proper supplementation mode of AM in ruminant diets. As a result, the study explored the impacts of AM and CAM addition on nutrient digestibility, growth performance, rumen fermentation, microflora, and digestive enzyme activities in the rumen and small intestine of bulls.
2 Materials and methods
2.1 AM and CAM supplementation
The AM was generated by Bacillus licheniformis (Ronozyme RumiStar, DSM Nutritional Products, Basel, Switzerland), and AM activity is 600 KNU/g. 1 KNU is the enzyme level produced during the 2-step α-amylase/α-glucosidase reaction at 37°C and pH 7.0 with 6 μmol p-nitrophenol/min based on 1.86 mM ethylidene-G7-pnitrophenyl-maltoheptaoside (16). The CAM (AM = 40%, hydrogenated fat (C16:0-C18:0 ratio = 2:1) = 37%, calcium stearate = 13%, and bentonite powder = 10%) was produced following Wang et al.’s procedure (17). The release rates of CAM, identified using nylon bag techniques, were 25.3% and 69.5% in the rumen and intestine of ruminal and duodenal fistula bulls, respectively (17).
2.2 Animals and experimental design
In this study, our experimental protocols gained approval from the Animal Care and Use Committee of Shanxi Agricultural University. In a randomized block design, 60 Holstein bulls with an age of 365 ± 11.5 days and a body weight (BW) of 457.5 ± 9.35 kg were selected and assigned to three groups: without AM addition, AM 0.6 g/kg DM addition, or CAM 0.6 g AM/kg DM addition, respectively. The AM supplementation amount was determined based on the results of Bachmann et al. (18) and Arturo et al. (19), with the addition of AM 0.5 g/kg DM increasing milk yield in dairy cows. The AM or CAM was added to the premix, mixed with the concentrate, and then incorporated into the total mixed rations (TMR). The basal diet composition and components (Table 1) were prepared as recommended by NASEM (20). Bulls were raised in separate stalls (3 m × 3 m), and fed at 07:00 and 19:00 daily, with free access to water and feed. Additionally, the course of the experiment was 80 days, including a 20-day adaptation period and a 60-day data and sample acquisition period.
2.3 Data collection and sampling procedures
Individual bull BW was determined on days 0/30/60 before feeding at 07:00. We recorded daily DM intake (DMI) for each bull. The feed efficiency (FE) was calculated by dividing ADG by DMI. From days 51 to 57 and for each bull, TMR and refusal samples were gathered daily, and feces (300 g) were gathered from the rectum every 6 h. TMR, refusal, and fecal samples were kept at −20°C, composited by individual animals, dried under 65°C until an unchanged weight was reached, and then ground using a 1-mm filter. The method of AOAC was adopted to measure the contents of DM (method 934.01), OM (method 942.05), acid detergent fiber (ADF; method 973.18), CP (method 990.03), and ether extract (EE; method 920.39) (21). NDF was measured according to the method proposed by Van Soest et al. (22), whereas acid-insoluble ash was identified as depicted by Van-Keulen and Young (23). Starch was enzymatically analyzed in accordance with Hall (24).
On the 58th and 59th days, 200 mL of ruminal fluid was collected from each bull at 04:00, 10:00, 16:00, and 22:00 with the stomach tube. To prevent salivary contamination, we eliminated the initial ruminal fluid (200 mL). An electric pH meter (Sartorius Basic pH Meter PB-10, Sartorius AG) was used to determine the ruminal fluid pH, followed by filtering with the four-layer medical gauze. The collected filtrates were preserved at −20°C and − 80°C, respectively. The AOAC method was adopted to identify ammonia N level (21), while gas chromatography (GC, Trace 1,300; Thermo Fisher Scientific Co., Ltd., Shanghai, China) was conducted to measure VFA using 2-ethylbutyric acid as the endogenous reference.
The ruminal fluid samples preserved at −80°C were employed to determine microbial enzyme activities and populations. We measured the enzyme activities according to the reports of Agarwal et al. (25) and Miller (26). The RBB + C method was used to isolate total microbial DNA from 1.5 mL of ruminal fluid homogenate as Yu and Morrison’s report (27). Extracted DNA purity was determined by agarose gel electrophoresis, while its content was analyzed using a NanoDrop 2000 spectrophotometer (Thermo Scientific, United States). Table 2 presents the primer sequences of microbes. With the use of the regular PCR, DNA standard obtained from samples for PCR assays was acquired by pooling microbial DNA of the treatment set. Subsequently, Pure Link TM Quick Gel Extraction and PCR Purification Combo Kit (Thermo Fisher Scientific Co., Ltd., Shanghai, China) was used for purifying PCR products, while the spectrophotometer was used for quantification. In accordance with the PCR product length as well as mass concentration, we evaluated copy number concentration in every standard substance. The standard curve of the target microorganism was established by 10-fold serial dilutions method (28). The StepOneTM system (Thermo Fisher Scientific Co., Ltd., Shanghai, China) was employed for qPCR amplification and detection. Each sample was measured thrice. The reaction volume of 20 μL was prepared, consisting of SYBR Premix Ex Taq TM II (10 μL, Takara Biotechnology Co., Ltd., Dalian, China), each primer (0.8 μL), template DNA (2 μL), double-standard sterile water (6.0 μL), and ROX Reference Dye II (0.4 μL). RT-PCR conditions were as follows: 2-min initial denaturation under 50°C and 2 min under 95°C, 15 s under 95°C, and 1 min under 60°C for 45 cycles, followed by product elongation. From 60°C to 95°C, the temperature was increased at a rate of 1°C every 30 s.
On day 60 and before the morning feeding, blood samples were collected for all bulls via coccygeal vessels. The samples were subjected to 15-min centrifugation at 2,000g and 4°C to obtain serum and were preserved under −20°C. Glucose, total protein, insulin, albumin, urea N, and lactic acid contents were determined using ELISA kits (Shanghai Duma Biological Co., Ltd., Shanghai, China).
On the 61st day, we selected five bulls from each group at random for slaughter. After slaughtering, 50 cm of duodenum, anterior, middle, and posterior segments of the jejunum, and 50 cm of the ileum were quickly harvested. Then, chyme specimens collected from every bull were blended with the equivalent amount of 0.9% NaCl solution and were homogenized for 15 min, prior to 10-min centrifugation (4°C and 11,000×g) to obtain supernatants for determining AM, trypsin, and lipase activities by ELISA kits (Shanghai Enzyme Link Biological Co., Ltd., Shanghai, China).
2.4 Computation and statistical analysis
The SAS MIXED procedure was used to determine DMI, BW, ADG, and FE (2002; Proc Mixed) (29). The model is shown below:
Other measurement results, which were explored, are shown below:
where Yijklm denotes a dependent variable; μ indicates the total average; Bi represents random effect of ith block; Gj denotes fixed effects of AM (j = 0 or 0.6 g/kg DM); Hk denotes fixed effects of CAM (k = 0 or CAM 0.6 g AM/kg DM); Tl denotes fixed effect of feeding time (30 or 60 d); (TG)jl denotes the interaction effect of feeding time (30 or 60 d) with AM (0 or 0.6 g/kg); (TH)kl denotes interaction effect of feeding time (30 or 60 d) with CAM (0 or CAM 0.6 g AM/kg); (TGH)jkl denotes the interaction effect of feeding time (30 or 60 d), AM (0 or 0.6 g/kg), and CAM (0 or CAM 0.6 g AM/kg); Rm denotes random effects of mth bull, whereas εijklm denotes a residual error. The covariance structure for variables was first-order autoregressive, which was determined by the lowest Akaike’s information criterion (AIC). p < 0.05 denotes statistically significant effects.
3 Results
3.1 Growth performance
0.6 g AM/kg DM supplementation (AM or CAM) did not affect DMI and BW during this trial but elevated ADG (p < 0.05) and FE (p < 0.05) (Table 3). DMI and BW were similar; however, ADG and FE were greater (p < 0.05) after CAM supplementation relative to AM supply.
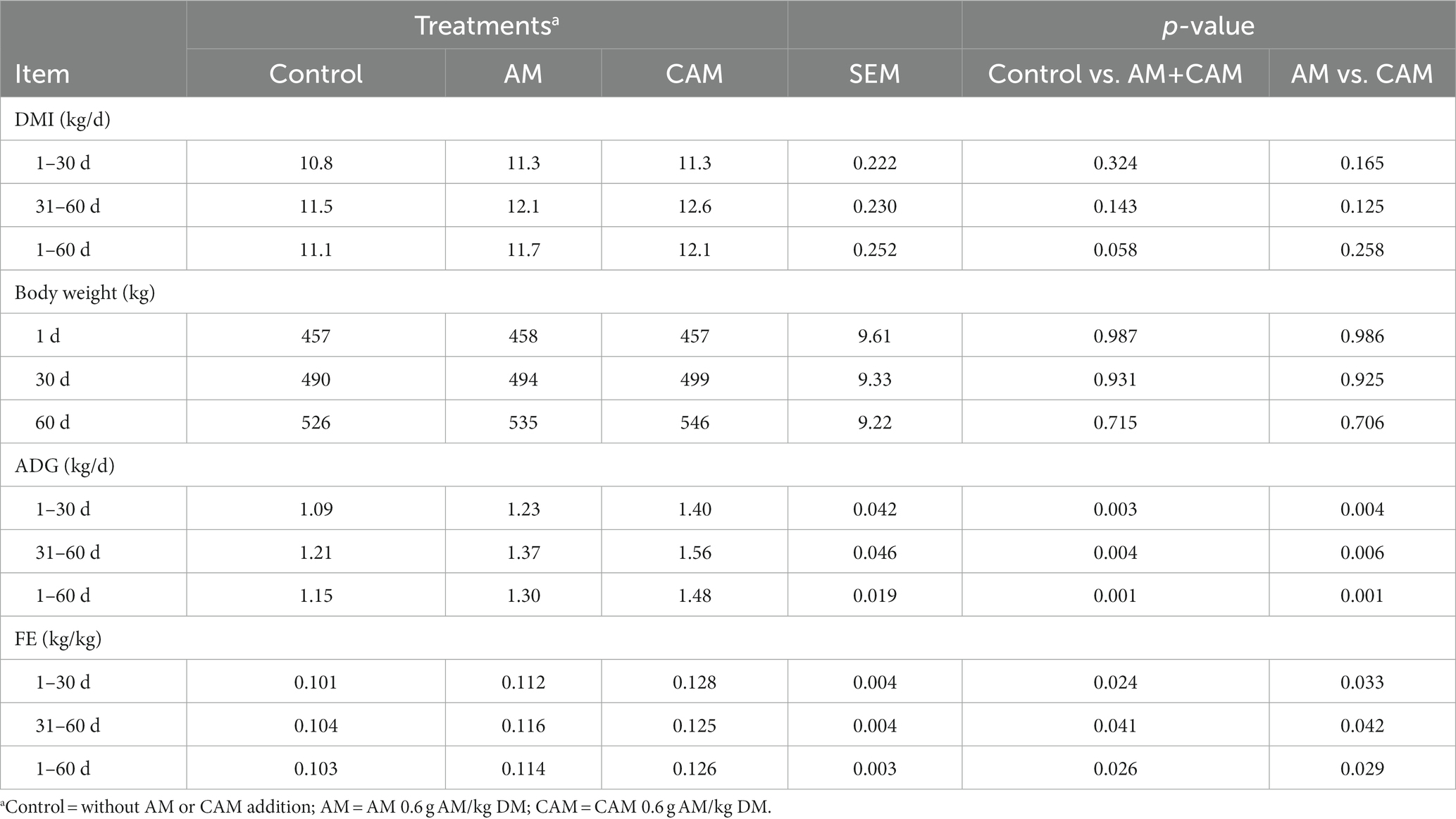
Table 3. Effects of α-amylase (AM) and coated α-amylase (CAM) on DMI, ADG, and FE of Holstein bulls.
3.2 Total-tract nutrient digestibility and ruminal fermentation
Adding 0.6 g/kg DM AM (AM or CAM) could increase (p < 0.05) total-tract digestibility of DM, OM, CP, EE, NDF, ADF, and starch (Table 4). The DM, OM, EE, and NDF digestibility were similar, CP and starch digestibility were greater (p < 0.05), while ADF digestibility was lower (p = 0.012) after CAM supplementation relative to AM addition.
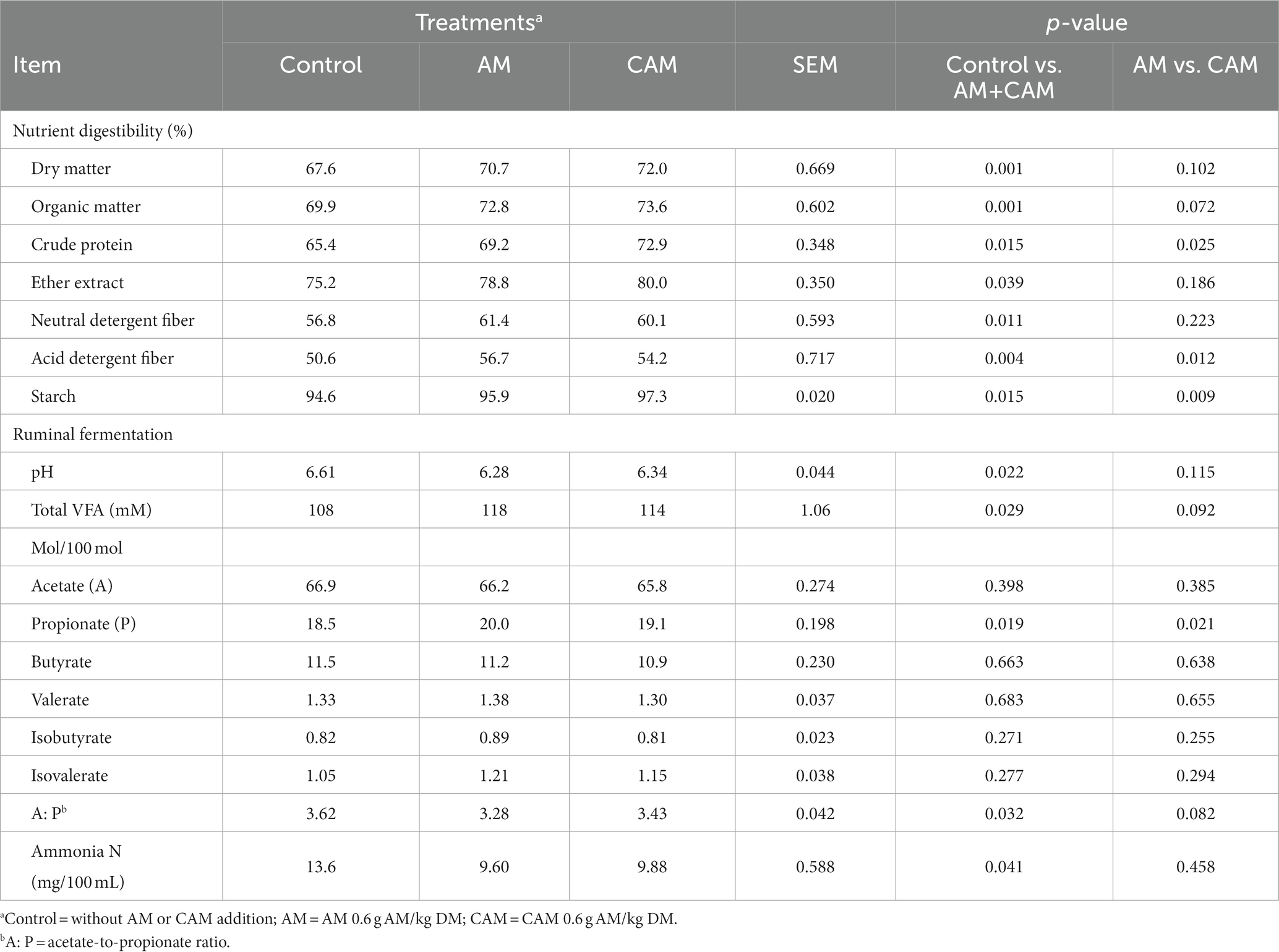
Table 4. Effects of α-amylase (AM) and coated α-amylase (CAM) on nutrient digestion and ruminal fermentation of Holstein bulls.
As shown in Table 4, ruminal pH, acetate-to-propionate ratio, and content of ammonia N decreased (p < 0.05), whereas the total VFA content and molar proportion of propionate increased (p < 0.05). In addition, acetate, butyrate, valerate, isobutyrate, and isovalerate showed unchanged molar proportions for bulls consuming diet supplementation with 0.6 g/kg DM AM (AM or CAM). Bulls receiving CAM addition had similar ruminal pH, total VFA content and acetate, butyrate, valerate, isobutyrate, and isovalerate molar proportions, acetate-to-propionate ratio, and concentration of ammonia N compared with those in the AM group. However, propionate molar proportion was greater (p = 0.021) after CAM supplementation compared with AM addition.
3.3 Ruminal enzymatic activities and microflora
Adding 0.6 g/kg DM AM (AM or CAM) enhanced the carboxymethyl cellulase, cellobiase, pectinase, and AM activities (p < 0.05), but it made no difference to xylanase or protease activity (Table 5). In addition, cellobiase and carboxymethyl cellulase had lower activities (p < 0.05), while xylanase, pectinase, AM, and protease had similar activities for the CAM group than for AM addition.
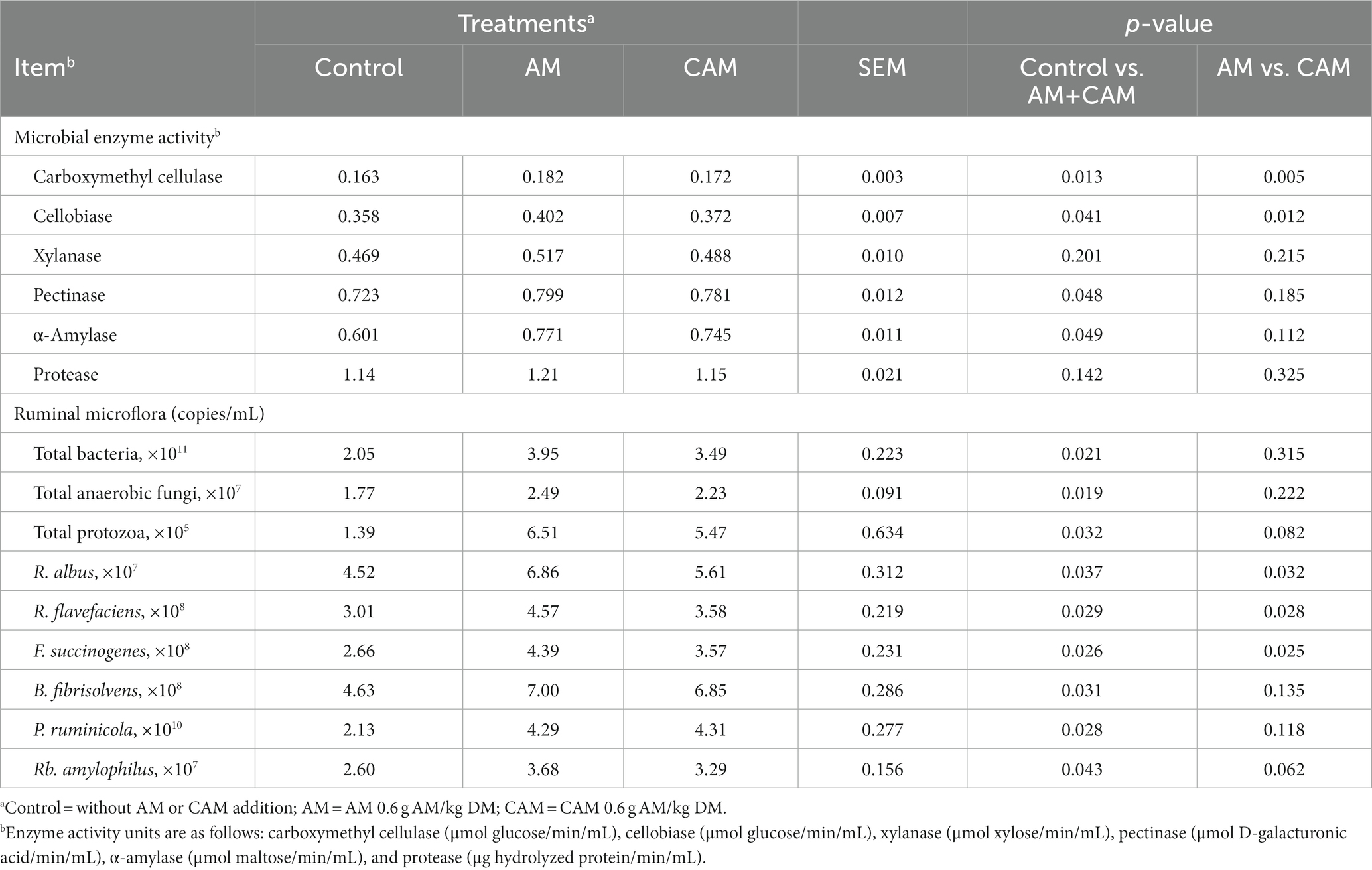
Table 5. Effects of α-amylase (AM) and coated α-amylase (CAM) on ruminal microbial enzyme activities and microflora of Holstein bulls.
The total bacterial, fungal, protozoa, Ruminococcus albus, Ruminococcus flavefaciens, Fibrobacter succinogenes, Butyrivibrio fibrisolvens, Prevotella ruminicola, and Ruminobacter amylophilus populations were increased (p < 0.05) by 0.6 g/kg DM AM (AM or CAM) supplementation. Bulls receiving AM supplementation had greater (p < 0.05) R. albus, R. flavefaciens, and F. succinogenes populations than those receiving CAM addition. Nevertheless, no significant difference was found in total fungal, bacterial, protozoa, P. ruminicola, B. fibrisolvens, and Rb. amylophilus populations for AM and CAM addition.
3.4 Small intestinal enzyme activity
Adding 0.6 g/kg DM AM (AM or CAM) did not affect the activity of lipase in the whole small intestine and the activities of AM and trypsin in the duodenum, but increased (p < 0.05) AM and trypsin activities in the proximal, middle, and distal jejunum and ileum (Table 6). The lipase activity in the whole small intestine and AM and trypsin activities in the duodenum were similar, but AM and trypsin activities in the jejunum and ileum increased (p < 0.05) after CAM supplementation compared with AM supplementation.
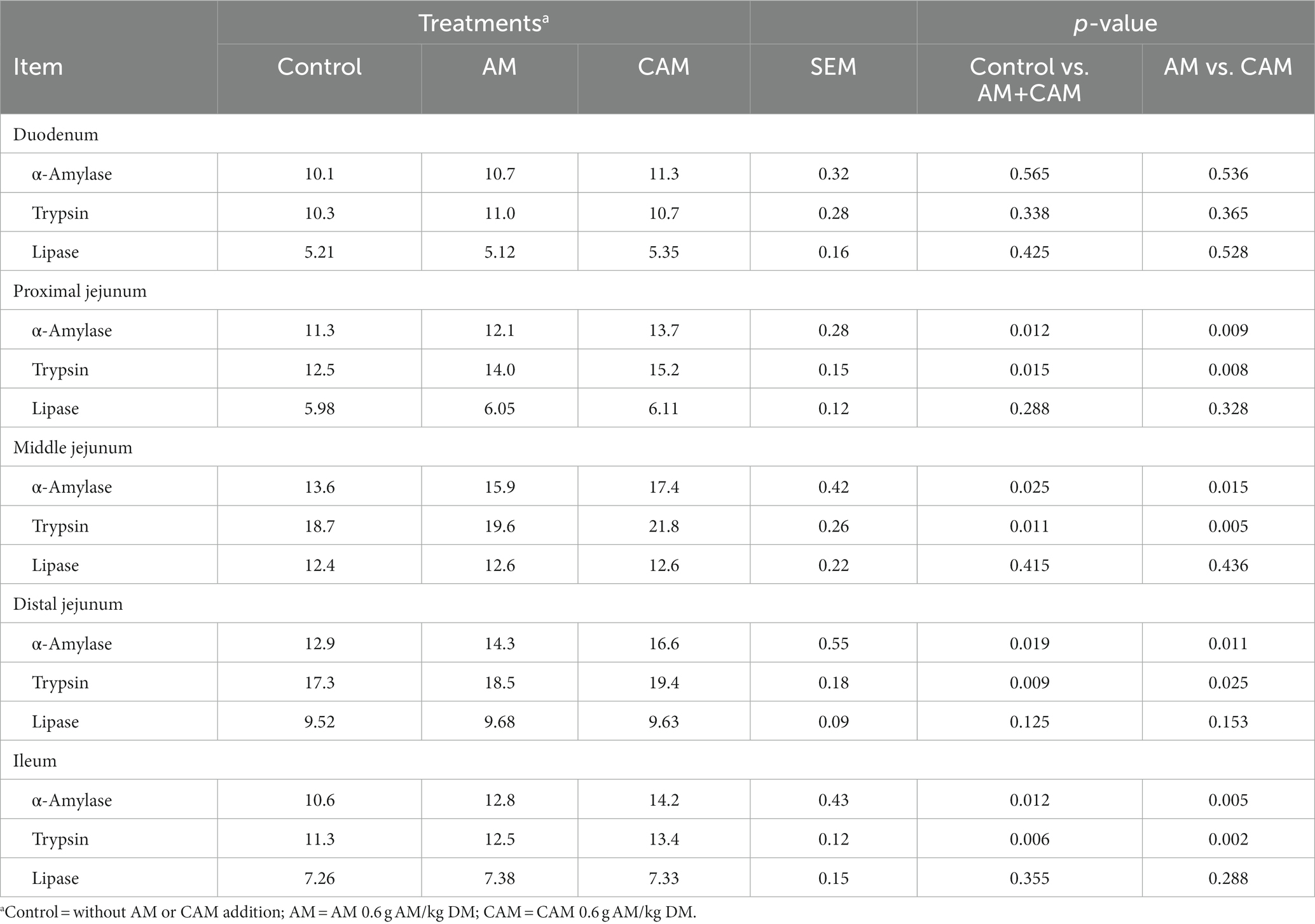
Table 6. Effects of α-amylase (AM) and coated α-amylase (CAM) on enzyme activity in the small intestine contents of Holstein bulls (U/g).
3.5 Blood parameters
Adding 0.6 g/kg DM AM (AM or CAM) elevated (p < 0.05) glucose, albumin, and total protein levels, but it made no difference to insulin, urea N, and lactic acid levels in the blood (Table 7). Bulls receiving CAM supplementation exhibited increased (p < 0.05) glucose, albumin, and total protein levels compared with bulls receiving AM; however, no difference was observed in insulin, urea N, and lactic acid levels between the two groups.
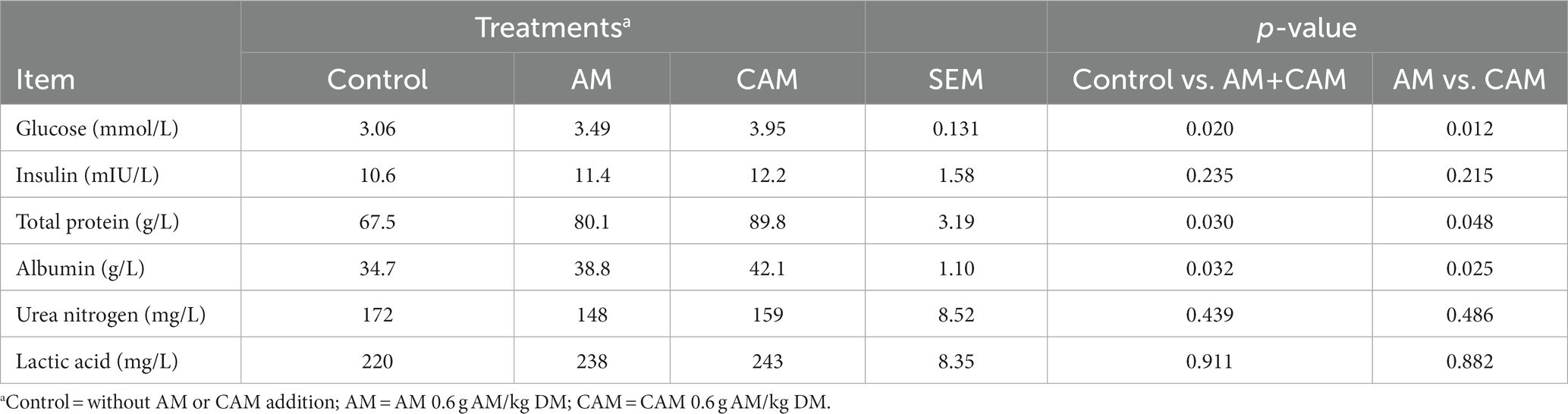
Table 7. Effects of α-amylase (AM) and coated α-amylase (CAM) on blood metabolites of Holstein bulls.
4 Discussion
The present study explored the impact of supplementing AM in bull diets on performance, nutrient digestibility, intestinal digestive enzyme activities, ruminal fermentation, and blood metabolites. The dietary added fat, resulting from the coated CAM, was 0.55 g/kg DM and had a weak impact on the growth performance, nutrient digestion, and rumen fermentation of bulls. Thus, the effects of added fat from CAM were not discussed.
When AM or CAM was supplemented in bull diets, the response of DMI was limited and consistent with the results in finishing steers (30) or dairy cows (8). As a result, the increase in ADG was caused by the increasing nutrient digestibility as well as rumen total VFA level. Furthermore, such increased total-tract starch digestion, rumen propionate molar proportion, and blood glucose concentration indicated that the addition of AM or CAM improved the energy supply efficiency of starch. The energy utilization efficiency of dietary starch was positively associated with the starch degradation rates in the small intestine and rumen (31). Defrain et al. (32) also found that AM supplementation tended to increase blood glucose concentration in postpartum dairy cows. The current results suggested that the dietary addition of AM or CAM at 360 KUN AM/kg DM improved feed utilization efficiency in bulls as evidenced by the increase in FE. Similarly, other studies reported that 110 or 210 KNU AM/kg DM supplementation increased ADG in finishing steers (3), and 300 KNU AM/kg DM addition increased feed efficiency in dairy cows (9, 19). Nevertheless, DiLorenzo et al. (30) discovered that AM addition at 600 KNU/kg DM made no impact on ADG and FE. Such different findings might be associated with different AM addition levels. Research found that production performance increased quadratically with increasing supplementation levels of AM in steers (3) or lactating Holstein cows (6).
In agreement with the results in dairy cows (4, 8, 9), dietary AM or CAM addition increased the total-tract digestibility of nutrients. Nutrient digestibility response was related to the enhanced ruminal carboxymethyl cellulase, cellobiase, pectinase, and AM activities as well as AM and trypsin activities within the small intestine. According to these findings, AM or CAM supplementation improved nutrient digestibility in the small intestine and rumen. Such enhanced small intestine trypsin activity might be associated with an improvement in starch digestibility with AM or CAM addition as starch had a negative effect on intestinal trypsin activity (33). Similarly, studies in weaned pigs and broilers found that exogenous AM addition increased the activities of AM and trypsin in the small intestine (14, 15).
The reduction of rumen pH was observed with AM or CAM addition. The average value of rumen pH for bulls receiving AM or CAM addition was 6.31, which was suitable for nutrient degradation and microbial growth (34, 35). The decreased pH was related to the increased rumen total VFA level (36). Total VFA levels were changed due to positive responses of rumen enzyme activities and microbial populations, indicating the stimulatory effects of exogenous AM on nutrient degradation and microbial growth. The supplementary AM hydrolyzed starch to maltodextrins, which was used as a substrate for microbial growth (3). Similarly, other studies reported that AM addition tended to increase the total VFA concentration in cows fed high-starch diets (8). While a limited response in acetate molar proportion was observed, the acetate concentration increased to 72.3, 78.1, and 75.0 mM for the control, AM, and CAM groups, respectively. The result conformed to the changes of ADF and NDF apparent digestibility, caused by the increase in microbial populations and cellulolytic enzyme activity. Rumen carboxymethyl cellulase, cellobiase, and pectinase are secreted by fungi, protozoa, F. succinogenes, B. fibrisolvens, R. flavefaciens, and R. albus, which are used to hydrolyze fiber to acetate (37). The elevated propionate molar proportion, coupled with the reduced acetate-to-propionate ratio, suggested that dietary AM or CAM addition altered the rumen fermentation pattern for further propionate production. These results conformed to the changes in AM activity as well as amylolytic bacterial populations, including B. fibrisolvens, P. ruminicola, and Rb. amylophilus with AM or CAM addition. Moreover, the increase in AM activity was mainly caused by the positive responses of amylolytic bacteria, showing a synergistic effect of exogenous AM and rumen microbes, as reported by Noziere et al. (8). Similarly, other studies indicated that AM supplementation increased ruminal propionate molar proportion and AM activity in dairy cows (8) and stimulated B. fibrisolvens D1 growth in vitro (7). However, according to Tricarico et al. (6), AM addition increased the butyrate and acetate molar proportions and the acetate-to-propionate ratio. The inconsistent results may be caused due to the differences in diet composition, especially in starch content.
The decreased rumen ammonia N content did not conform to the unaltered protease activity or the elevated protozoa and protein-degrading bacterial populations (B. fibrisolvens, Rb. amylophilus, and P. ruminicola). Given the positive responses of blood concentrations of albumin and total protein and the limited response of blood urea nitrogen, the reduction of rumen ammonia N content might be caused by an elevation of microbial protein production. Moreover, the elevation of the total VFA level increased the carbon skeleton and energy supply to facilitate microbial protein generation. Furthermore, as found by Gado et al. (38), supplementation of exogenous enzyme mixture including AM increased the duodenal microbial N flow in Brown Swiss.
Bulls fed diets added with CAM had greater ADG and FE compared with those receiving AM supply, indicating that AM should be supplied in the form of CAM, as rumen total VFA concentration was similar for both CAM and AM additions. The greater ADG was correlated with the greater total-tract starch and CP digestibility. In addition, it was caused by the AM released from CAM in the intestine. Furthermore, the increased total-tract starch and CP digestibility were related to greater intestinal AM and trypsin activity with CAM addition. The results further showed that increasing starch digestion had a stimulatory effect on trypsin activity and CP digestion in the intestine, as reported in broilers by Jiang et al. (15). The greater CP digestibility contributed to an improvement in protein utilization efficiency, as reflected by the observed greater blood albumin and total protein contents for CAM addition, which can be used as indicators of protein utilization efficiency (39). Rumen propionate concentration for CAM and AM addition was 21.8 and 23.6 mM, respectively; however, the blood glucose concentration was greater for bulls receiving CAM supply. The results showed that supplementation with CAM had a greater improvement in intestinal digestion and energy supply of starch. When dietary starch was digested in the small intestine and rumen, the energy utilization efficiency was 60% and 48%, respectively (40). Bulls receiving CAM addition had lower ADF apparent digestibility, and this was consistent with the results that rumen carboxymethyl cellulase and cellobiase activity and F. succinogenes, R. albus, and R. flavefaciens were lower after CAM supplementation than AM addition. The results suggested that the AM released from CAM in the rumen probably did not support the optimum growth of cellulolytic bacteria. Furthermore, similarly observed AM activity for CAM and AM addition further suggested that the increased AM activity due to AM supply was caused by the stimulatory effects of AM on ruminal microbial growth. Exogenous AM degraded more starch into oligosaccharides, providing more substrates for microbial growth and reproduction, thereby increasing the number of microorganisms (7).
5 Conclusion
The supplementation of 0.6 g/kg DM AM (AM or CAM) promoted ADG and nutrient digestibility of bulls. These positive impacts were mostly caused by the increment in ruminal microbial population and intestinal digestive enzyme activity. The AM should be supplied in the form of CAM, reflected as the greater ADG observed for bulls receiving CAM compared with those consuming AM addition.
Data availability statement
The raw data supporting the conclusions of this article will be made available by the authors, without undue reservation.
Ethics statement
The animal study was approved by Animal Care and Use Committee of Shanxi Agricultural University. The study was conducted in accordance with the local legislation and institutional requirements.
Author contributions
XZ: Formal analysis, Resources, Writing – original draft. FX: Resources, Writing – original draft. KX: Formal analysis, Resources, Writing – original draft. QL: Funding acquisition, Writing – review & editing. GG: Resources, Validation, Writing – original draft. WH: Data curation, Writing – original draft. YZ: Visualization, Writing – original draft. CW: Validation, Writing – review & editing.
Funding
The author(s) declare financial support was received for the research, authorship, and/or publication of this article. This study received funding from the Modern Agricultural Industrial Technology System of Shanxi Province (2023CYJSTX13).
Acknowledgments
The authors want to express their gratitude to the entire personnel of the beef cattle unit at the Shanxi Agriculture University for their assistance in animal care.
Conflict of interest
FX is employed by DSM Nutritional Products Animal Nutrition & Health.
The remaining authors declare that the research was conducted in the absence of any commercial or financial relationships that could be construed as a potential conflict of interest.
Publisher’s note
All claims expressed in this article are solely those of the authors and do not necessarily represent those of their affiliated organizations, or those of the publisher, the editors and the reviewers. Any product that may be evaluated in this article, or claim that may be made by its manufacturer, is not guaranteed or endorsed by the publisher.
References
2. Harmon, DL, and Mcleod, KR. Glucose uptake and regulation by intestinal tissues: implications and whole-body energetics. J Anim Sci. (2001) 79:59–72. doi: 10.2527/jas2001.79E-SupplE59x
3. Tricarico, JM, Abney, MD, Galyean, ML, Rivera, JD, Hanson, KC, McLeod, KR, et al. Effects of a dietary aspergillus oryzae extract containing alpha-amylase activity on performance and carcass characteristics in finishing beef cattle. J Anim Sci. (2007) 85:802–11. doi: 10.2527/jas.2006-427
4. Mccarthy, MM, Engstrom, MA, Azem, E, and Gressley, TF. The effect of an exogenous amylase on performance and total-tract digestibility in lactating dairy cows fed a high-byproduct diet. J Dairy Sci. (2013) 96:3075–84. doi: 10.3168/jds.2012-6045
5. Klingerman, CM, Hu, W, McDonell, EE, DerBedrosian, MC, and Kung, L Jr. An evaluation of exogenous enzymes with amylolytic activity for dairy cows. J Dairy Sci. (2009) 92:1050–9. doi: 10.3168/jds.2008-1339
6. Tricarico, JM, Johnston, JD, Dawson, KA, Hanson, KC, McLeod, KR, and Harmon, DL. The effects of an aspergillus oryzae extract containing alpha-amylase activity on ruminal fermentation and milk production in lactating Holstein cows. Anim Sci. (2005) 81:365–74. doi: 10.1079/ASC50410365
7. Tricarico, JM, Johnston, JD, and Dawson, KA. Dietary supplementation of ruminant diets with an aspergillus oryzae α-amylase. Anim Feed Sci Technol. (2008) 145:136–50. doi: 10.1016/j.anifeedsci.2007.04.017
8. Nozière, P, Steinberg, W, Silberberg, M, and Morgavi, DP. Amylase addition increases starch ruminal digestion in first-lactation cows fed high and low starch diets. J Dairy Sci. (2014) 97:2319–28. doi: 10.3168/jds.2013-7095
9. Gencoglu, H, Shaver, RD, Steinberg, W, Ensink, J, Ferraretto, LF, Bertics, SJ, et al. Effect of feeding a reduced-starch diet with or without amylase addition on lactation performance in dairy cows. J Dairy Sci. (2010) 93:723–32. doi: 10.3168/jds.2009-2673
10. Beauchemin, KA, Morgavi, DP, McAllister, TA, Yang, WZ, Rode, LM, Garnsworthy, PC, et al. The use of enzymes in ruminant diets In: PC Garnsworthy and J Wiseman, editors. Recent advances in animal nutrition. Loughborough, England: Nottingham University Press (2001). 297–322.
11. Morgavi, DP, Beauchemin, KA, Nsereko, VL, Rode, LM, Iwaasa, AD, Yang, WZ, et al. Synergy between the ruminal fibrolytic enzymes and enzymes from Trichoderma longibrachiatum. J Dairy Sci. (2000) 83:1310–21. doi: 10.3168/jds.S0022-0302(00)74997-6
12. Huntington, GB. Starch utilization by ruminants: from basics to the bunk. J Anim Sci. (1997) 75:852–67. doi: 10.2527/1997.753852x
13. Harmon, DL, Yamka, RM, and Elam, NA. Factors affecting intestinal starch digestion in ruminants: a review. Can J Anim Sci. (2004) 84:309–18. doi: 10.4141/A03-077
14. Wang, X, and He, RG. The effect of extrusion processing and enzyme supplementation of corn on blood parameters and amylase activity of weaning piglets. Chin J Vet Sci. (2006) 26:329–32. doi: 10.16303/j.cnki.1005-4545.2006.03.033
15. Jiang, ZY, Zhou, YM, and Wang, T. Influence of exogenous alpha-amylase supplementation on development of digestive organs and intestinal enzyme activities of 21-day-old broilers. Acta Vet Zootechnica Sin. (2007) 38:672–7. doi: 10.3969/j.issn.1006-6314-B.2007.01.007
16. Jung, S., and Vogel, K. Determination of Ronozyme RumiStar alpha-amylase activity in feed and per se samples. Regulatory Report No. 2500706. DSM Nutritional Products Ltd., Basel, Switzerland (2008).
17. Wang, C, Liu, Q, Guo, G, Huo, WJ, Ma, L, Zhang, YL, et al. Effects of rumen-protected folic acid on ruminal fermentation, microbial enzyme activity, cellulolytic bacteria and urinary excretion of purine derivatives in growing beef steers. Anim Feed Sci Technol. (2016) 221:185–94. doi: 10.1016/j.anifeedsci.2016.09.006
18. Bachmann, M, Bochnia, M, Mielenz, N, Spilke, J, Souffrant, WB, and Azem, E, et al. Impact of ɑ-amylase supplementation on energy balance and performance of high-yielding dairy cows on moderate starch feeding. Anim Sci J (2018), 89; 367–376. doi: 10.1111/asj.12939
19. Arturo, SR, Marcos, N, Ronaldo, B, Renata, AN, Nilson, N, Tiago, S, et al. Effect of exogenous amylase on lactation performance of dairy cows fed a high-starch diet. J Dairy Sci. (2018) 101:7199–207. doi: 10.3168/jds.2017-14331
20. NASEM. Nutrient requirements of beef cattle. 8th rev. ed. Washington, DC: National Academies Press (2006).
21. AOAC. Official methods of analysis. 18th ed. Gaithersburg, MD: Association of Official Analytical Chemists International (2006).
22. Van Soest, PJ, Robertson, JB, and Lewis, BA. Methods for dietary fiber, neutral detergent fiber and non-starch polysaccharides in relation to animal nutrition. J Dairy Sci. (1991) 74:3583–97. doi: 10.3168/jds.S0022-0302(91)78551-2
23. Van-Keulen, J, and Young, BA. Evaluation of acid-insoluble ash as a natural marker in ruminant digestibility studies. J Anim Sci. (1977) 44:282–7. doi: 10.2527/jas1977.442282x
24. Hall, MB. Analysis of starch, including maltooligosaccharides, in animal feeds: a comparison of methods and a recommended method for AOAC collaborative study. J AOAC Int. (2009) 92:42–9. doi: 10.1093/jaoac/92.1.42
25. Agarwal, N, Kamra, DN, Chaudhary, LC, Agarwal, I, Sahoo, A, and Pathak, NN. Microbial status and rumen enzyme profile of crossbred calves fed on different microbial feed additives. Lett Appl Microbiol. (2002) 34:329–36. doi: 10.1046/j.1472-765X.2002.01092.x
26. Miller, GL. Use of dinitrosalisylic acid reagent for determination of reducing sugar. Anal Chem. (1959) 31:426–8. doi: 10.1021/ac60147a030
27. Yu, Z, and Morrison, M. Improved extraction of PCR-quality community DNA from digesta and fecal sample. Biotechniques. (2004) 36:808–12. doi: 10.2144/04365ST04
28. Kongmun, P, Wanapat, M, Pakdee, P, and Navanukraw, C. Effect of coconut oil and garlic powder on in vitro fermentation using gas production technique. Livest Sci. (2010) 127:38–44. doi: 10.1016/j.livsci.2009.08.008
30. DiLorenzo, N, Smith, DR, Quinn, MJ, May, ML, Ponce, CH, Steinberg, W, et al. Effects of grain processing and supplementation with exogenous amylase on nutrient digestibility in feedlot diets. Livest Sci. (2011) 137:178–84. doi: 10.1016/j.livsci.2010.11.003
31. Yao, JH, and Shen, J. Rumen degradable starch regulate the gut health and nutrient utilization in ruminants. Feed Industry. (2020) 8:1–7. doi: 10.13302/j.cnki.fi.2020.08.001
32. Defrain, JM, Hippen, AR, Kalscheur, KF, and Tricarico, JM. Effects of dietary alpha-amylase on metabolism and performance of transition dairy cows. J Dairy Sci. (2005) 88:4405–13. doi: 10.3168/jds.S0022-0302(05)73127-1
33. Swanson, KC, Matthews, JC, Woods, CA, and Harmon, DL. Postruminal administration of partially hydrolyzed starch and casein influences pancreatic α-amylase expression in calves. J Nutr. (2022) 132:376–81. doi: 10.1093/jn/132.3.376
34. Kopecny, J, and Wallace, RJ. Cellular location and some properties of proteolytic enzymes of rumen bacteria. Appl Environ Microbiol. (1982) 43:1026–33. doi: 10.1128/aem.43.5.1026-1033.1982
35. Wales, WJ, Kolver, ES, Thorne, PL, and Egan, AR. Diurnal variation in ruminal pH on the digestibility of highly digestible perennial ryegrass during continuous culture fermentation. J Dairy Sci. (2004) 87:1864–71. doi: 10.3168/jds.S0022-0302(04)73344-5
36. Dijkstra, J, Ellis, JL, Kebreab, E, Strathe, AB, Lopez, S, France, J, et al. Ruminal pH regulation and nutritional consequences of low pH. Anim Feed Sci Technol. (2012) 172:22–33. doi: 10.1016/j.anifeedsci.2011.12.005
37. Wang, Y, and Mcallister, TA. Rumen microbes, enzymes and feed digestion-a review. Asian-Aust J Anim Sci. (2002) 15:1659–76. doi: 10.5713/ajas.2002.1659
38. Gado, HM, Salem, AZM, Robinson, PH, and Hassan, M. Influence of exogenous enzymes on nutrient digestibility, extent of ruminal fermentation as well as milk production and composition in dairy cows. Anim Feed Sci Technol. (2009) 154:36–46. doi: 10.1016/j.anifeedsci.2009.07.006
39. Lohakare, JD, Pattanaik, AK, and Khan, SA. Effect of dietary protein levels on the performance, nutrient balances, metabolic profile and thyroid hormones of crossbred calves. Asian-Aust J Anim Sci. (2006) 19:1588–96. doi: 10.5713/ajas.2006.1588
Keywords: α-amylase, growth performance, nutrient digestion, rumen fermentation, bulls
Citation: Zhang X, Xue F, Xu K, Liu Q, Guo G, Huo W, Zhang Y and Wang C (2024) Effects of α-amylase and coated α-amylase supplementation on growth performance, nutrient digestion, and rumen fermentation in Holstein bulls. Front. Vet. Sci. 10:1330616. doi: 10.3389/fvets.2023.1330616
Edited by:
Anusorn Cherdthong, Khon Kaen University, ThailandReviewed by:
Chanadol Supapong, Rajamangala University of Technology Srivijaya, ThailandShahryar Kargar, Shiraz University, Iran
Copyright © 2024 Zhang, Xue, Xu, Liu, Guo, Huo, Zhang and Wang. This is an open-access article distributed under the terms of the Creative Commons Attribution License (CC BY). The use, distribution or reproduction in other forums is permitted, provided the original author(s) and the copyright owner(s) are credited and that the original publication in this journal is cited, in accordance with accepted academic practice. No use, distribution or reproduction is permitted which does not comply with these terms.
*Correspondence: Qiang Liu, liuqiangabc@163.com