- 1College of Veterinary Medicine, South China Agricultural University, Guangzhou, China
- 2Guangdong Technology Research Center for Traditional Chinese Veterinary Medicine and Natural Medicine, Guangzhou, China
- 3International Institute of Traditional Chinese Veterinary Medicine, Guangzhou, China
This study aimed to evaluate the effects of Chinese herbal mixtures (CHMs) on productive performance, egg quality, immune status, anti-apoptosis ability, caecal microbiota, and offspring meconial microbiota in hens. A total of 168 thirty-week-old Wenchang breeder hens were randomly divided into two groups, with each group comprising six replicate pens of fourteen hens. The groups were fed a basal diet (CON group) and a basal diet with 1,000 mg/kg CHMs (CHMs group) for 10 weeks. Our results showed that dietary supplementation with CHMs increased the laying rate, average egg weight, hatch of fertile, and offspring chicks’ weight while concurrently reducing the feed conversion ratio (FCR) and embryo mortality (p < 0.05). The addition of CHMs resulted in significant improvements in various egg quality parameters, including eggshell strength, albumen height, haugh unit, and the content of docosatetraenoic acid (C20:4n-6) in egg yolk (p < 0.05). The supplementation of CHMs had a greater concentration of IgA and IgG while decreasing the content of IL-6 in serum compared with the CON group (p < 0.05). Addition of CHMs to the diet increased the expression of Bcl-2 and IL-4 in liver and ovary, decreased the expression of IL-1β, Bax, and Caspase-8 in jejunum and ovary, and decreased the expression of NF-κB in liver, jejunum, and ovary (p < 0.05). Moreover, dietary CHMs reduced the abundance of Desulfovibrio in caecal microbiota as well as decreased the abundance of Staphylococcaceae_Staphylococcus and Pseudomonadaceae_Pseudomonas in the offspring meconial microbiota (p < 0.05). In conclusion, the CHMs could improve productive parameters by enhancing immune status, anti-apoptosis capacity, and modulating the caecal microbiota of Wenchang breeder hens, as well as maintaining the intestinal health of the offspring chicks.
1 Introduction
A number of announcements from many countries stated that the application of antibiotics in livestock feeds was either strictly restricted or completely prohibited. The production and health state of breeder hens are challenged by more stress factors, including impaired gut function (1) and immune stress (2), which contribute to a downward trend in egg production rate and egg quality. Therefore, it is critical to produce safe feed additives to improve animal health protection. Due to their rich ingredients, Chinese herbal medicines are gaining popularity as dietary supplements for animals (3). Chinese herbal remedies are natural ingredients that have been used in livestock production as safe feed additives to preserve animal health and prevent sickness (4, 5).
A previous study found that Epimedium is a flavonoid-rich herb with a range of potential biological activities, such as antioxidant and antibacterial qualities (6). Angelica roots are primarily composed of chemical constituents such as ferulic acid, Z-ligustilide, butylidenephthalide, and various polysaccharides (7). Among these compounds, ferulic acid has anti-inflammatory and immunomodulatory effects (8). Rehmanniae radix preparata possesses several pharmacological properties, such as antioxidative (9) and those that enhance immunity (10). The primary components of Radix bupleuri extract consist of saikosaponins, flavonoids, polyacetylenes, and lignans (11), as well as having also been utilized as a remedy for immunomodulatory and antioxidative effects in human and animal species (12). The effective active substances in Pericarpium citri reticulatae include flavonoids, total phenols, carotenoids, and polysaccharides (13). The major components of Leonurus japonicus include alkaloids, diterpenes, and flavones (14). The pharmacological activities of Yimucao, such as lipid-lowering, anti-inflammatory, anti-oxidative, immunomodulatory, and anti-cancer activities (14, 15). Paeoniflorin, a major bioactive constituent in Radix paeoniae (16). A study conducted on mice using Paeonia lactiflora extract revealed its antioxidant, immunological, and anti-inflammatory effects (17). Eucommia bark is abundant in bioactive compounds, including lignans, phenolics, and iridoids. It has demonstrated anti-osteoporosis (18), anti-inflammatory, and antioxidant effects (19). L. barbarum polysaccharides are vital bioactive constituents found in L. barbarum, known for their diverse range of bioactivities, including antioxidant and immunomodulatory effects (20), as well as liver protection (21). The polysaccharide called codonopsis pilosula has generated a lot of attention owing to its prebiotic, antioxidant, anti-tumor, immunomodulatory, and anti-fatigue qualities (22). Astragalus polysaccharide has been shown to exhibit antioxidant, immune-regulating, and cardiovascular disease-alleviating effects (23). Chinese herbal medicines contain a variety of active ingredients, including polysaccharides, alkaloids, amino acids, vitamins, and more (24). These components can enhance animal performance by boosting the body’s anti-apoptotic (25), anti-inflammatory, and antioxidant capabilities (26), as well as by modulating microbial composition (27). Previous research has shown that adding herbal mixture (Paeonia lactiflora, licorice, dandelion, and tea polyphenols) can enhance growth performance, boost immunity, and alter the composition of the intestinal microbiota in weaning pigs (28). Certain studies conducted on pigeons have discovered that the addition of AEF (Astragalus, Epimedium, and Ligustrum lucidum) extract to their drinking water resulted in improved intestinal health and enhanced growth performance, especially under conditions of stress pigeons (29). A herbal mixture made up of numerous herbs includes multiple active components that may be more effective biologically than a single herb, as well as having a wider range of applications and better production results (28, 42).
In the animal body, the intestinal microbiota not only governs the digestion and absorption of nutrients (30) but also influences immune homeostasis and chronic diseases (31). It’s worth noting that the gut microbiota can be passed from the mother to her offspring through vertical transmission (32). The transmission of the microbiota is influenced by the microbiome composition of maternal hens’ feces, embryos, and chicks’ ceca. Maternal nutritional strategies play a critical role in regulating the phenotypic traits of animal offspring, and ensuring proper maternal nutrition is essential for early embryonic development (33). Furthermore, the nutrients in eggs are necessary for the early growth and development of chicks. There is a hypothesis that alterations in the maternal diet might affect the nutrient constituents of the eggs, consequently influencing the nutritional requirements and gut microbiota of chicken offspring (34).
To date, the combination of CHMs (Epimedium, Angelica sinensis, Rehmanniae Radix Preparata, Radix Bupleuri, Pericarpium citri reticulatae, Leonurus japonicus, Radix Paeoniae Alba, Eucommia ulmoides, Lycii fructus, Codonopsis pilosula, and Astragalus membranaceus) has not been extensively studied in Wenchang Breeder Hens. Furthermore, the potential influence of maternal supplementation with CHMs on the meconium microbiota of offspring has yet to be fully understood. The objective of this study was to explore the potential benefits of maternal supplementation with CHMs on various aspects, including productive performance, egg quality, immune status, anti-apoptosis ability, and intestinal microbiota, and to investigate the potential influence on the meconial microbiota structure in offspring chicks.
2 Materials and methods
2.1 Preparation of Chinese herbal mixtures
The raw materials of Chinese herbal mixtures were purchased from Hebei Anguo Qi’an Pharmaceutical Co. Ltd. in China, and then the herbs were crushed into fine powder, sifted through an 80-mesh sieve, and mixed thoroughly in proportion and stored at room temperature (25°C) for later use. The CHMs has the following contents per 100 g: Epimedium 10.5 g, Angelica sinensis 10.5 g, Rehmanniae Radix Preparata 5.3 g, Radix Bupleuri 5.3 g, Pericarpium citri reticulatae 5.3 g, Leonurus japonicus 15.8 g, Radix Paeoniae Alba 5.3 g, Eucommia ulmoides 10.5 g, Lycii fructus 10.5 g, Codonopsis pilosula 10.5 g, and Astragalus membranaceus 10.5 g in the ratio 2:2:1:1:1:1:3:1:2:2:2:2:2. The proximate chemical composition of CHMs was determined using AOAC (Association of Official Analytical Chemists) procedures (35), which included crude protein, ether extract, crude fiber, ash, total phosphorus, and calcium. The total polysaccharides in CHMs were determined using the phenol-sulfuric acid method with glucose as the standard (36). The active ingredients and nutrient composition of CHMs are shown in Table 1.
2.2 Animals, diet, and experimental design
All experimental protocols were approved by the Animal Care and Use Committee of the South China Agricultural University (approval number: SYXK 2019-0136, Guangzhou, China).
A total of 168 thirty-week-old Wenchang breeder hens were randomly assigned to two groups, each consisting of six replicates, with fourteen hens in each replicate. These Wenchang breeder hens were sourced from Enping Jilong Industrial Co., Ltd. (Jiangmen, China) and were housed and fed at the Enping Jilong hens production facility. The groups were fed a basal diet (CON group) and a basal diet with 1,000 mg/kg CHMs (CHMs group) for 10 weeks. The photoperiod was maintained at 16 h of light and 8 h of darkness throughout the study. Each hen received 88 g of feed per day to prevent overfeeding and had unlimited access to fresh water. Breeder hens underwent artificial insemination, with 35 μL of pooled semen administered to each bird every 3 days, following the procedure outlined by Liu et al. (37). The ingredient and nutrient composition of the basic diets was shown in Table 2.
2.3 Sample collection
At the end of the feeding period, one hen per replicate (a total of six hens) was randomly selected and subjected to a 12 h fasting period for sample collection. Blood samples were drawn from the wing vein into tubes and allowed to stand at room temperature for 20 min. Subsequently, the tubes were centrifuged at 3000 × g for 10 min at 4°C to collect the serum. These serum samples were stored at −20°C for future analysis. Hens were euthanized by exsanguination and necropsied, and the liver, ovary, and mucosal samples of the middle jejunum were collected immediately and quickly frozen at −80°C for further analysis.
In the final week of the experiment, eggs from each group were collected and subsequently incubated using the same incubator. After hatching, meconium samples were collected from the offspring chicks by gently massaging their abdominal regions. Both cecal digesta and meconium samples were aseptically collected and then stored at −80°C for 16S rRNA analysis.
2.4 Measurement of the laying and hatching performance and egg quality
Laying performance was assessed throughout the experiment by daily recording of egg production, egg weight, and the number of qualified eggs. Laying rate, average egg weight, qualified egg rate, and feed conversion rate were calculated based on the data collected from each replicate.
At the conclusion of the experimental period, a random selection of 10 eggs from each replicate was used to evaluate egg quality. We measured both the horizontal and vertical diameters of these selected eggs using a Vernier caliper (530-101, Mitutoyo, Japan) to calculate the egg shape index. Eggshell strength was determined with an eggshell strength tester (ESG-1, Yaoen, Nanjing, China), while eggshell thickness and weight were measured separately using a Vernier caliper and an electronic balance (FB224, Hengping, Shanghai, China), respectively. Furthermore, egg yolk color, albumen height, and the Haugh unit were assessed using an automatic egg quality tester (EA-01, Orka, Israel).
For hatching performance assessment, all eggs were incubated in the same incubator (Bengbu Sanyuan Incubation Equipment Co., Ltd., Anhui, China) during the experimental period. The incubator maintained a temperature range of 37.2°C to 38.0°C and a relative humidity of 60 to 75%. Eggs were manually turned 12 times a day throughout the incubation period and were lightly sprayed with water once daily, starting from the 15th day of incubation until they hatched (38). The parameters, including fertility, hatching of fertile eggs, hatchability of set eggs, embryo mortality, and the weight of the hatched chicks, were recorded and calculated.
2.5 Measurements of fatty acid content of egg yolk
At the conclusion of the experimental period, five eggs per replicate were randomly selected to assess the fatty acid content in the egg yolk. The fatty acid composition of the samples was determined using gas chromatography. The mean level of each fatty acid was then used to calculate the total content of saturated fatty acids (SFA), monounsaturated fatty acids (MUFA), and polyunsaturated fatty acids (PUFA). Fatty acid measurement services were provided by Waltek Testing Group (Foshan) Co., Ltd.
2.6 Analysis of biochemical components in serum
The activities of caspase-8 (caspase-8; Detection range 83.75 pmol/L ~ 120 pmol/L), the serum concentration of immunoglobulin A (IgA; Detection range 10 μg/mL ~ 320 μg/mL), immunoglobulin G (IgG; Detection range 75 μg/mL ~ 2,400 μg/mL), and interleukin-6 (IL-6; Detection range 1 pg./mL ~ 32 pg./mL) were examined by Enzyme-linked immunosorbent assay (ELISA). All ELISA kits were purchased from Shanghai Enzyme-linked Biotechnology Co., Ltd. (China). Serum concentrations of IgA, IgG, IL-6, and Caspase 8 activity were measured following the instructions of the ELISA kit.
2.7 Real-time quantitative polymerase chain reaction (RT-qPCR)
Total RNA was extracted from tissue samples (liver, ovary, and jejunal mucosa) using the RNA isolator Total RNA Extraction Reagent kit (Vazyme). Subsequently, total cDNA was synthesized using HiScript III RT SuperMix for qPCR (+gDNA wiper) (Vazyme) with 1 μg of total RNA, followed by RT-qPCR amplification using the ChamQ universal SYBR qPCR Master Mix (Vazyme). Gene primers for qRT-PCR were designed by Primer Premier 6.0 software (Premier Biosoft International, United States), synthesized by Tsingke Biotechnology Co., Ltd. (Beijing, China), and used in this study (Table 3). The relative expression of the target gene was analyzed using the 2−ΔΔCt method, normalized against the geometric mean of the expression of β-actin and GAPDH (39).
2.8 Cecal and meconial microbiota analysis in breeder hens and offspring chicks
This trial followed the established procedures of previous researchers (40). To isolate total microbial DNA from the cecal and meconial content samples, we utilized the QIAamp DNA Stool Kit (Qiagen, Valencia, United States). The V3–V4 region of the bacterial 16S rRNA gene was amplified through PCR with the following primers: forward primer 5′-ACTCCTACGGGAGGCAGCA-3′ and reverse primer 5′-GGACTACHVGGGTWTCTAAT-3′. PCR products were then purified using Vazyme VAHTS™ DNA Clean Beads (Vazyme) and quantified with a PicoGreen dsDNA Assay Kit (Invitrogen, United States). Subsequently, we conducted 16S rRNA sequencing on the Illumina Novaseq_PE250 platform (Illumina), with sequencing services provided by Personal Biotechnology Co., Ltd. in Shanghai, China. Data collection and analysis were carried out using the Genescloud Platform1.
2.9 Statistical analysis
All data were initially organized using Excel software and subsequently subjected to statistical analysis with SPSS 20 and GraphPad Prism 7.0 software. For the comparison of data between two groups, a two-tailed unpaired Student’s t-test was employed. The data are presented as the means ± SEM. Significance was determined at p < 0.05.
3 Results
3.1 Effects of dietary CHMs on laying performance of breeder hens
Regarding the laying performance of breeder hens, as presented in Table 4, there was no significant difference was observed in the percentage of qualified eggs between the CON and CHMs groups. However, the CHMs group showed a significant increase in laying rate and average egg weight (p < 0.05) compared to the CON group, along with a decrease in the feed conversion ratio (p < 0.05).
3.2 Effects of dietary CHMs on hatchability of breeder hens
The fertilizing capacity and hatchability results were presented in Table 5. The embryo mortality of the CHMs group was significantly decreased (p < 0.05) than that of the CON group. In addition, supplementing CHMs to the diet led to a significant increase in the hatching of fertile eggs and the weight of offspring chicks compared to the CON group (p < 0.05).
3.3 Effects of dietary CHMs on egg quality of breeder hens
Concerning the egg quality indices shown in Table 6, the CHMs in diet did not affect egg shape index, eggshell thickness, eggshell ratio, egg yolk colour and yolk percentage at 10 weeks. However, CHMs significantly improved eggshell strength (p < 0.05), albumen height (p < 0.01) and haugh unit (p < 0.05).
3.4 Effects of dietary CHMs on fatty acid content in egg yolk
The fatty acid contents of egg yolk from breeder hens fed with CHMs were presented in Table 7. Regarding SFA, compared to the CON group, CHMs significantly increased margaric acid (C17:0) in the yolk (p < 0.05), and we also observed a tendency to increase behenic acid (C22:0, p = 0.086). Furthermore, there was a significant increase in cis-11-Eicosenoic acid (C20:1), a type of monounsaturated fatty acid (MUFA), in the CHMs group (p < 0.05). In the case of polyunsaturated fatty acids (PUFAs), CHMs-supplemented diets resulted in a significant increase in the content of dihomo γ-linolenic acid (C20:3n-6) and docosatetraenoic acid (C20:4n-6) in egg yolk (p < 0.05).
3.5 Effects of dietary CHMs on immunoglobulins and inflammatory factors of breeder hens
To further investigate the impact of CHMs on immune function, we assessed the levels of immunoglobulins and inflammatory factors in breeder hens (Figure 1). The serum concentrations of IgA and IgG in the CHMs group were significantly higher than those in the CON group (p < 0.01 and p < 0.05, respectively). With the introduction of CHMs, the serum levels of the proinflammatory cytokine IL-6 in breeder hens were significantly lower than those in the CON group (p < 0.05). Moreover, dietary supplementation with CHMs led to increased mRNA expression of IL-4 (p < 0.05 and p < 0.05, respectively) and reduced mRNA expression of NF-κB (p < 0.05 and p < 0.01, respectively) in both the liver and ovary. It also resulted in decreased mRNA expression of IL-1β in the ovary of breeder hens compared to the CON group (p < 0.05). In the jejunal mucosa of breeder hens, CHMs feed supplementation significantly decreased the relative mRNA levels of MyD88, NF-κB, and IL-1β (p < 0.05, p < 0.05, and p < 0.01, respectively) compared to the CON group.
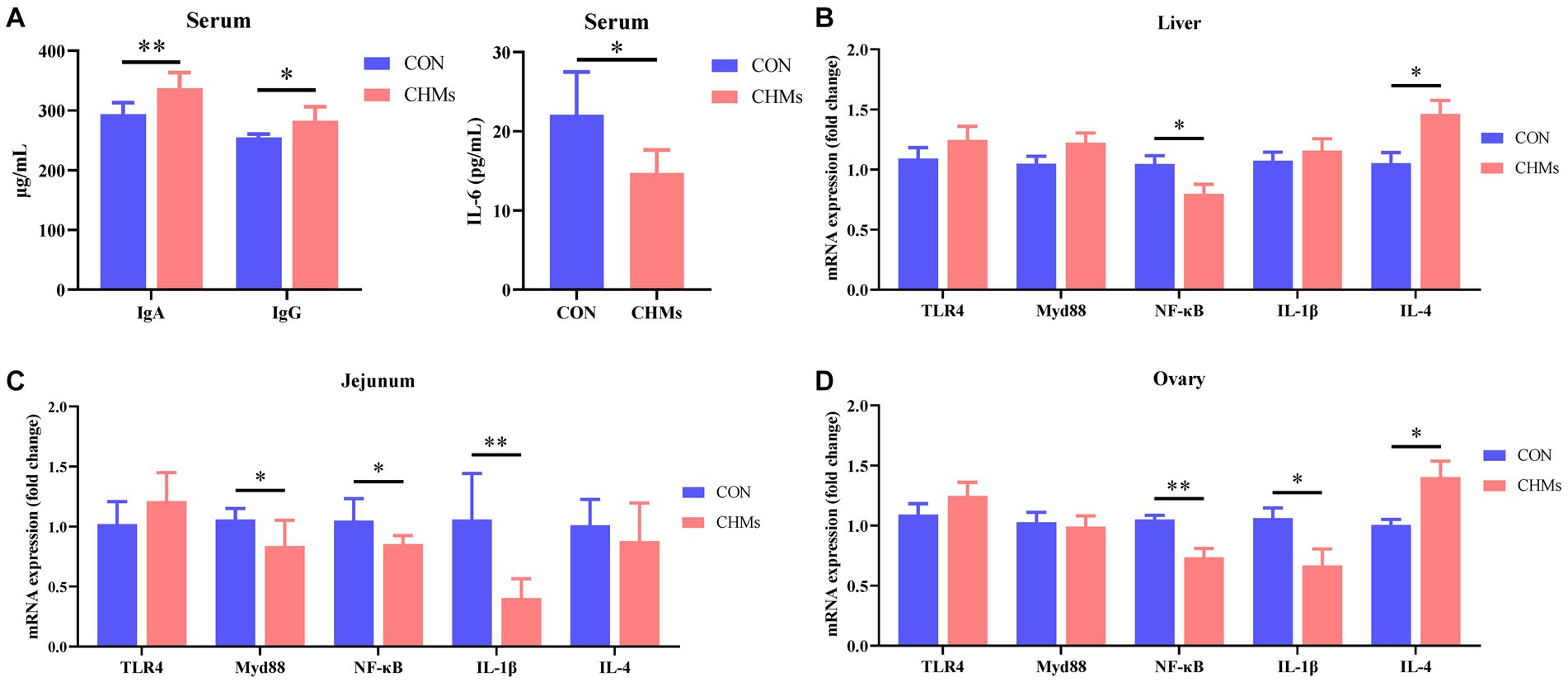
Figure 1. Effects of dietary CHMs on immunoglobulins and inflammatory factors of breeder hens. (A) Serum IgA, IgG; (B) Serum IL-6; (C,D) The expressions of inflammatory genes (TLR4, MyD88, NF-κB, IL-1β, IL-4) in liver, jejunum and ovary, respectively. The data were expressed as mean ± SEM (n = 6). *p < 0.05 and **p < 0.01 vs. CON group.
3.6 Effects of dietary CHMs on apoptosis-related factors of breeder hens
As shown in Figure 2, in the serum of breeder hens fed with dietary CHMs, there was a trend towards lower Caspase-8 concentrations (p = 0.087). Additionally, a significant decrease in the mRNA expression of Caspase-8 was observed in the jejunal mucosa and ovary of the CHMs group (p < 0.05). In comparison to the CON group, the mRNA expression of the anti-apoptotic gene Bcl-2 showed a significant increase (p < 0.05), and the Bax/Bcl-2 ratio was significantly down-regulated (p < 0.05) in the liver of breeder hens in the CHMs group. Additionally, in the jejunal mucosa, the CHMs group exhibited a higher relative mRNA expression of the pro-apoptotic gene Bax compared to the CON group (p < 0.05). In the ovary of breeder hens, the CHMs group had higher expression of Bcl-2 (p < 0.05) and lower expression of Bax (p < 0.05) compared to the CON group, resulting in a highly significant downregulation of the Bax/Bcl-2 ratio (p < 0.01).
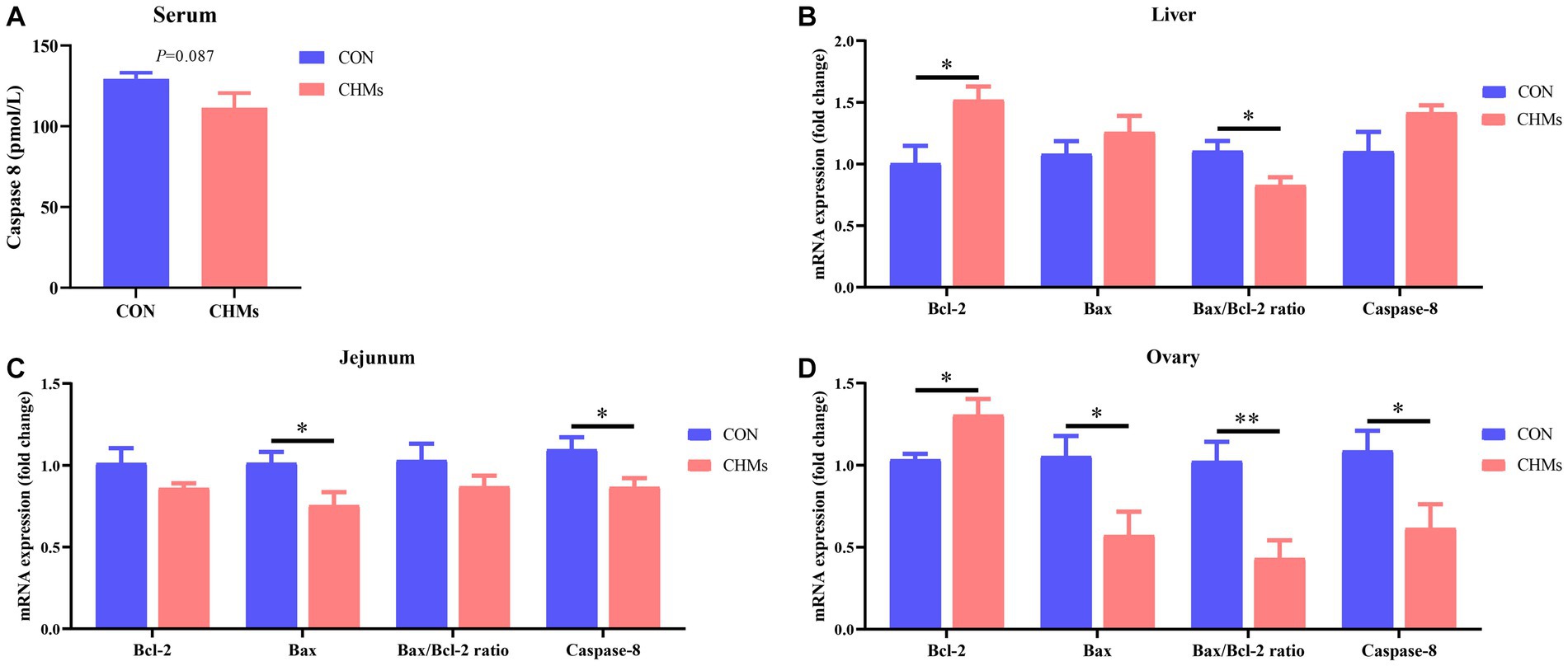
Figure 2. The impact of dietary CHMs on apoptosis-related factors in breeder hens. (A) Serum Caspase-8 concentration; (B–D) Relative mRNA expression of apoptosis-related genes in the live, jejunum and ovary, including Bcl-2, Bax, Bax/Bcl-2 ratio and Caspase-8. The data were expressed as mean ± SEM (n = 6). *p < 0.05 and **p < 0.01 vs. CON group.
3.7 Description of the 16S rRNA gene sequencing data
To explore the effects of dietary supplementation with CHMs on the gut microbiota, we performed 16S rRNA gene sequencing on the cecal content and meconium of breeder hens and offspring chicks, respectively. The diversity of the cecal and meconial microbiota was shown in Figure 3. Venn diagram analysis revealed that 1,699 OTUs were overlapped among the two groups, and 12,341, and 10,151 specific OTUs were unique to the CON and CHMs groups on the cecal microbiota, respectively (Figure 3A). Concerning the meconial microbiota of offspring chicks, a Venn diagram analysis revealed 48 shared OTUs between the CON and CHMs groups. The CON group exhibited 396 unique OTUs, while the CHMs group had 188 unique OTUs (Figure 3B). Based on the PCA (principal component analysis) scatterplot, a clear separation was observed between the cecal samples of the CON and CHMs groups, with no overlapping clusters (Figure 3C). However, there was no obvious separation between the meconium samples of CON and CHMs groups (Figure 3D). Then, we employed Chao 1, Observed_species, Shannon index, and Simpson index to assess the alpha diversity of the cecal and meconial microbiota. The Shannon and Simpson diagrams indicated that the α diversity of the cecal microbiota in breeder hens was visibly lower in the CHMs group than that in the CON group (p < 0.05) (Figure 3E). Furthermore, in comparison to the CON group, the CHMs group exhibited a decrease in the Shannon indices in the meconial microbiota (p < 0.05) (Figure 3F). No significant differences were observed in the Chao 1 and Observed_species indices of the cecal and meconial microbiota between the two groups (p > 0.05).
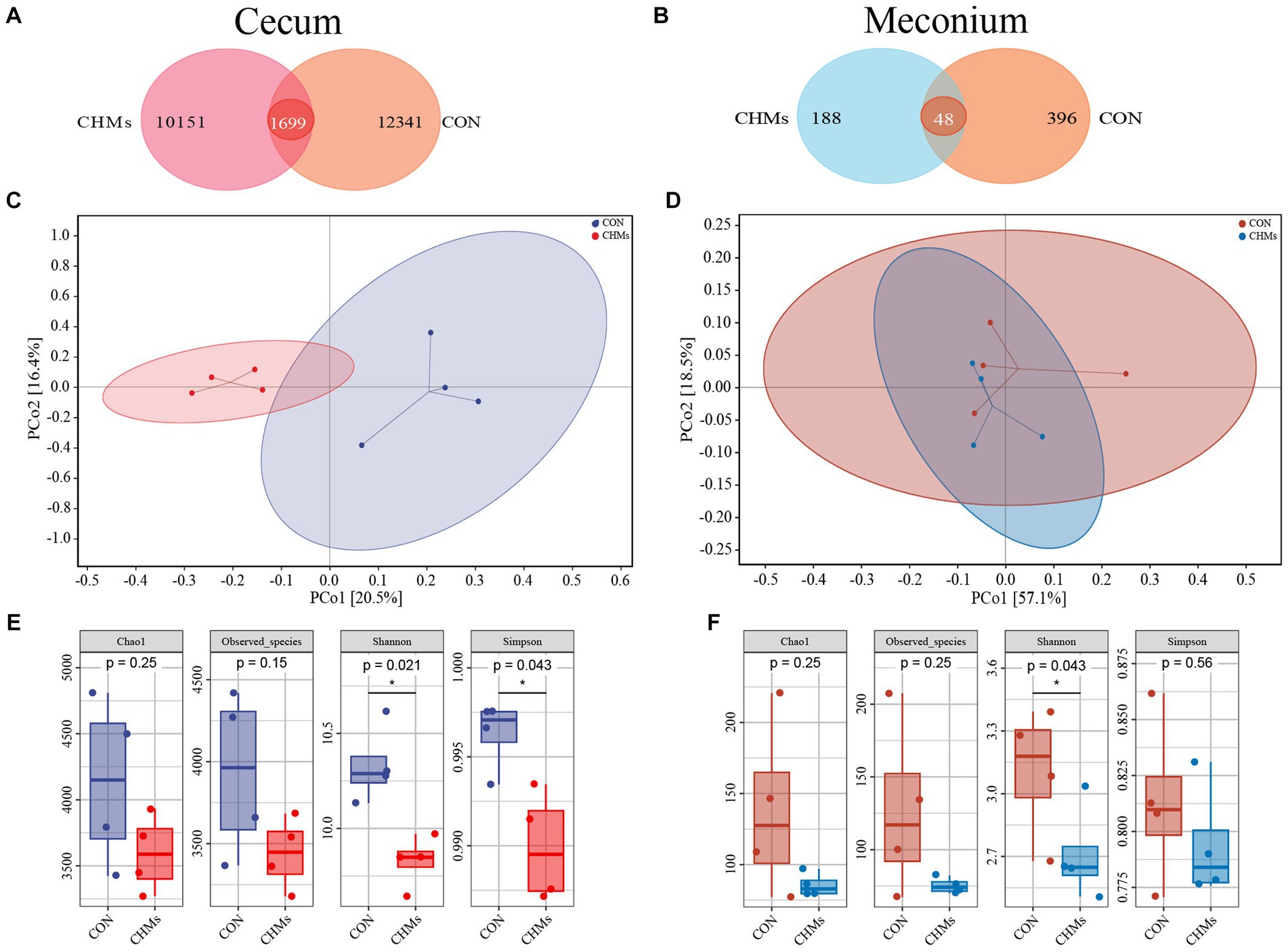
Figure 3. Gut microbiota diversity of breeder hens and offspring chicks. (A,B) Venn diagram of caecal and meconial microbiota on OTUs level. (C,D) PCoA two-dimensional figure based on Bray_Curtis distance analysis. (E,F) Alpha diversity, including Chao 1, Observed_species, Shannon index and Simpson index. The data were expressed as mean ± SEM (n = 4). *p < 0.05, vs. CON group.
3.8 Changes in cecal and meconial microbiota communities
To explore how maternal nutrition interventions, immunity, and microbes may play roles in maternal effects, we analyzed the changes in the composition of the cecal and meconial microbiota communities at the phylum and genus levels (Figure 4). In the cecal microbiota of breeder hens, the three most abundant bacterial phyla were Bacteroidetes, Firmicutes, and Proteobacteria (Figure 4A). The dominant genera included Bacteroides, Oscillospira, and Lactobacillus. At the phylum level, analysis revealed significantly lower abundances of Proteobacteria in the cecal microbiota of breeder hens in the CHMs group compared to the CON group (p < 0.05) (Figure 4C). However, the abundances of Bacteroidetes and Spirochaetes exhibited an upward trend in the CHMs group, respectively (p = 0.079, p = 0.071). Notably, at the genus level of the cecal microbiota, the intervention of CHMs significantly reduced the relative abundance of Desulfovibrio (p < 0.05) (Figure 4E). Compared to the CON groups, there was a decreasing trend in the relative abundance of Bacteroides in the CHMs group (p = 0.071), while the relative abundance of Ruminococcaceae_Ruminococcus showed an upward trend in the CHMs group (p = 0.087).
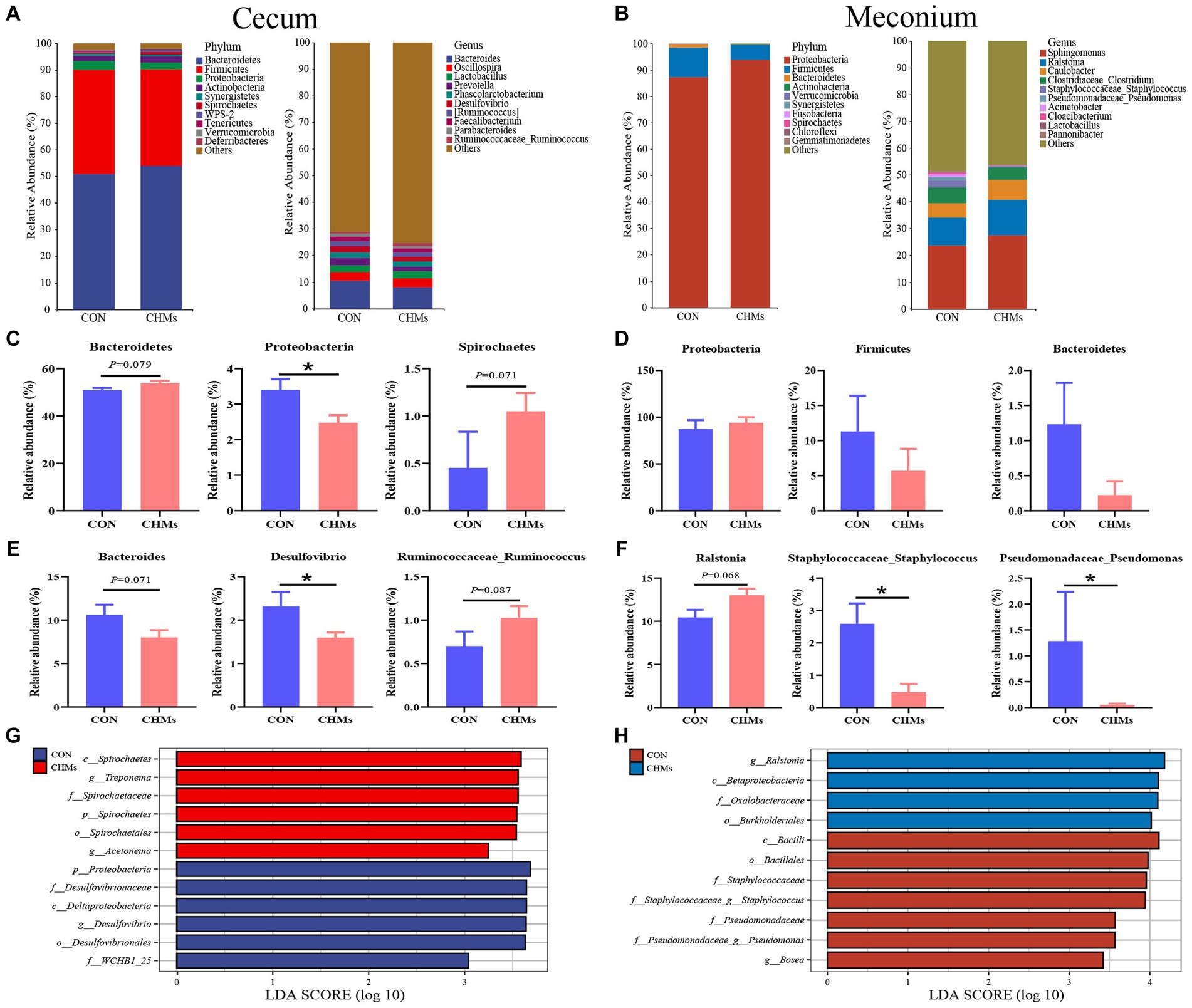
Figure 4. Gut microbiota structure of breeder hens and offspring chicks. (A,B) Relative abundance of caecal and meconial microbiota on phylum and genus level. (C–F) The significant difference genera and phylum between two groups on caecal and meconial microbiota, respectively. (G,H) LEfSE taxonomic cladogram between two groups on caecal and meconial microbiota (LDA > 3). The data were expressed as mean ± SEM (n = 4). *p < 0.05, vs. CON group.
Next, we investigated the composition and structure of the meconial microbiota based on some maternal effects benefiting offspring fitness. In the meconial microbiota of offspring chicks, Firmicutes and Bacteroidetes emerged as the two most abundant bacterial phyla in both the CON and CHMs groups, accounting for over 98% of the total phyla (Figure 4B). At the genus level, Sphingomonas and Ralstonia were the dominant genera in the meconial microbiota, followed by Caulobacter and Clostridiaceae_Clostridium. No significant differences were observed in the bacterial phyla between the two groups (p > 0.05) (Figure 4D). In comparison to the CON group, the CHMs group exhibited a lower relative abundance of Staphylococcaceae_Staphylococcus and Pseudomonadaceae_Pseudomonas (p < 0.05) (Figure 4F). The relative abundance of Ralstonia showed an upward trend in the CHMs group (p = 0.068).
The Linear Discriminant Analysis (LDA) Effect Size (LEfSe) was employed to identify the major microflora with significant differences between the CON group and CHMs group. The LEfSe taxonomic cladogram of cecal microbes revealed that Spirochaetes (class), Treponema (genus), and Spirochaetaceae (family) exhibited higher abundance in the CHMs group (Figure 4G). While the Proteobacteria (phylum), Desulfovibrionaceae (family), and Deltaproteobacteria (class) were the most significant abundance of the CON group. Regarding the LEfSe analysis of meconial microbes, some bacterial groups, including the Ralstonia (family), Betaproteobacteria (class) and Oxalobacteraceae (family) in CHMs group had a higher score, whereas some other bacterial groups, such as Bacilli (class), Bacillales (order), Staphylococcaceae (family) in CON group had a higher score (Figure 4H).
3.9 Correlation analysis of altered intestinal microbe and different indicators
Potential correlations between the meconial and cecal microbiota alterations with many different indicators were evaluated employing a Spearman correlation analysis (Figure 5). The genera Desulfovibrio and the phylum Proteobacteria were negatively correlated with laying performance (r = −0.88, −0.83, p < 0.05) but positively correlated with the level of serum IL-6 (r = 0.95, 0.81, p < 0.05) (Figure 5A). Additionally, the level of serum IgG and laying performance were positively correlated with the phyla Bacteroidetes (r = 0.76, 0.90, p < 0.05). As shown in Figure 5B, the weight of offspring chicks had a connection positively with the abundances of Ralstonia (r = 0.76; p < 0.05), but it was negative correlated with Staphylococcaceae_Staphylococcus and Pseudomonadaceae_Pseudomonas (r = −0.81, −0.74, p < 0.05).
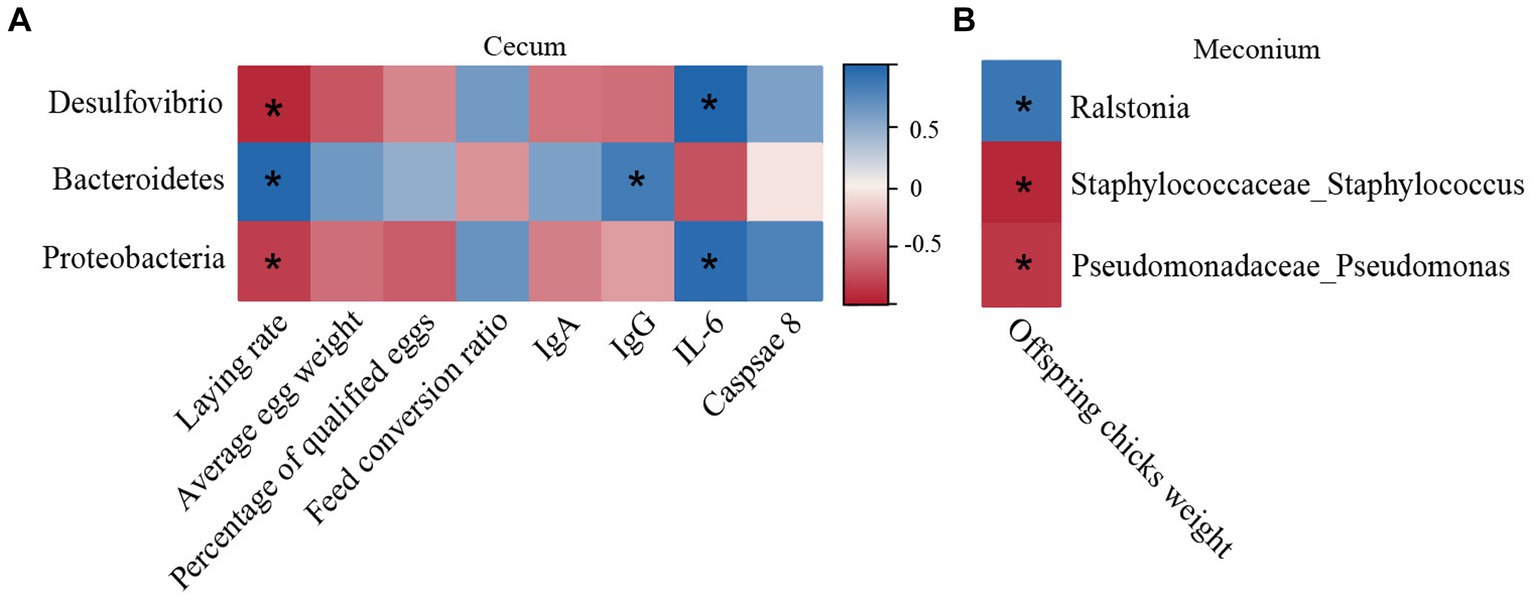
Figure 5. Matrix diagram of the correlation analysis. (A) Spearman correlation analysis among the cecal microbiota, laying performance, and serum indices. (B) Spearman correlation analysis between the meconial microbiota and weight of offspring chicks. Positive and negative correlations are shown by the blue and red matrices, respectively. *p < 0.05 (n = 4).
4 Discussion
In the current experiment with Wenchang Breeder Hens, we found that dietary supplementation with CHMs beneficially affected laying rate and average egg weight, although it reduced feed conversion rate. Despite the different combinations of Chinese herbal mixture (Pine needle and Artemisia annua), Li et al.’s study also reported that CHM increased egg production rates in hens but decreased feed conversion rates, which may be related to the regulation of cholesterol metabolism by CHM (41). Epimedium in CHMs has been shown to have multiple regulatory functions, including sexual dysfunction, hormone metabolism, immune function, and antioxidant properties (6). In our study, these great potentials were confirmed in the growth performance of laying hens. CHMs reduced embryonic mortality while increasing the hatching of fertile eggs and offspring chicks’ weight in this study. Therefore, the increase in egg production and improvement in hatching performance may be attributed to the improved health status of laying hens fed a diet supplemented with CHMs. However, due to the complexity of CHMs and the limitations of this study, further research is still needed to investigate their impact on animals.
Extracts of plant-derived polyphenols and polysaccharides demonstrate strong antioxidant activity, which effectively prevent protein breakdown by lowering oxidation of proteins and lipids (42). Early studies have suggested that the addition of Chinese herbs, specifically a combination of R. astragali, S. miltiorrhiza Bunge, and C. monnieri, led to a significant improvement of the albumen height and haugh unit in eggs (43). In terms of egg quality, dietary supplementation with CHMs significantly increased eggshell strength, albumin height, and haugh unit in this study. The CHMs contain a combination of polysaccharides and polyphenols derived from various Chinese herbs. It is plausible that the cumulative effects of these compounds contributed to the observed improvement in egg quality in this study. In addition, a significant increase was also observed in the content of margaric acid (SFA), cis-11-Eicosenoicacid (MUFA), dihomo γ-linolenic acid (n-6 PUFA), and docosatetraenoic acid (n-6 PUFA) in egg yolk. Previous studies have shown that the lipids in eggs, such as egg yolk oil, phospholipids, and fatty acids, are high-quality nutrients with anti-inflammatory, antioxidant, cardiovascular protection, memory improvement, and physiological homeostasis functions (44). Particularly, polyunsaturated fatty acids (PUFAs) are regarded as one of the most crucial components that influence the normal development and physiological functions of the body (45). Numerous studies have shown that C20:3n-6, a long-chain polyunsaturated fat, is considered one of the most important components of breast milk (46). The high level of C20:3n-6 makes eggs from hens treated with CHMs a better choice for pregnant women and infants. In general, the intervention of CHMs improved the external and internal quality of eggs, which is a benefit for subsequent production or marketing.
Numerous clinical studies have found that CHMs have pleiotropic mechanisms of action in reproductive tract diseases, regulating the expression of estrogen receptors, reactive oxygen species, inflammatory factors, and apoptosis-related proteins (47). Inflammatory damage and cell apoptosis caused by reproductive tract infections are common causes of reproductive dysfunction in laying hens (48, 49). The reproductive function of hens is closely related to the levels of inflammatory factors and apoptosis-related genes in the body, which is particularly important for layer production. Li et al.’s study found that Shaoyao decoction improves tissue fibrosis and treats radiation enteritis by reducing inflammation and apoptotic responses (50). The findings of this study suggest that CHMs tend to decrease the activity of caspase-8 enzyme in hens’ serum. The relative expression of genes for apoptosis-related proteins in liver, jejunum, and ovarian tissues was also determined in our study. CHMs regulated the expression of mRNAs including Bax, Bcl-2, Caspase-8 in the above three tissues and activated the anti-apoptotic responses in hens. In a study conducted by Yan et al., lotus leaf extract was observed to upregulate the mRNA expression levels of the Caspase-8, Caspase-9, and Caspase-10 in laying hens. Simultaneously, it downregulated the mRNA expression levels of Caspase-3 and Caspase-7. This regulation of caspases had an impact on cell apoptosis and immune function, ultimately resulting in an improvement in salpingitis among laying hens (51). CHMs diet could regulate the ability of ovarian cell apoptosis in breeder hens.
In recent years, the immunomodulatory, anti-inflammatory, and anti-apoptotic effects of Chinese medicines have been widely studied. In our study, CHMs treatment increased the concentrations of serum immunoglobulins IgA and IgG in hens, which are considered to potent inducers for promoting neutrophils against pathogens (52). In addition, CHMs decreased the concentration of the pro-inflammatory factor IL-6 in hens’ serum. The relative expression of genes for inflammation-related factors in liver, jejunum, and ovarian tissues was also determined in our study. CHMs regulated the expression of mRNAs including NF-κB, IL-4, IL-1β, and MyD88 in the above three tissues and activated the anti-inflammatory responses in hens. IgA, IgM, and IgG are the major serum immunoglobulins of the body as well as their concentrations in plasma have a significant relationship with immunity in laying hens (53). The interleukin (IL) family plays a crucial role in regulating inflammation, with IL-1β and IL-6 assuming vital roles in maintaining intestinal structural integrity, modulating the intestinal immune systems, and being involved with various apoptotic and inflammatory responses (54). Chinese herbal medicines (CHMs) contain numerous bioactive substances, including polysaccharides, volatile oils, flavonoids, alkaloids, and glycosides, which can enhance the body’s immunity (28). Therefore, we hypothesize that CHMs possess potent anti-inflammatory properties and the ability to regulate apoptosis protein-related genes, ultimately bolstering the immunity and health status of breeder hens.
It is widely known that the cecum of chickens contains a rich gut microbiota. Several studies have shown that herbal supplements or extracts have a beneficial effect on the composition of the intestinal microbiota in chickens. Evidently, the modulation of gut microbial structure by CHMs is intricately linked to the enhancement of growth performance, reproductive performance, and immune function in the organism (55, 56). The study by Song et al. demonstrated that dietary supplementation with astragalus polysaccharides significantly decreased the population of Clostridium perfringens in the cecum of broilers with necrotizing enteritis (57). In our study, CHMs altered the structure of the cecum microbiota in hens. Despite a decrease in the alpha diversity of the microbiota, there was an increase in beneficial bacteria (like Ruminococcus) and a decrease in pathogenic bacteria (like Desulfovibrio) in the cecum of hens. In the cecum of hens treated with CHMs, there was a significant decrease in Desulfovibrio (58), which is considered an intestinal noxious bacterium, while Ruminococcus (59), known for its capacity to degrade complex polysaccharides and transform them into a range of nutrients for their hosts, exhibited an inclination to increase. The fermented Chinese herbal compound containing Bupleurum chinense can reduce the abundance of Desulfovibrio in broiler cecum (27). Peng et al.’s (60) findings confirmed that supplementation of the diet with Eucommia ulmoides extract might simulate an immune response by altering gut microbial populations. On the one hand, this could be owing to the key bioactive ingredients of CHMs, such as polysaccharides, which have anti-inflammatory and immunomodulatory actions that enhance gut bacterial abundance. Another hand, and it could be the outcome of interactions between gut microbiota after CHMs intake.
Prior to egg laying, all the necessary components to support the growth of the offspring must be completely passed from the mother to the egg. We infer that CHMs produce favorable adaptive maternal effects to ensure the growth and intestinal health of the offspring by enhancing maternal immunity and anti-apoptotic effects as well as maintaining intestinal health, but their maternal effects in the offspring need to be investigated in depth. The immunity and health of the hen during laying influence the safety and development of the offspring embryo (61). Ding et al. (62) revealed that maternal microbial colonization occurs throughout embryonic development and claimed that the microbes present in the egg are transported from the oviduct during egg formation (despite the fact that they related the maternal fecal microbiota to the embryonic microbiota). Furthermore, consistent with the findings of the present study, previous research has demonstrated that meconium samples were predominantly composed of Proteobacteria and Firmicutes, with a lesser abundance of the phyla Actinobacteriota and Bacteroidota (63). Staphylococcus and Pseudomonas are both common foodborne pathogens and the most common causative agents of human septicemia (64, 65). The CHMs treatment led to a significant reduction in the relative abundance of Staphylococcus and Pseudomonas in the meconium of offspring. Gut microbes exert a profound impact on various physiological functions of the host, encompassing aspects from energy metabolism to immune responses, especially those related to the gut system of immunity (66). The gut microbes of newly hatched chicks were sourced from the maternal magnum, which was transferred into egg whites for colonization of the embryonic gut (61). Before long, environmental, genetic, and other factors shape the unique gut microbiota of offspring chicks. Current studies on Sphingomonas and Ralstonia have focused on botany and environmental soils since it can help plants to combat stressful environments (67, 68), but its role in the poultry gut microbiota remains to be investigated.
We performed Spearman correlation analysis of the significantly changed gut microbiota with phenotypic and physiological indicators. We observed a significant correlation between the hen’s egg production rate and the hen’s cecum microbiota, as well as body weight and meconium bacteria of offspring chicks. The reduction of harmful bacteria by the intervention of CHMs was negatively correlated with laying rate and chick body weight, which is consistent with the widely prevailing view (42). Therefore, we concluded that the addition of CHMs to diets maintains hen health by modulating the structure of the gut microbiota and reducing the relative abundance of opportunistic pathogens. Meanwhile, the resulting adaptive maternal effect maintains the initial weight and intestinal health of the offspring.
5 Conclusion
To summarize, this study showed that the addition of CHMs to laying hen diets can improve laying rate, hatchability, and eggs’ external and nutritional quality. We suggest that these positive effects are related to the expression of mRNAs for immunoglobulins, apoptosis-related proteins, and inflammatory factors in hens, along with changes in the gut microbiota. Notably, CHMs significantly reduced the relative abundance of opportunistic pathogens in the hens’ cecum and offspring’s meconium, which may account for the improved performance and egg quality, immunity, anti-apoptosis level, and anti-inflammatory level as well as further drive the maternal effects of Wenchang breeders by CHMs. In conclusion, these findings suggest that CHMs are a valuable feed additive for breeder hens.
Data availability statement
The datasets presented in this study can be found in online repositories. The names of the repository/repositories and accession number can be found below: https://www.ncbi.nlm.nih.gov/sra/PRJNA1033218.
Ethics statement
The animal studies were approved by Animal Care and Use Committee of the South China Agricultural University. The studies were conducted in accordance with the local legislation and institutional requirements. Written informed consent was obtained from the owners for the participation of their animals in this study.
Author contributions
ML: Data curation, Formal analysis, Investigation, Methodology, Writing – original draft, Writing – review & editing. JH: Conceptualization, Visualization, Writing – original draft. MM: Investigation, Methodology, Writing – review & editing. GH: Investigation, Methodology, Software, Writing – review & editing. YZ: Data curation, Investigation, Writing – review & editing. YD: Investigation, Methodology, Software, Writing – review & editing. QQ: Project administration, Supervision, Writing – review & editing. WL: Conceptualization, Project administration, Supervision, Writing – review & editing. SG: Conceptualization, Funding acquisition, Writing – review & editing.
Funding
The author(s) declare financial support was received for the research, authorship, and/or publication of this article. This work was supported by grants from the National Key Research and Development Program (2022YFD1801103), Hainan Province’s Key Research and Development Project (ZDYF2023XDNY067) and “Quancheng Scholars” Construction Project (2021).
Conflict of interest
The authors declare that the research was conducted in the absence of any commercial or financial relationships that could be construed as a potential conflict of interest.
Publisher’s note
All claims expressed in this article are solely those of the authors and do not necessarily represent those of their affiliated organizations, or those of the publisher, the editors and the reviewers. Any product that may be evaluated in this article, or claim that may be made by its manufacturer, is not guaranteed or endorsed by the publisher.
Abbreviations
CHMs, Chinese herbal mixtures; FCR, feed conversion ratio; SFA, saturated fatty acids; MUFA, monounsaturated fatty acids; PUFA, polyunsaturated fatty acids; IgA, immunoglobulin A; IgM, immunoglobulin G; IL-6, interleukin-6; Bax, BCL2-Associated X; BCL-2, B-cell lymphoma-2; GAPDH, glyceraldehyde-3-phosphate dehydrogenase; IL-1β, interleukin-1β IFN-γ, interferon-γ MyD88, Myeloid Differentiation Factor 88; NF-κB, Nuclear factor-kappa B; TLR4, Toll-like receptor 4.
Footnotes
References
1. Shao, Y, Wang, Y, Yuan, Y, and Xie, Y. A systematic review on antibiotics misuse in livestock and aquaculture and regulation implications in China. Sci Total Environ. (2021) 798:149205. doi: 10.1016/j.scitotenv.2021.149205
2. Matur, E, Akyazi, I, Eraslan, E, Ergul, EE, Eseceli, H, Keten, M, et al. The effects of environmental enrichment and transport stress on the weights of lymphoid organs, cell-mediated immune response, heterophil functions and antibody production in laying hens. Anim Sci J. (2016) 87:284–92. doi: 10.1111/asj.12411
3. Wang, X, Wang, Y, Mao, Y, Hu, A, Xu, T, Yang, Y, et al. The beneficial effects of traditional Chinese medicine on antioxidative status and inflammatory cytokines expression in the liver of piglets. Front Vet Sci. (2022) 9:937745. doi: 10.3389/fvets.2022.937745
4. Gong, J, Yin, F, Hou, Y, and Yin, Y. Review: Chinese herbs as alternatives to antibiotics in feed for swine and poultry production: potential and challenges in application. Can J Anim Sci. (2014) 94:223–41. doi: 10.4141/CJAS2013-144
5. Guo, S, Liu, L, Lei, J, Qu, X, He, C, Tang, S, et al. Modulation of intestinal morphology and microbiota by dietary macleaya cordata extract supplementation in xuefeng black-boned chicken. Animal. (2021) 15:100399. doi: 10.1016/j.animal.2021.100399
6. Ma, H, He, X, Yang, Y, Li, M, Hao, D, and Jia, Z. The genus epimedium: an ethnopharmacological and phytochemical review. J Ethnopharmacol. (2011) 134:519–41. doi: 10.1016/j.jep.2011.01.001
7. Chao, WW, and Lin, BF. Bioactivities of major constituents isolated from angelica sinensis (danggui). Chin Med. (2011) 6:29. doi: 10.1186/1749-8546-6-29
8. Zhai, Y, Wang, T, Fu, Y, Yu, T, Ding, Y, and Nie, H. Ferulic acid: a review of pharmacology, toxicology, and therapeutic effects on pulmonary diseases. Int J Mol Sci. (2023) 24:24. doi: 10.3390/ijms24098011
9. Liu, CY, Ma, RF, Wang, LL, Zhu, RY, Liu, HX, Guo, YB, et al. Rehmanniae Radix in osteoporosis: a review of traditional Chinese medicinal uses, phytochemistry, pharmacokinetics and pharmacology. J Ethnopharmacol. (2017) 198:351–62. doi: 10.1016/j.jep.2017.01.021
10. Zhou, Y, Wang, S, Feng, W, Zhang, Z, and Li, H. Structural characterization and immunomodulatory activities of two polysaccharides from rehmanniae radix praeparata. Int J Biol Macromol. (2021) 186:385–95. doi: 10.1016/j.ijbiomac.2021.06.100
11. Yuan, B, Yang, R, Ma, Y, Zhou, S, Zhang, X, and Liu, Y. A systematic review of the active saikosaponins and extracts isolated from radix bupleuri and their applications. Pharm Biol. (2017) 55:620–35. doi: 10.1080/13880209.2016.1262433
12. Yang, F, Dong, X, Yin, X, Wang, W, You, L, and Ni, J. Radix bupleuri: a review of traditional uses, botany, phytochemistry, pharmacology, and toxicology. Biomed Res Int. (2017) 2017:7597596. doi: 10.1155/2017/7597596
13. Chen, XM, Tait, AR, and Kitts, DD. Flavonoid composition of orange peel and its association with antioxidant and anti-inflammatory activities. Food Chem. (2017) 218:15–21. doi: 10.1016/j.foodchem.2016.09.016
14. Shang, X, Pan, H, Wang, X, He, H, and Li, M. Leonurus japonicus houtt: ethnopharmacology, phytochemistry and pharmacology of an important traditional Chinese medicine. J Ethnopharmacol. (2014) 152:14–32. doi: 10.1016/j.jep.2013.12.052
15. Bi, SJ, Yue, SJ, Bai, X, Feng, LM, Xu, DQ, Fu, RJ, et al. Danggui-yimucao herb pair can protect mice from the immune imbalance caused by medical abortion and stabilize the level of serum metabolites. Front Pharmacol. (2021) 12:754125. doi: 10.3389/fphar.2021.754125
16. Tan, YQ, Chen, HW, Li, J, and Wu, QJ. Efficacy, chemical constituents, and pharmacological actions of radix paeoniae rubra and radix paeoniae alba. Front Pharmacol. (2020) 11:1054. doi: 10.3389/fphar.2020.01054
17. Su-Hong, C, Qi, C, Bo, L, Jian-Li, G, Jie, S, and Gui-Yuan, L. Antihypertensive effect of radix paeoniae alba in spontaneously hypertensive rats and excessive alcohol intake and high fat diet induced hypertensive rats. Evid Based Complement Alternat Med. (2015) 2015:731237. doi: 10.1155/2015/731237
18. He, X, Wang, J, Li, M, Hao, D, Yang, Y, Zhang, C, et al. Eucommia ulmoides oliv: Ethnopharmacology, phytochemistry and pharmacology of an important traditional Chinese medicine. J Ethnopharmacol. (2014) 151:78–92. doi: 10.1016/j.jep.2013.11.023
19. Kwon, SH, Ma, SX, Hwang, JY, Ko, YH, Seo, JY, Lee, BR, et al. The anti-inflammatory activity of eucommia ulmoides oliv. Bark. Involves nf-kappab suppression and nrf2-dependent ho-1 induction in bv-2 microglial cells. Biomol Ther (Seoul). (2016) 24:268–82. doi: 10.4062/biomolther.2015.150
20. Feng, L, Xiao, X, Liu, J, Wang, J, Zhang, N, Bing, T, et al. Immunomodulatory effects of lycium barbarum polysaccharide extract and its uptake behaviors at the cellular level. Molecules. (2020) 25:25. doi: 10.3390/molecules25061351
21. Jia, W, Li, J, Yan, H, et al. Lycium barbarum polysaccharide attenuates high-fat diet-induced hepatic steatosis by up-regulating sirt1 expression and deacetylase activity. Sci Rep. (2016) 6:209. doi: 10.1038/srep36209
22. Zou, YF, Zhang, YY, Paulsen, BS, Fu, YP, and Yin, ZQ. Prospects of codonopsis pilosula polysaccharides: structural features and bioactivities diversity. Trends Food Sci Technol. (2020) 103:1. doi: 10.1016/j.tifs.2020.06.012
23. Gong, A, Duan, R, Wang, HY, Kong, XP, Dong, T, Tsim, K, et al. Evaluation of the pharmaceutical properties and value of astragali radix. Medicines. (2018) 5:5. doi: 10.3390/medicines5020046
24. Kuralkar, P, and Kuralkar, SV. Role of herbal products in animal production - an updated review. J Ethnopharmacol. (2021) 278:114246. doi: 10.1016/j.jep.2021.114246
25. Ibtisham, F, Nawab, A, Niu, Y, Wang, Z, Wu, J, Xiao, M, et al. The effect of ginger powder and Chinese herbal medicine on production performance, serum metabolites and antioxidant status of laying hens under heat-stress condition. J Therm Biol. (2019) 81:20–4. doi: 10.1016/j.jtherbio.2019.02.002
26. Xie, T, Bai, SP, Zhang, KY, Ding, XM, Wang, JP, Zeng, QF, et al. Effects of lonicera confusa and astragali radix extracts supplementation on egg production performance, egg quality, sensory evaluation, and antioxidative parameters of laying hens during the late laying period. Poult Sci. (2019) 98:4838–47. doi: 10.3382/ps/pez219
27. Zhou, X, Li, S, Jiang, Y, Deng, J, Yang, C, Kang, L, et al. Use of fermented Chinese medicine residues as a feed additive and effects on growth performance, meat quality, and intestinal health of broilers. Front Vet Sci. (2023) 10:1157935. doi: 10.3389/fvets.2023.1157935
28. Xu, Q, Cheng, M, Jiang, R, Zhao, X, Zhu, J, Liu, M, et al. Effects of dietary supplement with a Chinese herbal mixture on growth performance, antioxidant capacity, and gut microbiota in weaned pigs. Front Vet Sci. (2022) 9:971647. doi: 10.3389/fvets.2022.971647
29. Zhang, X, Zhou, S, Liang, Y, Xie, G, Zhu, M, Wang, Z, et al. Effects of astragalus, epimedium, and fructus ligustri lucidi extractive on antioxidant capacity, production performance, and immune mechanism of breeding pigeons under stress. Poult Sci. (2023) 102:102350. doi: 10.1016/j.psj.2022.102350
30. Chang, EB, and Martinez-Guryn, K. Small intestinal microbiota: the neglected stepchild needed for fat digestion and absorption. Gut Microbes. (2019) 10:235–40. doi: 10.1080/19490976.2018.1502539
31. Gao, J, Xu, K, Liu, H, Liu, G, Bai, M, Peng, C, et al. Impact of the gut microbiota on intestinal immunity mediated by tryptophan metabolism. Front Cell Infect Microbiol. (2018) 8:13. doi: 10.3389/fcimb.2018.00013
32. Lee, S, La, TM, Lee, HJ, Choi, IS, Song, CS, Park, SY, et al. Characterization of microbial communities in the chicken oviduct and the origin of chicken embryo gut microbiota. Sci Rep. (2019) 9:6838. doi: 10.1038/s41598-019-43280-w
33. Wang, C, Wei, S, Liu, B, Wang, F, Lu, Z, Jin, M, et al. Maternal consumption of a fermented diet protects offspring against intestinal inflammation by regulating the gut microbiota. Gut Microbes. (2022) 14:2057779. doi: 10.1080/19490976.2022.2057779
34. Yang, J, Zhang, KY, Bai, SP, Wang, JP, Zeng, QF, Peng, HW, et al. The impacts of egg storage time and maternal dietary vitamin e on the growth performance and antioxidant capacity of progeny chicks. Poult Sci. (2021) 100:101142. doi: 10.1016/j.psj.2021.101142
36. Chen, L, and Huang, G. Antioxidant activities of sulfated pumpkin polysaccharides. Int J Biol Macromol. (2019) 126:743–6. doi: 10.1016/j.ijbiomac.2018.12.261
37. Liu, X, Peng, C, Qu, X, Guo, S, Chen, JF, He, C, et al. Effects of bacillus subtilis c-3102 on production, hatching performance, egg quality, serum antioxidant capacity and immune response of laying breeders. J Anim Physiol Anim Nutr. (2019) 103:182–90. doi: 10.1111/jpn.13022
38. Xia, WG, Chen, W, Abouelezz, K, Ruan, D, Wang, S, Zhang, YN, et al. The effects of dietary se on productive and reproductive performance, tibial quality, and antioxidant capacity in laying duck breeders. Poult Sci. (2020) 99:3971–8. doi: 10.1016/j.psj.2020.04.006
39. Livak, KJ, and Schmittgen, TD. Analysis of relative gene expression data using real-time quantitative pcr and the 2(−delta delta c(t)) method. Methods. (2001) 25:402–8. doi: 10.1006/meth.2001.1262
40. Liu, M, Zhou, J, Li, Y, Ding, Y, Lian, J, Dong, Q, et al. Effects of dietary polyherbal mixtures on growth performance, antioxidant capacity, immune function and jejunal health of yellow-feathered broilers. Poult Sci. (2023) 102:102714. doi: 10.1016/j.psj.2023.102714
41. Li, XL, He, WL, Wang, ZB, and Xu, TS. Effects of Chinese herbal mixture on performance, egg quality and blood biochemical parameters of laying hens. J Anim Physiol Anim Nutr (Berl). (2016) 100:1041–9. doi: 10.1111/jpn.12473
42. Liu, B, Ma, R, Yang, Q, Yang, Y, Fang, Y, Sun, Z, et al. Effects of traditional Chinese herbal feed additive on production performance, egg quality, antioxidant capacity, immunity and intestinal health of laying hens. Animals (Basel). (2023) 13:2510. doi: 10.3390/ani13152510
43. Zhang, L, Zhong, G, Gu, W, Yin, N, Chen, L, and Shi, S. Dietary supplementation with daidzein and Chinese herbs, independently and combined, improves laying performance, egg quality and plasma hormone levels of post-peak laying hens. Poult Sci. (2021) 100:101115. doi: 10.1016/j.psj.2021.101115
44. Xiao, N, Zhao, Y, Yao, Y, Wu, N, Xu, M, Du, H, et al. Biological activities of egg yolk lipids: a review. J Agric Food Chem. (2020) 68:1948–57. doi: 10.1021/acs.jafc.9b06616
45. Czumaj, A, and Sledzinski, T. Biological role of unsaturated fatty acid desaturases in health and disease. Nutrients. (2020) 12:12. doi: 10.3390/nu12020356
46. Miles, EA, Childs, CE, and Calder, PC. Long-chain polyunsaturated fatty acids (lcpufas) and the developing immune system: a narrative review. Nutrients. (2021) 13:13. doi: 10.3390/nu13010247
47. Meresman, GF, Gotte, M, and Laschke, MW. Plants as source of new therapies for endometriosis: a review of preclinical and clinical studies. Hum Reprod Update. (2021) 27:367–92. doi: 10.1093/humupd/dmaa039
48. Li, R, Guo, K, Liu, C, Wang, J, Tan, D, Han, X, et al. Strong inflammatory responses and apoptosis in the oviducts of egg-laying hens caused by genotype viid Newcastle disease virus. BMC Vet Res. (2016) 12:255. doi: 10.1186/s12917-016-0886-2
49. Wang, J, Tang, C, Wang, Q, Li, R, Chen, Z, Han, X, et al. Apoptosis induction and release of inflammatory cytokines in the oviduct of egg-laying hens experimentally infected with h9n2 avian influenza virus. Vet Microbiol. (2015) 177:302–14. doi: 10.1016/j.vetmic.2015.04.005
50. Li, Z, Gao, Y, Du, L, Yuan, Y, Huang, W, Fu, X, et al. Anti-inflammatory and anti-apoptotic effects of shaoyao decoction on x-ray radiation-induced enteritis of c57bl/6 mice. J Ethnopharmacol. (2022) 292:115158. doi: 10.1016/j.jep.2022.115158
51. Yan, P, Liu, J, Huang, Y, Li, Y, Yu, J, Xia, J, et al. Lotus leaf extract can attenuate salpingitis in laying hens by inhibiting apoptosis. Poult Sci. (2023) 102:102865. doi: 10.1016/j.psj.2023.102865
52. Gimpel, AK, Maccataio, A, Unterweger, H, Sokolova, MV, Schett, G, and Steffen, U. Iga complexes induce neutrophil extracellular trap formation more potently than igg complexes. Front Immunol. (2021) 12:761816. doi: 10.3389/fimmu.2021.761816
53. Khan, SR, Burgh, ACVD, Peeters, RP, Hagen, PMV, Dalm, VASH, and Chaker, L. Determinants of serum immunoglobulin levels: a systematic review and meta-analysis. Front Immunol. (2021) 12:1103. doi: 10.3389/fimmu.2021.664526
54. Sims, JE, and Smith, DE. The il-1 family: regulators of immunity. Nat Rev Immunol. (2010) 10:89–102. doi: 10.1038/nri2691
55. Chen, Y, Akhtar, M, Ma, Z, Hu, T, Liu, Q, Pan, H, et al. Chicken cecal microbiota reduces abdominal fat deposition by regulating fat metabolism. Npj Biofilms Microb. (2023) 9:28. doi: 10.1038/s41522-023-00390-8
56. Rychlik, I. Composition and function of chicken gut microbiota. Animals. (2020) 10:103. doi: 10.3390/ani10010103
57. Song, B, Li, P, Yan, S, Liu, Y, Gao, M, Lv, H, et al. Effects of dietary astragalus polysaccharide supplementation on the th17/treg balance and the gut microbiota of broiler chickens challenged with necrotic enteritis. Front Immunol. (2022) 13:781934. doi: 10.3389/fimmu.2022.781934
58. Hagiya, H, Kimura, K, Nishi, I, Yamamoto, N, Yoshida, H, Akeda, Y, et al. Desulfovibrio desulfuricans bacteremia: a case report and literature review. Anaerobe. (2018) 49:112–5. doi: 10.1016/j.anaerobe.2017.12.013
59. La Reau, AJ, and Suen, G. The ruminococci: key symbionts of the gut ecosystem. J Microbiol. (2018) 56:199–208. doi: 10.1007/s12275-018-8024-4
60. Peng, MJ, Huang, T, Yang, QL, Peng, S, Jin, YX, and Wang, XS. Dietary supplementation eucommia ulmoides extract at high content served as a feed additive in the hens industry. Poult Sci. (2022) 101:101650. doi: 10.1016/j.psj.2021.101650
61. Gong, H, Wang, T, Wu, M, Chu, Q, Lan, H, Lang, W, et al. Maternal effects drive intestinal development beginning in the embryonic period on the basis of maternal immune and microbial transfer in chickens. Microbiome. (2023) 11:41. doi: 10.1186/s40168-023-01490-5
62. Ding, J, Dai, R, Yang, L, He, C, Xu, K, Liu, S, et al. Inheritance and establishment of gut microbiota in chickens. Front Microbiol. (2017) 8:1967. doi: 10.3389/fmicb.2017.01967
63. Zwirzitz, B, Oladeinde, A, Johnson, J, Zock, G, Milfort, MC, Fuller, AL, et al. Temporal dynamics of the cecal and litter microbiome of chickens raised in two separate broiler houses. Front Physiol. (2023) 14:1083192. doi: 10.3389/fphys.2023.1083192
64. Burke, TL, Rupp, ME, and Fey, PD. Staphylococcus epidermidis. Trends Microbiol. (2023) 31:763–4. doi: 10.1016/j.tim.2023.01.001
65. Goldberg, JB. Pseudomonas: global bacteria. Trends Microbiol. (2000) 8:55–7. doi: 10.1016/s0966-842x(99)01671-6
66. Fathima, S, Shanmugasundaram, R, Adams, D, and Selvaraj, RK. Gastrointestinal microbiota and their manipulation for improved growth and performance in chickens. Foods. (2022) 11:11. doi: 10.3390/foods11101401
67. Asaf, S, Numan, M, Khan, AL, and Al-Harrasi, A. Sphingomonas: from diversity and genomics to functional role in environmental remediation and plant growth. Crit Rev Biotechnol. (2020) 40:138–52. doi: 10.1080/07388551.2019.1709793
Keywords: Chinese herbal mixtures, Wenchang breeder hens, egg quality, immune status, intestinal microbiota
Citation: Liu M, Huang J, Ma M, Huang G, Zhang Y, Ding Y, Qu Q, Lv W and Guo S (2023) Effects of dietary Chinese herbal mixtures on productive performance, egg quality, immune status, caecal and offspring meconial microbiota of Wenchang breeder hens. Front. Vet. Sci. 10:1320469. doi: 10.3389/fvets.2023.1320469
Edited by:
Ilaria Biasato, University of Turin, ItalyReviewed by:
Keyu Zhang, Chinese Academy of Agricultural Sciences, ChinaYun Peng Fan, Northwest A&F University, China
Copyright © 2023 Liu, Huang, Ma, Huang, Zhang, Ding, Qu, Lv and Guo. This is an open-access article distributed under the terms of the Creative Commons Attribution License (CC BY). The use, distribution or reproduction in other forums is permitted, provided the original author(s) and the copyright owner(s) are credited and that the original publication in this journal is cited, in accordance with accepted academic practice. No use, distribution or reproduction is permitted which does not comply with these terms.
*Correspondence: Weijie Lv, lvwj@scau.edu.cn; Shining Guo, shin-ing@scau.edu.cn
†These authors have contributed equally to this work and share first authorship