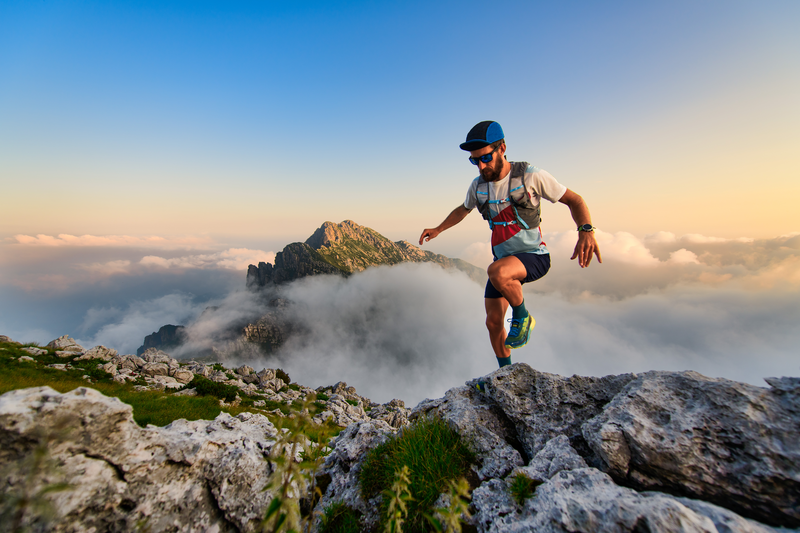
94% of researchers rate our articles as excellent or good
Learn more about the work of our research integrity team to safeguard the quality of each article we publish.
Find out more
REVIEW article
Front. Vet. Sci. , 19 December 2023
Sec. Parasitology
Volume 10 - 2023 | https://doi.org/10.3389/fvets.2023.1309151
Helminth parasite infections are widespread in smallholder farming systems affecting farmers and livestock animals. There are pathogenic parasites that populate the gut of their host and coexist closely with the gut microbiota. The physical and immunological environment of the gut can be modified by parasites and microbiota creating a wide range of interactions. These interactions modify the development of infection, affects overall host health, and can modify the way a host interacts with its bacterial microbiota. In addition, where there is a high worm burden parasites will affect the health of the host and intestinal tract colonization. This review highlights key studies on the interaction between helminth parasites and the intestinal microbiome to understand the relationship between parasitic worm infections and gut microbiome health in chickens. Finally, the review discusses modulations, molecular changes, and the importance of helminth-microbiome interactions for the host.
Poultry production plays an important role in extensive smallholder agriculture in developing countries as compared to cattle and goats, this highlights the importance of poultry husbandry in rural areas (1). A research study conducted from 2015 to 2020 by the World Bank presented stats on the proportion of livestock husbandry in 24 developing countries (2). The study was disaggregated by developing sub-regions and concluded that poultry was owned by 75, 78, and 57% of the sampled rural households in East Asia and the Pacific, Latin America and the Caribbean, and Sub-Saharan Africa, respectively (1, 2). This partially supports the importance of extensive poultry production within developing countries as a source of high-quality protein kept by most rural households within developing countries.
Extensive poultry production systems are free-range systems characterized by high mortality rates, open contaminated feeds, contaminated water sources, and litter systems within the surrounding environments, which allows for modes of parasites transmission from intermediate hosts such as ants, grasshoppers, earthworms, mites, and beetles (3). These intermediate hosts can withstand harsh environmental conditions and serve as food for chickens, making transmission of the infective stage of the parasites to chickens highly possible. In addition, microorganisms are also evident in eggs prior to hatching and are transferred from the mother via the chicken oviduct (4), or from the habitat through the pores in the eggshell (5). In addition, the gut microbiomes are also vulnerable to bacterial pathogens such as Salmonella and Campylobacter which can be a reservoir of antibiotic resistance and transmission leading to serious public health threats (6–8).
Tapeworms (cestodes), roundworms (nematodes), and flukes (trematodes) are helminth parasites that cause intestinal helminthiasis (9). With nematodes being the most economically important intestinal parasites in poultry (9). Ascaridia galli is one of the most common nematode parasite infecting chickens in different countries (10–15) with infections occurring more in chickens raised in backyard and free-range systems than in chickens raised in cage and poultry house production systems (16). According to a meta-analysis and systematic review study on the prevalence of helminth infections in chickens over time (based on articles published between 1942 and 2019), free-range and backyard systems had a significantly higher prevalence rate of helminth infections (84.8 and 82.6%) respectively than those reared in cage poultry production systems (63.6%) (16). Moreover, the pooled prevalence outcomes indicated that A. galli had the higher infection rate of 35.9% as compared to Heterakis gallinarum (28.5%), Raillietina spp. (19.0%), and Capillaria spp. (5.90%), with more than 30 helminth species identified. Furthermore, a study by Shifaw et al. (17) showed that chickens kept in floor production systems were infected with a minimum of one or more helminth parasite species.
The intestinal tract of a chicken is populated by a microbial population consisting of protozoa, fungi, bacteria, and viruses that have developed along with the host immune system (18–20). This microbial community has an important role in the physical performance, growth, and health of the host (21–24). In addition, the interaction between the host and intestinal microbiota aid in digestion of nutrients, immune system growth, and pathogen exclusion (25–28). Furthermore, a healthy microbial population is important for gut homeostasis and host metabolism, which affects animal physiology and health, with the host providing a tolerant environment and nutrients for bacterial colonization and growth (27).
The health and functionality of the chicken gut is influenced by the gastrointestinal microbiota and feed (19) with the gut microbiota forming protective barriers by adhering to the epithelial wall of the enterocyte to reduce the colonization of pathogenic bacteria (24). These bacteria also produce lower triglyceride and induce non-pathogenic immune responses and produce organic acids (e.g., lactic acid), fatty acids (acetic acid, butyric acid, and propionic acid) vitamins, (e.g., vitamin B group,) and antimicrobial compounds (e.g., bacteriocins) all of which offer nutrition and protection to the chicken (24, 29, 30).
Smallholder chicken production systems play a crucial role in poverty alleviation in resource-poor settings. The system tends to be characterized by chickens that get left to forage for themselves to meet their nutritional needs with little to no input (Figure 1) (31, 32) and is used particular by poor households, small-scale farmers, and landless communities located in rural settlements and villages (33). Chickens raised under such farming systems are susceptible to parasitic infections (34, 35) and encounter difficulties that include lack of quality feed ingredients, lack of quality water, lack of effective vaccination programs and unsuccessful marketing tactics, and mortality among others (36) due to not following well-managed and safe productive procedures and being free-range scavenging chickens. This approach creates a conducive environment that results in disease strain on scavenging chickens, because of various mixed ages in a flock and potential for disease transmission from other poultry species kept in the same area (37). Furthermore, no breeding programs exists in these systems and replacement stock is obtained from natural incubation (38).
Of the challenges experienced by chickens raised under a scavenging system, parasitism is a fundamental problem with both ectoparasites and endoparasites infecting chickens. Helminths are reported as one of the most common endoparasites worldwide found in scavenging chickens (16), with chickens raised under extensive smallholder farming systems being likely to pick up larvae, infective eggs, and intermediate hosts of parasites during scavenging (39). Furthermore, contact with infected feces causes a higher risk of endo-parasitism and Salmonella infection. A study conducted in selected villages of KwaZulu-Natal province of South Africa found A. galli to be a common endo-parasite present in all sampled sites with an infection rate of 58.3%, while H. beramporia and Heterakis gallinarum were present in only three sampled sites (14). The high pool prevalence of helminth infections is known to have an impact on welfare (40), production performance (41) of free-range chickens and susceptibility to secondary infections, thus causing significant economic losses for farmers (42).
Gastrointestinal helminth parasites are intricate multicellular organisms classified under various taxonomic families “but collectively share the capacity to downregulate the host immune response directed toward themselves (parasite-specific immunoregulation)” (43). A study by Malatji et al. (40) reported the downregulation of mast cell protease 1a-like, cytochrome P450 and apolipoprotein B in village chickens infected by A. galli parasites. These findings supported the results by Balqis et al. (44) that indicated the significant role played by mast cells in controlling A. galli infection. This is the strategy used by parasites to promote their survival by altering the activation of the immune response of the host during parasite infection. Furthermore, Malatji et al. (40) identified Arachidonic acid metabolism as one of the pathways that were significantly impacted in chickens infected by A. galli parasites. Arachidonic acid is able to regulate immune functions and Freeman et al. (45) showed that it can act as an inhibitor for Type 1 helper T cell (Th1) response.
These parasites are eukaryotic organisms that infect several hosts including livestock. Helminth infections are sub-clinical even when occurring in lower numbers but can suppress animal productivity and welfare through reduced weight gain and efficiency of feed utilization (46). In addition, factors such as the distribution of the intermediate hosts, their rate and the number of infective parasite eggs and larvae can influence helminth infection (47). Furthermore, studies have shown that the most widespread helminth parasites are Heterakis gallinarum and A. galli as reported in smallholder faming systems research (9, 13, 16, 48).
While there is knowledge of the presence of helminth parasites in smallholder farming systems (16), there is little information on the interaction between helminth parasites and the gut microbiota. Several mechanisms are involved in the regulation of gut functionality and health, which makes it crucial to understand interactions between helminth parasites and the intestinal microbiome so that strategies are put together for the modulation of gut functionality and health to improve animal performance (49, 50).
Microbes are organisms that abundantly colonize the gastrointestinal tract of their host. These microbial organisms inhabit the intestinal tract of chickens, and are important for host metabolism and gut homeostasis, and affect the animal’s health and physiology (51). The gut microbiota is the microbial population that includes pathogenic, commensal and symbiotic microorganisms that are in and on multicellular organisms and are more abundant than germinal and somatic cells of the host (52). The collective genome of these symbionts is known as the microbiome (52). Thus, unraveling the development of the chicken microbiome and how it is influenced by different factors (35, 53, 54). These factors include dietary factors (such as dietary supplements, probiotics, and antibiotic growth promoters) that are of value, as the microbiota composition and functionality are linked with animal performance and health and can be modulated using dietary interventions (55, 56). Chickens host a range of microbial communities with the main sites carrying the most abundant microbiota being the skin, respiratory and reproductive tract, and gastrointestinal tract (20, 57, 58). The microbiota has a protective role in transmissible and non-transmissible diseases, and the gastrointestinal tract is the most vital site with the highest bacterial diversity and abundance (20). The intestinal tract helps maintain homeostasis, providing the organism with the ability to withstand physical, psychological, environmental stresses (49).
More than 900 bacterial species populate the gastrointestinal tract and aid in defense against pathogens, as well as digestion of food (35, 51). These bacterial species form a protective barrier through a variety of suggested mechanisms, including the production of antimicrobial factors, competition for nutrients and attachment to epithelial cells and preventing the opportunity for colonization of enteric pathogens (59, 60). Another distinctive trait of the gut microbiota is that commensal bacteria stimulate the early stages of the immunological system growth, including both the native and acquired immunologic responses, and regulate mucosal immunity (tolerance vs. inflammation), all depending on the microbial composition (19, 21, 61). Furthermore, the microbiota reduces and prevents colonization by exclusion, and the production of bactericidal substances and bacteriostatic (62). Also, the taxonomic composition of the microbiota is influenced by various components such as the animals age, diet, organ and animal antimicrobial usage (53). In addition, the intestinal microbiota is essential to hosts health, which makes it important to explain the mechanisms changing its diversity and composition.
Gastrointestinal helminths and bacteria populate the same ecological niche in livestock farming systems, where these microbes have relation with and influence each other (63). These intestinal helminths are host immune modulators that have developed spatially and temporally with the gut microbiota, which results in potential mechanistic interaction (64). Hence eukaryotic microbiota and parasites can modify the immunological and physical environment of the intestine which creates several chances of interaction. As a result, such interactions may modify infection results and have critical effect on the hosts health and diseases.
Interaction of bacteria with enteric helminths can contribute to the permanence of microbial prevalence. This can be seen in the link that exists between the gastrointestinal nematode (Schistosoma sp.) and bacterial Salmonella sp. Furthermore, Schistosoma sp. helps with the preservation of Salmonella sp. in the gastrointestinal tract of Mus musculus, due to bacteria connected to nematode folds (65). However, in chickens, enteritis per Salmonella sp. can reduce the presences of A. galli parasite established when the infection is late (66). This parasitic infection is susceptible to bacteria like Pasteurella multocida and E. coli (67, 68), however, A. galli is capable of producing bactericide molecules (69).
The chicken’s intestinal tract consists of the glandular stomach, known as the proventriculus, the gizzard known as the ventriculus and the small and large intestine (70, 71), with a metabolic function that develops the microbial community. It is densely colonized by a community of microbiota which include fungi, protozoa and bacteria that interact with the consumed feed and host. These intestinal helminths are prevalent in free-range and backyard poultry systems (47, 72) and represent a major constraint to poultry productivity.
Studies have shown that helminth infection can significantly alter the predicted metabolic potential and the composition of the gut microbiota (46, 73, 74), indicating that disturbance of the basal gut microbiota function may be a factor in reduced productivity in infected species (46). Additionally, infection can have a negative impact on animal health by increasing susceptibility to secondary infections and modulating host immunity (46).
Under normal conditions, bacteria tend to have unfavorable effect on gut health of the host as they produce some level of toxic compounds as a byproduct of metabolism when they compete with the host for nutrition (50). Village chickens carry different types of bacteria that affect their health (75). Studies have been conducted and detected Salmonella spp. in village chickens. Recently, studies reported the prevalence of 27 and 29% of Salmonella spp. on indigenous chickens from Tanzania and Iran, respectively (76, 77).
Escherichia coli is a motile Gram-negative bacterium belonging to the Enterobacteriaceae family. It is a natural inhabitant of the intestinal microbiota of chickens including their mucosal surface and it is present in the poultry habitat (26, 78). The majority of E. coli are non-pathogenic to the chicken host; however, 10–15% of E. coli isolated from the gastro-intestinal tract of broiler chickens may be infective (79). Avian Pathogenic E. coli (APEC) is a subset of extraintestinal pathogenic E. coli (ExPEC) that causes disease outside the animals’ gastrointestinal tract. It affects all poultry species in all types of production systems (80) and all chicken age groups (81). Furthermore, APEC result in localized and systemic infections in poultry, resulting in production loss and rapid mortality (82, 83).
Enterococcus spp. also showed to cause different diseases like femoral head necrosis, osteomyelitis, skeletal disease, and spondylitis. In addition, these bacteria are also linked to musculoskeletal disease in chickens (84, 85). The Enterococcus spp. is known to cause infections due to its intrinsic ruggedness (86).
Over the course of evolution, livestock animals have coevolved with helminths and bacteria. The relationship between helminths microbiome and the host influences not only host-microbiome and host-helminth interactions but also the relationship between microbiome and helminth, with a significant impact on metabolic potential and host immunity (49, 87–89). There are benefits and costs to the host that are brought about by a normal gut microbial community (90, 91). The main benefits coming from the commensal microbiota are immune stimulation, contributions to host nutrition (feed conversion rates), competitive exclusion of non-indigenous microbes or pathogens (90).
The interactions between the GIT and microorganisms also influence the animals’ growth, stability of the microbial communities and animal’s health (92). Moreover, helminths and microbiota have immunomodulatory abilities and contribute to immune homeostasis within the host. Furthermore, gastrointestinal parasites can alter the diversity and composition of the intestinal microbiota in the host, with previous articles reporting that intestinal parasites can significantly change the composition and abundance of the intestinal microbiota (49, 50, 63, 93).
Helminth parasitic infection in smallholder farming systems is ubiquitous with negative impacts that invade livestock environments. These impacts require biosecurity measures that can benefit chicken production in smallholder farming systems. Biosecurity is a hygiene, segregation, or management procedure that aims at reducing any potential infectious microbes in and around farm environmental areas (94). To improve poultry production health monitoring and detection of parasitic infections is important, as this mitigates the spread of helminth parasites in smallholder farming areas, landless communities, and poor households. Control strategies are important in maximizing the possibilities of successful intervention which would assist in decreasing productivity losses, and range contamination for subsequent production cycles (42).
Biosecurity precautions are not essentially practiced across smallholder farmers in Africa as farmers in rural areas do not understand the potential risk to livestock and argue that the benefits of biosecurity measures do not outweigh the costs (95). Hence, they are faced with several challenges due to substandard biosecurity measures. These challenges can be seen in poultry raised through free-range systems with feed limited to what chickens can find on their own, which results in high prevalence rate of microbial infection, low productivity and high mortality rates (75). Biosecurity measures are an important part of any public health and animal plan as well as disease control and prevention for better survival and production output.
Although it may not be common in many smallholder farming systems, some of the biosecurity measures that are taken by some rural/smallholder farmers include isolation of livestock from sources of infectious contamination. This involves separating different livestock species from possible infectious areas to avoid exposing healthy livestock to contaminated areas (96). Farmers also limit the addition of new chickens into an existing flock, by keeping new poultry in isolation for 30 days before releasing them into an existing flock (96). This helps prevent cross-contamination which is also controlled by separating and identifying clean and unclean farming areas for decontamination and sanitization processes. Furthermore, privileged farmers control and minimize contamination by disinfecting work equipment and livestock areas, and using protective clothing for husbandry, which helps contribute to decrease and elimination of diseases (96). Additionally, other farmers provide clean supply of feed, water, litter and biocontainment throughout the farm as a biosecurity measures (97).
Furthermore, understanding the host–parasite relationship is important for the inception of strategic approaches to avoid increasing dependence on anthelmintics and frequent usage (98). There is also a paucity of research on the resistance of anthelmintic strategies (benzimidazoles) in parasites such as A. galli, indicating the importance of optimizing tools for the monitoring and detection of anthelmintic resistance in poultry parasites (99). The control of A. galli roundworms can also be carried out by simply breaking the reproductive cycle by eradicating the intermediate hosts such as earthworms (100). Moreover, it is important to improve management and hygiene among the flock before considering anti-parasite control, by frequently cleaning the environment in which chickens scavenge. Awareness strategies are also necessary to educate farmers on the A. galli parasite, the effects that helminths have on chickens, and how farmers can play a role in controlling infection among their chickens. Also, several ways have been implemented such as traditional, chemical, immunological, managemental, biological, and genetical strategies.
Poultry farming generates money for many smallholder farmers in developing countries through the sale of meat, eggs, manure, and live chickens. Poultry have coevolved with helminths and bacteria throughout evolution, with intestinal helminths and microbiota inhabiting the same ecological niche. The mode of relation can be indirect or direct and infection can have indirect impact on species health by increasing susceptibility to secondary diseases and modulation of the host immunity. Helminth infection influence intestinal microbiome and helminth-microbiota interaction indicates that microbiota reduces and prevents colonization. Therefore, it is imperative to understand the helminth-microbiota interaction to improve chicken productivity in low-output/low-input chicken production systems in order to develop effective control strategies against helminth infections in different parts of Africa and other developing countries.
MN: Funding acquisition, Writing – original draft. RS: Writing – review & editing. DM: Conceptualization, Funding acquisition, Supervision, Writing – review & editing.
The author(s) declare financial support was received for the research, authorship, and/or publication of this article. The authors acknowledged the generous financial assistance from the National Research Foundation of South Africa (Grant nos. 121875 and MND200423515978).
The authors declare that the research was conducted in the absence of any commercial or financial relationships that could be construed as a potential conflict of interest.
All claims expressed in this article are solely those of the authors and do not necessarily represent those of their affiliated organizations, or those of the publisher, the editors and the reviewers. Any product that may be evaluated in this article, or claim that may be made by its manufacturer, is not guaranteed or endorsed by the publisher.
1. Birhanu, MY, Osei-Amponsah, R, Yeboah Obese, F, and Dessie, T. Smallholder poultry production in the context of increasing global food prices: roles in poverty reduction and food security. Anim Front. (2023) 13:17–25. doi: 10.1093/af/vfac069
2. RHoMIS (2021). The rural household multiple indicator survey (RHoMIS) data of 35,713 farm households in 32 countries Harvard Dataverse. doi: 10.7910/DVN/TFXQJN
3. Radfar, MH, Asl, EN, Seghinsara, HR, Dehaghi, MM, and Fathi, S. Biodiversity and prevalence of parasites of domestic pigeons (Columba livia domestica) in a selected semiarid zone of South Khorasan, Iran. Trop Anim Health Prod. (2012) 44:225–9. doi: 10.1007/s11250-011-0002-3
4. Gantois, I, Ducatelle, R, Pasmans, F, Haesebrouck, F, Gast, R, Humphrey, TJ, et al. Mechanisms of egg contamination by Salmonella Enteritidis. FEMS Microbiol Rev. (2009) 33:718–38. doi: 10.1111/j.1574-6976.2008.00161.x
5. Roto, SM, Kwon, YM, and Ricke, SC. Applications of in OVO technique for the optimal development of the gastrointestinal tract and the potential influence on the establishment of its microbiome in poultry. Front Vet Sci. (2016) 3:63. doi: 10.3389/fvets.2016.00063
6. Kumar, S, Chen, C, Indugu, N, Werlang, GO, Singh, M, Kim, WK, et al. Effect of antibiotic withdrawal in feed on chicken gut microbial dynamics, immunity, growth performance and prevalence of foodborne pathogens. PLoS One. (2018) 13:e0192450. doi: 10.1371/journal.pone.0192450
7. Mancabelli, L, Ferrario, C, Milani, C, Mangifesta, M, Turroni, F, Duranti, S, et al. Insights into the biodiversity of the gut microbiota of broiler chickens. Environ Microbiol. (2016) 18:4727–38. doi: 10.1111/1462-2920.13363
8. Zhou, W, Wang, Y, and Lin, J. Functional cloning and characterization of antibiotic resistance genes from the chicken gut microbiome. Appl Environ Microbiol. (2012) 78:3028–32. doi: 10.1128/AEM.06920-11
9. Ola-Fadunsin, SD, Uwabujo, PI, Sanda, IM, Ganiyu, IA, Hussain, K, Rabiu, M, et al. Gastrointestinal helminths of intensively managed poultry in Kwara central, Kwara state, Nigeria: its diversity, prevalence, intensity, and risk factors. Vet World. (2019) 12:389–96. doi: 10.14202/vetworld.2019.389-396
10. Alam, MN, Mostofa, M, Khan, MAHNA, Alim, MA, Rahman, AKMA, and Trisha, AA. Prevalence of gastrointestinal helminth infections in indigenous chickens of selected areas of Barisal district, Bangladesh. Bangladesh J Vet Med. (2014) 12:135–9. doi: 10.3329/bjvm.v12i2.21275
11. Baboolal, V, Suratsingh, V, Gyan, L, Brown, G, Offiah, NV, Adesiyun, AA, et al. The prevalence of intestinal helminths in broiler chickens in Trinidad. Veterinarski Arhiv. (2012) 82:591–7. Available at: https://hrcak.srce.hr/93351
12. Kaingu, FB, Kibor, AC, Shivairo, R, Kutima, H, Okeno, TO, Waihenya, R, et al. Prevalence of gastro-intestinal helminthes and coccidia in indigenous chicken from different agro-climatic zones in Kenya. Afr J Agric Res. (2010) 5:458–62.
13. Malatji, DP, Tsotetsi, AM, van Marle-Koster, E, and Muchadeyi, FC. Population genetic structure of Ascaridia galli of extensively raised chickens of South Africa. Vet Parasitol. (2016) 216:89–92. doi: 10.1016/j.vetpar.2015.12.012
14. Mlondo, S, Tembe, D, Malatji, MP, Khumalo, ZTH, and Mukaratirwa, S. Molecular identification of helminth parasites of the Heterakidae and Ascarididae families of free-ranging chickens from selected rural communities of KwaZulu-Natal Province of South Africa. Poult Sci. (2022) 101:101979. doi: 10.1016/j.psj.2022.101979
15. Nghonjuyi, NW, Kimbi, HK, and Tiambo, CK. Study of gastro–intestinal parasites of scavenging chickens in Fako division, Southwest Cameroon. J Adv Parasitol. (2014) 1:30–4. doi: 10.14737/journal.jap/1.2.30.34
16. Shifaw, A, Feyera, T, Walkden-Brown, SW, Sharpe, B, Elliott, T, and Ruhnke, I. Global and regional prevalence of helminth infection in chickens over time: a systematic review and meta-analysis. Poult Sci. (2021) 100:1–9. doi: 10.1016/j.psj.2021.101082
17. Shifaw, A, Feyera, T, Sharpe, B, Elliott, T, Walkden-Brown, SW, and Ruhnke, I. Prevalence and magnitude of gastrointestinal helminth infections in cage-free laying chickens in Australia. Vet Parasitol Reg Stud Reports. (2023) 37:100819. doi: 10.1016/j.vprsr.2022.100819
18. Kogut, MH, Lee, A, and Santin, E. Microbiome and pathogen interaction with the immune system. Poult Sci. (2020) 99:1–5. doi: 10.1016/j.psj.2019.12.011
19. Shang, Y, Kumar, S, Oakley, B, and Kim, WK. Chicken gut microbiota: importance and detection technology. Front Vet Sci. (2018) 5:254. doi: 10.3389/fvets.2018.00254
20. Wei, S, Morrison, M, and Yu, Z. Bacterial census of poultry intestinal microbiome. Poult Sci. (2013) 92:671–83. doi: 10.3382/ps.2012-02822
21. Brisbin, JT, Gong, J, and Sharif, S. Interactions between commensal bacteria and the gut-associated immune system of the chicken. Anim Health Res Rev. (2008) 9:101–10. doi: 10.1017/s146625230800145x
22. Jankowski, J, Juskiewicz, J, Gulewicz, K, Lecewicz, A, Slominski, BA, and Zdunczyk, Z. The effect of diets containing soybean meal, soybean protein concentrate, and soybean protein isolate of different oligosaccharide content on growth performance and gut function of young turkeys. Poult Sci. (2009) 88:2132–40. doi: 10.3382/ps.2009-00066
23. Okamoto, T, Morino, K, Ugi, S, Nakagawa, F, Lemecha, M, Ida, S, et al. Microbiome potentiates endurance exercise through intestinal acetate production. Am J Physiol Endocrinol Metab. (2019) 316:E956–66. doi: 10.1152/ajpendo.00510.2018
24. Yegani, M, and Korver, DR. Factors affecting intestinal health in poultry. Poult Sci. (2008) 87:2052–63. doi: 10.3382/ps.2008-00091
25. Apajalahti, J, and Vienola, K. Interaction between chicken intestinal microbiota and protein digestion. Anim Feed Sci Technol. (2016) 221:323–30. doi: 10.1016/j.anifeedsci.2016.05.004
26. Oakley, BB, Lillehoj, HS, Kogut, MH, Kim, WK, Maurer, JJ, Pedroso, A, et al. The chicken gastrointestinal microbiome. FEMS Microbiol Lett. (2014) 360:100–12. doi: 10.1111/1574-6968.12608
27. Pan, D, and Yu, Z. Intestinal microbiome of poultry and its interaction with host and diet. Gut Microbes. (2014) 5:108–19. doi: 10.4161/gmic.26945
28. Vispo, C, and Karasov, WH. The interaction of avian gut microbes and their host: an elusive symbiosis. Gastrointestinal Microbiol. (1997) 1:116–55. doi: 10.1007/978-1-4615-4111-0_5
29. Apajalahti, J. Comparative gut microflora, metabolic challenges, and potential opportunities. J Appl Poult Res. (2005) 14:444–53. doi: 10.1093/japr/14.2.444
30. Jeurissen, SHM, Lewis, F, van der Klis, JD, Mroz, Z, Rebel, JMJ, and ter Huurne, AAHM. Parameters and techniques to determine intestinal health of poultry as constituted by immunity, integrity, and functionality. Curr Issues Intest Microbiol. (2002) 3:1–14.
31. Manyelo, TG, Selaledi, L, Hassan, ZM, and Mabelebele, M. Local chicken breeds of Africa: their description, uses and conservation methods. Animals. (2020) 10:2–12. doi: 10.3390/ani10122257
32. Tufarelli, V, Ragni, M, and Laudadio, V. Feeding forage in poultry: a promising alternative for the future of production systems. Agriculture. (2018) 8:2–6. doi: 10.3390/agriculture8060081
33. Fanatico, AC, Owens, CM, and Emmert, JL. Organic poultry production in the United States: broilers. J Appl Poult Res. (2009) 18:355–66. doi: 10.3382/japr.2008-00123
34. Berhe, M, Mekibib, B, Bsrat, A, and Atsbaha, G. Gastrointestinal helminth parasites of chicken under different management system in Mekelle town, Tigray region, Ethiopia. J Vet Med. (2019) 2019:1307582. doi: 10.1155/2019/1307582
35. De Meyer, F, De Grande, A, Ducatelle, R, Van Immerseel, F, and Clark, M. The chicken microbiota and how to modulate it In: Atterbury R, Baigent S, Nair V, Barrow P, editors. Poultry health: a guide for professionals. (2021). 257–62. doi: 10.1079/9781789245042.0017
36. Idowu, PA, Zishiri, O, Nephawe, KA, and Mtileni, B. Current status and intervention of South Africa chicken production – a review. Worlds Poult Sci J. (2021) 77:115–33. doi: 10.1080/00439339.2020.1866965
37. Acamovic, T, Sinurat, A, Natarajan, A, Anitha, K, Chandrasekaran, D, Shindey, D, et al. Poultry. Livestock and wealth creation: improving the husbandry of animals kept by resource-poor people in developing countries. Nottingham: NUP (2005).
38. Thieme, O., Sonaiya, E.B., Rota, A., Alders, R.G., Saleque, M.A., and De Besi, G. (2014). Family poultry development–issues, opportunities, and constraints. Global Forum on Food Security and Nutrition (FSN Forum). Available at: https://www.fao.org/fsnforum/resources/reports-and-briefs/family-poultry-development-issues-opportunities-and-constraints (Accessed July 7, 2022).
39. Suhaila, A, and Sabrina, D. Study of Parasities in commercial free-range chickens in northern peninsular Malaysia. Malaysian J Vet Res. (2015) 6:53–8.
40. Malatji, DP, van Marle-Koster, E, and Muchadeyi, FC. Gene expression profiles of the small intestine of village chickens from an Ascaridia galli infested environment. Vet Parasitol. (2019) 276:100012. doi: 10.1016/j.vpoa.2019.100012
41. Skallerup, P, Luna, LA, Johansen, MV, and Kyvsgaard, NC. The impact of natural helminth infections and supplementary protein on growth performance of free-range chickens on smallholder farms in El sauce, Nicaragua. Prev Vet Med. (2005) 69:229–44. doi: 10.1016/j.prevetmed.2005.02.003
42. Sharma, N, Hunt, PW, Hine, BC, and Ruhnke, I. The impacts of Ascaridia galli on performance, health, and immune responses of laying hens: new insights into an old problem. Poult Sci. (2019) 98:6517–26. doi: 10.3382/ps/pez422
43. Gazzinelli-Guimaraes, PH, and Nutman, TB. Helminth parasites and immune regulation. F1000Res. (2018) 7:1685. doi: 10.12688/f1000research.15596.1
44. Balqis, U, Hambal, M, and Priosoeryanto, BP. Mucosal mast cells response in the jejunum of Ascaridia galli-infected laying hens. Media Peternakan. (2013) 36:113. doi: 10.5398/medpet.2013.36.2.113
45. Freeman, TC, Ivens, A, Baillie, JK, Beraldi, D, Barnett, MW, Dorward, D, et al. A gene expression atlas of the domestic pig. BMC Biol. (2012) 10:90. doi: 10.1186/1741-7007-10-90
46. Williams, AR, Myhill, LJ, Stolzenbach, S, Nejsum, P, Mejer, H, Nielsen, DS, et al. Emerging interactions between diet, gastrointestinal helminth infection, and the gut microbiota in livestock. BMC Vet Res. (2021) 17:62. doi: 10.1186/s12917-021-02752-w
47. Magwisha, HB, Kassuku, AA, Kyvsgaard, NC, and Permin, A. A comparison of the prevalence and burdens of helminth infections in growers and adult free-range chickens. Trop Anim Health and Prod. (2002) 34:205–14. doi: 10.1023/a:1015278524559
48. Van, NT, Cuong, NV, Yen, NT, Nhi, NT, Kiet, BT, Hoang, NV, et al. Characterisation of gastrointestinal helminths and their impact in commercial small-scale chicken flocks in the Mekong Delta of Vietnam. Trop Anim Health Prod. (2020) 52:53–62. doi: 10.1007/s11250-019-01982-3
49. Kers, JG, Velkers, FC, Fischer, EA, Hermes, GD, Stegeman, JA, and Smidt, H. Host and environmental factors affecting the intestinal microbiota in chickens. Front Microbiol. (2018) 9:235. doi: 10.3389/fmicb.2018.00235
50. Yadav, S, and Jha, R. Strategies to modulate the intestinal microbiota and their effects on nutrient utilization, performance, and health of poultry. J Anim Sci Biotechnol. (2019) 10:2–11. doi: 10.1186/s40104-018-0310-9
51. Borda-Molina, D, Seifert, J, and Camarinha-Silva, A. Current perspectives of the chicken gastrointestinal tract and its microbiome. Comput Struct Biotechnol J. (2018) 16:131–9. doi: 10.1016/j.csbj.2018.03.002
52. Sender, R, Fuchs, S, and Milo, R. Revised estimates for the number of human and Bacteria cells in the body. PLoS Biol. (2016) 14:e1002533. doi: 10.1371/journal.pbio.1002533
53. Ding, J, Dai, R, Yang, L, He, C, Xu, K, Liu, S, et al. Inheritance and establishment of gut microbiota in chickens. Front Microbiol. (2017) 8:1967. doi: 10.3389/fmicb.2017.01967
54. Pedroso, AA, Menten, JF, Lambais, MR, Racanicci, AMC, Longo, FA, and Sorbara, JOB. Intestinal bacterial community and growth performance of chickens fed diets containing antibiotics. Poult Sci. (2006) 85:747–52. doi: 10.1093/ps/85.4.747
55. Wang, Y, Sun, J, Zhong, H, Li, N, Xu, H, Zhu, Q, et al. Effect of probiotics on the meat flavour and gut microbiota of chicken. Sci Rep. (2017) 7:6400. doi: 10.1038/s41598-017-06677-z
56. Wisselink, HJ, Cornelissen, JBWJ, Mevius, DJ, Smits, MA, Smidt, H, and Rebel, JM. Antibiotics in 16-day-old broilers temporarily affect microbial and immune parameters in the gut. Poult Sci. (2017) 96:3068–78. doi: 10.3382/ps/pex133
57. Fathima, S, Shanmugasundaram, R, Adams, D, and Selvaraj, RK. Gastrointestinal microbiota and their manipulation for improved growth and performance in chickens. Foods. (2022) 11:1401. doi: 10.3390/foods11101401
58. Hinton, A Jr, and Ingram, KD. Use of oleic acid to reduce the population of the bacterial flora of poultry skin. J Food Prot. (2000) 63:1282–6. doi: 10.4315/0362-028X-63.9.1282
59. Lan, YMWA, Verstegen, MWA, Tamminga, S, and Williams, BA. The role of the commensal gut microbial community in broiler chickens. Worlds Poult Sci J. (2005) 61:95–104. doi: 10.1079/WPS200445
60. Sommer, F, and Bäckhed, F. The gut microbiota—masters of host development and physiology. Nat Rev Microbiol. (2013) 11:227–38. doi: 10.1038/nrmicro2974
61. Clavijo, V, and Flórez, MJ. The gastrointestinal microbiome and its association with the control of pathogens in broiler chicken production: a review. Poult Sci. (2018) 97:1006–21. doi: 10.3382/ps/pex359
62. Dame-Korevaar, A, Kers, JG, Van der Goot, J, Velkers, FC, Ceccarelli, D, Mevius, DJ, et al. Competitive exclusion prevents colonization and compartmentalization reduces transmission of ESBL-producing Escherichia coli in broilers. Front Microbiol. (2020) 11:566619. doi: 10.3389/fmicb.2020.566619
63. Zaiss, MM, and Harris, NL. Interactions between the intestinal microbiome and helminth parasites. Parasite Immunol. (2016) 38:5–11. doi: 10.1111/pim.12274
64. Kreisinger, J, Bastien, G, Hauffe, HC, Marchesi, J, and Perkins, SE. Interactions between multiple helminths and the gut microbiota in wild rodents. Philos Trans R Soc. (2015) 370:2–11. doi: 10.1098/rstb.2014.0295
65. Phillips, SM, Reid, WA, Doughty, BL, and Bentley, AG. The immunologic modulation of morbidity in schistosomiasis. Studies in athymic mice and in vitro granuloma formation. Am J Trop Med and Hyg. (1980) 29:820–31. doi: 10.4269/ajtmh.1980.29.820
66. Eigaard, NM, Schou, TW, Permin, A, Christensen, JP, Ekstrøm, CT, Ambrosini, F, et al. Infection and excretion of Salmonella Enteritidis in two different chicken lines with concurrent Ascaridia galli infection. Avian Pathol. (2006) 35:487–93. doi: 10.1080/03079450601071696
67. Dahl, C, Permin, A, Christensen, JP, Bisgaard, M, Muhairwa, AP, Petersen, KMD, et al. The effect of concurrent infections with Pasteurella multocida and Ascaridia galli on free range chickens. Vet Microbiol. (2002) 86:313–24. doi: 10.1016/s0378-1135(02)00015-9
68. Permin, A, Christensen, JP, and Bisgaard, M. Consequences of concurrent Ascaridia galli and Escherichia coli infections in chickens. Acta Vet Scand. (2006) 47:43–54. doi: 10.1186/1751-0147-47-43
69. Midha, A, Schlosser, J, and Hartmann, S. Reciprocal interactions between nematodes and their microbial environments. Front Cell Infect Microbiol. (2017) 7:144. doi: 10.3389/fcimb.2017.00144
70. Alshamy, Z, Richardson, KC, Hünigen, H, Hafez, HM, Plendl, J, and Al Masri, S. Comparison of the gastrointestinal tract of a dual-purpose to a broiler chicken line: a qualitative and quantitative macroscopic and microscopic study. PLoS One. (2018) 13:e0204921. doi: 10.1371/journal.pone.0204921
71. Raphulu, T, and Jansen Van Rensburg, C. Growth performance and digestive tract development of indigenous scavenging chickens under village management. J Agric Rur Dev Trop Subtrop. (2018) 119:105–11.
72. Solomon, T, and Yobsan, T. The prevalence of gastrointestinal helminthes of free range backyard chicken (Gallus gallus domesticus) in Digalu Andtijo District. Arsi zone, Oromiya region. J Biol Agric Health. (2017) 7:33–40.
73. Li, RW, Li, W, Sun, J, Yu, P, Baldwin, RL, and Urban, JF. The effect of helminth infection on the microbial composition and structure of the caprine abomasal microbiome. Sci Rep. (2016) 6:20606. doi: 10.1038/srep20606
74. Peachey, LE, Jenkins, TP, and Cantacessi, C. This gut ain’t big enough for both of us. Or is it? Helminth–microbiota interactions in veterinary species. Trends Parasitol. (2017) 33:619–32. doi: 10.1016/j.pt.2017.04.004
75. Igizeneza, A, Bebora, LC, Nyaga, PN, and Njagi, LW. Village-indigenous chicken bacterial carriage after the heavy rains of 2018, Kenya: indicator on environmental contamination with pathogenic/zoonotic bacteria. Vet Med Int. (2022) 2022:5437171. doi: 10.1155/2022/5437171
76. Boraei-nezhad, G, Saadati, D, Jahantigh, M, and Saadat-Jou, S. Prevalence of Salmonella infection in village chickens and determination of the tetracycline resistance genes in the Salmonella isolates in the Sistan region, Iran. Braz J Microbiol. (2023) 54:2375–82. doi: 10.1007/s42770-023-01033-y
77. Rukambile, E, Sintchenko, V, Muscatello, G, Wang, Q, Kiiru, J, Maulaga, W, et al. Campylobacter and Salmonella in scavenging indigenous chickens in rural Central tanzania: prevalence, antimicrobial resistance, and genomic features. Microbiol Res. (2021) 12:440–54. doi: 10.3390/microbiolres12020030
78. Johnson, TJ, Wannemuehler, Y, Doetkott, C, Johnson, SJ, Rosenberger, SC, and Nolan, LK. Identification of minimal predictors of avian pathogenic Escherichia coli virulence for use as a rapid diagnostic tool. J Clin Microbiol. (2008) 46:3987–96. doi: 10.1128/jcm.00816-08
79. Harry, EG, and Hemsley, LA. The association between the presence of septicaemia strains of Escherichia coli in the respiratory and intestinal tracts of chickens and the occurrence of coli septicaemia. Vet Rec. (1965) 77:35–40.
80. Guabiraba, R, and Schouler, C. Avian colibacillosis: still many black holes. FEMS Microbiol Lett. (2015) 362:fnv118. doi: 10.1093/femsle/fnv118
81. Lutful Kabir, SM. Avian colibacillosis and salmonellosis: a closer look at epidemiology, pathogenesis, diagnosis, control and public health concerns. Int J Environ Res Public Health. (2010) 7:89–114. doi: 10.3390/ijerph7010089
82. Abdelaziz, AM, Mohamed, MH, Fayez, MM, Al-Marri, T, Qasim, I, and Al-Amer, AA. Molecular survey and interaction of common respiratory pathogens in chicken flocks (field perspective). Vet World. (2019) 12:1975. doi: 10.14202/vetworld.2019.1975-1986
83. Vandekerchove, D, De Herdt, P, Laevens, H, and Pasmans, F. Colibacillosis in caged layer hens: characteristics of the disease and the aetiological agent. Avian Pathol. (2004) 33:117–25. doi: 10.1080/03079450310001642149
84. Robbins, KM, Suyemoto, MM, Lyman, RL, Martin, MP, Barnes, HJ, and Borst, LB. An outbreak and source investigation of enterococcal spondylitis in broilers caused by Enterococcus cecorum. Avian Dis. (2012) 56:768–73. doi: 10.1637/10253-052412-Case.1
85. Roy, K, Islam, MS, Paul, A, Ievy, S, Talukder, M, Sobur, MA, et al. Molecular detection and antibiotyping of multi-drug resistant Enterococcus faecium from healthy broiler chickens in Bangladesh. Vet Med Sci. (2022) 8:200–10. doi: 10.1002/vms3.669
86. Santagati, M, Campanile, F, and Stefani, S. Genomic diversification of enterococci in hosts: the role of the mobilome. Front Microbiol. (2012) 3:95. doi: 10.3389/fmicb.2012.00095
87. Hauck, R. Interactions between parasites and the bacterial microbiota of chickens. Avian Dis. (2017) 61:428–36. doi: 10.1637/11675-051917-review.1
88. Johnson, J, and Reid, WM. Ascaridia galli (Nematoda): development and survival in gnotobiotic chickens. Exp Parasitol. (1973) 33:95–9. doi: 10.1016/0014-4894(73)90013-1
89. Springer, WT, Johnson, J, and Reid, WM. Histomoniasis in gnotobiotic chickens and turkeys: biological aspects of the role of bacteria in the etiology. Exp Parasitol. (1970) 28:383–92. doi: 10.1016/0014-4894(70)90106-2
90. Dibner, JJ, and Richards, JD. Antibiotic growth promoters in agriculture: history and mode of action. Poult Scie. (2005) 84:634–43. doi: 10.1093/ps/84.4.634
91. Gaskins, HR, Collier, CT, and Anderson, DB. Antibiotics as growth promotants: mode of action. Anim Biotechnol. (2002) 13:29–42. doi: 10.1081/ABIO-120005768
92. Apajalahti, J, Kettunen, A, and Graham, H. Characteristics of the gastrointestinal microbial communities, with special reference to the chicken. Worlds Poult Sci J. (2004) 60:223–32. doi: 10.1079/WPS200415
93. Celi, P, Cowieson, AJ, Fru-Nji, F, Steinert, RE, Kluenter, A-M, and Verlhac, V. Gastrointestinal functionality in animal nutrition and health: new opportunities for sustainable animal production. Anim Feed Sci Technol. (2017) 234:88–100. doi: 10.1016/j.anifeedsci.2017.09.012
94. Huber, N, Andraud, M, Sassu, EL, Prigge, C, Zoche-Golob, V, Käsbohrer, A, et al. What is a biosecurity measure? A definition proposal for animal production and linked processing operations. One Health. (2022) 15:100433. doi: 10.1016/j.onehlt.2022.100433
95. Moore, DA, Merryman, ML, Hartman, ML, and Klingborg, DJ. Comparison of published recommendations regarding biosecurity practices for various production animal species and classes. J Am Vet Med Assoc. (2008) 233:249–56. doi: 10.2460/javma.233.2.249
96. Tabler, T. (2020). Improved biosecurity and extension programming can benefit African chicken production. Available at: https://extension.msstate.edu/sites/default/files/publications/publications/P3505_web.pdf (Accessed March 14, 2022).
97. Alders, RG, Dumas, SE, Rukambile, E, Magoke, G, Maulaga, W, Jong, J, et al. Family poultry: multiple roles, systems, challenges, and options for sustainable contributions to household nutrition security through a planetary health lens. Matern Child Nutr. (2018) 14:e12668. doi: 10.1111/mcn.12668
98. Katakam, KK, Nejsum, P, Kyvsgaard, NC, Jørgensen, CB, and Thamsborg, SM. Molecular and parasitological tools for the study of Ascaridia galli population dynamics in chickens. Avian Pathol. (2010) 39:81–5. doi: 10.1080/03079451003599284
99. Tarbiat, B, Jansson, DS, Tyden, E, and Hoglund, J. Evaluation of Benzimidazole resistance status in Ascaridia Galli. Parasitology. (2017) 144:1338–45. doi: 10.1017/s0031182017000531
Keywords: Ascaridia galli, chickens, helminth, intestinal microbiota, microbiota, nematodes
Citation: Nemathaga M, Smith RM and Malatji DP (2023) Interactions between the helminth and intestinal microbiome in smallholder chicken farming systems. Front. Vet. Sci. 10:1309151. doi: 10.3389/fvets.2023.1309151
Received: 07 October 2023; Accepted: 24 November 2023;
Published: 19 December 2023.
Edited by:
Nicola Pugliese, University of Bari Aldo Moro, ItalyReviewed by:
Christi L. Swaggerty, Agricultural Research Service (USDA), United StatesCopyright © 2023 Nemathaga, Smith and Malatji. This is an open-access article distributed under the terms of the Creative Commons Attribution License (CC BY). The use, distribution or reproduction in other forums is permitted, provided the original author(s) and the copyright owner(s) are credited and that the original publication in this journal is cited, in accordance with accepted academic practice. No use, distribution or reproduction is permitted which does not comply with these terms.
*Correspondence: Dikeledi P. Malatji, bWFsYXRkcEB1bmlzYS5hYy56YQ==
Disclaimer: All claims expressed in this article are solely those of the authors and do not necessarily represent those of their affiliated organizations, or those of the publisher, the editors and the reviewers. Any product that may be evaluated in this article or claim that may be made by its manufacturer is not guaranteed or endorsed by the publisher.
Research integrity at Frontiers
Learn more about the work of our research integrity team to safeguard the quality of each article we publish.