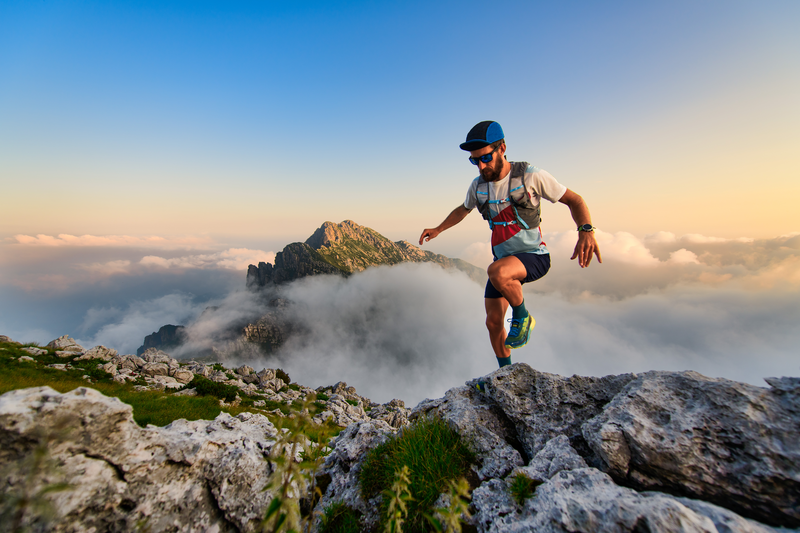
95% of researchers rate our articles as excellent or good
Learn more about the work of our research integrity team to safeguard the quality of each article we publish.
Find out more
ORIGINAL RESEARCH article
Front. Vet. Sci. , 06 December 2023
Sec. Animal Nutrition and Metabolism
Volume 10 - 2023 | https://doi.org/10.3389/fvets.2023.1282055
This article is part of the Research Topic Nutrition Regulation and Stress in Ruminant View all 18 articles
Calf diarrhea causes huge economic losses to livestock due to its high incidence and mortality rates. Alkaline mineral complex water is an alkaline solution containing silicon, sodium, potassium, zinc, and germanium, and has biological benefits and therapeutic effects. This study aimed to evaluate the impact of alkaline mineral complex water supplementation on the health of calves and to investigate the effect of Alkaline mineral complex water supplementation on neonatal calf serum variables and the liver transcriptome. Sixty Holstein calves (age 1.88 ± 0.85 days, weight 36.63 ± 3.34 kg) were selected and randomly divided into two groups: the T group (treatment group with alkaline mineral complex water supplemented during the experiment) and C group (control group without alkaline mineral complex water supplementation). Alkaline mineral complex water supplementation significantly increased the body weight for calves aged 60 d and average daily gain during the experimental period (1–60 d). In addition, Alkaline mineral complex water supplementation could significantly decrease the diarrhea rate for calves aged 16–30 d, enhance the T-AOC, IgG, IGF-1, and IGFBP-2 in concentrations. The results of KEGG enrichment analysis in transcriptomics indicate that Alkaline mineral complex water supplementation inhibited the target IL-1B gene of the NF-kappa B signaling pathway of liver. Alkaline mineral complex water supplementation decreased calf diarrhea and improved partial immune function, anti-inflammatory activity, antioxidant capacity, and health of calves. Alkaline mineral complex is a candidate to replace medicated feed additives. Alkaline mineral complex waterAlkaline mineral complex waterAlkaline mineral complex waterAlkaline mineral complex waterAlkaline mineral complex waterAlkaline mineral complex waterAlkaline mineral complex water.
In contemporary farm production, it is well-established that farm animals undergo a series of stressors post-birth, significantly impacting their overall welfare. These stressors encompass a range of factors, including separation from maternal animals (1), the stress of transportation (2), challenges posed by the farm environment (3), the stress of weaning (4), antibiotic usage (5), heat stress (6) and adjustments in their dietary regimen (6). Newborn animals, in particular, exhibit heightened vulnerability to these stressors and require a higher level of care and attention to ensure their well-being (7, 8). Adequate nutrition of calves is a fundamental requirement for efficient production in later life. Suboptimal nutrition before weaning could have detrimental long-term effects on metabolic health and could thereby decrease production efficiency (7).
Water is an essential component of the diet and plays an important role in animal nutrition (9). Natural alkaline mineral water usually contains sodium, potassium, zinc, metasilicic acid, and other rare minerals (10). These alkaline minerals are rich in inorganic components in biota and play key roles in physiological metabolism and catalysis (11). Alkaline mineral water or mineral supplementation can improve the quality of life of patients with diarrhea-predominant irritable bowel syndrome and improve the gut health of young animals (12, 13). Calf diarrhea (CD) is a common stress disease associated with livestock production (14). Calf diarrhea causes huge economic losses to livestock due to its high incidence and mortality rates (15, 16). Antibiotics are routinely used to treat CD (17); however, excessive use of antibiotics can lead to various side effects, the most significant of which is infection by multidrug-resistant bacteria, which is increasing world-wide (18). Therefore, it is critical to find a low-cost, convenient and without side effect method to improve CD and calf immunity.
Alkaline mineral complex (AMC) water has biological benefits and therapeutic effects, including improving the quality of life of patients with cancer, conferring anti-oxidative effects, promoting intestinal health, and treating intestinal inflammatory diseases and diarrhea (12, 19, 20). The Alkaline mineral complex water used in this study was an alkaline solution (pH 9.1) containing silicon, sodium, potassium, zinc, and germanium, and its functions were based on its mineral composition (21). These minerals are essential for various physiological functions [such as digestion and immune biosynthesis in animals (22, 23)]. The liver is a key metabolic and central immune organ (24). Research has shown that the liver responds to these minerals, affecting their immune function (25). Recent research has shown that Alkaline mineral complex water can improve piglet diarrhea. Study of Chen et al. (26) showed that Alkaline mineral complex water (as used in this study) can improve intestinal morphology and inflammatory reactions, promote intestinal health, and accelerate the growth performance of weaned piglets. In addition, Alkaline mineral complex waterAlkaline mineral complex water can improve the structure and function of intestinal microflora, maintain intestinal epithelial regeneration, and improve the ability of piglets to recover from diarrhea (21, 27). In this experiment, we expected Alkaline mineral complex water supplementation to improve CD, enhance partial immune function, and promote their growth, development, and health. As calves are born with an esophageal sulcus reflex, their digestive mode is similar to that of non-ruminants (28). At present, it is known that Alkaline mineral complex water could improve diarrhea and intestinal immune function. However, as the largest immune organ in the liver, it is unclear how the expression of liver function genes and participation in immunity can be enhanced by Alkaline mineral complex water supplementation. In addition, the value of AMC in ruminants has not yet been explored. There has been no research on AMC in ruminants, and the effect of Alkaline mineral complex water on the liver transcriptome has not yet been studied. Hence, our study aimed to evaluate the impact of Alkaline mineral complex water supplementation on the health of calves and to investigate the effect of Alkaline mineral complex water supplementation on calf serum variables and the liver transcriptome.
All experimental methods and humane endpoints for decreasing pain in animals were performed after approval by the Experimental Animal Welfare and Animal Experiment Ethics Review Committee of China Agricultural University (Approval No. AW01103202-1-32). Animal research was conducted in accordance with State Council Order No. 676 of the People’s Republic of China (Regulations on the Administration of Experimental Animals).
The experiment was conducted on a commercial dairy farm in Yinchuan, Ningxia, China. According to the principle of similar body weight (BW), and age (1–3 d), 60 neonatal Holstein calves aged 1.88 ± 0.85 d (mean ± SD) and weighing 36.63 ± 3.34 kg BW were selected. Briefly, according to a completely randomized design, 60 neonatal calves were randomly divided into two groups in the experimental cattle farm: the T group (treatment group with alkaline mineral complex water supplemented during the experiment) and C group (control group without alkaline mineral complex water supplementation), with 30 calves (including 10 male calves and 20 female calves) in each group. All experimental calves were housed in individual hutches (each hutch has an area of at least 2.5 × 1.6 m). Calves could have direct visual and tactile contact, and have hay bedding. Calves were fed at 06:00 and 16:00 daily on the farm, and the daily liquid feed rate during the experimental period was 4 L/calf at 1–7 d of age, 6 L/calf at 8–14 d, 8 L/calf at 15–25 d, and 10 L/calf at 26–50 d; this was reduced by 2 L/calf every 2 d between 51 and 60 days of age until weaning. Pelleted feed was added to calves at 31 days of age. The water and pellets were changed daily during feeding to ensure the pellets were clean and fresh. To ensure that there was no interference from other mineral elements in this experiment, pure drinking water was used. The experiment included 7 d of adaptation, followed by 53 d (until weaning) of formal experimentation. During the 16–30 d experimental period, two calves in the C group died because they were unable to recover from diarrheal symptoms.
Experimental diet according to Nutrient Requirements of Dairy Cattle (NRC) (29). The ingredients and nutrient compositions of the experimental diets and liquid feed are listed in Supplementary Tables S1–S3. AMC is a liquid colloidal substance that is soluble in water, and it contains Na2SiO3, ZnO, Ge-132. Alkaline mineral complex water was supplemented using a continuous syringe during feeding in the afternoon during the experimental period (Supplementary Figure S1). The calves were fed twice at 06:00 and 16:00 and had free access to water. The liquid feed for 1–7 d of age of calves was fed in bottles with rubber nipples, and the calves were guided to use buckets for liquid feed. Calves after 8 days of age consume liquid feed in buckets. According to Chen et al. (26), based on the nutritional needs of calves, Alkaline mineral complex water supplementation should be 5 mL per cow per day. All the raw materials for AMC were provided by Nail Biotechnology Co., Ltd. (Beijing, China). The compositions of the AMC are listed in Table 1. Both pellet feed (Borui Technology Co., Ltd., Changchun, China) and feeding milk (Nutrifeed Co., Ltd., Veghel, Netherlands) were commercial feed. The mixing ratio of feeding milk was: (milk replacer 1: water 7): normal milk =1:1. All feed implemented Current National Standards and Industry Standards for Feed Industry of China. The feed provided in this study was carefully monitored to ensure that aflatoxin levels were well below the established safety limits for animal feed.
BW was measured at birth and every month thereafter. Body weight was measured by weighing the calves 2 h before the morning feeding (when the calves’ stomachs were empty). The weight of the pellet feed was measured before feeding (06:00) every day, and the pellet feed intake of the previous day for calves was calculated. The severity of diarrhea in the experimental calves was graded according to the consistency of feces. The fecal scores were as follows:
1 = normal (retains shape), 2 = soft (flows across a surface), 3 = muddy (liquid), and 4 = severe diarrhea (very watery) (14).
Diarrhea rate (%) = (total number of calves with diarrhea × days of diarrhea)/(total number of experimental calves × experimental period days) × 100 (30).
One independently trained observer collected the fecal score data. Calves were defined as having diarrhea with scores of 3 or 4.
Ten calves from each experimental group were randomly selected for blood analysis. Blood samples were collected as described by Liu et al. (30). On days 1, 15, 30, 45, and 60 of the experimental period, blood was collected through jugular puncture using a vacuum blood collection vessel without an anticoagulant 2 h after morning feeding. Blood samples were centrifuged at 3,000 × g for 15 min at 4°C to collect serum and stored in 1.5 mL microcentrifuge tubes at −80°C for later analysis.
According to the radioimmunoassay method, insulin (INS) and growth hormone (GH) were analyzed with a measuring instrument (BFM-96, Zhongcheng Electromechanical Technology Development Co., Ltd., Hefei, China). Total cholesterol (TC), blood urea nitrogen (BUN), alkaline phosphatase (ALP), total protein (TP), albumin (ALB), globulin (GLB), malondialdehyde (MDA), glutathione peroxidase (GSH-PX), superoxide dismutase (SOD) and total antioxidant capacity (T-AOC) were determined according to the manufacturer’s instructions, using commercial reagent kits (Nanjing Jiancheng Co., Ltd., Nanjing, China) and an automatic biochemical analyzer (CLS880, Zecen Biotechnology Co., Ltd., Jiangsu, China). The β-hydroxybutyric acid (BHBA), nonestesterified fatty acid (NEFA), immunoglobulin G (IgG), insulin-like growth factor-1 (IGF-1), insulin-like growth factor binding protein-2 (IGFBP-2), zonula occludens-1 (ZO-1), endothelin-1 (ET-1), diamine oxidase (DAO) and D-lactic acid (D-LA) were analyzed using ELISA kits (Abcam, Cambridge, U K) with a microplate reader (Thermo Multiskan Ascent, Thermo Fisher Scientific, Shanghai, China).
Ten calves were randomly selected for humane slaughter at 60 d of the experimental period (five calves in Group C and five calves in Group T). After slaughtering, liver samples were collected from the same areas of calves in the C and T groups. Liver sample collection was done according to the method of Zhao et al. (31). Briefly, liver samples were quickly sectioned, washed with precooled PBS (4°C), placed in 2 mL sterile cryopreservation tubes, quickly frozen in liquid nitrogen, and then stored at −80°C for further analysis.
According to the manufacturer’s instructions (Invitrogen, Carlsbad, CA, United States), total RNA was extracted from liver tissue using TRIzol® Reagent and genomic DNA was removed using DNase I (TaKara, Kusatsu, Japan). RNA degradation and contamination were monitored by 1% agarose gel electrophoresis. RNA quality was determined using a 2,100 Biological Analyzer (Agilent Technologies, Santa Clara, CA, United States) and quantified using an ND-2000 (NanoDrop Technologies, Wilmington, DE, United States). Only high-quality RNA samples (OD260/280 = 1.8–2.2, OD260/230 ≥ 2.0, RIN ≥ 8.0, 28S:18S ≥ 1.0 > 1 μg) were used to construct the sequencing library.
RNA purification, reverse transcription, library construction, and sequencing were performed at Shanghai Majorbio Bio-pharm Biotechnology Co., Ltd. (Shanghai, China), according to the manufacturer’s instructions (Illumina, San Diego, CA, United States). The transcriptome library was prepared using the TruSeqTM RNA sample preparation Kit from Illumina (San Diego, CA, USA) using 1 μg of total RNA. Briefly, mRNA was isolated according to the poly(A) selection method using oligo(dT) beads and then fragmented using fragmentation buffer. Next, double-stranded cDNA was synthesized using a SuperScript double-stranded cDNA synthesis kit (Invitrogen) with random hexamer primers (Illumina). Then the synthesized cDNA was subjected to end-repair, phosphorylation and ‘A’ base addition, according to Illumina’s library construction protocol. Libraries were selected for cDNA target fragments of 300 bp on 2% low-range ultra-agarose, followed by PCR amplification using Phusion DNA polymerase (NEB) for 15 PCR cycles. After quantification using TBS380, the paired-end RNA-seq library was sequenced using an Illumina NovaSeq 6000 sequencer (2 × 150 bp read length).
The data displayed in the tables and figures represent the mean ± standard error of the mean (SEM). Before conducting statistical analysis, the normality and homogeneity of data of average daily gain, feed intake and serum variables were tested based on the description of Marta et al. (32). The effects of Alkaline mineral complex water supplementation on serum variables in neonatal calves were assessed using SAS software (version 9.4; SAS Institute Inc., Cary, NC, United States). According to the following model, a block experimental design was used for the time, group, and interaction effects between treatment and group:
Y is the dependent variable, μ is the overall mean, Ti is the time effect, Gj is the treatment effect, TGij is the interaction effect between T and G, Eijl is the random residual error. Statistical significance was set at p < 0.05. different. One-way analysis of variance using SAS 9.4, the statistical significance of the effect of Alkaline mineral complex water supplementation on se-rum variables. Differences were considered statistically significant at p < 0.05, and trends were declared at 0.05 ≤ p ≤ 0.10.
The source of the reference gene was Bos taurus, the version of the reference genome was ARS-UCD1.2, and the source of the reference genome was http://asia.ensembl.org/Bo. ctaurus/Info/Index. The Clean Reads of each sample were sequenced with the specified reference genome. Transcriptome analysis of liver samples was performed using the Majorbio Cloud Platform (www.majorbio.com) (33). Differential expression analysis was performed using DESeq2, and DEGs with log2(fold change) ≥ 1 and P-adjusted ≤0.05 (DESeq2) were considered to be significant DEGs. Kyoto En-cyclopedia of Genes and Genomes (KEGG) pathway enrichment analysis was per-formed using R software (version 3.3.1). The KEGG pathway function was considered significantly enriched when the p-value was <0.05. All other statistical analyzes were performed using GraphPad Prism version 9 (GraphPad Software).
The effect of alkaline mineral complex water supplementation on the diarrhea rate is shown in Figure 1. Alkaline mineral complex water supplementation significantly decreasedthe diarrhea rate for calves aged 16–30 d (p < 0.05).
Figure 1. Effects of alkaline mineral complex water supplementation on diarrhea rate of calves. C is the control group; T is the treatment group. *p < 0.05. n = 30 per experimental group.
Alkaline mineral complex water supplementation for pellet feed intake is presented in Table 2 and Figure 2. Alkaline mineral complex water supplementation for BW and average daily gain (ADG) are presented in Table 3. AMC supplementation significantly increased the BW for calves aged 60 d (p < 0.05) and ADG during the experimental period (1–60 d) (p < 0.05). In addition, due to the natural characteristics of calves, they prefer milk more. All liquid feed calves fed daily can be consumed.
Table 2. Effects of alkaline mineral complex water supplementation on pellets feed intake in calves.
Figure 2. Effects of alkaline mineral complex water supplementation on pellets feed intake of calves. C is the control group; T is the treatment group. n = 30 per experimental group.
Table 3. Effects of alkaline mineral complex water supplementation on body weight and average daily gain in calves.
The effects of alkaline mineral complex water supplementation on serum variables are presented in Table 4. Compared to calves in the T and C groups, alkaline mineral complex water supplementation significantly reduced BUN levels during the experimental period (p < 0.05) and significantly increased T-AOC and IgG levels (p < 0.05). A significant interaction was observed between the experimental groups and AMC supplementation time for GLB, IGF-1, IGFBP-2, and ET-1 (p < 0.05). Further analysis of serum variable data revealed that alkaline mineral complex water supplementation significantly increased IGF-1 at 30 and 45 days of the experimental period and IGFBP-2 at 45 d of experimental period (p < 0.05) (Figure 3), while significantly decreasing GLB and IGFBP-2 at 15 d and ET-1 at 30 d of experimental period (p < 0.05) (Figure 3).
Figure 3. Effects of alkaline mineral complex water supplementation on serum variables in calves. (A) GLB, (B) IGF-1, (C) IGFBP-2, (D) ET-1. C is the control group; T is the treatment group. Data are presented as the means ± SEM (n = 10 per experimental group). *p < 0.05.
A total of 19,019 genes were detected and tested for differential expression in the liver samples, and 30 were differentially expressed between the T and C groups (p < 0.05) (Figure 4). Table 3 lists the significant DEGs in the liver samples of the calves; some unnamed genes are not listed. Respectively, DDIT4, TAT, NOCT, FOSB, SPRY3, LU-RAP1L, ARHGEF26, DUSP1, FHIT, et al. and GSTT4, IL1B, DUSP6, MID1IP1, OSGIN1, COBL, TFAP4, et al. transcripts were up and downregulated in the T group compared to the C group (Table 5).
Figure 4. Volcano map of differentially expressed genes in the liver of calves at 60 d of the experimental period. The horizontal coordinate is the multiple of gene/transcript expression difference between the two samples, namely the value obtained by dividing the amount expressed by treated samples by the expression amount of control samples; the vertical coordinate is the statistical test value of gene expression difference, namely the p value. The larger the −log10(p-value) is, the more significant the difference in ex-pression is. Both the horizontal and vertical values are logized. Each dot in the figure represents a specific gene, with red, blue, and gray dots representing significantly up-regulated, significantly downregulated, and non-significant genes, respectively. The points on the left and right are genes with downregulated and upregulated expression, respectively. The closer to the two sides and the point on the top, the more significant the expression difference.
Table 5. Significantly differentially expressed genes in the liver samples of calves at 60 d of the experimental period.
The KEGG enrichment analysis results for Alkaline mineral complex water supplementation are presented in Figure 5. The significant enrichment pathway was the NF-kappa B signaling pathway (P-adjusted <0.05). The MAPK signaling pathway and transcriptional mis-regulation in cancer tended to be significantly enriched, although these changes were not statistically significant (0.05 ≤ p ≤ 0.10).
Figure 5. Kyoto Encyclopedia of Genes and Genomes enrichment analysis in the liver samples of 60 days of the experimental period of calves. The vertical axis represents the pathway names, and the horizontal axis represents the ratio of the enrichment factor (sample number of genes/transcripts enriched in this pathway to the background number of annotated genes/transcripts). The larger the enrichment factor, the greater the degree of enrichment. The size of dots indicates the number of genes in this pathway, and the colors of the dots correspond to different P-adjusted ranges. The enrichment results of top 20 were displayed when P-adjusted <0.05.
Alkaline mineral complex water is rich in multiple trace elements. Although the role of AMC in non-ruminant is generally considered positive, its effectiveness in cattle farming has not yet been confirmed. In this study, we evaluated the impact of alkaline mineral complex water supplementation on the health of calves and investigated the effect of alkaline mineral complex water supplementation on production performance, serum variables, and the liver transcriptome. Our results indicated that alkaline mineral complex water supplementation could improve the health and promote the growth of calves.
The CD rate is related to age; calves are at a high risk for CD and death in early life, and CD in the first 3 weeks of life is a major cause of death (34, 35). With the growth and development of calves, their immunity improves and their CD rate decreases (36, 37). Alkaline mineral complex water contains silicates, which have anti-inflammatory properties and can effectively treat diarrhea (38). In this study, alkaline mineral complex water supplementation decreased the CD rate at 16–30 d of the experimental period. Alkaline mineral complex water supplementation had no significant effect in other experimental periods, possibly because Alkaline mineral complex water the immunity of calves improved with their growth and development after 30 d.
Feeding and management of calves at a young age are crucial for the growth, health, and future production performance of dairy cows. The BW of calves reflects their growth and development and is related to the incidence of diseases, reproductive performance, and milk yield (39). CD could have an impact on the BW of calves, which is negatively correlated with BW even after recovery from CD, and the ADG of calves could decrease (40). In this study, Alkaline mineral complex water supplementation increased BW and ADG. Feed palatability is a critical factor influencing feed intake and, subsequently, animal performance (41). In this experiment, calves could quickly consume all liquid feed every day due to their preference for milk. And there was no difference in the intake of pellet feed for calves. Therefore, AMC supplementation did not interfere with the normal feeding of calves in this experiment. In particular, Alkaline mineral complex water supplementation significantly increased the BW of calves aged 60 d and significantly increased the ADG during the experimental period (1–60 d). In this experiment, the decrease in CD and the increase in feed intake may be the reasons for the increase in calf BW and ADG. Increases in BW and ADG are of great significance for future calf production.
BUN is an important indicator of the balance between amino acid and protein metabolism in animals. Coma et al. (42) showed that animals with lower BUN concentrations had normal amino acid metabolism and higher protein synthesis rates. In this study, AMC supplementation significantly reduced BUN levels during the experimental period, which may indicate that AMC supplementation can improve the utilization rate of dietary protein in calves. This may be one of the reasons for the increase in BW and ADG during the experimental period.
Alkaline mineral complex water is an excellent source of silicon and zinc; these elements are related to the immune system and have anti-inflammatory and antioxidant effects (23, 43–45). Excessive serum globulin levels are associated with inflammation (46). Endothelial damage may increase ET-1 production (47). Alkaline mineral complex water supplementation significantly reduced GLB and ET-1, especially on days 15 and 30 of the experiment. Calves were most vulnerable and had the highest rates of CD in the period within 30 d after their birth. IgG can indicate the strength and weakness of the immune system, and the survival and well-being of calves are strongly dependent on IgG (48). Total antioxidant capacity represents the total antioxidant levels of various antioxidant substances and enzymes (49). In this study, AMC supplementation significantly increased T-AOC and IgG levels. Changes in these serum variables could imply that Alkaline mineral complex water has immunomodulator, anti-inflammatory, and antioxidant effects on calves and that alkaline mineral complex water supplementation improved their health. In addition, AMC supplementation promoted calf growth. AMC supplementation significantly increased IGF-1 and IGFBP-2 levels, particularly in the later period of the experiment. IGF-1 and IGFBP-2 are growth-promoting factors that costimulate osteoblast differentiation (50, 51); increases in BW and ADG confirmed this result. IGF signal transduction is regulated by conserved members of the IGFBP family. IGFBP-5 is a multifunctional protein that conditionally regulates IGF signaling as a molecular switch (52). In the present study, the increase in IGF-1 and IGFBP-2 levels in the treatment group may have been due to the significant upregulation of IGFBP-5. However, the specific regulatory mechanism of IGFBP-5 gene is still unclear and warrant further investigation.
The liver is the largest organ in the body and plays an important role in the immune system (53). NF-kappa B signaling pathway is a highly conserved evolutionary pathway that plays important functions in regulating immune and inflammatory responses (54). In the liver, NF-kappa B signaling pathway is an important transcriptional regulator of inflammatory response and plays an essential role in regulating liver inflammatory signaling pathways (55). In this study, alkaline mineral complex water supplementation significantly regulated the NF-kappa B signaling pathway in the liver. Chen et al. found that Alkaline mineral complex water could target the inhibition of NF-kappa B signaling pathway through microbial-intestinal interaction (27). IL-1B, one of the main cytokines involved in the pathogenesis of many inflammation-related diseases, and it can regulate the NF-kappa B signaling pathway (56, 57). In this study, alkaline mineral complex water supplementation significantly downregulated the IL-1B gene Research showed that trace elements that are abundant in alkaline mineral complex water could inhibit the IL-1B gene (58). This study showed that alkaline mineral complex water supplementation could inhibit the target genes of the NF-kappa B signaling pathway, affected the immune function of the liver, and improved the health of calves.
Calves, especially female calves, play a crucial role in the future of any herd, making them an important component of dairy farms. Raising calves requires a significant investment of resources and time to achieve profitability (59). Therefore, raising calves requires consideration of cost. Some limitations need to be acknowledged. Although alkaline mineral complex can be proven beneficial for calves, it is necessary to continuously supplement alkaline mineral complex before weaning. If large-scale fed can reduce the number of days for alkaline mineral complex supplementation, it can save costs. The cost of commercial alkaline mineral complex supplementation in calves does not exceed 0.04 dollars per calf per d, indicating that alkaline mineral complex is more economical compared to regular antibiotics. In the future, emphasis should be placed on determining the number of days calves are supplemented with alkaline mineral complex to save more costs.
Under the experimental conditions applied, Alkaline mineral complex water supplementation promoted the growth and health of the calves, possibly by enhancing T-AOC, IgG, IGF-1, and IGFBP-2, thereby improving growth-promoting factors, antioxidant status, and partial immune function in calves. These effects decrease CD and promote the growth of calves. It is worth noting that Alkaline mineral complex water supplementation could inhibit the target IL-1B gene of the NF-kappa B signaling pathway, affect the immune and anti-inflammatory functions of the liver, and improve the health of calves. Overall, alkaline mineral complex water supplementation decreased calf diarrhea and improved, partial immune function, anti-inflammatory activity, antioxidant capacity, and health of calves; thus, it is a candidate to replace medicated feed additives.
The datasets presented in this study can be found in online repositories. The names of the repository/repositories and accession number(s) can be found at: https://www.ncbi.nlm.nih.gov/bioproject; PRJNA1008432.
The animal study was approved by Experimental Animal Welfare and Animal Experiment Ethics Committee of China Agricultural University. The study was conducted in accordance with the local legislation and institutional requirements.
CG: Conceptualization, Data curation, Investigation, Methodology, Writing – original draft. XW: Investigation, Methodology, Validation, Writing – review & editing. DD: Investigation, Resources, Writing – review & editing. FK: Writing – review & editing. SW: Writing – review & editing. XS: Data curation, Software, Writing – review & editing. SL: Funding acquisition, Project administration, Supervision, Writing – review & editing. XX: Methodology, Project administration, Resources, Writing – review & editing. LZ: Methodology, Resources, Writing – review & editing.
The author(s) declare financial support was received for the research, authorship, and/or publication of this article. This research was funded by Key Research and Development Project of Ningxia Hui Autonomous Region (2018BBF33006) and earmarked fund for CARS36.
The authors wish to acknowledge Shanghai Majorbio Bio-pharm Technology Co., Ltd. for help in sequencing technology of the samples of this study.
The authors declare that the research was conducted in the absence of any commercial or financial relationships that could be construed as a potential conflict of interest.
All claims expressed in this article are solely those of the authors and do not necessarily represent those of their affiliated organizations, or those of the publisher, the editors and the reviewers. Any product that may be evaluated in this article, or claim that may be made by its manufacturer, is not guaranteed or endorsed by the publisher.
The Supplementary material for this article can be found online at: https://www.frontiersin.org/articles/10.3389/fvets.2023.1282055/full#supplementary-material
1. Webb, LE, Marcato, F, Bokkers, E, Verwer, CM, Wolthuis-Fillerup, M, Hoorweg, FA, et al. Impact of early dam contact on veal calf welfare. Sci Rep. (2022) 12:22144. doi: 10.1038/s41598-022-25804-z
2. Sardi, L, Gastaldo, A, Borciani, M, Bertolini, A, Musi, V, Garavaldi, A, et al. Pre-Slaughter sources of fresh meat quality variation: the case of heavy pigs intended for protected designation of origin products. Animals (Basel). (2020) 10:2386. doi: 10.3390/ani10122386
3. Roadknight, N, Mansell, P, Jongman, E, Courtman, N, and Fisher, A. Invited review: The welfare of young calves transported by road. J Dairy Sci. (2021) 104:6343–57. doi: 10.3168/jds.2020-19346
4. Vickery, HM, Neal, RA, Stergiadis, S, and Meagher, RK. Gradually weaning goat kids may improve weight gains while reducing weaning stress and increasing creep feed intakes. Front Vet Sci. (2023) 10:1200849. doi: 10.3389/fvets.2023.1200849
5. Masebo, NT, Marliani, G, Cavallini, D, Accorsi, PA, Di Pietro, M, Beltrame, A, et al. Health and welfare assessment of beef cattle during the adaptation period in a specialized commercial fattening unit. Res Vet Sci. (2023) 158:50–5. doi: 10.1016/j.rvsc.2023.03.008
6. Giorgino, A, Raspa, F, Valle, E, Bergero, D, Cavallini, D, Gariglio, M, et al. Effect of dietary organic acids and botanicals on metabolic status and milk parameters in mid-late lactating goats. Animals (Basel). (2023) 13:797. doi: 10.3390/ani13050797
7. Kenéz, Á, Koch, C, Korst, M, Kesser, J, Eder, K, Sauerwein, H, et al. Different milk feeding intensities during the first 4 weeks of rearing dairy calves: Part 3: Plasma metabolomics analysis reveals long-term metabolic imprinting in Holstein heifers. J Dairy Sci. (2018) 101:8446–60. doi: 10.3168/jds.2018-14559
8. Eckert, E, Brown, HE, Leslie, KE, DeVries, TJ, and Steele, MA. Weaning age affects growth, feed intake, gastrointestinal development, and behavior in Holstein calves fed an elevated plane of nutrition during the preweaning stage. J Dairy Sci. (2015) 98:6315–26. doi: 10.3168/jds.2014-9062
9. Miller, JD, Workman, CL, Panchang, SV, Sneegas, G, Adams, EA, Young, SL, et al. Water security and nutrition: current knowledge and research opportunities. Adv Nutr. (2021) 12:2525–39. doi: 10.1093/advances/nmab075
10. Bertoni, M, Olivieri, F, Manghetti, M, Boccolini, E, Bellomini, MG, Blandizzi, C, et al. Effects of a bicarbonate-alkaline mineral water on gastric functions and functional dyspepsia: a preclinical and clinical study. Pharmacol Res. (2002) 46:525–31. doi: 10.1016/s1043661802002323
11. Nan, H, Zhao, L, Yang, F, Liu, Y, Xiao, Z, Cao, X, et al. Different alkaline minerals interacted with biomass carbon during pyrolysis: Which one improved biochar carbon sequestration? J Clean Prod. (2020) 255:120162. doi: 10.1016/j.jclepro.2020.120162
12. Shin, DW, Yoon, H, Kim, HS, Choi, YJ, Shin, CM, Park, YS, et al. Effects of alkaline-reduced drinking water on irritable bowel syndrome with diarrhea: a randomized double-blind, placebo-controlled pilot study. Evid Based Complement Alternat Med. (2018) 2018:9147914. doi: 10.1155/2018/9147914
13. Shannon, MC, and Hill, GM. Trace mineral supplementation for the intestinal health of young monogastric animals. Front Vet Sci. (2019) 6:73. doi: 10.3389/fvets.2019.00073
14. Islam, J, Tanimizu, M, Shimizu, Y, Goto, Y, Ohtani, N, Sugiyama, K, et al. Development of a rational framework for the therapeutic efficacy of fecal microbiota transplantation for calf diarrhea treatment. Microbiome. (2022) 10:31. doi: 10.1186/s40168-021-01217-4
15. Huang, MZ, Cui, DA, Wu, XH, Hui, W, Yan, ZT, Ding, XZ, et al. Serum metabolomics revealed the differential metabolic pathway in calves with severe clinical diarrhea symptoms. Animals (Basel). (2020) 10:10. doi: 10.3390/ani10050769
16. Wu, YY, Nie, CX, Xu, C, Luo, RQ, Chen, HL, Niu, JL, et al. Effects of dietary supplementation with multispecies probiotics on intestinal epithelial development and growth performance of neonatal calves challenged with Escherichia coli K99. J Sci Food Agric. (2022) 102:4373–83. doi: 10.1002/jsfa.11791
17. Eibl, C, Bexiga, R, Viora, L, Guyot, H, Felix, J, Wilms, J, et al. The antibiotic treatment of calf diarrhea in four european countries: a survey. Antibiotics (Basel). (2021) 10:910. doi: 10.3390/antibiotics10080910
18. Uddin, TM, Chakraborty, AJ, Khusro, A, Zidan, B, Mitra, S, Emran, TB, et al. Antibiotic resistance in microbes: History, mechanisms, therapeutic strategies and future prospects. J Infect Public Health. (2021) 14:1750–66. doi: 10.1016/j.jiph.2021.10.020
19. Costantino, M, Conti, V, Corbi, G, and Filippelli, A. Hydropinotherapy with sulphurous mineral water as complementary treatment to improve glucose metabolism, oxidative status, and quality of life. Antioxidants (Basel). (2021) 10:1773. doi: 10.3390/antiox10111773
20. Pavelic, K, Hadzija, M, Bedrica, L, Pavelic, J, Dikic, I, Katic, M, et al. Natural zeolite clinoptilolite: new adjuvant in anticancer therapy. J Mol Med (Berl). (2001) 78:708–20. doi: 10.1007/s001090000176
21. Chen, J, Zhao, BC, Dai, XY, Xu, YR, Kang, JX, and Li, JL. Drinking alkaline mineral water confers diarrhea resistance in maternally separated piglets by maintaining intestinal epithelial regeneration via the brain-microbe-gut axis. J Adv Res. (2022) 52:29–43. doi: 10.1016/j.jare.2022.12.008
22. Bao, YM, and Choct, M. Trace mineral nutrition for broiler chickens and prospects of application of organically complexed trace minerals: a review. Anim Prod Sci. (2009) 49:269. doi: 10.1071/EA08204
23. Jurkic, LM, Cepanec, I, Pavelic, SK, and Pavelic, K. Biological and therapeutic effects of ortho-silicic acid and some ortho-silicic acid-releasing compounds: New perspectives for therapy. Nutr Metab (Lond). (2013) 10:2. doi: 10.1186/1743-7075-10-2
24. Li, J, Yin, LM, Wang, L, Li, JZ, Huang, PF, Yang, HS, et al. Effects of vitamin B6 on growth, diarrhea rate, intestinal morphology, function, and inflammatory factors expression in a high-protein diet fed to weaned piglets. J Anim Sci. (2019) 97:4865–74. doi: 10.1093/jas/skz338
25. Kozeniecki, M, Ludke, R, Kerner, J, and Patterson, B. Micronutrients in liver disease: roles, risk factors for deficiency, and recommendations for supplementation. Nutr Clin Pract. (2020) 35:50–62. doi: 10.1002/ncp.10451
26. Chen, J, Xu, YR, Kang, JX, Zhao, BC, Dai, XY, Qiu, BH, et al. Effects of alkaline mineral complex water supplementation on growth performance, inflammatory response, and intestinal barrier function in weaned piglets. J Anim Sci. (2022) 100:100. doi: 10.1093/jas/skac251
27. Chen, J, Xu, XW, Kang, JX, Zhao, BC, Xu, YR, and Li, JL. Metasilicate-based alkaline mineral water confers diarrhea resistance in maternally separated piglets via the microbiota-gut interaction. Pharmacol Res. (2023) 187:106580. doi: 10.1016/j.phrs.2022.106580
28. Hegland, RB, Lambert, MR, Jacobson, NL, and Payne, LC. Effect of dietary and managemental factors on reflex closure of the esophageal groove in the dairy calf 1. J Dairy Sci. (1957) 40:1107–13. doi: 10.3168/jds.S0022-0302(57)94602-7
29. NRC. Nutrient requirements of dairy cattle. 7th rev. ed. Washington, DC: Proceedings of the National Academy of Sciences of the United States of America (2001). 381 p.
30. Liu, S, Ma, JY, Zhou, J, Wu, JD, Li, JH, Alugongo, GM, et al. Tributyrin supplementation in pasteurized waste milk: Effects on growth performance, health, and blood parameters of dairy calves. J Dairy Sci. (2021) 104:12496–507. doi: 10.3168/jds.2021-20645
31. Zhao, XW, Zhu, HL, Qi, YX, Wu, T, Huang, DW, Ding, HS, et al. Quantitative comparative phosphoproteomic analysis of the effects of colostrum and milk feeding on liver tissue of neonatal calves. J Dairy Sci. (2021) 104:8265–75. doi: 10.3168/jds.2020-20097
32. Marta, P, Marco, T, Andrea, F, Daniela, F, and Damiano, C. Effect of does parity order on litter homogeneity parameters. Ital J Anim Sci. (2020) 19:1188–94. doi: 10.1080/1828051X.2020.1827990
33. Ren, Y, Yu, G, Shi, C, Liu, L, Guo, Q, Han, C, et al. Majorbio Cloud: A one-stop, comprehensive bioinformatic platform for multiomics analyses. Imeta. (2022) 1:1. doi: 10.1002/imt2.12
34. Kim, HS, Whon, TW, Sung, H, Jeong, YS, Jung, ES, Shin, NR, et al. Longitudinal evaluation of fecal microbiota transplantation for ameliorating calf diarrhea and improving growth performance. Nat Commun. (2021) 12:161. doi: 10.1038/s41467-020-20389-5
35. Pempek, J, Trearchis, D, Masterson, M, Habing, G, and Proudfoot, K. Veal calf health on the day of arrival at growers in Ohio. J Anim Sci. (2017) 95:3863–72. doi: 10.2527/jas2017.1642
36. Kertz, AF, Hill, TM, Quigley, JR, Heinrichs, AJ, Linn, JG, and Drackley, JK. A 100-Year Review: Calf nutrition and management. J Dairy Sci. (2017) 100:10151–72. doi: 10.3168/jds.2017-13062
37. Lora, I, Gottardo, F, Contiero, B, Dall, AB, Bonfanti, L, Stefani, A, et al. Association between passive immunity and health status of dairy calves under 30 days of age. Prev Vet Med. (2018) 152:12–5. doi: 10.1016/j.prevetmed.2018.01.009
38. González, R, Sánchez De Medina, F, Martínez-Augustin, O, Nieto, A, Gálvez, J, Risco, S, et al. Anti-inflammatory effect of diosmectite in hapten-induced colitis in the rat. Br J Pharmacol. (2004) 141:951–60. doi: 10.1038/sj.bjp.0705710
39. Rashad, A, Amin, AH, Mahdy, AE, Aziz, MA, El-Barbary, A, and El-Hedainy, D. The impact of milk suckling protocol and schedule on body weight and some morphometric measurements of Holstein heifers. Trop Anim Health Prod. (2022) 54:187. doi: 10.1007/s11250-022-03182-y
40. Schinwald, M, Creutzinger, K, Keunen, A, Winder, CB, Haley, D, and Renaud, DL. Predictors of diarrhea, mortality, and weight gain in male dairy calves. J Dairy Sci. (2022) 105:5296–309. doi: 10.3168/jds.2021-21667
41. Vinassa, M, Cavallini, D, Galaverna, D, Baragli, P, Raspa, F, Nery, J, et al. Palatability assessment in horses in relation to lateralization and temperament. Appl Anim Behav Sci. (2020) 232:105110. doi: 10.1016/j.applanim.2020.105110
42. Coma, J, Carrion, D, and Zimmerman, DR. Use of plasma urea nitrogen as a rapid response criterion to determine the lysine requirement of pigs. J Anim Sci. (1995) 73:472–81. doi: 10.2527/1995.732472x
43. Kim, EJ, Bu, SY, Sung, MK, Kang, MH, and Choi, MK. Analysis of antioxidant and anti-inflammatory activity of silicon in murine macrophages. Biol Trace Elem Res. (2013) 156:329–37. doi: 10.1007/s12011-013-9829-y
44. Powell, SR. The antioxidant properties of zinc. J Nutr. (2000) 130:1447S–54S. doi: 10.1093/jn/130.5.1447S
45. Prasad, AS. Zinc: an antioxidant and anti-inflammatory agent: role of zinc in degenerative disorders of aging. J Trace Elem Med Biol. (2014) 28:364–71. doi: 10.1016/j.jtemb.2014.07.019
46. Mohri, M, Sharifi, K, and Eidi, S. Hematology and serum biochemistry of Holstein dairy calves: age related changes and comparison with blood composition in adults. Res Vet Sci. (2007) 83:30–9. doi: 10.1016/j.rvsc.2006.10.017
47. Cozza, EN, Gomez-Sanchez, CE, Foecking, MF, and Chiou, S. Endothelin binding to cultured calf adrenal zona glomerulosa cells and stimulation of aldosterone secretion. J Clin Invest. (1989) 84:1032–5. doi: 10.1172/JCI114226
48. Drikic, M, Windeyer, C, Olsen, S, Fu, Y, Doepel, L, and De Buck, J. Determining the IgG concentrations in bovine colostrum and calf sera with a novel enzymatic assay. J Anim Sci Biotechnol. (2018) 9:69. doi: 10.1186/s40104-018-0287-4
49. Wang, S, Hu, F, Diao, Q, Li, S, Tu, Y, and Bi, Y. Comparison of growth performance, immunity, antioxidant capacity, and liver transcriptome of calves between whole milk and plant protein-based milk replacer under the same energy and protein levels. Antioxidants (Basel). (2022) 11:270. doi: 10.3390/antiox11020270
50. Xi, G, Rosen, CJ, and Clemmons, DR. IGF-I and IGFBP-2 Stimulate AMPK activation and autophagy, which are required for osteoblast differentiation. Endocrinology. (2016) 157:268–81. doi: 10.1210/en.2015-1690
51. Morimoto-Kamata, R, Tsuji, D, and Yui, S. Cathepsin g-induced insulin-like growth factor (IGF) elevation in MCF-7 medium is caused by proteolysis of IGF binding protein (IGFBP)-2 but not of IGF-1. Biol Pharm Bull. (2020) 43:1678–86. doi: 10.1248/bpb.b20-00389
52. Duan, C, and Allard, JB. Insulin-like growth factor binding protein-5 in physiology and disease. Front Endocrinol (Lausanne). (2020) 11:100. doi: 10.3389/fendo.2020.00100
53. Bogdanos, DP, Gao, B, and Gershwin, ME. Liver immunology. Compr Physiol. (2013) 3:567–98. doi: 10.1002/cphy.c120011
54. Xiao, C, and Ghosh, S. NF-kappa B, an evolutionarily conserved mediator of immune and inflammatory responses. Adv Exp Med Biol. (2005) 560:41–5. doi: 10.1007/0-387-24180-9_5
55. Luedde, T, and Schwabe, RF. NF-κB in the liver-linking injury, fibrosis and hepatocellular carcinoma. Nat Rev Gastroenterol Hepatol. (2011) 8:108–18. doi: 10.1038/nrgastro.2010.213
56. Zhang, H. Anti-IL-1beta therapies. Recent Pat DNA Gene Seq. (2011) 5:126–35. doi: 10.2174/187221511796392024
57. Cheng, CY, Hsieh, HL, Sun, CC, Lin, CC, Luo, SF, and Yang, CM. IL-1 beta induces urokinase-plasminogen activator expression and cell migration through PKC alpha, JNK1/2, and NF-kappa B in A549 cells. J Cell Physiol. (2009) 219:183–93. doi: 10.1002/jcp.21669
58. Jarosz, M, Olbert, M, Wyszogrodzka, G, Mlyniec, K, and Librowski, T. Antioxidant and anti-inflammatory effects of zinc. Zinc-dependent NF-kappa B signaling. Inflammopharmacology. (2017) 25:11–24. doi: 10.1007/s10787-017-0309-4
59. Cavallini, D, Raspa, F, Marliani, G, Nannoni, E, Martelli, G, Sardi, L, et al. Growth performance and feed intake assessment of Italian Holstein calves fed a hay-based total mixed ration: preliminary steps towards a prediction model. Vet Sci (Basel). (2023) 10:554. doi: 10.3390/vetsci10090554
Keywords: calf, stress, anti-inflammatory activity, antioxidant capacity, liver transcriptome
Citation: Guo C, Wang X, Dai D, Kong F, Wang S, Sun X, Li S, Xu X and Zhang L (2023) Effects of alkaline mineral complex supplementation on production performance, serum variables, and liver transcriptome in calves. Front. Vet. Sci. 10:1282055. doi: 10.3389/fvets.2023.1282055
Received: 23 August 2023; Accepted: 15 November 2023;
Published: 06 December 2023.
Edited by:
Xianwen Dong, Chongqing Academy of Animal Science, ChinaReviewed by:
Yongjiang Wu, Chongqing University of Arts and Sciences, ChinaCopyright © 2023 Guo, Wang, Dai, Kong, Wang, Sun, Li, Xu and Zhang. This is an open-access article distributed under the terms of the Creative Commons Attribution License (CC BY). The use, distribution or reproduction in other forums is permitted, provided the original author(s) and the copyright owner(s) are credited and that the original publication in this journal is cited, in accordance with accepted academic practice. No use, distribution or reproduction is permitted which does not comply with these terms.
*Correspondence: Shengli Li, bGlzaGVuZzA2NzdAMTYzLmNvbQ==; Xiaofeng Xu, eHV4aWFvZmVuZ25kQDEyNi5jb20=
†These authors have contributed equally to this work
Disclaimer: All claims expressed in this article are solely those of the authors and do not necessarily represent those of their affiliated organizations, or those of the publisher, the editors and the reviewers. Any product that may be evaluated in this article or claim that may be made by its manufacturer is not guaranteed or endorsed by the publisher.
Research integrity at Frontiers
Learn more about the work of our research integrity team to safeguard the quality of each article we publish.