- 1Department of Clinical Sciences, College of Veterinary Medicine, Cornell University, Ithaca, NY, United States
- 2Department of Biomedical Sciences and New York Animal Health Diagnostic Center, College of Veterinary Medicine, Cornell University, Ithaca, NY, United States
- 3Department of Molecular Medicine, College of Veterinary Medicine, Cornell University, Ithaca, NY, United States
Differentiating canine acanthomatous ameloblastoma (CAA) from oral squamous cell carcinoma (OSCC) based on routine histopathology can be challenging. We have previously shown that more than 95% of CAAs harbor an HRAS p.Q61R somatic mutation, while OSCCs carry either wild-type alleles or other MAPK pathway activating mutations (e.g., HRAS p.Q61L, BRAF p.V595E). Given that HRAS p.Q61R mutations are highly prevalent in CAA, we hypothesized that a RAS Q61R-specific rabbit monoclonal antibody may be a useful tool for confirmation of CAA by immunohistochemical (IHC) staining. In the present study, we assessed IHC staining of archived formalin-fixed and paraffin-embedded biopsy samples with a diagnosis of CAA (n = 23), using a RAS Q61R-specific rabbit monoclonal antibody (SP174) and an automated IHC stainer. Negative control samples consisted of HRAS p.Q61R mutation-negative OSCC tumors with either a known HRAS p.Q61L mutation (n = 1), BRAF p.V595E mutation (n = 4), or wild-type corresponding alleles (n = 3). We found that all 23 CAAs showed diffuse and strong membranous RAS Q61R immunoreactivity (100% sensitivity), while none of the 8 OSCCs showed immunoreactivity (100% specificity). The data supports the use of RAS Q61R-specific rabbit monoclonal antibody for diagnostic IHC confirmation of CAA and ruling out OSCC in dogs.
1. Introduction
Canine acanthomatous ameloblastoma (CAA) is a common neoplasm that originates from odontogenic epithelium (1–4). It has been reported in multiple breeds of dogs of a wide age range (mean ~ 9 years) without an apparent sex predilection (5, 6). The tumor is characterized by a discrete space-occupying mass arising from a dentated area, with a variable degree of invasion of underlying anatomical structures, including the jawbone (7, 8). The current treatment of choice for CAA is wide-margin excision (i.e., mandibulectomy or maxillectomy), which typically offers long-term remission but is technically complex and frequently results in occlusal dysfunction (9–12). Other treatment modalities have been described, including marginal excision, radiation therapy and intralesional bleomycin injections, but long-term remission rates are either low or variable, and side effects can be considerable (13–15).
Definitive diagnosis of CAA is based on routine histopathological examination (3, 16). However, due to overlapping clinical, radiological, and histological features between CAA and oral squamous cell carcinoma (OSCC), up to ~30% of cases may be misdiagnosed (6, 17). This has important clinical implications given fundamental differences in biological behavior including significantly more rapid growth as well as higher metastatic potential of OSCC relative to CAA (8, 18). Although some of the molecular underpinnings driving OSCC and CAA tumorigenesis appear to be similar (e.g., aberrant MAPK pathway signaling) (19), we have shown in previous studies that their mutational landscape is distinct (20). Importantly, we have found that more than 95% of CAAs harbor an HRAS p.Q61R somatic mutation, while OSCCs typically harbor corresponding wild-type (WT) alleles or other MAPK pathway activating mutations (e.g., BRAF p.V595E, HRAS p.Q61L) (20, 21). Arguably, identifying the mutational profile of tumor tissues is critical to distinguishing between these different epithelial cell tumors. However, genotyping experiments usually require molecular biology and/or sequencing capacity that are not readily available in standard diagnostic settings. Moreover, accurate diagnosis of CAA and differentiation from OSCC is critical to making informed clinical decisions and adequate prognostication early in the course of the disease.
Immunohistochemistry (IHC) is an alternative technique that does not require a highly specialized infrastructure and can be used to produce a signal that corresponds to a mutated protein, providing a means to infer point mutations (22, 23). For this, monoclonal antibodies offering high sensitivity and specificity are required. One commonly used antibody, which we hypothesized might suit this purpose, is designated SP174. This recombinant rabbit monoclonal antibody was originally designed to detect human RAS Q61R, and is commercially-available (24). Of note, SP174 cross-reacts with different isoforms of RAS, making it useful for screening for p.Q61R mutations in NRAS, KRAS, and HRAS (22–26). Given that canine and human RAS isoforms share 98–100% sequence homology and based on the high frequency of HRAS p.Q61R somatic mutations in CAA (20, 21, 27), we hypothesized that SP174 could detect RAS Q61R in CAA and rule out OSCC. Therefore, the aim of this study was to determine the sensitivity and specificity of IHC using SP174 to detect RAS Q61R in CAA tumor tissue and a lack of immunoreactivity with OSCC, including tumors that harbor a closely related HRAS p.Q61L mutation.
2. Methods
Representative formalin-fixed and paraffin-embedded (FFPE) tissue sections from archived CAA and OSCC cases were assessed by IHC staining for the presence of RAS Q61R immunoreactivity using standard protocols and an automated IHC stainer (Bond-Max automated IHC staining system; Leica). All samples were submitted as biopsy specimens, obtained between 2013 and 2021, that did not undergo decalcification to preserve critical antigenic epitopes. The diagnosis of CAA was made based on initial hematoxylin and eosin assessment by a board-certified veterinary pathologist. The presence of an HRAS p.Q61R mutation was confirmed in the majority of CAAs using molecular assays, as previously described (8, 20, 21, 28). Briefly, 4-μm tissue sections mounted on charged slides were deparaffinized (AR9222, Bond dewax solution; Leica), and after heat epitope retrieval (AR9640, Bond epitope retrieval solution 2; Leica) for 30–40 min, the slides were incubated with RAS Q61R-specific rabbit monoclonal antibody (SP174; ab227658; Abcam, Cambridge, MA, United States) raised against Ras mutated Q61R synthetic peptide (25) diluted 1:80 or 1:100 for 60 min followed by polymeric horseradish peroxidase (DS9800; Bond polymer refine detection; Leica) linker antibody conjugate detection system for 30 min, and hematoxylin counterstain (DS9390; Leica) for 5 min. Negative controls consisted of confirmed HRAS p.Q61R-negative OSCC FFPE tissues (20, 21). Positive immunoreactivity was identified as brown staining with diaminobenzidine (DAB). The IHC evaluation was performed independently by two pathologists (M.M.M. and G.E.D), while blinded to the results of previous molecular analyses and medical records.
The use of archived diagnostic material, and/or review and data collection from medical records of client-owned animals for the purposes of this study was approved by Cornell University’s Veterinary Clinical Studies Committee and was considered exempt from review by Cornell University’s Institutional Animal Care and Use Committee.
3. Results
A total of 31 cases were enrolled representing 23 CAA and 8 OSCC tumors (Table 1; Figure 1). Of the 8 OSCC tumors, 5 corresponded to the papillary subtype (29). The average age was 8.85 ± 3.26 and 8.25 ± 3.11 for CAA and OSCC, respectively (p = 0.74, Mann–Whitney test). Sex distribution was 52% males and 48% with no significant differences based on tumor type (p = 0.92, Pearson’s chi-squared test). Seventeen dog breeds were represented with Labrador retriever and mixed breed dogs being the two most common, accounting for 22.6 and 12.9%, respectively. Of the 31 cases, 17 CAA and all 8 OSCC had been previously genotyped using RNA-seq, PCR and Sanger sequencing and/or restriction fragment length polymorphism experiments (20, 21). An HRAS p.Q61R somatic mutation was confirmed in all 17 genotyped CAA tumors. Only wild-type HRAS p.Q61 alleles were detected in 7 of the 8 OSCC tumors, and an HRAS p.Q61L mutation was detected in the remaining OSCC tumor. Four of the 8 OSCC tumors harbored a BRAF p.V595E mutation.
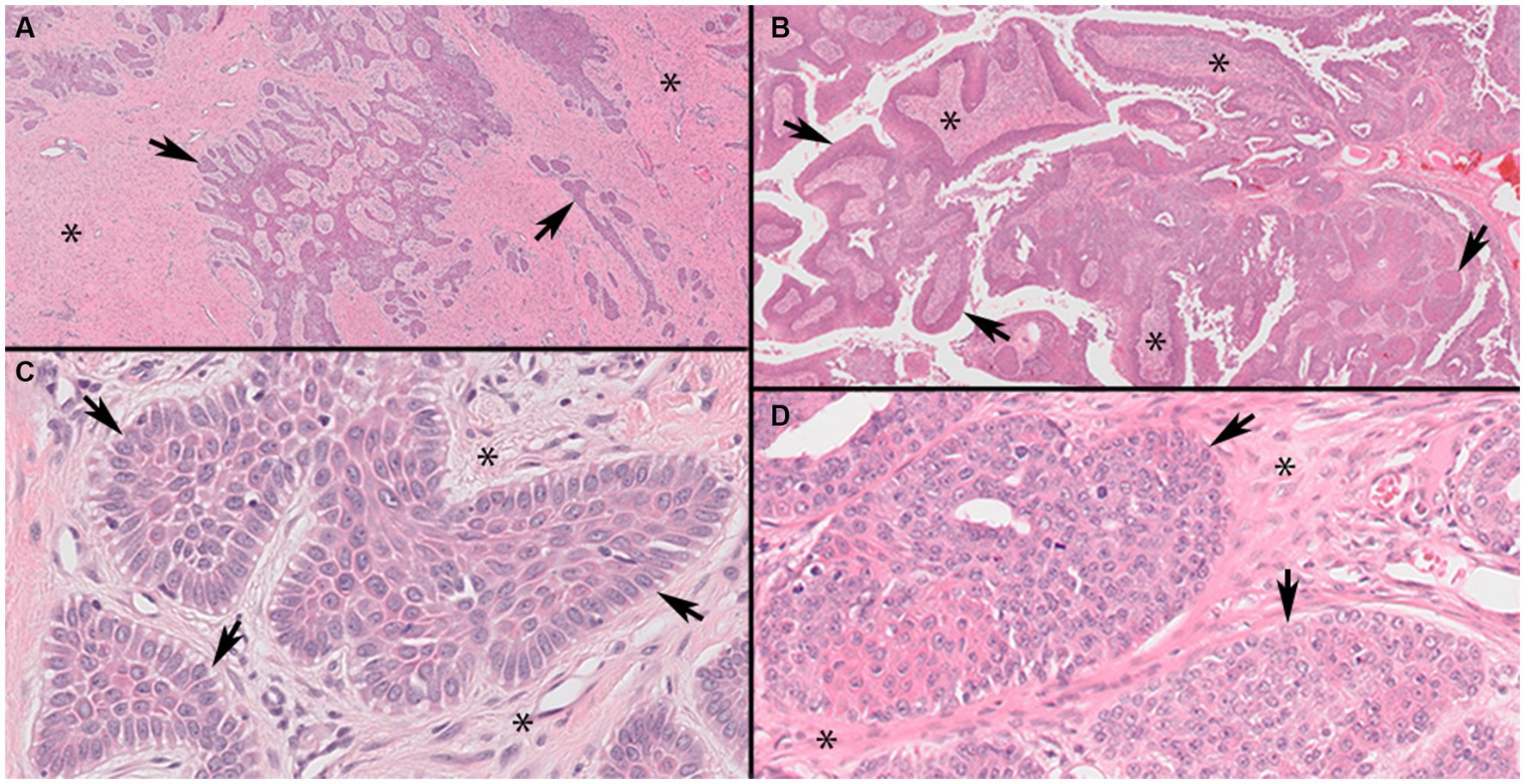
Figure 1. Representative photomicrographs of canine acanthomatous ameloblastoma (CAA) case 9 and oral squamous cell carcinoma, papillary subtype (pOSCC), case 27 (Panels A,C, ×4; B,D, ×40 original magnifications). Low magnification of CAA (Panel A) showing characteristic anastomosing cords and trabeculae of odontogenic epithelium (arrows) within a background of stellate mesenchyme (asterisks). Higher magnification of a CAA (Panel C) showing neoplastic islands with characteristic outer layer of epithelial cells oriented with their long axes perpendicular to the basement membrane (palisading) and anti-basilar nuclear polarization (arrows). Low magnification of a pOSCC (Panel B) showing characteristic exophytic papillary pattern consisting of broad trabeculae of neoplastic stratified squamous epithelium (arrows) supported by fibrovascular connective tissue stroma (asterisks). Higher magnification of a pOSCC (Panel D) showing irregularly arranged epithelial cells that lack anti-basilar nuclear polarization and indistinct epithelial to mesenchymal junction at the periphery of individual neoplastic epithelial lobules (arrows).
All 23 CAAs showed diffuse and strong membranous RAS Q61R immunoreactivity of all neoplastic epithelial cells (100% sensitivity, Figure 2) consistent with the known membranous localization of RAS (https://www.proteinatlas.org/ENSG00000213281-NRAS) (30), while none of the 8 OSCCs showed immunoreactivity (100% specificity). Critical IHC parameters for optimal immunoreactivity included: (i) heat epitope retrieval for 30–40 min; less than 30 min showed positive but faint staining, (ii) 1:80 or 1:100 primary antibody dilution for 60 min, and (iii) Bound Polymer Refine Detection for 30 min; less than 30 min showed positive but faint staining. Repeated RAS Q61R IHC staining in three separate runs with serial sections taken from a subset of four CAA tissue samples yielded similar results (100% reproducibility). Normal gingival epithelium in a subset of CAA tissue samples (n = 9) showed no immunoreactivity, confirming RAS Q61R mutation is a reliable feature of CAA (Figure 3). The presence of strong immunoreactivity in a tissue sample taken from a 7-month-old dog with CAA indicates that like adult CAA, HRAS p.Q61R mutations likely underlie CAA in juvenile dogs. Unfortunately, a tissue sample from this juvenile dog was not available for genotyping.
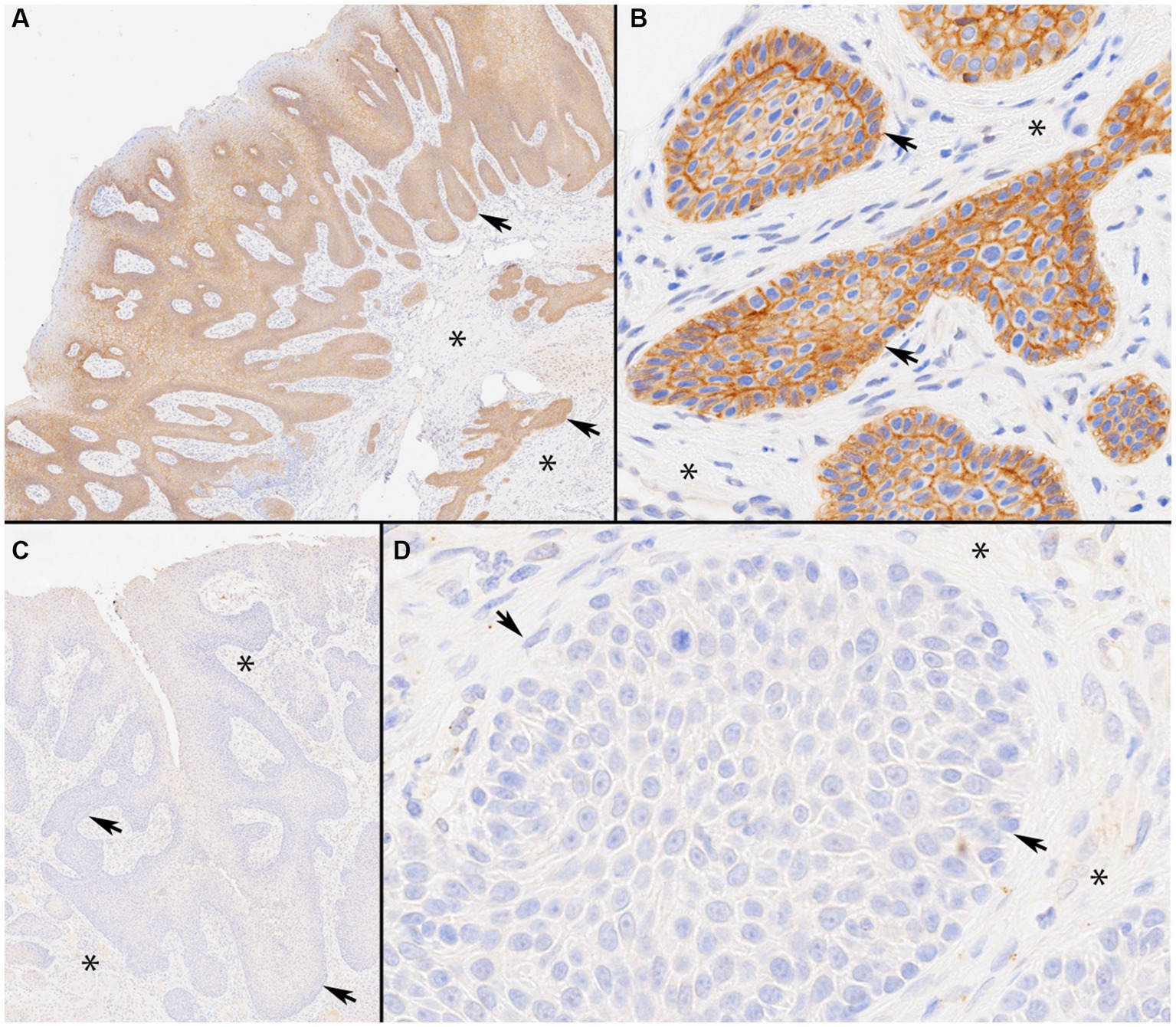
Figure 2. Immunolabeling of canine acanthomatous ameloblastoma (CAA) with RAS Q61R-specific rabbit monoclonal antibody (SP174). Photomicrographs of representative CAA case 9 with a confirmed HRAS p.Q61R mutation. Note strong membranous RAS immunoreactivity throughout CAA neoplastic trabeculae and islands (Panels A, ×4 and B, ×40 original magnifications). The staining highlights anti-basilar nuclear polarization in CAA (Panel B). By contrast, a representative oral squamous cell carcinoma (OSCC), papillary subtype, case 27 with a confirmed HRAS p.Q61L mutation, shows a lack of RAS immunoreactivity throughout (Panels C, ×4 and D, ×40 original magnifications). In each panel, asterisks represent stroma and arrows indicate neoplastic epithelial cells arranged in anastomosing trabeculae and lobules.
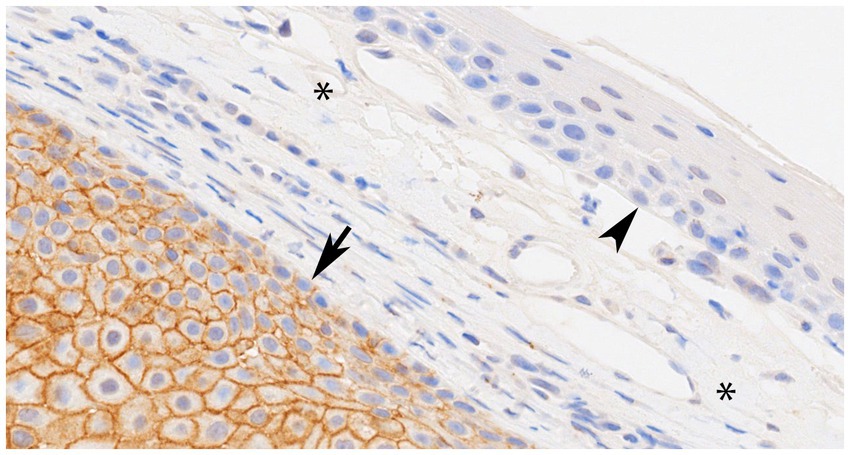
Figure 3. Representative photomicrograph of canine acanthomatous ameloblastoma (CAA), case 11 with confirmed HRAS p.Q61R mutation showing normal gingival epithelium (arrowhead) and connective tissue stroma (asterisks) without RAS immunoreactivity (internal negative control), while an underlying island of CAA neoplastic epithelial cells (arrow) shows strong membranous RAS immunoreactivity throughout (×40 original magnification).
4. Discussion
In this study, we assessed the utility of RAS Q61R-specific SP174 antibody for confirmation of CAA and distinction from OSCC. Given fundamental biological differences (6, 8, 18), accurate distinction between CAA and OSCC is essential for proper clinical decision making and prognostication. Results showed that SP174 cross-reacts with RAS Q61R in CAA FFPE tissues with 100% sensitivity and 100% specificity, allowing accurate distinction of these relatively common tumor types.
Other IHC strategies have been previously proposed to differentiate between CAA and OSCC (31, 32). For example, distinction based on cytokeratin and calretinin protein expression patterns has been suggested (32), but assessment relies purely on semi-quantitative scores and the diagnostic accuracy of these approaches has yet to be independently validated. Moreover, unbiased genomic profiling of CAA and OSCC shows variable expression patterns of the genes encoding these proteins without an obvious association with tumor type (19), suggesting that the patterns of cytokeratin and calretinin immunoreactivity in tumor tissues may not allow reliable tumor type distinction.
Another IHC-based strategy consists of determining the Ki67 labeling index of neoplastic epithelial cells (8, 33, 34). Ki67 is a nuclear protein expressed by cells that are actively engaged in the cell cycle (33). We have previously shown that the Ki67 labelling index of neoplastic cells is significantly lower in CAA compared to OSCC (8), which is a useful diagnostic complement to routine histopathological assessment. However, there is no established cutoff value that can reliably distinguish tumors with intermediate Ki67 labelling indices. In contrast, the strong and diffuse positive SP174 immunoreactivity of CAAs reported here offers a simple, easily interpreted binary distinction in which reactivity is either positive or negative, thus minimizing subjectivity, which is an inherent limitation with semi-quantitative scoring schemes commonly used for other IHC applications. Additionally, as shown in some of the cases in this report, CAA sections that contain non-neoplastic, normal surface gingival epithelium serve as an internal negative control minimizing interpretative bias.
Apart from CAA confirmation, the RAS Q61R-specific SP174 antibody may be used in the context of precision medicine, as proposed for human medical oncology (23–26). Arguably, rapid detection of mutated genes using IHC allows attending clinicians to rationally select targeted therapies for neoplasms known to harbor HRAS, NRAS or KRAS p.Q61R mutations including ameloblastoma, melanoma, colorectal, urothelial and thyroid cancers, among others (35). A highly specific and comparable diagnostic approach is available using the monoclonal antibody ‘VE1’ for detection of BRAF p.V600E in human cancer (23, 36, 37). Given that homologous encoding somatic mutations (i.e., BRAF p.V595E) are highly recurrent in some tumors of dogs, including papillary OSCC and urothelial carcinoma (20, 27, 38), VE1 IHC might be a useful tool that would complement RAS Q61R-specific SP174 antibody IHC. However, the use of VE1 for detection of mutated BRAF protein in dogs has yet to be validated. Indeed, our attempts to optimize VE1 IHC protocols using OSCC tumors confirmed to harbor a BRAF p.V595E mutation have been unsuccessful despite a high degree of sequence homology (data not shown). Although a cause for the lack of VE1 antibody reactivity with the corresponding mutated protein in canine oral tumors is uncertain, we speculate that level expression of the mutated BRAF allele may be below the detection limit of IHC, which could account for the lack of reactivity.
As reported herein, reliable detection of RAS Q61R with SP174 antibody in tumor tissues is possible using FFPE tissues that have not been decalcified together with optimization of IHC parameters, which may vary between various protocols, reagents, and laboratory equipment. Ideally, optimization of IHC protocols should be done using CAA tumors with a confirmed HRAS p.Q61R mutation as positive control, and normal oral or OSCC tumor tissues not harboring the mutation as negative controls. Additionally, because only FFPE tissues that were not decalcified were used in our study, the sensitivity and specificity of IHC staining with the RAS Q61R-specific SP174 antibody with tissues that may have been decalcified for various durations is unknown. Finally, even though recurrent HRAS p.Q61R mutations in oral tissues of dogs have only been demonstrated in CAA, they are not necessarily exclusive to this oral tumor type and thus additional comparative studies are required to determine the extent to which SP174 allows distinction between CAA and other proliferative lesions and normal oral epithelium.
In conclusion, this study demonstrates that IHC with RAS Q61R-specific SP174 antibody reagent is a highly sensitive, relatively simple, and specific method for confirmation of CAAs harboring an HRAS p.Q61R mutation.
Data availability statement
The raw data supporting the conclusions of this article will be made available by the authors, without undue reservation.
Ethics statement
The project referenced above has been approved by the Cornell University Veterinary Clinical Studies Committee (CUVCSC) following an ethical and scientific review. The project was exempted from review by the Cornell University Institutional Animal Care and Use Committee (IACUC) office because approval by the IACUC office was unnecessary for use of archival tissues. Owner consent for research use of such samples is signed by every client who consents to any treatment at the Cornell University Hospital for Animals.
Author contributions
SP: Conceptualization, Data curation, Formal analysis, Funding acquisition, Investigation, Methodology, Project administration, Supervision, Visualization, Writing – original draft. MM: Investigation, Writing – review & editing. WK: Conceptualization, Funding acquisition, Resources, Writing – review & editing. GD: Conceptualization, Investigation, Methodology, Supervision, Validation, Writing – review & editing.
Funding
The author(s) declare financial support was received for the research, authorship, and/or publication of this article. This study was performed using funds kindly provided by the Cornell Richard P. Riney Canine Health Center.
Acknowledgments
The authors thank the staff of the Histology Laboratory at the Cornell Animal Health Diagnostic Center for retrieving archived tissue blocks and excellent technical assistance with processing, sectioning, and scanning routine hematoxylin and eosin and state-of-the-art immunohistochemical staining of tissue sections.
Conflict of interest
The authors declare that the research was conducted in the absence of any commercial or financial relationships that could be construed as a potential conflict of interest.
The author(s) declared that they were an editorial board member of Frontiers, at the time of submission. This had no impact on the peer review process and the final decision.
Publisher’s note
All claims expressed in this article are solely those of the authors and do not necessarily represent those of their affiliated organizations, or those of the publisher, the editors and the reviewers. Any product that may be evaluated in this article, or claim that may be made by its manufacturer, is not guaranteed or endorsed by the publisher.
References
1. Bostock, DE, and White, RA. Classification and behaviour after surgery of canine 'epulides'. J Comp Pathol. (1987) 97:197–206. doi: 10.1016/0021-9975(87)90040-5
2. Gardner, DG. Canine acanthomatous epulis. The only common spontaneous ameloblastoma in animals. Oral Surg Oral Med Oral Pathol Oral Radiol Endod. (1995) 79:612–5. doi: 10.1016/S1079-2104(05)80104-0
3. Verstraete, FJ, Ligthelm, AJ, and Weber, A. The histological nature of epulides in dogs. J Comp Pathol. (1992) 106:169–82. doi: 10.1016/0021-9975(92)90046-W
4. Yoshida, K, Yanai, T, Iwasaki, T, Sakai, H, Ohta, J, Kati, S, et al. Clinicopathological study of canine oral epulides. J Vet Med Sci. (1999) 61:897–902. doi: 10.1292/jvms.61.897
5. Fiani, N, Verstraete, FJ, Kass, PH, and Cox, DP. Clinicopathologic characterization of odontogenic tumors and focal fibrous hyperplasia in dogs: 152 cases (1995-2005). J Am Vet Med Assoc. (2011) 238:495–500. doi: 10.2460/javma.238.4.495
6. Goldschmidt, SL, Bell, CM, Hetzel, S, and Soukup, J. Clinical characterization of canine acanthomatous ameloblastoma (CAA) in 263 dogs and the influence of postsurgical histopathological margin on local recurrence. J Vet Dent. (2017) 34:241–7. doi: 10.1177/0898756417734312
7. Amory, JT, Reetz, JA, Sánchez, MD, Bradley, CW, Lewis, JR, Reiter, AM, et al. Computed tomographic characteristics of odontogenic neoplasms in dogs. Vet. Radiol. Ultrasound. (2014) 55:147–58. doi: 10.1111/vru.12101
8. Peralta, S, Grenier, JK, McCleary-Wheeler, AL, and Duhamel, GE. Ki67 labelling index of neoplastic epithelial cells differentiates canine acanthomatous ameloblastoma from oral squamous cell carcinoma. J Comp Pathol. (2019) 171:59–69. doi: 10.1016/j.jcpa.2019.08.001
9. Verstraete, FJ. Mandibulectomy and maxillectomy. Vet Clin North Am Small Anim Pract. (2005) 35:1009–1039, viii. doi: 10.1016/j.cvsm.2005.03.005
10. Warshaw, SL, Carney, PC, Peralta, S, and Fiani, N. Piezosurgical bone-cutting technology reduces risk of maxillectomy and mandibulectomy complications in dogs. J Am Vet Med Assoc. (2023) 261:1–7. doi: 10.2460/javma.23.03.0130
11. Bar-Am, Y, and Verstraete, FJ. Elastic training for the prevention of mandibular drift following mandibulectomy in dogs: 18 cases (2005-2008). Vet Surg. (2010) 39:574–80. doi: 10.1111/j.1532-950X.2010.00703.x
12. Fiani, N, and Peralta, S. Extended subtotal Mandibulectomy for the treatment of oral tumors invading the Mandibular Canal in dogs—A novel surgical technique. Front Vet Sci. (2019) 6:339. doi: 10.3389/fvets.2019.00339
13. Yoshida, K, Watarai, Y, Sakai, Y, Yanai, T, Masegi, T, and Iwasaki, T. The effect of intralesional bleomycin on canine acanthomatous epulis. J Am Anim Hosp Assoc. (1998) 34:457–61. doi: 10.5326/15473317-34-6-457
14. McEntee, MC, Page, RL, Theon, A, Erb, HN, and Thrall, DE. Malignant tumor formation in dogs previously irradiated for acanthomatous epulis. Vet Radiol Ultrasound. (2004) 45:357–61. doi: 10.1111/j.1740-8261.2004.04067.x
15. Theon, AP, Rodriguez, C, Griffey, S, and Madewell, BR. Analysis of prognostic factors and patterns of failure in dogs with periodontal tumors treated with megavoltage irradiation. J Am Vet Med Assoc. (1997) 210:785–8.
16. Murphy, BG, Bell, CM, and Soukup, JW. Veterinary Oral and Maxillofacial Pathology. (2019) Wiley Online Library.
17. Soukup, JW, Snyder, CJ, Simmons, BT, Pinkerton, ME, and Chun, R. Clinical, histologic, and computed tomographic features of oral papillary squamous cell carcinoma in dogs: 9 cases (2008-2011). J Vet Dent. (2013) 30:18–24. doi: 10.1177/089875641303000102
18. Fulton, AJ, Nemec, A, Murphy, BG, Kass, PH, and Verstraete, FJ. Risk factors associated with survival in dogs with nontonsillar oral squamous cell carcinoma 31 cases (1990-2010). J Am Vet Med Assoc. (2013) 243:696–702. doi: 10.2460/javma.243.5.696
19. Peralta, S, Duhamel, GE, Katt, WP, Heikinheimo, K, Miller, AD, Ahmed, F, et al. Comparative transcriptional profiling of canine acanthomatous ameloblastoma and homology with human ameloblastoma. Sci Rep. (2021) 11:17792. doi: 10.1038/s41598-021-97430-0
20. Peralta, S, Webb, SM, Katt, WP, Grenier, JK, and Duhamel, GE. Highly recurrent BRAF p.V595E mutation in canine papillary oral squamous cell carcinoma. Vet Comp Oncol. (2023) 21:138–44. doi: 10.1111/vco.12869
21. Peralta, S, McCleary-Wheeler, AL, Duhamel, GE, Heikinheimo, K, Grenier, JK, and Ultra-Frequent, HRAS. Q61R somatic mutation in canine acanthomatous ameloblastoma reveals pathogenic similarities with human ameloblastoma. Vet Comp Oncol. (2019) 17:439–45. doi: 10.1111/vco.12487
22. Uguen, A, Gueguen, P, Guibourg, B, Duigou, S, Pochic, M, Conq, G, et al. Is SP174 immunohistochemistry an interesting ancillary tool to determine RAS mutational status in colorectal carcinoma? Appl Immunohistochem Mol Morphol. (2017) 25:e25–6. doi: 10.1097/PAI.0000000000000375
23. Uguen, A, Talagas, M, Costa, S, Samaison, L, Paule, L, Alavi, Z, et al. NRASQ61R, BRAFV600Eimmunohistochemistry: a concomitant tool for mutation screening in melanomas. Diagn Pathol. (2015) 10:121. doi: 10.1186/s13000-015-0359-0
24. Felisiak-Goląbek, A, Inaguma, S, Kowalik, A, Wasąg, B, Wang, ZF, Zięba, S, et al. SP174 antibody lacks specificity for NRAS Q61R and cross-reacts with HRAS and KRAS Q61R mutant proteins in malignant melanoma. Appl Immunohistochem Mol Morphol. (2018) 26:40–5. doi: 10.1097/pai.0000000000000500
25. Pareja, F, Toss, MS, Geyer, FC, da Silva, EM, Vahdatinia, M, Sebastiao, APM, et al. Immunohistochemical assessment of HRAS Q61R mutations in breast adenomyoepitheliomas. Histopathology. (2020) 76:865–74. doi: 10.1111/his.14057
26. Uguen, A. The usefulness of SP174 anti-RASQ61R immunohistochemistry in melanoma, thyroid, and colorectal cancers. Arch Pathol Lab Med. (2017) 141:1601–1. doi: 10.5858/arpa.2017-0187-LE
27. Mochizuki, H, and Breen, M. Sequence analysis of RAS and RAF mutation hot spots in canine carcinoma. Vet Comp Oncol. (2017) 15:1598–605. doi: 10.1111/vco.12275
28. Head, KW, Cullen, JM, Dubielzig, RR, Else, RW, Misdorp, W, Patnaik, AK, et al. Histological classification of tumors of odontogenic origin of domestic animals In: K Head, editor. World Health Organization International Histological Classification of Tumors of Domestic Animals, Second Series, vol. 10. Washington, DC: Armed Forces Institute of Pathology (2003). 46–57.
29. Nemec, A, Murphy, B, Kass, PH, and Verstraete, FJ. Histological subtypes of oral non-tonsillar squamous cell carcinoma in dogs. J Comp Pathol. (2012) 147:111–20. doi: 10.1016/j.jcpa.2011.11.198
30. Pontén, F, Jirström, K, and Uhlen, M. The human protein atlas—a tool for pathology. J Pathol. (2008) 216:387–93. doi: 10.1002/path.2440
31. Thaiwong, T, Sledge, DG, Collins-Webb, A, and Kiupel, M. Immunohistochemical characterization of canine oral papillary squamous cell carcinoma. Vet Pathol. (2018) 55:224–32. doi: 10.1177/0300985817741732
32. Fulton, A, Arzi, B, Murphy, B, Naydan, DK, and Verstraete, FJ. The expression of calretinin and cytokeratins in canine acanthomatous ameloblastoma and oral squamous cell carcinoma. Vet Comp Oncol. (2014) 12:258–65. doi: 10.1111/j.1476-5829.2012.00356.x
33. Goodlad, RA. Quantification of epithelial cell proliferation, cell dynamics, and cell kinetics in vivo. Wiley Interdiscip Rev Dev Biol. (2017) 6. doi: 10.1002/wdev.274
34. Yu, CC, Woods, AL, and Levison, DA. The assessment of cellular proliferation by immunohistochemistry: a review of currently available methods and their applications. Histochem J. (1992) 24:121–31. doi: 10.1007/BF01047461
35. Li, S, Balmain, A, and Counter, CM. A model for RAS mutation patterns in cancers: finding the sweet spot. Nat Rev Cancer. (2018) 18:767–77. doi: 10.1038/s41568-018-0076-6
36. Dvorak, K, Aggeler, B, Palting, J, McKelvie, P, Ruszkiewicz, A, and Waring, P. Immunohistochemistry with the anti-BRAF V600E (VE1) antibody: impact of pre-analytical conditions and concordance with DNA sequencing in colorectal and papillary thyroid carcinoma. Pathology. (2014) 46:509–17. doi: 10.1097/pat.0000000000000119
37. Dvorak, K, Higgins, A, Palting, J, Cohen, M, and Brunhoeber, P. Immunohistochemistry with anti-BRAF V600E (VE1) mouse monoclonal antibody is a sensitive method for detection of the BRAF V600E mutation in Colon Cancer: evaluation of 120 cases with and without KRAS mutation and literature review. Pathol Oncol Res. (2019) 25:349–59. doi: 10.1007/s12253-017-0344-x
Keywords: canine acanthomatous ameloblastoma, oral tumor, immunohistochemistry, HRAS, somatic mutation, SP174
Citation: Peralta S, Marcinczyk MM, Katt WP and Duhamel GE (2023) Confirmation of canine acanthomatous ameloblastoma using RAS Q61R immunohistochemical staining of formalin-fixed paraffin-embedded tissues. Front. Vet. Sci. 10:1281022. doi: 10.3389/fvets.2023.1281022
Edited by:
Stephanie Lynne Goldschmidt, University of California, Davis, United StatesReviewed by:
Cynthia Bell, Specialty Oral Pathology for Animals, LLC, United StatesSami Al-Nadaf, University of California, Davis, United States
Alana Pavuk, Antech Diagnostics, United States
Copyright © 2023 Peralta, Marcinczyk, Katt and Duhamel. This is an open-access article distributed under the terms of the Creative Commons Attribution License (CC BY). The use, distribution or reproduction in other forums is permitted, provided the original author(s) and the copyright owner(s) are credited and that the original publication in this journal is cited, in accordance with accepted academic practice. No use, distribution or reproduction is permitted which does not comply with these terms.
*Correspondence: Santiago Peralta, c3A4ODhAY29ybmVsbC5lZHU=