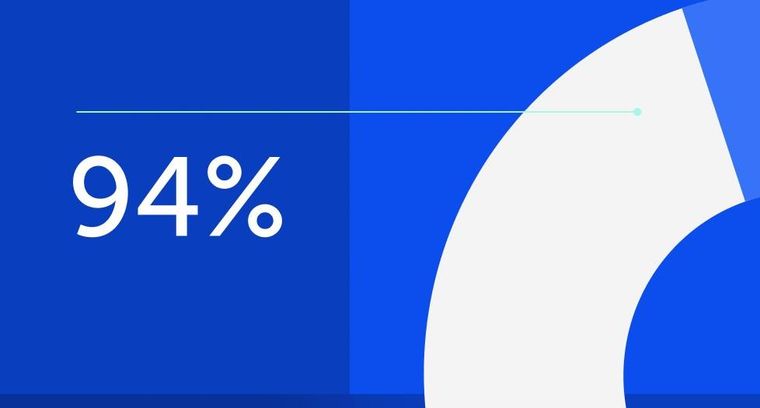
94% of researchers rate our articles as excellent or good
Learn more about the work of our research integrity team to safeguard the quality of each article we publish.
Find out more
ORIGINAL RESEARCH article
Front. Vet. Sci., 10 October 2023
Sec. Veterinary Infectious Diseases
Volume 10 - 2023 | https://doi.org/10.3389/fvets.2023.1276754
This article is part of the Research TopicPredicting, Managing, and Minimizing Mycotoxicosis in Farm AnimalsView all 5 articles
A correction has been applied to this article in:
Corrigendum: Evaluation of the efficacy of humic acids to counteract the toxic effects of aflatoxin B1 in turkey poults
This study aims to evaluate the efficacy of humic acid (HA) from worm compost as an adsorbent for aflatoxin B1 (AFB1) in turkey poults. The experiment involved the inclusion of 0.25% (w/w) HA in the diet of turkey poults consuming aflatoxin-contaminated feed (250 ng AFB1/g). A total of 350 1-day-old female Nicholas-700 turkey poults were randomly allocated to five equal groups: negative control (basal diet); positive control (basal diet + 250 ng AFB1/g; HA (basal diet + 0.25% HA); HA + AFB1 (basal diet + HA + 250 ng AFB1/g); and zeolite + AFB1 (basal diet + 0.25% zeolite + 250 ng AFB1/g). Each group had seven replicates of 10 poults (n = 70). The impact of HA addition was evaluated in terms of performance parameters, relative organ weights, liver histological lesions, and serum biochemical and hematological constituents. In general, the addition of HA improved body weight (BW), body weight gain (BWG), and feed conversion rate (FCR). Furthermore, HA effectively mitigated the toxic effects caused by AFB1 in the majority of the analyzed variables. The results indicated that HA effectively counteracted the AFB1-induced toxic effects in turkey poults. Based on these findings, it can be concluded that HA is capable of removing AFB1 from the contaminated diet.
Aflatoxin B1 (AFB1) is a highly toxic mycotoxin, primarily produced by Aspergillus flavus and Aspergillus parasiticus, and it poses a significant concern in poultry farming, especially for turkey poults (1, 2). Thus, extensive research has been conducted to investigate the harmful effects of AFB1 in animals, including their carcinogenic, mutagenic, teratogenic, and growth-inhibitory properties (3, 4). Contamination with aflatoxins leads to reduced feed quality and animal performance due to poor nutrient conversion and various issues, such as reproductive abnormalities (5). Aflatoxicosis in poultry manifests as listlessness, decreased appetite, reduced growth rate, inefficient feed utilization, decreased egg production, and increased mortality. Additionally, aflatoxin contamination in feed can impair both humoral and cellular immune responses, rendering birds more susceptible to environmental and infectious agents (4).
Previous studies have extensively reported the presence of potential aflatoxin-producing strains and AFB1 molecules in commercial poultry feeds. As a result, there is an urgent need for practical and cost-effective methods to decontaminate aflatoxin-contaminated feed on a large scale (6). Several physical, chemical, and biological techniques have been employed to decontaminate agricultural commodities with good results, but their implementation has been limited by uncertainty about their in vivo effectiveness (7). One practical approach is the utilization of non-nutritive adsorbent materials, which can effectively bind mycotoxins and inhibit their absorption in the gastrointestinal tract (8), thereby reducing their toxic impact on poultry and minimizing the carryover of these fungal metabolites into poultry products such as meat-end eggs (9). However, not all adsorbents exhibit significant effectiveness, and some of them have been found to hinder nutrient utilization. An optimal adsorbent material should exhibit a high affinity for aflatoxins, facilitating the formation of stable complexes with minimal risk of dissociation. Furthermore, it should possess a substantial binding capacity to prevent saturation (10).
Humic substances (HS) are widely distributed and can be found in areas undergoing decomposition or sedimentation, such as soils (11). Within the HS, three distinct components are distinguished based on their solubility: fulvic acids (FA), humic acids (HA), and humins (12). These substances have demonstrated a strong ability to bind various compounds, including heavy metals, herbicides, mutagens, aromatic compounds, minerals, and bacteria (13). Recent studies have shown that incorporating HS into poultry diets promotes growth by enhancing energy digestibility and nutrient retention (14, 15). Although the specific mechanisms behind this growth promotion are not fully understood, the inclusion of HA in the chicken diet leads to elevated intestinal viscosity and improved intestinal integrity (16, 17). In a recent study, HA derived from vermicompost demonstrated remarkable efficacy in adsorbing AFB1 in an in vitro model that simulates the digestive tract of birds (18). Therefore, this study aimed to evaluate the effects of HA on performance parameters, relative organ weight, liver histological lesions, and serum biochemical and hematological analyses in AFB1-exposed turkey poults.
A total of 350 1-day-old female Nicholas-700 turkey poults (Aviagen Inc., AR, USA) were raised in pens for 28 days. Poults were collectively weighed (10 birds/pen) and randomly allocated to one of the five groups: negative control (basal diet); positive control (basal diet + 250 ng AFB1/g; HA (basal diet + 0.25% HA); HA + AFB1 (basal diet + HA + 250 ng AFB1/g); and zeolite + AFB1 (basal diet + 0.25% zeolite + 250 ng AFB1/g). Each group had seven replicates of 10 poults (n = 70). A maize-soybean-based turkey poult diet was formulated to approximate the nutritional requirements recommended by the National Research Council (17) and adjusted to the breeder's recommendations (Table 1). AFB1, HA, and zeolite were added to the diet and mixed thoroughly to the specified level. Poults had ad libitum access to water and feed during the experiment. All animal handling procedures complied with the Institutional Animal Care and Use Committee (IACUC) at the University of Arkansas, Fayetteville (protocol no. 22020).
AFB1 was provided by Dr. Xiangwei Du, Veterinary Medical Diagnostic Laboratory, University of Missouri, Columbia, MO, USA. AFB1 was produced through the fermentation of rice (19) using an Aspergillus parasiticus strain. The aflatoxin content was determined using high-performance liquid chromatography with a fluorescence detection method, utilizing a Romer derivatization unit (Romer Labs Inc., Washington, MO) (20). The composition consisted of 86.82% AFB1, 8.22% AFG1, 4.55% AFB2, and 0.37% AFG2. Diets were analyzed to verify the actual AFB1 concentration.
The method for extracting and isolating HA from worm compost was conducted following the protocol outlined by (21) with minor modifications. The extraction of HA involved stirring the compost in a sodium hydroxide solution (1M) at a ratio of 1:4 (compost: alkali) for 2 h. The resulting suspension was allowed to stand at room temperature for 24 h and subsequently filtered using a Whatman grade 40 filter paper. The filtrate was centrifuged at 3,500 × g for 15 min, and the supernatant was carefully decanted. To precipitate the HA, the supernatant containing HA was acidified using hydrochloric acid (10%, v/v) with continuous agitation until reaching pH 2. HA was separated from fulvic acid through centrifugation at 3,500 × g for 15 min. The resulting precipitate (HA) was adjusted to pH 10 with 1M NaOH and subsequently dried at 60°C. Finally, the dried powder was finely ground using a Thomas Willey grinder and sieved through a 0.25-mm mesh.
A non-commercial zeolitic material was employed as a reference. The main elements in the zeolite were Si (40.93%), C (31.99%), O (14.13%), and Al (7.46%), with smaller amounts of Na (0.25%), K (1.80%), Mg (1.12%), Ca (1.45%), and Fe (0.87%). Characterization details are fully described by Maguey-González et al. (18).
Each pen replicates served as an individual experimental unit. Poults were collectively weighed each week to obtain body weight (BW) and body weight gain (BWG). The feed intake (FI) and feed conversion ratio (FCR) were determined weekly.
All birds were euthanized on day 28 by CO2 inhalation. The intestine, liver, spleen, and bursa of Fabricius were removed and weighed (21 birds per treatment). The relative organ weight ratio was calculated as follows:
The liver (three birds from each replicate) was removed on day 28. Hepatocellular degeneration and lymphoid and heterophilic infiltration were evaluated. Liver sections were fixed in neutral buffered formalin (10%) until processed. A transversal section of the middle part of the left hepatic lobule was prepared routinely, dehydrated in increasing alcohol concentrations, and embedded in paraffin. A 5-μm thick tissue sample was cut, stained with hematoxylin and eosin, and mounted with coverslips for the histological analysis.
Hepatocellular degeneration was scored as follows: Score: 0 (normal or absence of cellular swelling), 1 (mild vacuolar degeneration/fat deposition), 2 (moderate vacuolar degeneration/fat deposition), and 3 (severe vacuolar degeneration/fat deposition). Additionally, congestion of vessels, bile duct hyperplasia, and fibrosis were scored. The score was obtained by evaluating five fields with a magnification of 20 × per section of tissue. The scores were considered per treatment.
Quantification of inflammatory cells (lymphocytes) was obtained using an adapted methodology previously described (22). In a general field from the upper left end of the tissue cut, with a magnification of 53, an area of 3.4 mm2 was evaluated. The lesion score was assigned by counting the number of cell layers from the center of the cell cluster or the space of the perivascular area toward the perimeter of the cluster where the greatest number of cell layers were present. The lesion score was as follows: Score: 0 normal/minimal inflammatory infiltrate (1–11 cell layers/cluster), 1 mild inflammatory infiltrate (12–24 cell layers/cluster), 2 moderate inflammatory infiltrate (25–50 cell layers/cluster), and 3 severe inflammatory infiltrate (51–100 cell layers/cluster).
At the end of the experiment, 21 birds from each treatment were randomly selected, and blood was collected from their femoral veins. Blood was centrifuged at 1,118 × g at 4°C for 15 min. The serums were taken and preserved at −20°C until submitted for biochemical analysis. Serum concentrations of total protein, alanine aminotransferase (ALT), alkaline phosphatase (ALP), aspartate aminotransferase (AST), creatine kinase (CK), glutamate dehydrogenase (GLDH), creatinine, blood urea nitrogen (BUN), glucose, sodium, chloride, calcium, phosphorus, uric acid, and CO2 were determined using a Corning clinical chemistry analyzer (Chiron Corporation, San Jose, CA).
Fourteen birds from each treatment (2 birds/replicate) were randomly selected and bled from the femoral vein. Whole blood was collected in EDTA blood tubes and subjected to automated hematology analysis (Cell-Dyn; Abbott Diagnostics, Abbott Park, IL). Data acquired included the concentration of white cells, hematocrit (%), heterophils, lymphocytes, monocytes, basophils, and heterophils/lymphocytes ratio.
Data from performance, relative organ weights, serum biochemicals, and hematological analysis were subjected to ANOVA as a complete randomized design, using the general linear model procedure of SAS (23). The Duncan multiple range tests at P < 0.05 determined significant differences among means.
Data from lesion scores of liver histopathological analysis are expressed as median (mode; variance), and differences among median values of the groups were analyzed using the Mann-Whitney U-test with a level of significance set at P < 0.05.
Table 2 summarizes differences in BW, BWG, FI, and FCR in turkeys consuming a corn-soybean-based diet contaminated with AFB1 (250 ng AFB1/g) added with HA. The differences in BW started decreasing compared to the positive control group (221.92 g) and both HA treatments (249.49 and 241.09 g for HA and HA+AFB1, respectively) at day 14 (P < 0.0001). At day 21, the BW of both HA treatments (428.35 and 390.10 g for HA and HA+AFB1, respectively) was higher than the AFB1 treatments (348.78 g) (P < 0.0001). Similarly, at day 28, the BW of both HA treatments (637.47 and 571.95 g for HA and HA+AFB1, respectively) was higher than the AFB1 treatments (428.14 g) (P < 0.0001). Differences in BWG were observed from the second week (7–14 days). The BWG of both HA treatments was higher than the AFB1 treatments (P < 0.0001). Furthermore, the BWG of HA treatment was 178.85 g in the third week (14–21 d) (P < 0.0001) and 209.12 g in the fourth week (21–28 days) (P < 0.0001), followed by HA+AFB1 treatment (149.01 and 181.85 g, respectively) compared to positive control treatment (126.86 and 85.14 g, respectively). When comparing from 0–28 days, the BWG of both HA treatments remained significantly higher (581.13 and 515.25 g for HA and HA+AFB1, respectively) compared to the positive control treatment (371.76 g) (P < 0.0001). Furthermore, during the last week (21–28 days), the FI was significantly higher in both HA treatments (288.00 and 266.80 g for HA and HA+AFB1, respectively) when compared to the positive control treatment (141.75 g) (P < 0.0001). With respect to the FI from 0–28 days, it was significantly higher in both HA treatments (764.04 and 706.76 g for HA and HA+AFB1, respectively) compared to the positive control treatment (141.75 g) (P < 0.0001). Moreover, the FCR was consistently higher in the positive control group than in all the other treatments throughout the entire duration of the experiment. Finally, the FCR from 0–28 days was significantly higher in the negative control (1.56), positive control (1.52), and ZEO+AFB1 (1.51) treatments compared to both HA treatments (1.31 and 1.37 for HA and HA+AFB1, respectively) (P < 0.0001), denoting a better FC in the treatments added with HA.
Table 2. Evaluation of body weight (BW), body weight gain (BWG), feed intake (FI), and feed conversion ratio (FCR) in turkeys consuming a corn-soybean-based diet contaminated with aflatoxin B1 (250ppb) supplemented with humic acids.
Table 3 shows the relative weight of the liver, spleen, bursa of Fabricius, and intestine in poults consuming diets contaminated with AFB1 and HA. The relative weight of the liver was lower (P < 0.0001) in the positive control group (3.72 g) compared to the negative control group (2.89 g) and the HA (3.35 g) poults, while it was similar to the zeolite+AFB1 group (2.98 g) and higher than the HA+AFB1 (2.56) poults. The AFB1 inclusion in the positive control (0.15 g) caused an increase in the relative weight of the spleen (P < 0.006) compared to the negative control (0.12), and it showed similar values compared to the rest of the treatments. The negative control (0.17 g) poults showed a similar relative weight of the bursa of Fabricius compared to the positive control (0.19 g) and the zeolite+AFB1 (0.20 g) treatments, while it was lower compared to the HA (0.22 g) and HA+AFB1 (0.22 g) poults. The relative weight of the intestine of the positive control (8.25 g) was similar to that of the negative control (8.66 g) and the zeolite+AFB1 (8.07 g) treatment, which in turn were higher (P < 0.0001) than that of the HA (6.96 g) and HA+AFB1 (6.42 g) treatments. No significant differences were observed in the bursa/spleen ratio among treatments.
Table 3. The relative weight of liver, spleen, bursa of Fabricius, and intestine in turkeys consuming a corn-soybean-based diet contaminated with 250 ppb of aflatoxin B1 during 28 days, supplemented with humic acids.
Table 4 presents the blood cell counts of poults consuming diets contaminated with AFB1 and HA. No significant differences were observed in the white cells, hematocrit, heterophils, lymphocytes, basophils, or heterophils/lymphocyte ratio. The monocyte counts in the negative control (1,500/μL), positive control (2,423/μL), and HA+AFB1 (2,208/μL) poults were similar, and the counts were higher (P < 0.0001) between the HA (6,127/μL) and zeolite+AFB1 (5,648/μL) treatments.
Table 4. Blood count cells in turkeys consuming a corn-soybean-based diet contaminated with 250ppb of aflatoxin B1 during 28 days, supplemented with humic acids.
Table 5 presents the serum biochemical parameters in poults consuming diets contaminated with AFB1 supplemented with HA. The inclusion of AFB1 the positive control induced a significant decrease in serum levels of total protein (P < 0.0001), AST (P < 0.001), GLDH (P < 0.02), creatinine (P < 0.0001), BUN (P < 0.0009), glucose (P < 0.002), Ca (P < 0.0001), and P (P < 0.0002) compared to the negative control treatment. In poults receiving the diet with added HA, there was a partial restoration of total protein, creatinine, and Ca compared to the positive control, and there was a lower concentration of ALT, ALP, AST, BUN, glucose, P, and uric acid, but higher Na, Cl, and CO2 concentrations compared to the negative control. All the biochemical parameters were similar between HA+AFB1 and the positive control poults, with the exception of CO2, which was higher in the former group. In zeolite+AFB1 poults, the total protein, ALT, creatinine, and BUN were higher, and Ca concentrations were lower than the positive control.
Table 5. Serum biochemical parameters in turkeys consuming a corn-soybean-based diet contaminated with 250ppb of aflatoxin B1 during 28 days, supplemented with humic acids.
Table 6 summarizes the hepatocellular degeneration responses in poults consuming diets contaminated with AFB1 and supplemented with HA. The positive control treatment, combined with AFB1, showed significant increases (P < 0.05) in cellular degeneration and fibrosis compared to the negative control. In HA poults, lower (P < 0.05) vessel congestion was found compared to the positive and negative control groups, and lower bile duct hyperplasia was observed compared to the positive control group. The findings were corroborated by histopathology studies, which showed that the liver of turkeys from the AFB1 group had substantial cellular degeneration and fat deposition (Figures 1A–E). In HA+AFB1 poults, the cell degeneration was higher compared to the negative control, while the rest of the variable responses were similar compared to the positive and negative controls and the HA poults.
Table 6. Hepatocellular degeneration in turkeys consuming a corn-soybean-based diet contaminated with 250ppb of aflatoxin B1 during 28 days, supplemented with humic acids.
Figure 1. Histological images were taken using a 20X objective on H & E stained tissue sections of the liver. (A) Negative control; (B) Positive control; (C) HA; (D) HA+AFB1; (E) zeolite+AFB1. Black arrows show vacuolar degeneration; Blue arrows show inflammation; Yellow arrows show bile duct hyperplasia; Red arrows show fibrosis.
Regarding the zeolite+AFB1-fed poults, the cell degeneration was higher than the negative control. Meanwhile, vacuolar degeneration, congestion vessels, lymphocytic infiltration, and bile duct hyperplasia were similar to positive, negative, and HA poults. Conversely, fibrosis exhibited the lowest occurrence compared to all the other treatments.
The exposure of turkeys to AFB1 negatively impacts productivity, causing significant economic losses and health problems in the poultry industry because of its toxicity (24). In an earlier study, a significant decrease in BWG and FCR was observed in poults fed diets with quantities equal to or higher than 400 ppb from 2 to 7 weeks of age (25). In poults fed naturally contaminated whole corn kernels with AFB1 from 1 to 21 days of age, reduced BWG was observed (26). Reduced FI and BW were also seen in poults from 1 to 21 days of age fed diets added with 100 ppb of aflatoxins and from 1 to 42 days of age when diets contained 200 ppb of aflatoxins (27). In poults fed 100 ppb aflatoxin, reduced BWG was observed, and at higher inclusions of aflatoxins, reduced FI and higher mortalities were found (28). Other recent publications have reported reduced BWG and increased FCR in poult-fed aflatoxin-contaminated feeds (29, 30). The reduced BW and BWG and increased FCR in poults fed the positive control diet in the present study agree with previous findings, which show that the growth performance of poults is negatively affected by the consumption of AFB1.
Conversely, poults fed with HA showed increased BW, BWG, and FI, and reduced FCR, which is consistent with previous findings on broiler chickens supplemented with HS derived from worm composts (15). It is worth noting that HA-fed poults outperformed the negative control ones in BW, BWG, FI, and FCR by 31.8, 36.2, 14.3, and 16.0%, respectively. Interestingly, HA+AFB1-fed poults also improved the BW, BWG, FI, and FCR by 18.3, 20.7, 5.7, and 12.2%, respectively, compared to those of the negative control group. These results indicate that HA-fed turkeys not only restored the productive parameters that were adversely affected by AFB1 in the positive control group but also improved the productivity of the negative control group. To the best of our knowledge, this is the first study where HA supplementation is reported to improve the productivity of unchallenged and AFB1-challenged poults.
HS has demonstrated a strong ability to bind various compounds, including heavy metals, herbicides, mutagens, aromatic compounds, minerals, and bacteria (13). In a recent study, HA derived from vermicompost was characterized and found to be highly effective in adsorbing AFB1 in an in vitro model simulating a chicken's digestive tract (18). Due to its highly hydrophobic surfaces and a wide range of negatively charged functional groups (31), interactions between HA and AFB1 can occur through various mechanisms. Tan (9) has identified seven potential binding mechanisms for HA with gaseous, liquid, and solid components, which include (i) physical forces, (ii) chemical forces, (iii) hydrogen bonds, (iv) hydrophobic interactions, (v) electrostatic interactions, (vi) coordination reactions, and (vii) ligand exchange. Among these mechanisms, electrostatic interactions and hydrogen bonding play crucial roles in the HA-AFB1 interaction.
Additionally, other interactions, such as π- π stacking and hydrophobic interactions, can also be considered. Although this phenomenon is still being studied, it seems that aromatic structures and functional groups such as OH and COOH are also involved. Further details about the hypothetical mechanism by which HA binds the AFB1 molecule are found in a previous study by our research group (18).
Another important result was that zeolite+AFB1 poults had BW, BWG, and FI similar to those in the positive control but lower than the negative control group. The FCR was similar among the positive control, negative control, and zeolite+AFB1-fed poults. These results indicate that the protective effect of zeolite against AFB1 was overlooked in the present study. Zeolite is known to bind AFB1, preventing its absorption into the body, and has been shown to be partially protective against high levels of AFB1 (32, 33). In the literature, no studies looking at the protective effects of zeolite against AFB1 on the performance of turkey poults were found. Nonetheless, it was recently demonstrated that supplementation with zeolite in the diets of female and male turkey poults had a positive effect on growth performance compared to the control group (34). This topic deserves further investigation.
In terms of relative liver weight, the negative control treatment had the highest, followed by the treatments with HA, HA+AFB1, zeolite+AFB1, and the positive control. Similar to these findings, in poults fed AFB1-contaminated corn, a reduction in liver weight was reported, while in poults fed uncontaminated corn, increased liver weight was observed (26). Reduced liver weight in poults fed aflatoxin-contaminated feeds was also reported compared to a control group fed non-contaminated feed (30). Rauber et al. (27) conducted a study on poults with different aflatoxin levels (20–1,000 ppb). The liver weight of control poults and those fed diets with aflatoxin levels between 20 and 500 ppb showed no significant difference, with the exception of the group fed 50 ppb, which displayed a lower liver weight. However, poults that were fed diets containing 1,000 ppb of aflatoxins demonstrated an increase in liver weight (27). Furthermore, enlargement of the liver was reported in poults fed AFB1-contaminated feeds (35). These discrepancies regarding the effect of AFB1 feeding on the liver of poults need further research.
A larger spleen was reported in poults fed aflatoxin-contaminated corn (26) and rations (35), while spleens of similar weight between poults fed aflatoxin-contaminated and non-contaminated feeds were reported (30). In this research, the larger spleen was found in zeolite+AFB1 and positive control poults and the smallest in the negative control group. These results agree with research in which larger spleens were seen in poults fed aflatoxin-contaminated feeds. Concerning the bursa of Fabricius, both HA treatments demonstrated higher weights, and the positive control showed lower weights. Different from these findings, significant atrophy and degenerative changes were observed in the bursa in a study involving 7-day-old female turkey poults challenged with 0.2 mg AFB1/kg; the changes included degeneration, necrosis, and depletion of lymphoid cells (36). The higher weight of the bursa of Fabricius in poults fed aflatoxin-contaminated corn was also reported (26), while in other reports, a lower bursa weight was recorded in poults fed aflatoxin-contaminated feed (30, 35). In HA and HA+AFB1-fed poults, the weight of the intestine was lower; this was surprising since, in other animal species, it has been reported that HS increases the size of the intestine, the viscosity in the lumen, the mucosal surface area, and the number of goblet cells, which leads to increased intestinal weight (17, 37).
No significant differences were found in the number of cells, hematocrit, heterophils, lymphocytes, basophils, or hetero/lymph ratio. Significant differences were observed only in monocyte counts in the HA treatment. Monocytes are derived from the bone marrow and released into the bloodstream. They have a brief circulation in the blood before migrating into the tissues and differentiating into tissue macrophages. Macrophages play critical roles in various physiological functions, including inflammation, wound healing, immune regulation, and metabolic homeostasis. Therefore, a decrease in the number of peripheral blood monocytes in birds fed contaminated diets suggests a potential reduction of synthesis in the bone marrow or increased clearance from the bloodstream (38). Our findings corroborate the results of a previous study conducted by Bujnák et al. (39), which also reported no significant changes in red and white blood cell parameters with the addition of HS on blood variables in finishing pigs.
In the AFB1 treatment group, a notable decrease in serum levels of total protein, AST, CK, GLDH, creatinine, BUN, glucose, Ca, and P was observed when compared to the negative control treatment. These findings indicate that AFB1 exposure significantly impacts various serum markers, suggesting potential alterations in liver function and metabolic processes. The inclusion of AFB1 in the feed had detrimental effects on serum protein, cholesterol, glucose, and uric acid levels, while the activity of AST and ALT enzymes increased in the turkey poults and poultry chickens (40). Furthermore, the activities of γ-glutamyl transferase, lactate dehydrogenase, and aspartate aminotransferase were found to be elevated in poultry exposed to AFB1 (1, 35).
HA exhibited positive effects on certain serum enzyme activities and helped alleviate the serum biochemical changes associated with aflatoxin toxicity (41). Moreover, there were reductions observed in the levels of serum albumin, total protein, uric acid, and cholesterol with HA supplementation (42). The protection of HS against liver injury was also evaluated in several studies in which hepatotoxicity was induced using lipopolysaccharide, carbon tetrachloride, and ethanol in rats, reporting reduced AST, ALT, and ALP serum concentrations (43, 44).
Compared to the negative control, the positive control exhibited a significant increase in cellular degeneration and fibrosis. No significant differences were observed between the positive control and the HA+AFB1 treatment. The histopathology studies provide further support for the findings, demonstrating that the liver of turkeys from the AFB1 treatment exhibited significant cellular degeneration and fat deposition. The observed enlargement and weight increase of the liver can be attributed to an accumulation of lipid content, leading to a condition known as a friable and fatty liver in the liver of chickens affected by aflatoxicosis (45). Moreover, in aflatoxicosis, the presence of severe inflammatory cell infiltrates, primarily composed of lymphocytes and heterophils, is frequently observed. This inflammatory response is believed to be a mechanism by which the body responds to the degeneration and vacuolation of hepatocytes caused by AFB1 exposure (46).
One of the main future challenges is to develop new procedures that may achieve comparable decontamination efficiencies in a broad spectrum of feed matrices because no general all-purpose decontamination methods could be broadly employed. Notably, the novel emerging decontamination technologies should not change the physical–chemical properties of the treated feed products significantly (47–49). The utilization of HS as feed additives has been extensively researched and has shown diverse advantages. These benefits are often observed when HS is combined with other feed additives, leading to synergistic or enhanced effects. The potential economic advantage for livestock and poultry producers lies in utilizing HA from high-quality sources and being administered according to dosage recommendations supported by research. This approach ensures the optimal use of HS and its associated benefits.
The results of this study provide confirmation that the presence of 250 ng AFB1/g had severe toxic effects on various aspects of performance, organ health, biochemical and hematological parameters, and hepatic injury in turkey poults over 28 days. The current research employed HA as a dietary inclusion in relatively small amounts, demonstrating its effective use as an additive. The findings strongly suggest that HA derived from worm compost has the potential to serve as an effective adsorbent for mitigating AFB1 toxicity in turkey poults. Despite these positive findings, it is important to note that research on HA as mycotoxin binders is still relatively limited, and further studies are needed to fully understand their long-term effects and optimal inclusion levels in feed formulations. Nonetheless, their promising efficacy makes them a valuable area of research for addressing mycotoxin-related challenges in poultry production. At present, our research team is conducting experiments to study the effects of HA throughout poultry production.
The original contributions presented in the study are included in the article/supplementary material, further inquiries can be directed to the corresponding authors.
The animal study was approved by all animals handing procedures complied with the Institutional Animal Care and Use Committee (IACUC) at the University of Arkansas, Fayetteville (Protocol No. 22020). The study was conducted in accordance with the local legislation and institutional requirements.
JM-G: Conceptualization, Writing—review and editing, Methodology, Writing—original draft. MN-R: Conceptualization, Methodology, Writing—original draft, Investigation. SG-R: Writing—original draft, Conceptualization, Validation, Writing—review and editing, Funding acquisition, Investigation, Project administration. MÁ: Methodology, Writing—original draft, Funding acquisition, Investigation, Project administration. BS-C: Methodology, Writing—original draft. DH-P: Methodology, Writing—original draft. RM-G: Conceptualization, Software, Writing—original draft. XH-V: Writing—review and editing. JH-R: Formal analysis, Methodology, Software, Writing—review and editing. IL: Software, Writing—review and editing. RS-C: Software, Writing—review and editing. JL: Formal analysis, Writing—review and editing. AV-D: Writing—review and editing, Formal analysis, Investigation. XD: Software, Writing—review and editing. AM-A: Conceptualization, Investigation, Writing—review and editing, Validation. BH: Funding acquisition, Project administration, Writing—review and editing, Validation. GT-I: Conceptualization, Funding acquisition, Project administration, Writing—original draft, Writing—review and editing, Investigation, Validation.
The author(s) declare financial support was received for the research, authorship, and/or publication of this article. This research was supported by the Mexican Council of Science and Technology (CONACYT), the UNAM-PAPIIT grant number PDCPN_2017_4777, and by USDA Animal Health Awards (FY2021 and FY2022), and by USDA-NIFA Sustainable Agriculture Systems, Grant No. 2019-69012-29905. Title of Project: Empowering U.S. Broiler Production for Transformation and Sustainability USDA-NIFA.
The authors declare that the research was conducted in the absence of any commercial or financial relationships that could be construed as a potential conflict of interest.
The author(s) declared that they were an editorial board member of Frontiers, at the time of submission. This had no impact on the peer review process and the final decision.
All claims expressed in this article are solely those of the authors and do not necessarily represent those of their affiliated organizations, or those of the publisher, the editors and the reviewers. Any product that may be evaluated in this article, or claim that may be made by its manufacturer, is not guaranteed or endorsed by the publisher.
1. Monson M, Coulombe R, Reed K. Aflatoxicosis: lessons from toxicity and responses to aflatoxin B1 in poultry. Agriculture. (2015) 5:742–77. doi: 10.3390/agriculture5030742
2. Ijaz MU, Ishtiaq A, Ehsan N, Imran M, Zhu G. Hepatoprotective potential of genkwanin against aflatoxin B1-induced biochemical, inflammatory and histopathological toxicity in rats. PVJ. (2022) 42:493–8. doi: 10.29261/pakvetj/2022.048
3. Bhat R, Rai RV, Karim AA. Mycotoxins in food and feed: present status and future concerns. Compr Rev in Food Sci and Food Saf. (2010) 9:57–81. doi: 10.1111/j.1541-4337.2009.00094.x
4. Kiliç KD. Liver Histology and biochemistry of exposed newborn and infant rats with experimental aflatoxicosis. PVJ. (2022) 42:453–60. doi: 10.29261/pakvetj/2022.066
5. Binder EM, Tan LM, Chin LJ, Handl J, Richard J. Worldwide occurrence of mycotoxins in commodities, feeds and feed ingredients. Ani Feed Sci and Technol. (2007) 137:265–82. doi: 10.1016/j.anifeedsci.2007.06.005
6. Fouad A, Ruan D, El-Senousey H, Chen W, Jiang S, Zheng C. Harmful effects and control strategies of aflatoxin B1 produced by aspergillus flavus and aspergillus parasiticus strains on poultry: review. Toxins. (2019) 11:176. doi: 10.3390/toxins11030176
7. Haque MA, Wang Y, Shen Z, Li X, Saleemi MK, He C. Mycotoxin contamination and control strategy in human, domestic animal and poultry: a review. Microb Patho. (2020) 142:104095. doi: 10.1016/j.micpath.2020.104095
8. Nazhand A, Durazzo A, Lucarini M, Souto EB, Santini A. Characteristics, occurrence, detection and detoxification of aflatoxins in foods and feeds. Foods. (2020) 9:644. doi: 10.3390/foods9050644
9. Kolawole O, Siri-Anusornsak W, Petchkongkaw A, Meneely J, Elliott C. The efficacy of additives for the mitigation of aflatoxins in animal feed: a systematic review and network meta-analysis. Toxins. (2022) 14:707. doi: 10.3390/toxins14100707
10. Ye S-Q, Lv X-Z, Zhou A-G. In vitro evaluation of the efficacy of sodium humate as an aflatoxin b1 adsorbent. Aust J Basic Appl Sci. (2009) 3:1296–300.
11. Tan KH. Humic Matter in Soil and the Environment: Principles and Controversies. Boca Raton: CRC Press (2003), p. 408.
12. Peña-Méndez EM, Havel J, Patočka J. Humic substances—compounds of still unknown structure: applications in agriculture, industry, environment, and biomedicine. J Appl Biomed. (2005) 3:13–24. doi: 10.32725/jab.2005.002
13. Vašková J, Stupák M, Vidová Ugurbaş M, Žatko D, Vaško L. Therapeutic efficiency of humic acids in intoxications. Life. (2023) 13:971. doi: 10.3390/life13040971
14. Gomez-Rosales S, de Angeles M. Addition of a worm leachate as source of humic substances in the drinking water of broiler chickens asian-australas. J Anim Sci. (2015) 28:215–22. doi: 10.5713/ajas.14.0321
15. Domínguez-Negrete A, Gómez-Rosales S, Angeles M de L, López-Hernández LH, Reis de. Souza TC, Latorre-Cárdenas JD, Téllez-Isaias G. Addition of different levels of humic substances extracted from worm compost in broiler feeds. Animals (Basel). (2021) 11:3199. doi: 10.3390/ani11113199
16. Maguey-Gonzalez JA, Michel MA, Baxter MFA, Tellez G, Moore PA, Solis-Cruz B, et al. Effect of humic acids on intestinal viscosity, leaky gut and ammonia excretion in a 24 hr feed restriction model to induce intestinal permeability in broiler chickens. Anim Sci J. (2018) 89:1002–10. doi: 10.1111/asj.13011
17. de Lourdes Angeles M, Gómez-Rosales S, Téllez-Isaias G. Mechanisms of Action of Humic Substances as Growth Promoters in Animals. In: Humus and Humic Substances—Recent Advances. IntechOpen (2022).
18. Maguey-González JA, Nava-Ramírez M de J, Gómez-Rosales S, Ángeles M de L, Solís-Cruz B, Hernández-Patlán D, et al. Humic acids preparation, characterization, and their potential adsorption capacity for aflatoxin b1 in an in vitro poultry digestive model. Toxins. (2023) 15:83. doi: 10.3390/toxins15020083
19. Shotwell OL, Hesseltine CW, Stubblefield RD, Sorenson WG. Production of aflatoxin on rice. Appl Microbiol. (1966) 14:425–8. doi: 10.1128/am.14.3.425-428.1966
20. Göbel R, Lusky K. Simultaneous determination of aflatoxins, ochratoxin a, and zearalenone in grains by new immunoaffinity column/liquid chromatography. J of AOAC Int. (2004) 87:411–6. doi: 10.1093/jaoac/87.2.411
21. Maguey-Gonzalez JA, Michel MA, Baxter MFA, Solis-Cruz B, Hernandez-Patlan D, Merino-Guzman R, et al. Effects of humic acids on recovery of salmonella enterica serovar enteritidis. Ann of Anim Sci. (2018) 18:387–99. doi: 10.1515/aoas-2017-0037
22. Petrone VM, Escorcia M, Fehervari T, Téllez IG. Evaluation of an early granulocytic response of chick embryos inoculated with herpesvirus of Turkeys. Br Poult Sci. (2002) 43:213–7. doi: 10.1080/00071660120121418
24. Mishra SK, Swain BK, Mishra SK, Swain BK. “Aflatoxin Occurrence, Detection, and Novel Strategies to Reduce Toxicity in Poultry Species.,” In: Aflatoxins—Occurrence, Detection and Novel Detoxification Strategies. IntechOpen (2022).
25. Giambrone JJ, Diener UL, Davis ND, Panangala VS, Hoerr FJ. Effects of aflatoxin on young Turkeys and broiler chickens1. Poult Sci. (1985) 64:1678–84. doi: 10.3382/ps.0641678
26. McKenzie KS, Kubena LF, Denvir AJ, Rogers TD, Hitchens GD, Bailey RH, et al. Aflatoxicosis in turkey poults is prevented by treatment of naturally contaminated corn with ozone generated by electrolysis. Poult Sci. (1998) 77:1094–102. doi: 10.1093/ps/77.8.1094
27. Rauber RH, Dilkin P, Giacomini LZ, de Almeida CAA, Mallmann CA. Performance of Turkey poults fed different doses of aflatoxins in the diet1. Poult Sci. (2007) 86:1620–4. doi: 10.1093/ps/86.8.1620
28. Singh R, Shrivastava HP, Shrivastav AK. Effect of dietary aflatoxin on the performance of turkey poults. Indian Jl of Poult Sci. (2011) 46:185–8.
29. Oyegunwa AS, Ewuola EO, Agboola AF, Iyayi EA. Performance and blood profile of turkey poults fed with different doses of aflatoxin in the diets. Ethiopian J of Appl Sci and Tech. (2017) 8:19–27.
30. Gómez-Espinosa D, Cervantes-Aguilar F, Del Río-García J, Villarreal-Barajas T, Vázquez-Durán A, Méndez-Albores A. Ameliorative effects of neutral electrolyzed water on growth performance, biochemical constituents, and histopathological changes in turkey poults during aflatoxicosis. Toxins. (2017) 9:104. doi: 10.3390/toxins9030104
31. Piccolo A. The supramolecular structure of humic substances: A novel understanding of humus chemistry and implications in soil science. Adv Agron. (2002). 2:57–134 doi: 10.1016/S0065-2113(02)75003-7
32. Miazzo R, Rosa CAR, De Queiroz Carvalho EC, Magnoli C, Chiacchiera SM, Palacio G, et al. Efficacy of synthetic zeolite to reduce the toxicity of aflatoxin in broiler chicks. Poult Sci. (2000) 79:1–6. doi: 10.1093/ps/79.1.1
33. Ortatatli M, Oguz H, Hatipoglu F, Karaman M. Evaluation of pathological changes in broilers during chronic aflatoxin (50 and 100 ppb) and clinoptilolite exposure. Res in Vet Sci. (2005) 78:61–8. doi: 10.1016/j.rvsc.2004.06.006
34. Hcini E, Ben Slima A, Kallel I, Zormati S, Traore AI, Gdoura R. Does supplemental zeolite (clinoptilolite) affect growth performance, meat texture, oxidative stress and production of polyunsaturated fatty acid of Turkey poults? Lipids Health Dis. (2018) 17:177. doi: 10.1186/s12944-018-0820-7
35. Singh R. Ameliorative Effects of Mycodetox B1 on Production performance, organ weights and serum biochemicals during aflatoxicosis in turkey poults. Livest Res Int. (2019) 07:40–18.
36. Grozeva N, Valchev I. Lazarov L, Hristov Ts, Kanakov D, Dimitrov K, Binev R. Cloacal bursa morphology in turkey broilers challenged with aflatoxin B1 alone or co-administered with Mycotox NG. Bul J Vet Med. (2020) 23:121–9. doi: 10.15547/bjvm.2140
37. López-García YR, Gómez-Rosales S, Angeles M de L, Jiménez-Severiano H, Merino-Guzman R, Téllez-Isaias G. Effect of the addition of humic substances on morphometric analysis and number of goblet cells in the intestinal mucosa of broiler chickens. Animals. (2023) 13:212. doi: 10.3390/ani13020212
38. Swamy HVLN, Smith TK, Karrow NA, Boermans HJ. Effects of feeding blends of grains naturally contaminated with Fusarium mycotoxins on growth and immunological parameters of broiler chickens. Poult Sci. (2004) 83:533–43. doi: 10.1093/ps/83.4.533
39. Bujnák L, Hreško Šamudovská A, Mudronová D, Nad P, Marcinčák S, Maskalová I, et al. The effect of dietary humic substances on cellular immunity and blood characteristics in piglets. Agriculture. (2023) 13:636. doi: 10.3390/agriculture13030636
40. Muhammad KS, Ahmad R, Aisha K, Muhammad Z, Yongping X, Bilal M, et al. Toxic Effects of aflatoxin b1 on hematobiochemical and histopathological parameters of juvenile white leghorn male birds and their amelioration with vitamin e and moringa oleifera. PVJ. (2023) 23:53. doi: 10.29261/pakvetj/2023.053
41. Ghahri H, Habibian R, Fam MA. Evaluation of the efficacy of esterified glucomannan, sodium bentonite, and humic acid to ameliorate the toxic effects of aflatoxin in broilers. Turk J of Vet & Anim Sci. (2010) doi: 10.3906/vet-0903-19
42. Akaichi A, Jebali A, Benlarbi M, Mahjoub T, Kaboudi K, Chaouacha-Chekir RB, et al. Effects of humic acid and organic acids supplements on performance, meat quality, leukocyte count, and histopathological changes in spleen and liver of broiler chickens. Res in Vet Sci. (2022) 150:179–88. doi: 10.1016/j.rvsc.2022.07.001
43. Vetvicka V, Garcia-Mina JM, Proctor M, Yvin J-C. Humic acid and glucan: protection against liver injury induced by carbon tetrachloride. J of Med Food. (2015) 18:572–7. doi: 10.1089/jmf.2014.0091
44. Vetvicka V, GarciaMina J, Yvin J. Prophylactic effects of humic acid and #8211; glucan combination against experimental liver injury. J Intercult Ethnopharmacol. (2015) 4:249. doi: 10.5455/jice.20150519103113
45. McLean M, Dutton MF. Cellular interactions and metabolism of aflatoxin: an update. Pharmacol & Ther. (1995) 65:163–92. doi: 10.1016/0163-7258(94)00054-7
46. Hinton DM. Immunotoxicity of aflatoxin b1 in rats: effects on lymphocytes and the inflammatory response in a chronic intermittent dosing study. Toxicol Sci. (2003) 73:362–77. doi: 10.1093/toxsci/kfg074
47. Sipos P, Peles F, Brassó DL, Béri B, Pusztahelyi T, Pócsi I, et al. Physical and chemical methods for reduction in aflatoxin content of feed and food. Toxins. (2021) 13:204. doi: 10.3390/toxins13030204
48. Council NR, Agriculture B on Nutrition Nutrition S on P. Nutrient Requirements of Poultry: Ninth Revised Edition, 1994. New York, NY: National Academies Press (1994), p. 174.
Keywords: aflatoxin B1, humic acids, adsorbents, turkey poults, performance parameters
Citation: Maguey-González JA, Nava-Ramírez MdJ, Gómez-Rosales S, Ángeles MdL, Solís-Cruz B, Hernández-Patlán D, Merino-Guzmán R, Hernandez-Velasco X, Hernández-Ramírez JO, Loeza I, Senas-Cuesta R, Latorre JD, Vázquez-Durán A, Du X, Méndez-Albores A, Hargis BM and Téllez-Isaías G (2023) Evaluation of the efficacy of humic acids to counteract the toxic effects of aflatoxin B1 in turkey poults. Front. Vet. Sci. 10:1276754. doi: 10.3389/fvets.2023.1276754
Received: 12 August 2023; Accepted: 18 September 2023;
Published: 10 October 2023.
Edited by:
Ilias Giannenas, Aristotle University of Thessaloniki, GreeceReviewed by:
Aisha Khatoon, University of Agriculture, Faisalabad, PakistanCopyright © 2023 Maguey-González, Nava-Ramírez, Gómez-Rosales, Ángeles, Solís-Cruz, Hernández-Patlán, Merino-Guzmán, Hernandez-Velasco, Hernández-Ramírez, Loeza, Senas-Cuesta, Latorre, Vázquez-Durán, Du, Méndez-Albores, Hargis and Téllez-Isaías. This is an open-access article distributed under the terms of the Creative Commons Attribution License (CC BY). The use, distribution or reproduction in other forums is permitted, provided the original author(s) and the copyright owner(s) are credited and that the original publication in this journal is cited, in accordance with accepted academic practice. No use, distribution or reproduction is permitted which does not comply with these terms.
*Correspondence: Sergio Gómez-Rosales, Y2hlY29ncjhAZ21haWwuY29t; Abraham Méndez-Albores, YWxib3Jlc0B1bmFtLm14
Disclaimer: All claims expressed in this article are solely those of the authors and do not necessarily represent those of their affiliated organizations, or those of the publisher, the editors and the reviewers. Any product that may be evaluated in this article or claim that may be made by its manufacturer is not guaranteed or endorsed by the publisher.
Research integrity at Frontiers
Learn more about the work of our research integrity team to safeguard the quality of each article we publish.