- 1Department of Veterinary Medicine, University of Bari, Valenzano, Italy
- 2Department of Agricultural Sciences, University of Naples Federico II, Portici, Italy
The safety of reclaimed urban wastewater (RUW) for the production of hydroponic barley forage (HBF) was evaluated in terms of effluent and forage characteristics, as well as the health and performance of lactating cows. The study was conducted on a dairy farm equipped with two hydroponic chambers producing approximately 620 kg/d of HBF as fed. For experimental purposes, HBF was produced using RUW collected from an aqueduct plant processing urban wastewater in a membrane bioreactor treatment chain. A feeding trial was carried out with HBF derived from RUW. Sixty lactating cows were randomly assigned to two balanced groups fed a standard total mixed ration (TMR) or a TMR in which 10 kg of HBF replaced 1 kg of oat hay and 0.5 kg of maize. The experimental period lasted 7 weeks, including a 2-week adaptation period, during which each cow underwent a physical examination, BCS scoring, blood sampling for a complete blood count and biochemical panel, recording of body weight and milk yield and quality, including fatty acid composition and heavy metal content. Ruminal pH was continuously monitored by reticulorumen boluses, and nutrient digestibility and N balance were determined at week 7. RUW showed an acceptable microbial load and an overall good quality as irrigation water, even though the supply of N and P did not influence the yield and quality of HBF. The characteristics of HBF reflected the quality of RUW supplied to the hydroponic chambers and no anomalous components (i.e., high ion concentration) were found. Feeding RW-derived HBF to lactating cows had no major positive or negative effects on animal health and production, including milk quality, ruminal pH, in vivo digestibility, and N balance. The use of RUW under the conditions tested appears to be safe for the health status of lactating cows and the quality of the milk obtained. Overall, the results do not reveal any major limitations for the use of tertiary wastewater as irrigation water for the hydroponic production of forage barley, so that a wider use of wastewater in hydroponic systems seems realistic.
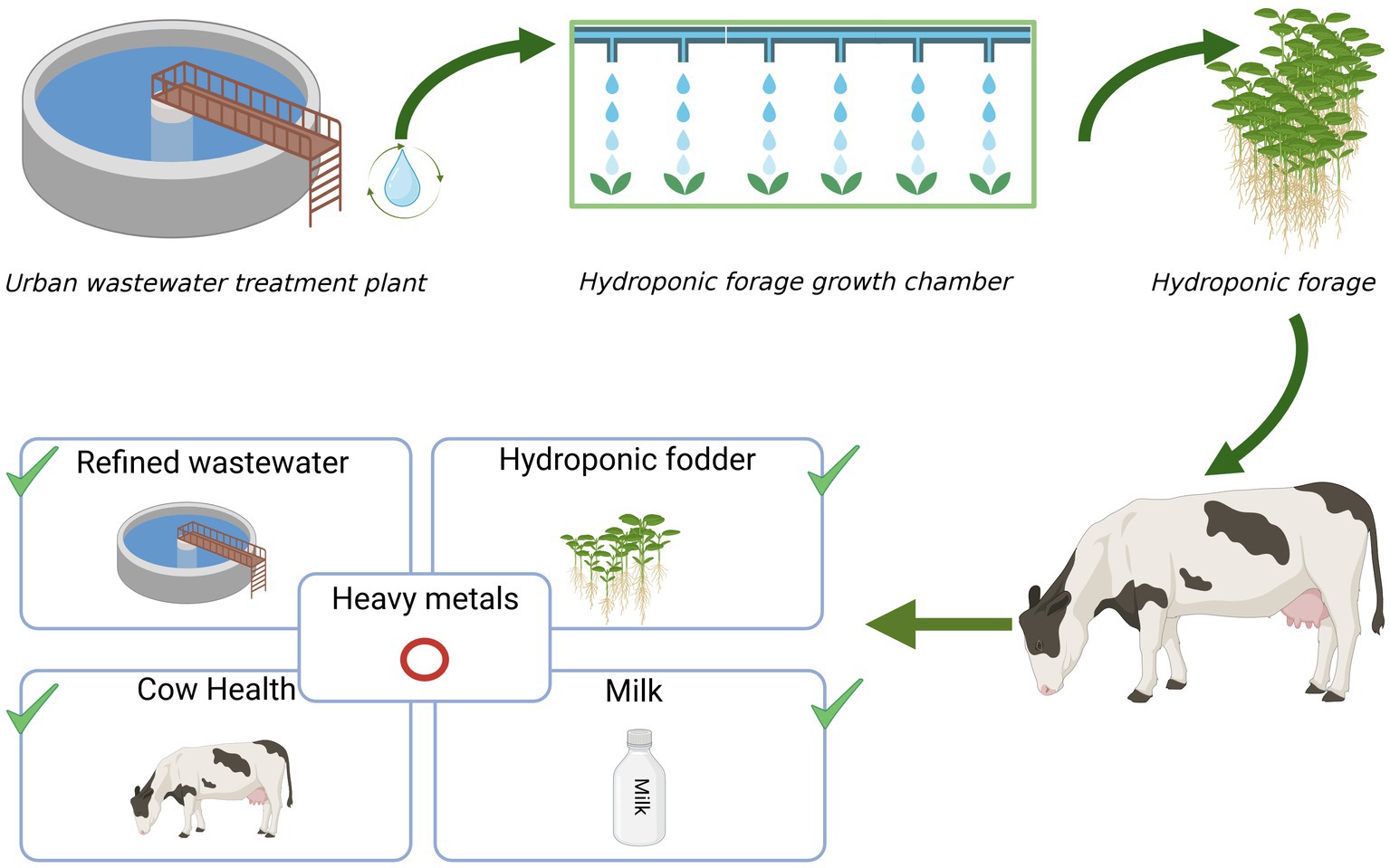
Created with BioRender.com.
1 Introduction
Ongoing climate change threatens global freshwater security which is one of the United Nations sustainable development goals (1). Despite the inevitable uncertainty in forecasting models, the countries of the Southern European Union (EU) are expected to experience severe changes in both the availability and quality of water resources, which potentially lead to unprecedented pressure on the water resources (2). Currently, the agricultural sector of some Southern regions of the Euro-Mediterranean area already uses 80% of water resources, while the rest of Europe consumes only 44% and the world about 75%, on average (3, 4). Within the primary sector, it is estimated that livestock production uses 29% of the total agriculture water demand, with a large going to crops to produce animal feed (5). In this scenario, recovering urban treated or reclaimed wastewater for irrigation purposes has an enormous potential to face increasing water scarcity, preserving potable resources and reducing the environmental impact associated with the discharge of effluents into water bodies (6, 7). To date, the use of reclaimed wastewater in producing vegetables and fruit for human consumption has been successfully implemented in many rural Mediterranean areas, proving an economically feasible option to mitigate the water consumption associated with several crops production (8–11). However, less attention has been paid to the use of wastewater in forage and feed production systems (5, 12).
The use of wastewater for agricultural purposes presents concerns related to the presence of pathogenic microorganisms that could be a source of infection for humans and animals (5, 13). Similarly, there may be organic and inorganic chemicals that can enter the food chain, accumulate in the soil, be absorbed by plants, and then have humans as final consumers (14, 15). Currently, in the European context, the use of wastewater for irrigation is strictly regulated. In treatment plants, urban wastewater, as defined by EU Directive 91/271, undergoes sequential treatment to remove suspended solids and pollutants as well as unwanted compounds and microorganisms (16). Wastewater treated to remove pathogens and stabilize water quality, hereafter referred to as reclaimed urban wastewater (RUW), can be used for irrigation of raw and processed food crops and non-food crops, such as pasture and forage (17). In this scenario, hydroponics is now seen as an agricultural technology that can be easily adapted to reuse alternative water sources, reducing both irrigation water demand and wastewater disposal, and improving the environmental and economic sustainability of crop production and wastewater treatment technologies (18). Hydroponics is a type of vertical, soilless, indoor cultivation in which the growing environment is controlled in terms of temperature, humidity, light and in which the use of chemicals tends to be much lower than in traditional crop systems (19, 20). In recent years, hydroponics has also been proposed to produce fresh forage, a natural, palatable, and easily digestible feed that can improve the health and performance of lactating animals and even the nutraceutical properties of dairy products (21–23), which is however not available year-round in the climatic conditions of southern Europe (24, 25). Fresh hydroponic forage (HF) production is based on indoor germination and growth for a short time (6–8 d) of cereal seeds (especially barley) characterized by high and rapid germinability (26). In addition to producing fresh, high-quality forage all year round, regardless of weather conditions and without land requirements, HF production is also expected to reduce water consumption compared to conventional forage crops (27, 28) and has been proposed as a suitable system for livestock farms with limited availability of arable land and irrigation water (29, 30). The use of alternative water sources has been found to increase water use efficiency as well as the nutritional quality of HF (31–34), but the effects of such forage on animal performance and health have not been addressed so far.
Furthermore, in the European context, taking into consideration the requirements defined by the recently enacted European regulation 2020/741 (17), it is pivotal to assess the potential animal health risks associated with the reuse of RUW for hydroponic fodder production. Indeed, though hydroponic fodder is not explicitly mentioned in the EU regulation, it falls under the category “non-food crops,” including pasture and forage.
Thus, assuming its safety, this study was designed as a first attempt to evaluate the use of RUW for HBF production in terms of water characteristics, feed quality, and effects on the health and production of dairy cows, elements that have been considered separately or not at all in the available literature.
2 Materials and methods
2.1 Ethics approval
The study was conducted according to the guidelines of the Declaration of Helsinki and approved by the Institutional Review Board of the Italian Ministry of Health (protocol code 74371-X/10, n. 1,031/2020-PR, Date of approval 19, November 2021). The withdrawal and use of RUW were authorized by the Apulian Aqueduct (Acquedotto Pugliese S.p.A.) which was a partner in the framework of the Hydrofodderpuglia research project financed by the Apulian region (PO FE5R 2014/2020-Azione 6.4-Sub-Az 6.4.a.DGR 2321/2017).
2.2 Study site and production of hydroponic barley fodder
The study was carried out on a dairy farm in the Apulian region, in southern Italy (40°48′N 16°56′E; 360 m a.s.l.). The farm has two hydroponics chambers (E-6-TC and EC-2-T models, Eleusis International, Madrid, Spain) with a potential daily production of hydroponic barley fodder (HBF) of 500 kg and 120 kg and a capacity of 32 and 8 perforated polyethylene trays (60×60 cm), respectively. Regardless of production capacity, each chamber consisted of a well-insulated room equipped with climate control, air extractors, a surface irrigation watering system, a fluorescent tube lighting system (40 W, with 12 to 16 daily light), and a set of shelves where the seed germination trays are placed. The temperature inside the chambers was set to a working range of 18°C to 21°C and the relative humidity was adjusted by about 70% using air circulation. Clean, sound, intact, untreated, barley seeds (Hordeum vulgare L.) of high quality (germination above 95%) were used. Daily, the seeds were first soaked in 1.5% sodium hypochlorite solution for 30 min, rinsed, and soaked in fresh water for 16 h. Then, the water was drained, and the seeds were left without water for 24 h (pre-germination). Subsequently, the seeds were spread into the perforated polyethylene trays at a sowing rate of 4–6 kg/m2. The growth cycle, from seed placement to harvest lasted 8 days, after which the fodder, consisting of a mass of roots, seed kernels and the aerial green part of the seedlings (from 12 to 14 cm), was manually discharged from the trays, scored for the presence of mould according to the five-point scale of Soder et al. (35), and loaded into the mixer wagon equipped with an electronic scale for inclusion in the total mixed ration (TMR).
For experimental purposes, HBF was produced using RUW taken from the treatment plant of the Apulian aqueduct located in Noci (40°48′N 17°08′E), which adopts a membrane bio reactor wastewater treatment chain. Once a week, RUW was taken from the waterworks reservoirs, transported to the farms in a dedicated tank and stored in non-toxic polyethylene tanks with the weekly addition of chlorine dioxide (0.58 ppm/L), connected to the growth chamber irrigation system. The amount of water required to daily produce 620 kg of HBF was approximately 1.5 m3. Before the start of the feeding trial, the characteristics of RUW were assessed for the main water quality parameters (17, 36) by a reference laboratory (EuroQuality Lab S.r.l., Gioia del Colle, Italy) according to standard methods (Supplementary Table S1).
To evaluate the potential influence of RUW on forage growth, the yield and height of forage produced using well water and RUW were measured in October 2021. Measurements were taken in both chambers for 10 consecutive days for each type of water. Furthermore, two samples of the forages so produced were evaluated for the presence and load of Escherichia coli (β-glucuronidase method) and Salmonella spp. and for the content of metals and non-metals (N, P, Na, Cd, Cr, Cu, Mn, Ni, Pb, Zn, Fe, K, Ca, Mg) by a reference laboratory (EuroQuality Lab S.r.l., Gioia del Colle, Italy) according to standard methods (Supplementary Table S2).
2.3 Animal enrolment
In November 2021, the health status of eighty lactating cows from the herd was evaluated. The animals were visually examined, weighed, and scored for body condition using a 1 to 5 scale (37). Blood samples were taken from the coccygeal vein, immediately used for quantification of the beta-hydroxybutyrate (BHB) level using a ketometer (CentriVet GK), and placed in a K3 EDTA tube (10 mL) to perform a complete blood count (CBC) (CELL-DYN 3700 Hematology Analyzer, Abbott), and in a plain tube (5 mL) to obtain serum after centrifugation (15 min at 1500 × g) for the biochemical panel (including albumin (Alb), alkaline phosphatase (ALP), alanine transaminase (ALT), aspartate transaminase (AST), calcium (Ca), chloride (Cl), creatinine (Cr), glucose (Glu), potassium (K), magnesium (Mg), sodium (Na), not esterified fatty acids (NEFA), phosphorus (PHOS), total bilirubin (T Bil), total iron (Ti), total proteins (Tp); Beckman Coulter, Clinical Chemistry Analyzer AU680). Furthermore, faecal samples (about 10 g) were collected from the rectum by using sterile polyethylene gloves, stored in plastic bags, and examined for gastrointestinal helminths (38). Deworming with eprinomectin (Eprinex Multiple Pour, Boehringer Ingelheim) was performed due to positive results. To be enrolled in the study, cows had to be clinically healthy, have a temperature of 38.8°C, 5–6 rumen contractions every three minutes measured by auscultation of the lumbar fossa, and no clinical signs of laminitis, metritis, and mastitis.
2.4 Study design, animals, and diets
Sixty dairy cows matched the selection criteria and were enrolled in a prospective, randomized, controlled, on field study that lasted from December 2021 to February 2022. The enrolled cows were randomized into two groups balanced for breed, milk yield, days in milk (DIM), parity, body condition score (BCS), and body weight (BW) (Supplementary Table S3). The control group (CG) was fed the TMR used by the farmer, while the other group (HBFG) was fed the same TMR in which 10 kg of HBF replaced 1 kg of oat hay, and 0.5 kg of maize. The diets were isonitrogenous and isoenergetic and formulated for a production level of 28 kg of energy-correct milk (ECM) per day (39). Rations were fed twice a day (08:00 and 15:00) in equal amounts, with several pushing of feed to the animals. Feed refusals (5%–10%) were removed daily before discharging the freshly prepared TMR. Animals had free access to drinking water. The groups were housed in two adjacent concrete-floored barns equipped with deep-bedded stalls (2.6 × 1.25 m, sand bedding) and an open-access clay training field (30 × 10 m). The trial lasted 9 weeks, 2 weeks for adaptation to diets, and 7 for experimental measurements and sampling according to the schedule shown in Figure 1.
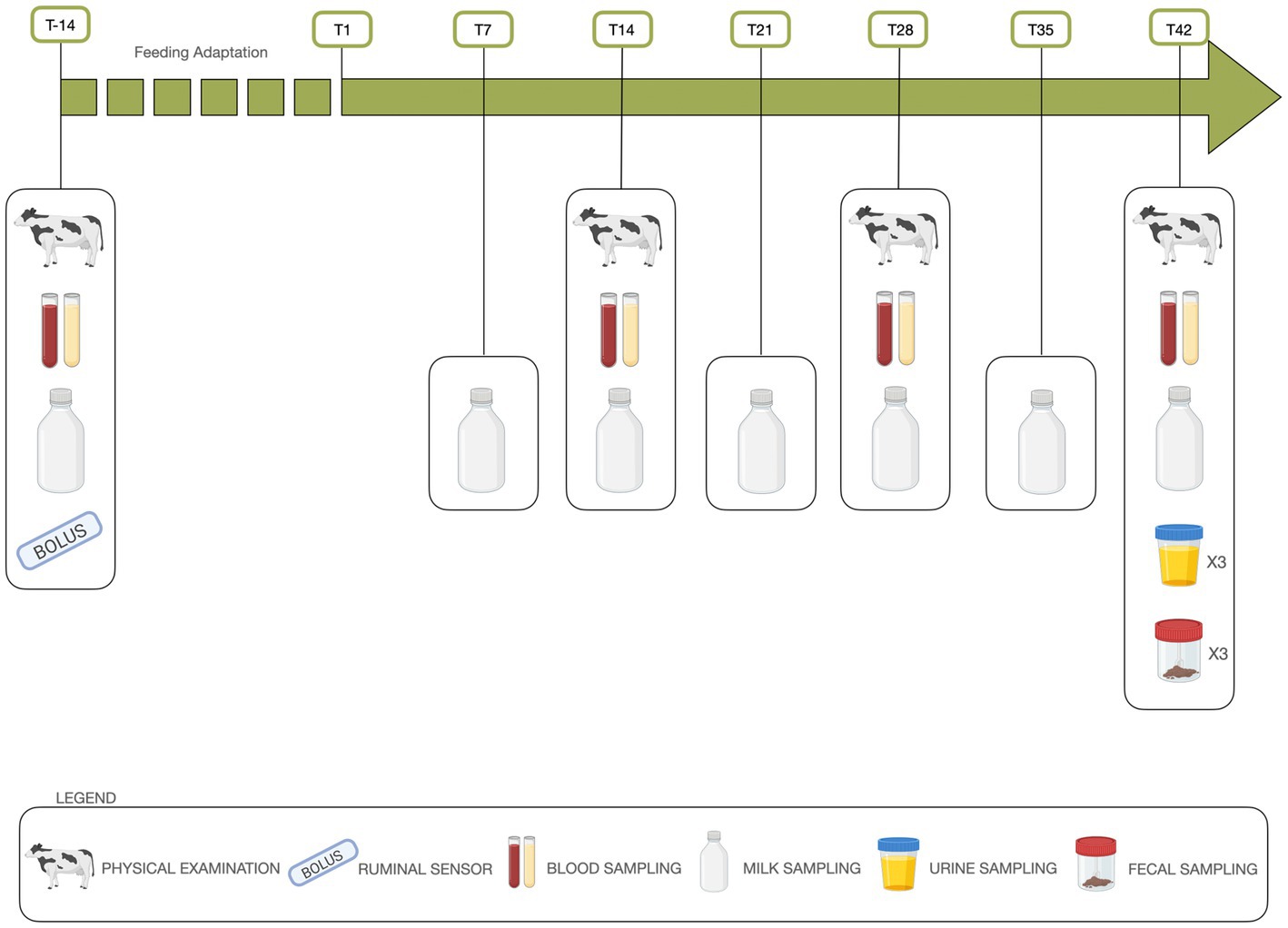
Figure 1. Timeline of the study project including time points and scheduled procedures. Created with BioRender.com.
2.5 Physical examination and blood sampling
The physical examination, body weight recording, BCS scoring, and blood sampling of the enrolled cows were repeated at T-14, T14, T28, and T42 as described above (Figure 1).
2.6 Dry matter intake, feed sorting, and feed analyses
Feed intake was weekly measured on a group basis by the difference between TMR discharged and residuals. Feeds and TMR were also sampled, pooled by group, and analyzed to determine the content of dry matter (DM; method 930.15), ash (method 942.05), crude protein (CP; method 976.05), and ether extract (EE; method 954.02) (40). Neutral detergent fiber (NDF), acid detergent fiber (ADF) inclusive of residual ash (41), acid detergent lignin (ADL) (42), total sugar (43), and enzymatic starch (44) were also determined. Proteins were fractionated (PA, PB1, PB2, PB3, PC) according to the Cornell Net Carbohydrate and Protein System (CNCPS) version 6.5 (45). The non-fibrous carbohydrates (NFC) were calculated as detailed elsewhere (46, 47). Additional samples of TMRs and refusals were taken every two weeks during the sampling period to assess physical structure of the diets (particle size, and physically effective NDF) and sorting activity by the cows. The particle size distribution of the fresh TMRs and refusals was stratified into long (>19 mm), medium (<19 mm, >8 mm), and short (<8 mm; Pan) particles using a Penn State particle separator (PSPS) with two screens (19 and 8 mm) (48). The physical effectiveness factor (pef), physically effective NDF (peNDF), and feed sorting index (SI) of the diets were determined according to DeVries et al. (49).
2.7 Milk sampling and analysis
Milk yield of each cow was measured using an electronic machine recorder (DeLaval Corp., Tumba, Sweden) and sampled in the morning milking (07:00) at weekly intervals (Figure 1). The samples were sent refrigerated at 4°C to the laboratory for the analysis of fat, protein, casein, lactose, total solids, non-fat solids, milk urea nitrogen (MUN), cryoscopy index, titration acidity, somatic cell count (SCC) and milk clotting properties which were performed on the same day using an infrared milk analyzer (CombiFoss TM7, Foss, Hillerød, Denmark). At T42, two more milk samples were collected from a selected subgroup of 10 cows/diet. One was evaluated for heavy metal concentration (As, Cd, Co, Cu, Ni, Pb) by inductively coupled plasma mass spectrometry (ICP-MS) while the other was used to determine fatty acid (FA) composition as detailed elsewhere (50). Milk fat was extracted by the Röse-Gottlieb method, the Supelco 37 component mixture (Supelco, Bellefonte, PA, United States) and a mixture of conjugated linoleic acid isomers (Nu-Chek Prep. Inc. Elysian, MN, United States) were used as external standards for gas chromatographic analysis.
2.8 Monitoring of ruminal pH
At enrolment (Figure 1), all cows received an indwelling wireless reticolo-ruminal sensor (pH Plus Bolus; smaXtec Animal Care GmbH, Graz, Austria) according to the manufacturer’s directions. Boluses provided continuous measurement of rumen pH, body temperature and activity levels of animals. The data were recorded every 10 min, transmitted wirelessly to a base station placed in the barn, and available in real time from a computer software/cell phone application. The risk of subacute rumen acidosis (SARA) was notified by the ruminal bolus when the pH value fell below 5.8. The daily pH mean, minimum and maximum values were calculated per cow and per day. The percentage of animals that presented at least one SARA risk alarm, number, and time (min) of SARA risk alarms were also measured.
2.9 Total tract apparent digestibility, microbial protein synthesis, and nitrogen balance
At T42 and for 3 consecutive days, faecal grab samples (∼200 g) were collected three times daily from a selected subgroup of 10 cows/diet, kept at +4°C in closed plastic bags, and then composited by cow. Samples of faeces, diets and refusals were dried, ground and analyzed for acid insoluble ash (AIA) (51), as well as for ash, organic matter (OM), CP, and NDF. The AIA content was used as an internal marker to estimate the apparent total tract digestibility of nutrients and the fecal output of DM (52).
At the same time as the faecal sampling, spot urine samples were also gathered either through voluntary urination or stimulation of the pudendal nerve according to the procedure of Spanghero (53). The samples (∼100 mL) were immediately acidified (10% v/v with sulfuric acid solution) and stored at −20° C until analysis. After thawing (4° C), the samples were assessed for total N (40) and creatinine and purine derivatives (PD; i.e., allantoin and uric acid) as described by George et al. (54). Daily urine volume and excretion of total N, allantoin, uric acid, and total PD (allantoin plus uric acid) were estimated based on BW and urinary creatinine concentration assuming a constant creatinine excretion rate of 29 mg/kg of BW (55). The ruminal synthesis of microbial nitrogen (MN) was estimated from the excretion of PD using the equation of Chen and Gomez (56). Nitrogen balance (NB) was calculated as reported by Spanghero and Kowalski (57).
2.10 Statistical analysis
The a priori sample size was based on 30 as a minimum and the ratio of 1:1 sample size was chosen. Bovine characteristics are reported as mean ± standard deviation (M ± SD), and as frequencies and percentages (%) for categorical variables. For testing the associations and the corrected randomization between arms (HBFG vs CG), the Wilcoxon rank-sum (Mann–Whitney) for continuous, and Chi-square or proportion test for categorical were used. Before statistical analysis, normality and homogeneity of variance of data were tested by the tests of Shapiro-Wilks and Levene, respectively. Repeated measures ANOVA was used to determine the mean difference of repeated parameters (namely BW, BCS, milk production, biochemical panel, and CBC) with treatment, time, and interaction as factors. Data on dry matter intake, in vivo digestibility, and nitrogen balance were analyzed by one-way ANOVA to determine the fixed effects of the treatment. Rumen bolus data were analyzed using the Wilcoxon rank sum test (Mann–Whitney). When testing the null hypothesis of no association, the probability level of error at two tails was 0.05. All statistical computations were made using StataCorp. 2021. Stata Statistical Software: Release 17. College Station, TX: StataCorp LLC.
3 Results
3.1 Reclaimed urban wastewater and hydroponic forages findings
The microbiological and chemical characteristics of the RUW used for HBF production are shown in Tables 1, 2. To assess the irrigation quality of RUW, the guidelines reviewed by Hashem and Qi (14) and Ayers and Westcot (36) were used for the parameters not included in Regulation 2020/741 (17).
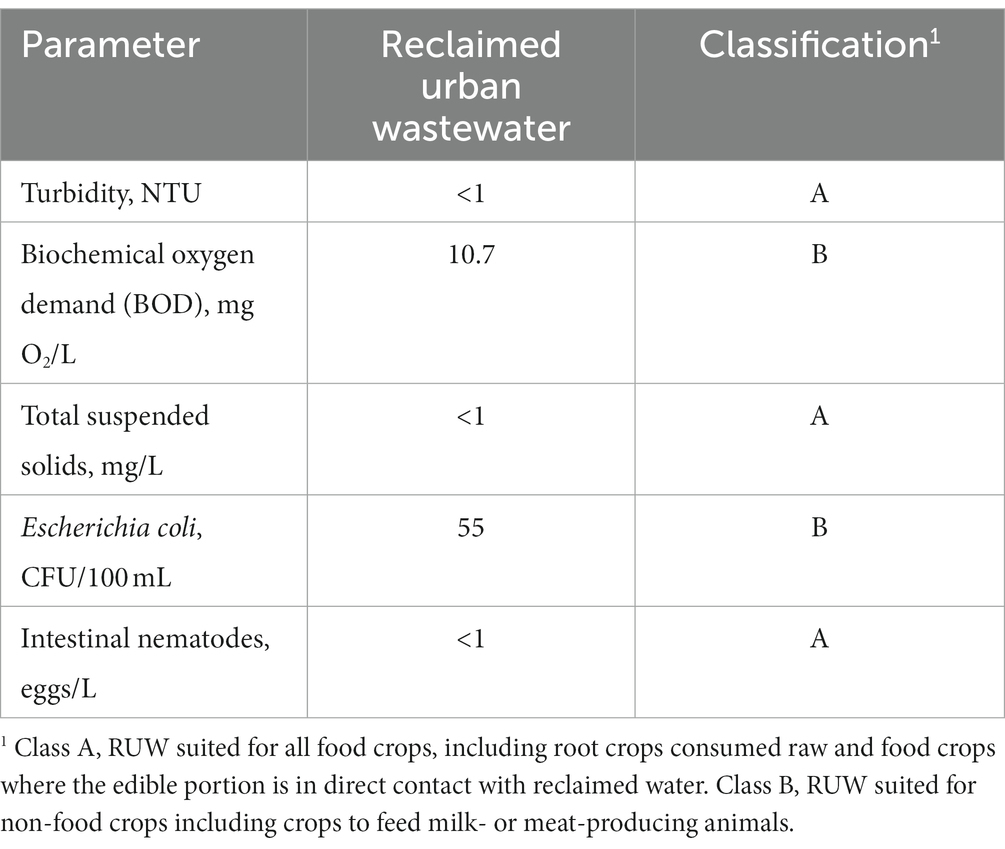
Table 1. Characteristics of the reclaimed urban wastewater used in the study in relation to the EU requirements for irrigation use (regulation 2020/741).
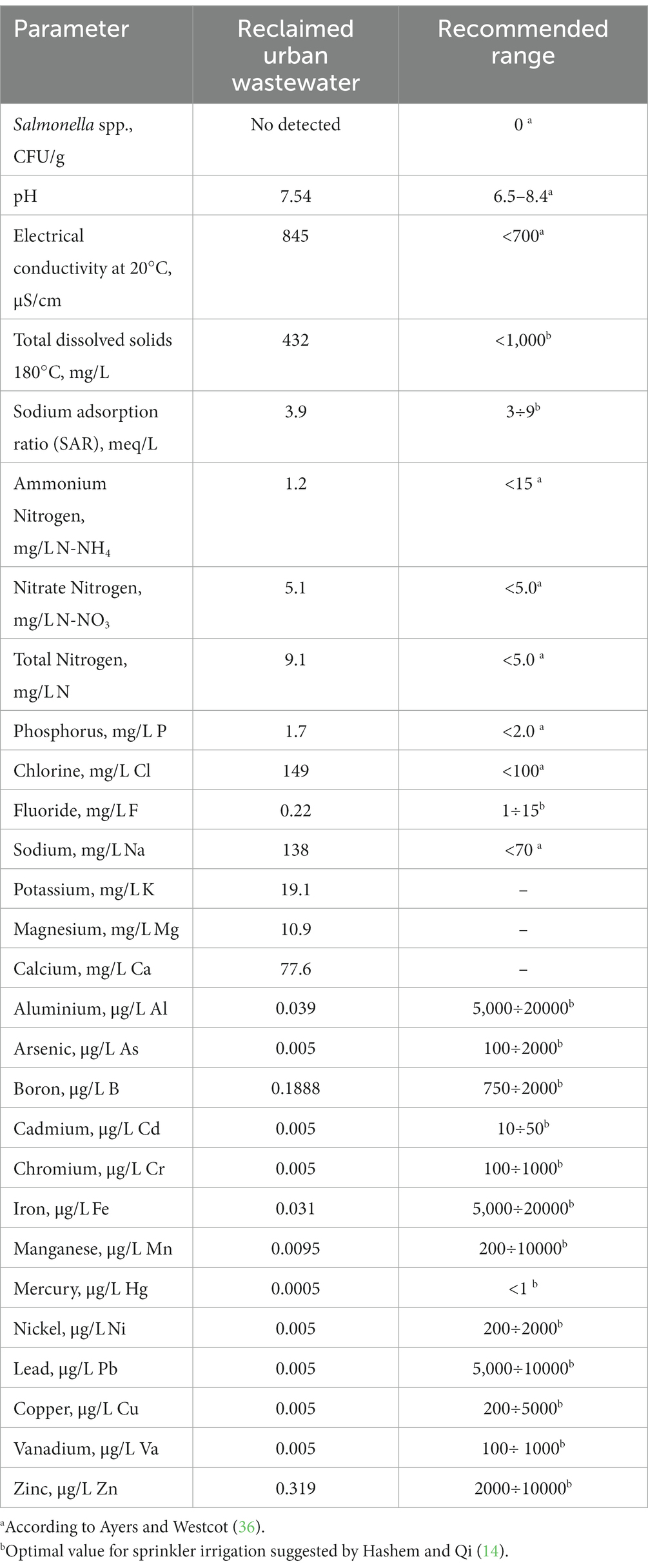
Table 2. Salmonella load and chemical and physical characteristics of the reclaimed urban wastewater used and recommended range for irrigation use.
The RUW was classified in class A for turbidity, total suspended solids, and presence of nematode eggs, whereas the BOD value and E. coli load led to the wastewater being classified as Class B (17). No Salmonella spp. was not detected. Levels above the recommended range but far from dangerous levels were detected for electrical conductibility, NO−3, total N, Na and Cl. The levels of metals were below or just close to detection limits. Table 3 shows the contaminant levels of HBF produced with RUW compared to the reference sample produced with well water.
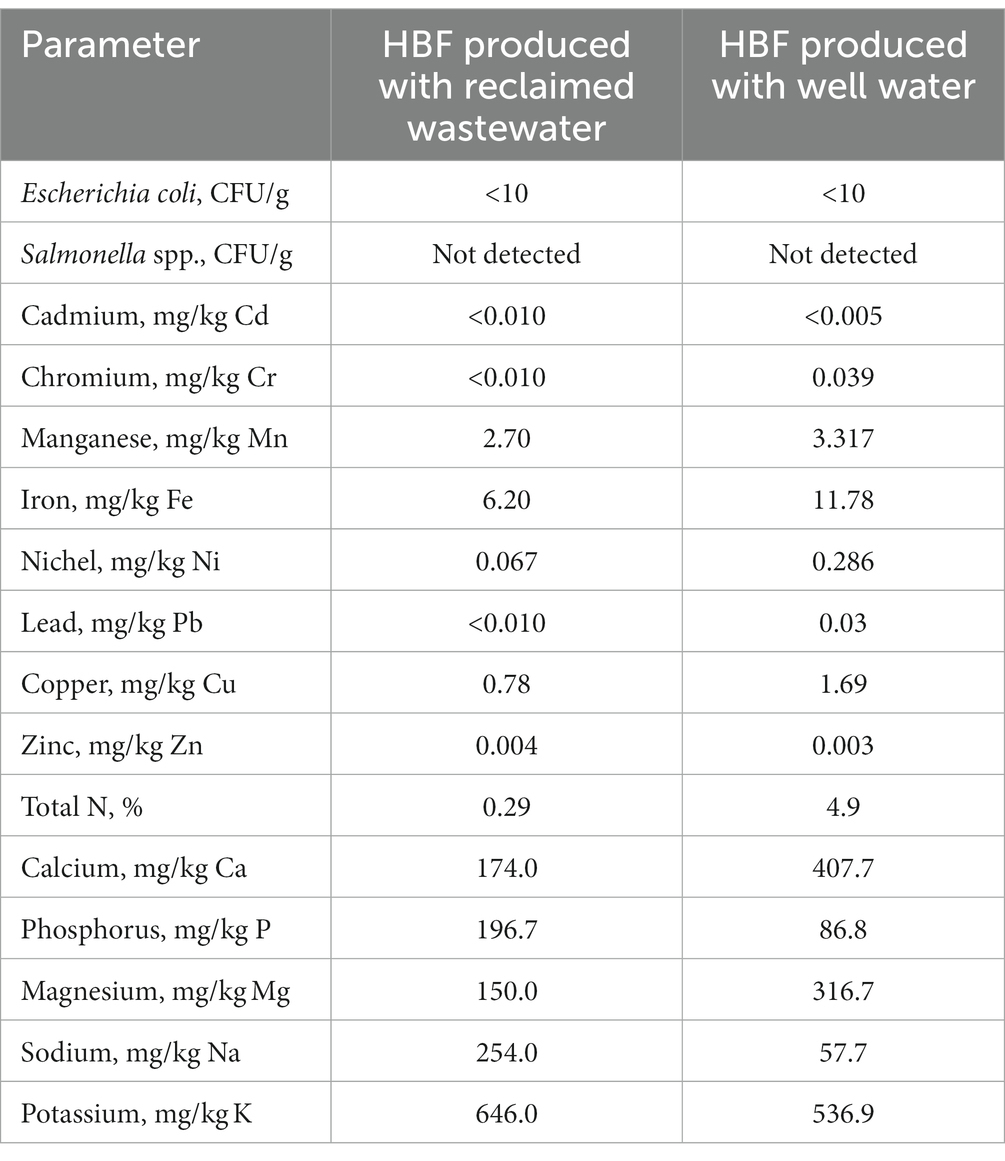
Table 3. Load of Escherichia coli and Salmonella spp. and content of metals and non-metals of hydroponic barley forage (HBF) produced with reclaimed wastewater and with well water.
No Salmonella or moulds were detected in both forages and the E. coli load remained below 10 CFU/g. The forage produced with RUW had almost five times the Na content and twice the P content of the reference sample, while the latter had a very high N content. There was no effect of water type on forage height (12.35 vs 12.38 cm for RUW and well water respectively, SEM 0.41, p = 0.94) and yield (545 vs 519 kg as fed, SEM 21.5, p = 0.41). The nutritional characteristics of HBF and TMR are shown in Tables 4, 5.
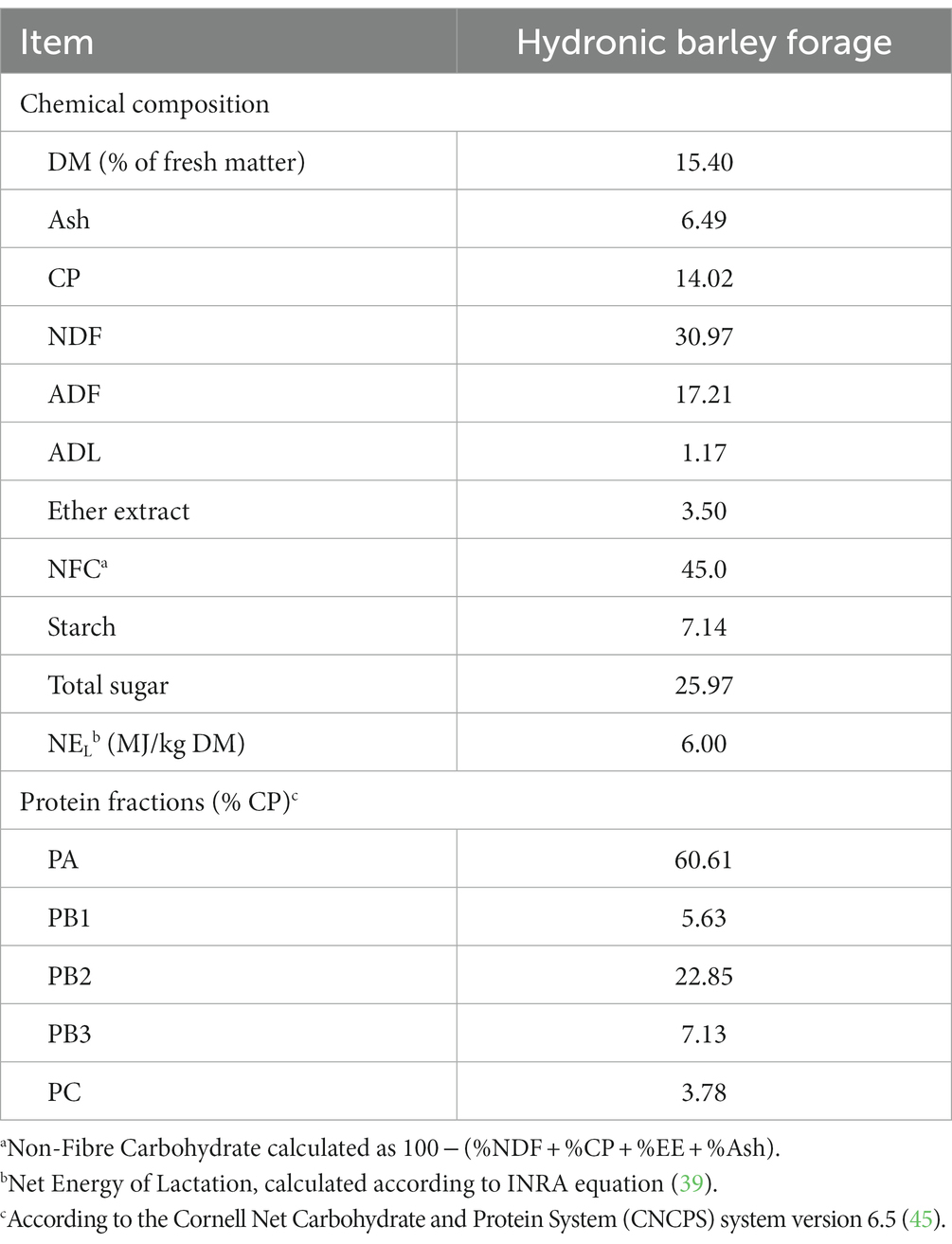
Table 4. Nutritional characteristics (% of dry matter unless stated) of hydronic barley forage used in the feeding trial.
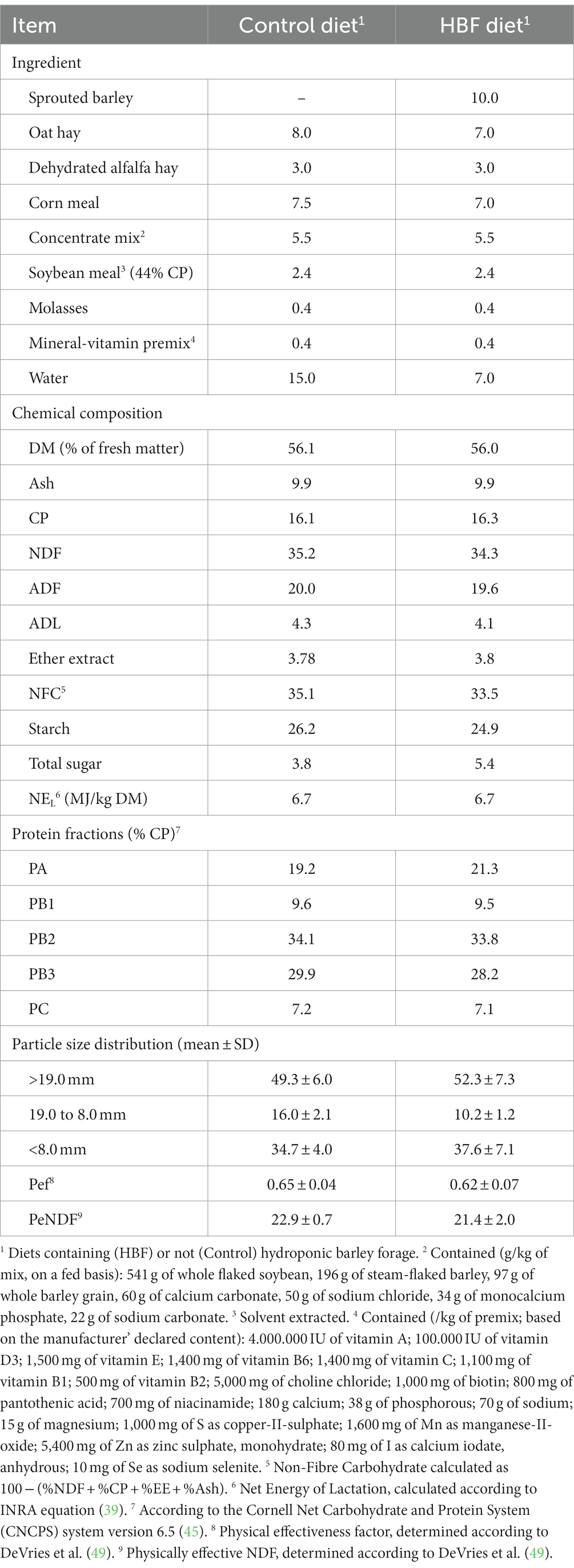
Table 5. Ingredients (kg as-fed), chemical composition (% of dry matter unless stated), and particle size distribution (% of dry matter retained of the sieve) of the diets fed to lactating cows.
The HBF was characterized by a significant amount of NFC and soluble sugars, a moderate CP content, consisting mainly of non-protein nitrogen and highly soluble protein, and a very low ADF and lignin content. However, the composition of the Control and HBF TMR was very similar.
3.2 Clinical, hematological, and biochemical findings
No cows showed clinical signs of active disease during the study. No significant differences were observed between the groups in terms of final BW (660.0 vs 661.7 kg for CG and HBFG, respectively, SEM 12.9, p = 0.91) and BCS (3.1 vs 3.2, SEM 0.04, p = 0.32). BHB and CBC values remained within the reference range and were not affected by the dietary treatment (Supplementary Table S4), as well as most of the biochemical findings (Table 6).
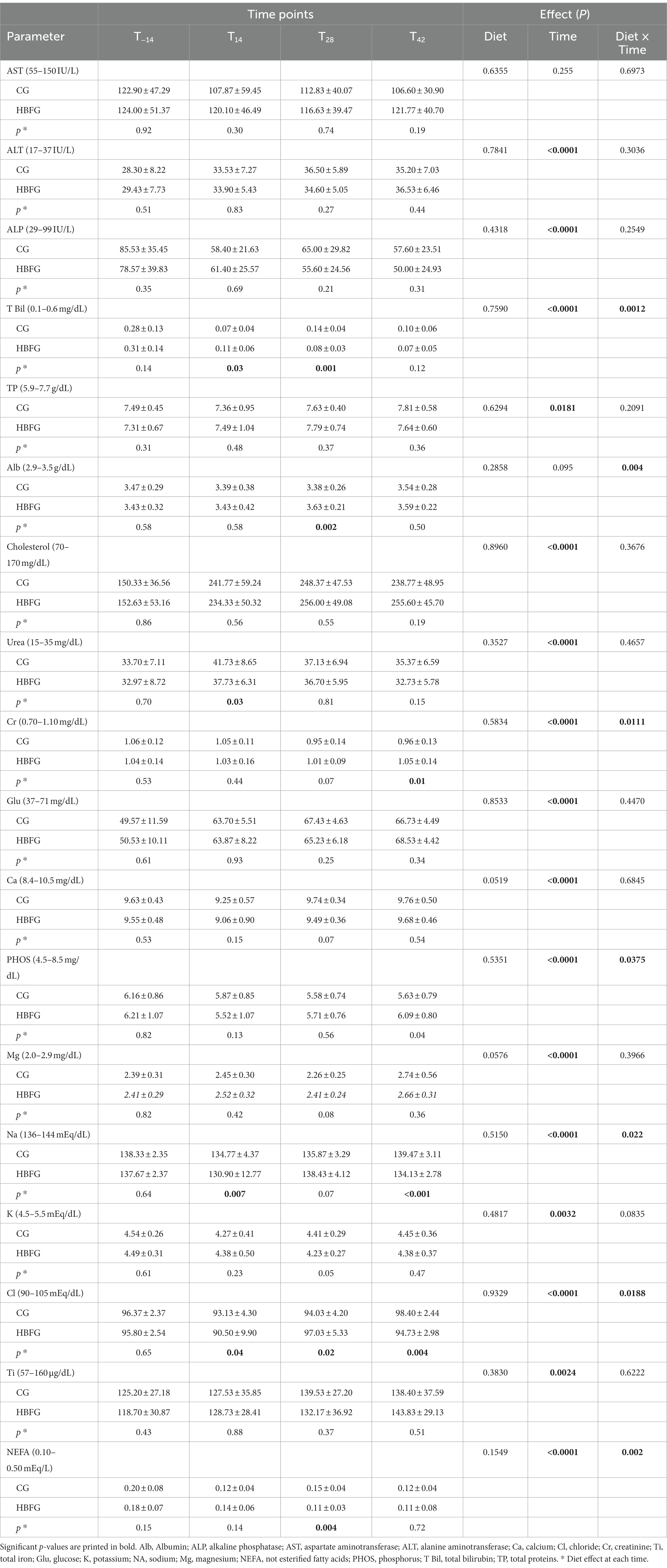
Table 6. Biochemical blood panel (Mean ± SD) of lactating cows fed diets containing (HBFG) or not (CG) hydroponic barley forage on days T-14, T14, T28, and T42.
The only exceptions were urea, phosphorus and cholesterol, whose levels were slightly outside the optimal reference range in both CG and HBFG (Table 6). Moreover, against similar initial values (T-14), Cl and Na levels showed an erratic trend, being higher in CG at T14 (p = 0.04 and p = 0.007, for Cl and Na, respectively) and at T42 (p = 0.004 and p < 0.001), and in HBFG at T28 (p = 0.02 and p = 0.07). Finally, the significance of the iterations for T Bil, Alb, Cr, PHOS and NEFA seems to be mainly due to the erratic differences, sometimes only numerical, between the two groups.
3.3 Dry matter intake, feed sorting, ruminal pH findings
Dry matter intake tended to be higher (p = 0.07) in HBFG group (22.1 vs 23.4 kg/head/d SEM 0.34 for CG vs. HBFG), whereas no differences were observed for diets particle size sorting (Supplementary Table S5). The daily mean pH, the percentage of animals with at least one SARA risk alarm and the number and time of SARA risk alarms detected by the ruminal pH sensor are shown in Table 7.
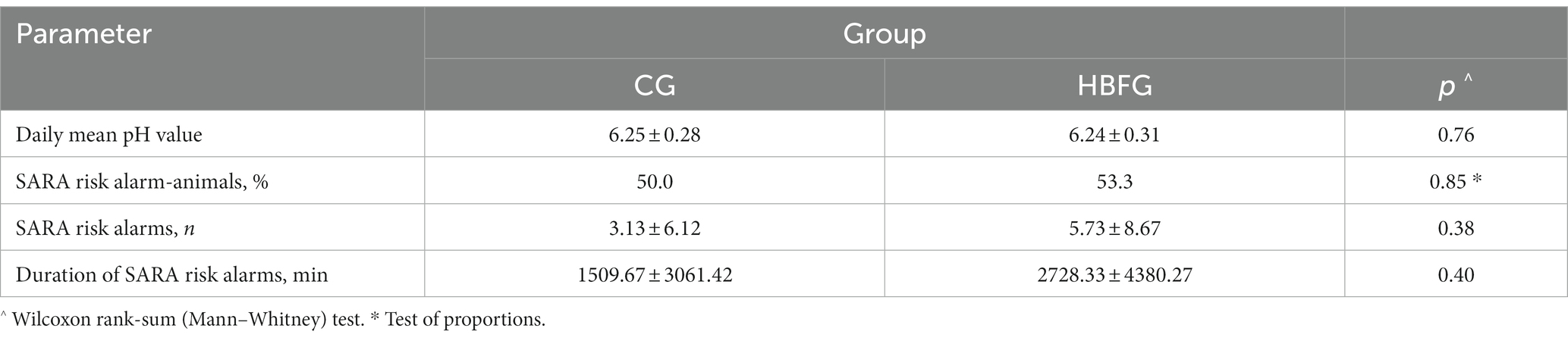
Table 7. Rumen pH (mean ± SD), percentage of animals that presented at least one subacute rumen acidosis (SARA) risk alarm, number and time (min) of SARA risk alarms detected during the experimental period by ruminal pH sensors in lactating cows fed diets containing (HBFG) or not (CG) hydroponic barley forage.
On average, approximately half of the cows (i.e., 16 vs 15 cows for CG and HBFG) in both groups had at least one SARA risk detected. The number and duration of SARA alerts were numerically higher for HBFG, but the differences were far from statistical significance.
3.4 Milk production and quality
The milk yield and milk macro components of the GC and HBFG groups are shown in Table 8. The inclusion of HBF from RUW had no effect on the actual milk yield (kg/d) or the percentage of fat, protein, non-fat solid and total solid. Consequently, ECM, fat, and protein production (kg/d) did not differ between the two groups, even though protein production was significantly higher at T1, so that a diet × time interaction was observed (data not shown). Similarly, the higher lactose content at T7 and T42 in HBFG explains the significance of the interaction.
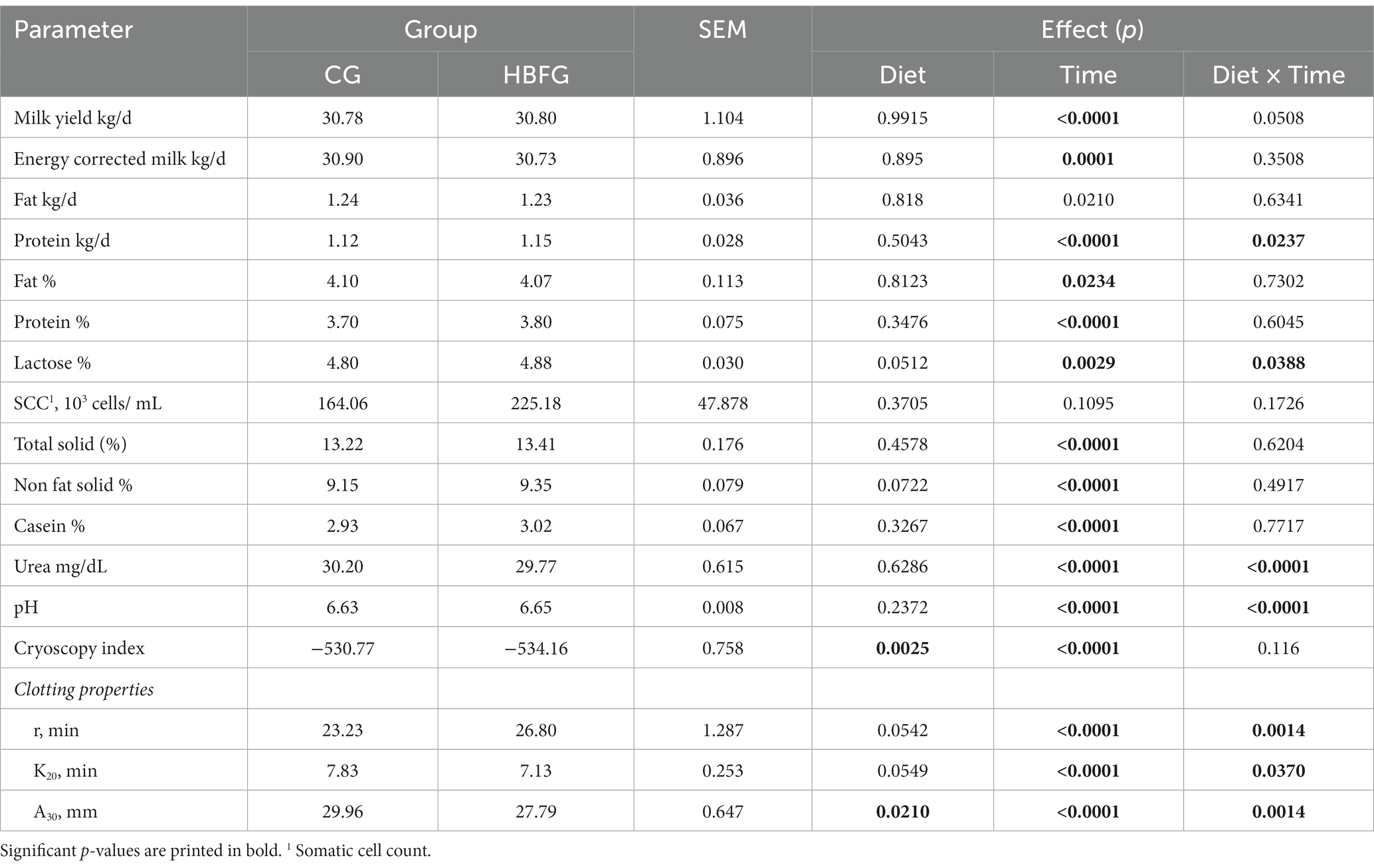
Table 8. Milk production (LSM) of cows fed diets containing (HBFG) or not (CG) hydroponic barley forage.
Regarding milk constituents and parameters related to technological and hygienic properties, no effects of diet were observed for SCC and casein. On the other hand, the values of cryoscopic index and rennet coagulation time (r) were higher (p = 0.003) and lower (p = 0.04), respectively, in HBFG than in CG. In addition, differences bordering on statistical significance were also observed for curd firming time (K20) (p = 0.06) and curd firmness (A30) (p = 0.04), which were higher and lower, respectively, in CG. Analogous to protein production and lactose content, the significance of the diet × time iterations found for urea, pH and coagulation properties reflect their irregular trend over the observation period (data not shown). Finally, no significant differences were found between the groups for the FA composition of the milk fat (Supplementary Table S6). No heavy metals were detected in the milk.
3.5 Digestibility, nitrogen balance, urine purine derivatives, microbial N supply
No differences between the groups were observed for in vivo digestibility (Supplementary Table S7). Table 9 shows the N metabolism parameters.
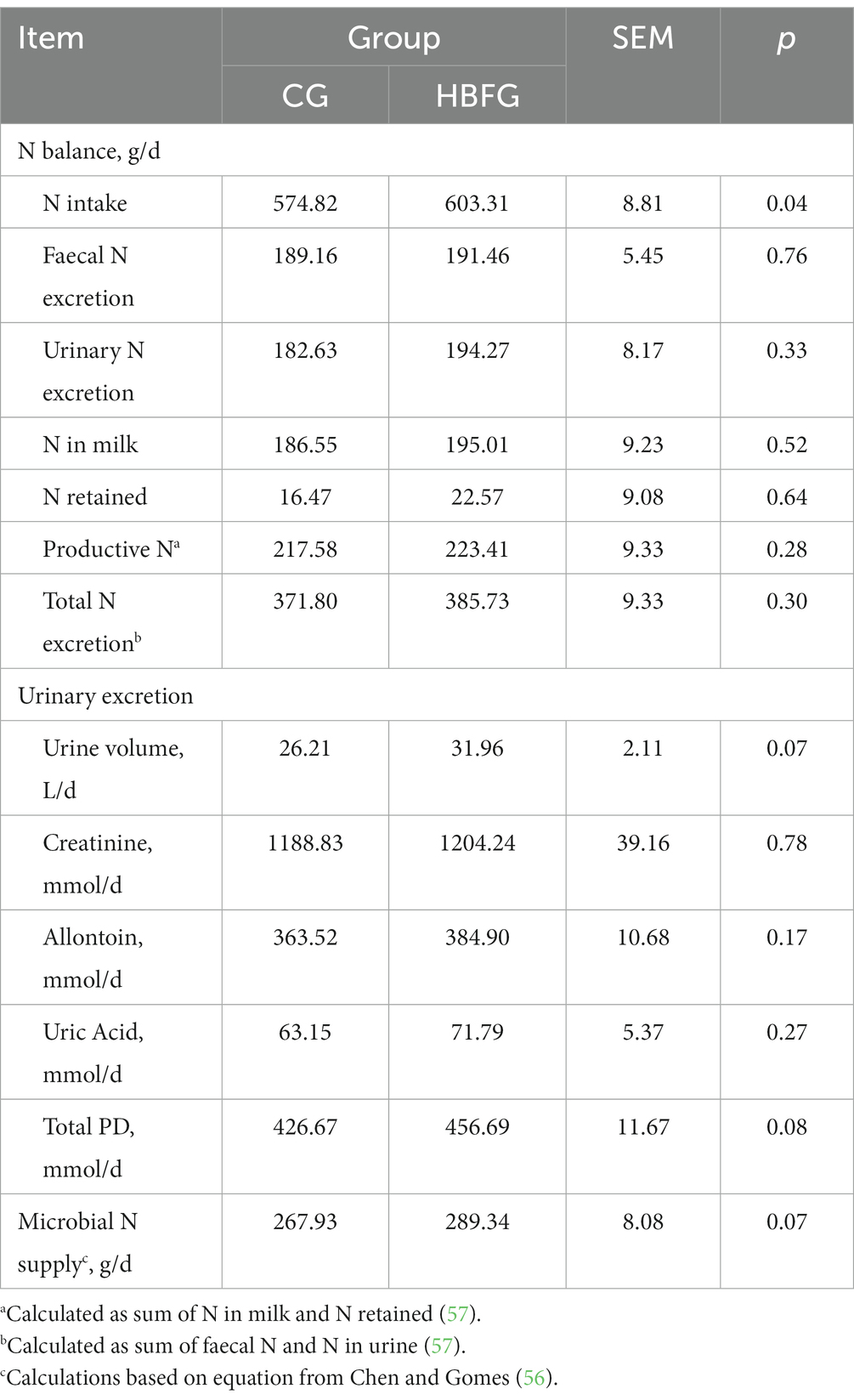
Table 9. Nitrogen balance, urinary excretion of creatinine and purine derivatives (PD), and microbial N supply (LSM) of lactating cows fed diets containing (HBFG) or not (CG) hydroponic barley forage.
The HBF-fed group had a higher (p = 0.04) N intake (+ 39 g/d) due to the higher DMI (+ 1.3 kg/d), together with a higher estimated urine volume (p = 0.06). In contrast, the distribution of N in milk, urine and faeces and the urinary excretion of allantoin and uric acid were not affected by the dietary treatments, although a trend towards an increase in estimated MN supply (p = 0.07) was observed in the HBFG group.
4 Discussion
4.1 Reclaimed urban wastewater and HBF yield and quality
The great interest in the use of reclaimed wastewater for agriculture purposes mainly concerns arid and semi-arid regions, but increasing water consumption for irrigation has led to technological innovations and regulatory frameworks for its safe use also in the EU. The criteria set out in Regulation 2020/741 (17) focus on the physico-chemical (e.g., oxygen demand, total suspended solids, turbidity) and microbiological (e.g., Salmonella and E. coli load) characteristics of the effluent. According to this legislative framework, the RUW used in the present study to produce HBF falls under ‘class B’ and can be used for non-food crops, including those intended for the feeding of dairy or meat livestock. Other irrigation water quality criteria related to human and livestock safety, crop growth, soil protection and irrigation methods (e.g., toxicant levels, pH, electrical conductivity, and SAR) indicated an overall good suitability of RUW (14, 36). There is no regulatory framework for the use of RUW both for HF production and, in general, for hydroponic systems, and the literature on this specific topic is scant and hardly addresses the issue of water quality. Connecting hydroponic systems to urban wastewater treatment plants can provide both a continuous supply of RUW throughout the year and nutrients to the plants, especially nitrogen and phosphorus, which as the main plant nutrients do not pose a risk per se in irrigation water (18). However, in contrast to the results of others (32, 34), who observed an improvement in fodder production, the yields of HBF herein described were not different when using RUW or well water. Thus, on the one hand, the use of RUW did not adversely affect the germination and growth processes, as may be the case when high levels of contaminants (i.e., heavy metals and toxic substances and organic pollutants) remain in the effluent (58, 59). On the other hand, the lack of improvement in yield indicates that the extremely short production cycle of HBF did not allow the nutrient supply of the RUW to be exploited. Hypothetically, the high nitrogen content of the HBF from the well water (Table 3) could be indicative of nitrate pollution of the groundwater, which could have masked the differences between the HBF yields. However, it is important to note that photosynthetic activity does not begin until five days after the first root appears, and only then is N required for plant growth (60).
The chemical composition of HBF differs from that of conventionally produced forage due to the very short growth cycle, which does not allow the deposition of cellulose and lignin on the cell wall, and for the presence of not only of the epigeal part (leaves and stems) but also of ungerminated or germinated residue seeds and young roots. These characteristics explain the lower content of lignin and cellulose, the better digestibility of NDF, and the higher content of soluble proteins and sugars compared to conventional forages (61, 62). As a feed, HBF could be compared to maize silage or mash, with the major difference, as already mentioned, that it is a fresh feed. Our results on the composition of HBF are largely in agreement with literature reports (31, 35, 63–66) further confirming that RUW did not interfere with normal seed germination and growth processes during HBF production.
4.2 Cow health
The use of RUW to produce HBF can pose health risks to lactating cows, mainly due to the potential presence of microbiological agents. Indeed, hydroponic forage production implies that water comes into contact with the forage, potentially carrying harmful microorganisms. As mentioned above, the EU Regulation aims to significantly reduce this risk by setting strict limits for Salmonella and E. coli loads, i.e., the main microbial species used for certificate water safety (67). The RUW disinfection with chlorine dioxide used in the present study and as it is commonly used in hydroponic systems to prevent mould growth in a high RH environment (68), provided a further microbiological risk reduction. Indeed, the HBF produced with RUW had no Salmonella spp., E. coli levels within safe limits [i.e., < 10 CFU (13)], and, considering that the mould index was close to zero during the trial, no relevant mould growth was detected. Urban effluent can contain levels of heavy metals that are hazardous to animal and human health (5, 13). However, these contaminants were at very low or near zero levels in the RUW used and were not detected in the HBF or in the milk samples collected at the end of the trial, i.e., after 7 weeks of daily feeding with the RUW-produced HBF. Compared to the existing literature, these results are of particular interest, because the use of RUW in traditional forage production systems can leads to feed contamination, exposing animals to an unavoidable microbiological risk (67, 69–72) and a dangerous increase in heavy metal intake (73–76).
The HBF-fed cows were in good health and no clinical manifestations of mastitis, laminitis, or other diseases or health and metabolic disorders occurred during the study. In addition to the absence of clinical signs of ongoing disease, indirect but crucial indicators of the health status of the cows such as CBC and key biochemical findings did not show significant variations (77, 78), being within the reference range for healthy cows and not differing substantially between the CG and HBFG groups. The very high Na and Cl contents in both RUW and HBF (Tables 2, 3) may have influenced their irregular serum levels (Table 6) through ionic excretion phenomena typical of the renal tubules (79), although a definitive explanation will require future investigation. Overall, these results support the hypotheses that the use of RUW for HBF production under the conditions tested can be considered safe for the health status of lactating cows. The effects of wastewater-derived feeds on animal metabolism and health have been investigated in a limited number of studies (5, 80). Therefore, this study can be considered one of the first to address the need for a more sustainable use of water in livestock production by recycling this finite and limiting resource. As for the study by Terrè et al. (5), they found that the use of RUW for drinking and preparing milk replacer had no short-term effects on the health and performance of young calves. In contrast, Al-Qudah et al. (80) found symptoms of nitrate poisoning in dairy herds fed fresh grass irrigated with treated municipal water.
4.3 Milk performances, dry matter intake, in vivo digestibility, and nitrogen balance
The inclusion of RUW-produced HBF in the diet had no significant effect on most of the physiological parameters and production traits measured during the feeding trial. A conservative level of inclusion was used, but it was in line with most studies evaluating the effect of hydroponic forage on milk yield and quality (35, 81–86). The lack of differences between the CG and the HBFG in terms of DMI and feed sorting, as well as in vivo digestibility and rumen pH, confirms both the palatability of the HBF and the regularity of the digestive process. The scientific literature and our results agree that HBF is a rich source of vitamins, minerals, bioactive enzymes, soluble sugars, and soluble nitrogen (87, 88). However, in agreement with our results, other work has found no effect of HBF on milk yield or quality (35, 82, 83, 89, 90). These contrasting results could easily be due to the different levels of HF inclusion and/or the different diets used. In particular, the improvement in milk yield seems to be related to the amount of HF included in the ration (30). The lack of significant differences in milk quality is also reported by most previous studies (35, 89, 90). Worth noting, the lack of significant differences for SCC is a non-trivial result, as feeding unhealthy, mouldy, contaminated, etc. feed can lead to increased SCC in milk even in the absence of clinical signs of disease (91, 92). The worsening trend of clotting aptitude in HBFG milk, probably driven by the higher cryoscopic index, is not confirmed in the literature and further research is needed to clarify this finding. However, all values of the rheological parameters were well within the normal range. It is well known that fresh forage can have positive effects on the FA composition of milk fat in terms of increasing PUFA, MUFA, and CLA content (21, 25, 93). The lack of significant effects of HBF that we found is not inconsistent with the literature because, as we have shown in previous work (21), low levels of fresh forage inclusion in the diet are unlikely to significantly alter milk fatty acid composition.
The lack of differences for in vitro digestibility is in agreement with the observation of others for similar diets (94, 95). For both groups, the level of N excretion was within the range reported by Spanghero and Kowalski (57) for similar N intake, while the NB estimate was lower, probably due to the higher milk N recorded in our study or a combination of unaccounted for N, such as urinary N excretion in forms not detected by the Kjeldhal method (e.g., nitrate), volatile losses of gaseous N and ammonia, and dermal scurf (57, 96). Although the N intake was higher for HBFG, no differences between the groups were observed for N excretion, in contrast with a large body of literature reporting a close relationship between N intake and N urinary excretion (97–101). This finding could be due to a more efficient utilization of ruminal ammonia in HBFG cows resulting in lower portal ammonia uptake and consequently lower urinary N (102). The tendentially higher total PD recovered in urine together with the lack of differences for urea concentration in milk and plasma can indirectly supports this hypothesis. The higher urine production of cows fed the HBF-containing diet probably reflects the higher intake of Na, along with other minerals and trace elements, resulting in increased urine production to maintain optimal plasma osmolarity (103, 104).
5 Conclusion
Water and land use associated with livestock production could be reduced by changing feed production methods and using additional water sources, such as wastewater from urban areas. In this study we investigated the effects of using tertiary urban wastewater for hydroponic barley forage production on forage quality, lactating cow health and performance, and milk quality. Wastewater treatment using membrane bioreactor technology was found to be effective in removing bacteria and nematode eggs, while on-farm disinfection of RUW further contributed to maintaining the coliform load at the level set by EU Regulation 741/2020 for irrigation water intended for crops fed to dairy or beef cattle. RUW showed good overall quality as irrigation quality and a fair N and P content as fertilizers, which, however, did not affect the yield and quality of HBF. The characteristics of HBF reflected the quality of the RUW delivered into the growth chambers. The feeding of lactating cows with RUW-derived HBF had no major positive or negative effects on animal health and production, including milk quality, in vivo digestibility, and nitrogen balance. It follows that the use of RUW under the conditions considered appears to be safe for the health status of lactating cows and the quality of the milk obtained. Overall, the results do not indicate any major limitations to the use of tertiary wastewater to produce hydroponic barley forage. Therefore, a wider application of RUW as irrigation water in hydroponic systems seems realistic. Future studies that take into account the accurate measurement of RUW consumption, characteristics and utilization of RUW effluent from hydroponic chamber along with the evaluation of the impact of higher feeding levels of RUW-produced HBF on animal health and performance, i.e., the main limitations of this study, will be of interest to fully validate these outcomes and provide a more comprehensive indication of the potential of the two coupled technologies to improve the environmental sustainability of animal agriculture. Furthermore, even if the quality of RUW is certified by the treatment plant, its use still requires on-farm monitoring of crops and the health status of lactating cows, as well as the adoption of appropriate hygiene practices.
Finally, it should be stressed that the economic aspect of HBF production cannot be considered “negligible” in the decision to use this type of cultivation, although it does support the objectives of this paper. Despite the possibility of reusing a water resource such as wastewater, hydroponic cultivation required significant economic investment and variable costs, mainly related to the purchase of growth chambers and barley seeds, which could affect the economic sustainability of this type of production. Further technological and design studies are needed to develop growth chambers that increase productivity and reduce costs.
Data availability statement
The raw data supporting the conclusions of this article will be made available by the authors, without undue reservation.
Ethics statement
The animal studies were approved by the Institutional Review Board of the Italian Ministry of Health (protocol code 74371-X/10, no. 1,031/2020-PR, Date of approval 19, November 2021). The studies were conducted in accordance with the local legislation and institutional requirements. Written informed consent was obtained from the owners for the participation of their animals in this study.
Author contributions
LC: Writing – original draft, Conceptualization, Funding acquisition. MC: Writing – original draft, Data curation, Investigation. FS: Data curation, Writing – original draft, Writing – review & editing. AF: Conceptualization, Investigation, Data curation, Writing – review & editing, Formal analysis, Supervision. FM: Writing – original draft, Writing – review & editing. GC: Funding acquisition, Project administration, Conceptualization, Writing – review & editing.
Funding
The author(s) declare financial support was received for the research, authorship, and/or publication of this article. This work was funded by the Apulian region under the Operational Programme FE5R 2014/2020-Azione 6.4-Sub-Az 6.4.a.DGR 2321/2017 with the project title “Utilizzo delle acque reflue affinate per la produzione di foraggio idroponico per l’alimentazione del bestiame” (Acronym Hydrofodderpuglia).
Conflict of interest
The authors declare that the research was conducted in the absence of any commercial or financial relationships that could be construed as a potential conflict of interest.
Publisher’s note
All claims expressed in this article are solely those of the authors and do not necessarily represent those of their affiliated organizations, or those of the publisher, the editors and the reviewers. Any product that may be evaluated in this article, or claim that may be made by its manufacturer, is not guaranteed or endorsed by the publisher.
Supplementary material
The Supplementary material for this article can be found online at: https://www.frontiersin.org/articles/10.3389/fvets.2023.1274466/full#supplementary-material
References
1. Feyen, L, Ciscar Martinez, JC, Gosling, S, Ibarreta Ruiz, D, Soria Ramirez, A, Dosio, A, et al. Climate change impacts and adaptation in Europe. JRC PESETA IV final report. Report no.: JRC119178. Amsterdam, Netherlands. Available at: https://econpapers.repec.org/paper/iptiptwpa/jrc119178.htm (Accessed June 15, 2023).
2. Mancuso, G, Lavrnić, S, and Toscano, A. Chapter three – reclaimed water to face agricultural water scarcity in the Mediterranean area: an overview using sustainable development goals preliminary data In: P Verlicchi, editor. Advances in chemical pollution, environmental management and protection. Wastewater treatment and reuse – Present and future perspectives in technological developments and management issues : Elsevier (2020). 113–43.
3. Belhassan, K. Water scarcity management In: A Vaseashta and C Maftei, editors. Water safety, security and sustainability: Threat detection and mitigation. Advanced sciences and Technologies for Security Applications. Cham: Springer International Publishing (2021). 443–62. doi: 10.1007/978-3-030-76008-3_19
4. Crovella, T, Paiano, A, and Lagioia, G. A meso-level water use assessment in the Mediterranean agriculture. Multiple applications of water footprint for some traditional crops. J Clean Prod. (2022) 330:129886. doi: 10.1016/j.jclepro.2021.129886
5. Terré, M, Bosch, C, Bach, A, Fàbregas, F, Biel, C, Rusiñol, M, et al. Exploring the use of tertiary reclaimed water in dairy cattle production. J Clean Prod. (2019) 229:964–73. doi: 10.1016/j.jclepro.2019.04.391
6. Mehmeti, A, and Canaj, K. Environmental assessment of wastewater treatment and reuse for irrigation: a Mini-review of LCA studies. Resources. (2022) 11:94. doi: 10.3390/resources11100094
7. Rao, AP, Patel, J, and Pradhan, AK. Application of alternative sources of water in agricultural food production — current trends and future prospects. Curr Opin Food Sci. (2022) 47:100877. doi: 10.1016/j.cofs.2022.100877
8. Gatta, G, Gagliardi, A, Disciglio, G, Lonigro, A, Francavilla, M, Tarantino, E, et al. Irrigation with treated municipal wastewater on artichoke crop: assessment of soil and yield heavy metal content and human risk. Water. (2018) 10:255. doi: 10.3390/w10030255
9. Libutti, A, Gatta, G, Gagliardi, A, Vergine, P, Pollice, A, Beneduce, L, et al. Agro-industrial wastewater reuse for irrigation of a vegetable crop succession under Mediterranean conditions. Agric Water Manag. (2018) 196:1–14. doi: 10.1016/j.agwat.2017.10.015
10. Pedrero, F, Camposeo, S, Pace, B, Cefola, M, and Vivaldi, GA. Use of reclaimed wastewater on fruit quality of nectarine in southern Italy. Agric Water Manag. (2018) 203:186–92. doi: 10.1016/j.agwat.2018.01.029
11. Canaj, K, Mehmeti, A, and Berbel, J. The economics of fruit and vegetable production irrigated with reclaimed water incorporating the hidden costs of life cycle environmental impacts. Resources. (2021) 10:90. doi: 10.3390/resources10090090
12. Ran, Y, Lannerstad, M, Herrero, M, Van Middelaar, CE, and De Boer, IJM. Assessing water resource use in livestock production: a review of methods. Livest Sci. (2016) 187:68–79. doi: 10.1016/j.livsci.2016.02.012
13. Lahlou, F-Z, Mackey, HR, and Al-Ansari, T. Wastewater reuse for livestock feed irrigation as a sustainable practice: a socio-environmental-economic review. J Clean Prod. (2021) 294:126331. doi: 10.1016/j.jclepro.2021.126331
14. Hashem, MS, and Qi, X. Treated wastewater irrigation—a review. Water. (2021) 13:1527. doi: 10.3390/w13111527
15. Khan, SAR, Ponce, P, Yu, Z, Golpîra, H, and Mathew, M. Environmental technology and wastewater treatment: strategies to achieve environmental sustainability. Chemosphere. (2022) 286:131532. doi: 10.1016/j.chemosphere.2021.131532
16. Chojnacka, K, Witek-Krowiak, A, Moustakas, K, Skrzypczak, D, Mikula, K, and Loizidou, M. A transition from conventional irrigation to fertigation with reclaimed wastewater: prospects and challenges. Renew Sust Energ Rev. (2020) 130:109959. doi: 10.1016/j.rser.2020.109959
17. European Parliament. Regulation (EU) 2020/741 of the European Parliament and of the council of 25 may 2020 on minimum requirements for water reuse. Off J Eur Union. (2020) 177:32–55.
18. Magwaza, LS, Odindo, AO, and Mditshwa, A. Hydroponic technology as decentralised system for domestic wastewater treatment and vegetable production in urban agriculture: a review. Sci Total Environ. (2020) 698:134154. doi: 10.1016/j.scitotenv.2019.134154
19. Velazquez-Gonzalez, RS, Garcia-Garcia, AL, Ventura-Zapata, E, Barceinas-Sanchez, JDO, and Sosa-Savedra, JC. A review on hydroponics and the technologies associated for medium- and small-scale operations. Agriculture. (2022) 12:646. doi: 10.3390/agriculture12050646
20. Pomoni, DI, Koukou, MK, Vrachopoulos, MG, and Vasiliadis, L. A review of hydroponics and conventional agriculture based on energy and water consumption, environmental impact, and land use. Energies. (2023) 16:1690. doi: 10.3390/en16041690
21. Uzun, P, Masucci, F, Serrapica, F, Napolitano, F, Braghieri, A, Romano, R, et al. The inclusion of fresh forage in the lactating buffalo diet affects fatty acid and sensory profile of mozzarella cheese. J Dairy Sci. (2018) 101:6752–61. doi: 10.3168/jds.2018-14710
22. Salzano, A, Neglia, G, D’Onofrio, N, Balestrieri, ML, Limone, A, Cotticelli, A, et al. Green feed increases antioxidant and antineoplastic activity of buffalo milk: a globally significant livestock. Food Chem. (2021) 344:128669. doi: 10.1016/j.foodchem.2020.128669
23. Neglia, G, Cotticelli, A, Vassetti, A, Matera, R, Staropoli, A, Vinale, F, et al. Buffalo milk and rumen fluid metabolome are significantly affected by green feed. Sci Rep. (2023) 13:1381. doi: 10.1038/s41598-022-25491-w
24. Gislon, G, Ferrero, F, Bava, L, Borreani, G, Prà, AD, Pacchioli, MT, et al. Forage systems and sustainability of milk production: feed efficiency, environmental impacts and soil carbon stocks. J Clean Prod. (2020) 260:121012. doi: 10.1016/j.jclepro.2020.121012
25. Serrapica, F, Masucci, F, Di Francia, A, Napolitano, F, Braghieri, A, Esposito, G, et al. Seasonal variation of chemical composition, fatty acid profile, and sensory properties of a mountain pecorino cheese. Foods. (2020) 9:1091. doi: 10.3390/foods9081091
26. Dogrusoz, MC. Can plant derived smoke solutions support the plant growth and forage quality in the hydroponic system? Int J Environ Sci Technol. (2022) 19:299–306. doi: 10.1007/s13762-021-03143-2
27. Ghasemi-Mobtaker, H, Sharifi, M, Taherzadeh-Shalmaei, N, and Afrasiabi, S. A new method for green forage production: energy use efficiency and environmental sustainability. J Clean Prod. (2022) 363:132562. doi: 10.1016/j.jclepro.2022.132562
28. Elmulthum, NA, Zeineldin, FI, Al-Khateeb, SA, Al-Barrak, KM, Mohammed, TA, Sattar, MN, et al. Water use efficiency and economic evaluation of the hydroponic versus conventional cultivation Systems for Green Fodder Production in Saudi Arabia. Sustainability. (2023) 15:822. doi: 10.3390/su15010822
29. Newell, R, Newman, L, Dickson, M, Vanderkooi, B, Fernback, T, and White, C. Hydroponic fodder and greenhouse gas emissions: a potential avenue for climate mitigation strategy and policy development. Facets. (2021) 6:334–57. doi: 10.1139/facets-2020-0066
30. Ahamed, MS, Sultan, M, Shamshiri, RR, Rahman, MM, Aleem, M, and Balasundram, SK. Present status and challenges of fodder production in controlled environments: a review. Smart Agric Technol. (2023) 3:100080. doi: 10.1016/j.atech.2022.100080
31. Al-Ajmi, A, Salih, AA, Kadim, I, and Othman, Y. Yield and water use efficiency of barley fodder produced under hydroponic system in GCC countries using tertiary treated sewage effluents. J Phytology. (2009) 1:342–8.
32. Al-Karaki, GN. Utilization of treated sewage wastewater for green forage production in a hydroponic system. Emirates J Food Agric. (2011) 23:80–94. doi: 10.9755/ejfa.v23i1.5315
33. Bamikole, AA, Sunday, OO, Tunde, AG, Yemisi, AR, and Alaba, JO. Water use efficiency and fodder yield of maize (Zea mays) and wheat (Triticum aestivum) under hydroponic condition as affected by sources of water and days to harvest. AJAR. (2020) 16:909–15. doi: 10.5897/AJAR2019.14503
34. Elsoury, HA, Aboukarima, AM, and Bayomi, MI. Effect of natural lighting, combination of soaking and irrigation, and seeding rate on barley green fodder production under farmer’s domestic room conditions. Misr J Agric Eng. (2015) 32:257–80. doi: 10.21608/mjae.2015.98722
35. Soder, KJ, Heins, BJ, Chester-Jones, H, Hafla, AN, and Rubano, MD. Evaluation of fodder production systems for organic dairy farms. Prof Anim Sci. (2018) 34:75–83. doi: 10.15232/pas.2017-01676
36. Ayers, RS, and Westcot, DW. Water quality for agriculture, vol. 29. Rome, Italy: Food and Agriculture Organization of the United Nations (1985).
37. Edmonson, AJ, Lean, IJ, Weaver, LD, Farver, T, and Webster, G. A body condition scoring chart for Holstein dairy cows. J Dairy Sci. (1989) 72:68–78. doi: 10.3168/jds.S0022-0302(89)79081-0
38. Zajac, AM, and Conboy, GA. eds. Fecal examination for the diagnosis of parasitism In: Veterinary clinical parasitology, 8th edn. Hoboken, New Jersey: Wiley-Blackwell (2012). 72–3.
39. Noziere, P, Sauvant, D, and Delaby, L. INRA feeding system for ruminants. Wageningen, Netherlands: Wageningen Academic Publishers (2018).
40. AOAC International. Official methods of analysis. 17th ed. Gaithersburg, MD: AOAC International (2002).
41. Van Soest, PJ, Robertson, JB, and Lewis, BA. Methods for dietary Fiber, neutral detergent Fiber, and nonstarch polysaccharides in relation to animal nutrition. J Dairy Sci. (1991) 74:3583–97. doi: 10.3168/jds.S0022-0302(91)78551-2
42. Robertson, JB, and Van Soest, PJ. The detergent system of analysis In: WPT James and O Theander, editors. The analysis of dietary fibre in food. New York, NY, USA: Marcel Dekker (1981). 123–58.
43. European Community. First commission directive 71/250/EEC of 15 (1971). Establishing community methods of analysis for the official control of feeding-stuffs. Available at: https://eur-lex.europa.eu/legal-content/EN/ALL/?uri=CELEX%3A31971L0250. (Accessed May 20, 2023).
44. Zhu, L, Jones, C, Guo, Q, Lewis, L, Stark, CR, and Alavi, S. An evaluation of total starch and starch gelatinization methodologies in pelleted animal feed1. J Anim Sci. (2016) 94:1501–7. doi: 10.2527/jas.2015-9822
45. Van Amburgh, ME, Collao-Saenz, EA, Higgs, RJ, Ross, DA, Recktenwald, EB, Raffrenato, E, et al. The Cornell net carbohydrate and protein system: updates to the model and evaluation of version 6.5. J Dairy Sci. (2015) 98:6361–80. doi: 10.3168/jds.2015-9378
46. Serrapica, F, Masucci, F, Romano, R, Santini, A, Manzo, N, Seidavi, A, et al. Peas may be a candidate crop for integrating silvoarable systems and dairy buffalo farming in southern Italy. Agrofor Syst. (2020) 94:1345–52. doi: 10.1007/s10457-018-0316-5
47. Serrapica, F, Masucci, F, Raffrenato, E, Sannino, M, Vastolo, A, Barone, CMA, et al. High Fiber cakes from Mediterranean multipurpose oilseeds as protein sources for ruminants. Animals. (2019) 9:918. doi: 10.3390/ani9110918
48. Lammers, BP, Buckmaster, DR, and Heinrichs, AJ. A simple method for the analysis of particle sizes of forage and Total mixed rations. J Dairy Sci. (1996) 79:922–8. doi: 10.3168/jds.S0022-0302(96)76442-1
49. DeVries, TJ, Beauchemin, KA, and von Keyserlingk, MAG. Dietary forage concentration affects the feed sorting behavior of lactating dairy cows. J Dairy Sci. (2007) 90:5572–9. doi: 10.3168/jds.2007-0370
50. Sacchi, R, Marrazzo, A, Masucci, F, Di Francia, A, Serrapica, F, and Genovese, A. Effects of inclusion of fresh forage in the diet for lactating buffaloes on volatile organic compounds of Milk and mozzarella cheese. Molecules. (2020) 25:1332. doi: 10.3390/molecules25061332
51. Van Keulen, J, and Young, BA. Evaluation of acid-insoluble ash as a natural marker in ruminant digestibility studies. J Anim Sci. (1977) 44:282–7. doi: 10.2527/jas1977.442282x
52. Lee, C, and Hristov, AN. Short communication: evaluation of acid-insoluble ash and indigestible neutral detergent fiber as total-tract digestibility markers in dairy cows fed corn silage-based diets. J Dairy Sci. (2013) 96:5295–9. doi: 10.3168/jds.2012-6442
53. Spanghero, M. Urinary pH and mineral excretion of cows fed four different forages supplemented with increasing levels of an anionic compound feed. Anim Feed Sci Technol. (2002) 98:153–65. doi: 10.1016/S0377-8401(02)00023-8
54. George, SK, Dipu, MT, Mehra, UR, Singh, P, Verma, AK, and Ramgaokar, JS. Improved HPLC method for the simultaneous determination of allantoin, uric acid and creatinine in cattle urine. J Chromatogr B. (2006) 832:134–7. doi: 10.1016/j.jchromb.2005.10.051
55. Valadares, RFD, Broderick, GA, Filho, SCV, and Clayton, MK. Effect of replacing alfalfa silage with high moisture corn on ruminal protein synthesis estimated from excretion of Total purine Derivatives1. J Dairy Sci. (1999) 82:2686–96. doi: 10.3168/jds.S0022-0302(99)75525-6
56. Chen, XB, and Gomes, MJ. Estimation of microbial protein supply to sheep and cattle based on urinary excretion of purine derivatives: An overview of the technical details. Rowett Research Institute: International Feed Resources Unit. Occasional publication (1992).
57. Spanghero, M, and Kowalski, ZM. Updating analysis of nitrogen balance experiments in dairy cows. J Dairy Sci. (2021) 104:7725–37. doi: 10.3168/jds.2020-19656
58. Chow, YN, Lee, LK, Zakaria, NA, and Foo, KY. Insight into the chromium-enriched industrial wastewater irrigation practice on Lablab purpureus. J Environ Eng. (2020) 146:04020077. doi: 10.1061/(ASCE)EE.1943-7870.0001742
59. Lee, E, Rout, PR, and Bae, J. The applicability of anaerobically treated domestic wastewater as a nutrient medium in hydroponic lettuce cultivation: nitrogen toxicity and health risk assessment. Sci Total Environ. (2021) 780:146482. doi: 10.1016/j.scitotenv.2021.146482
60. Taiz, L, Zeiger, E, Møller, IM, and Murphy, A. Plant physiology and development. 6th ed. Sunderland, MA, USA: Sinauer Associates Incorporated (2015).
61. Mpanza, TDE, Dhlamini, TC, Pierneef, RE, and Mbatha, KR. Enteric methane emission, rumen fermentation and microbial profiles of meat-master lambs supplemented with barley fodder sprouts. Fermentation. (2022) 8:434. doi: 10.3390/fermentation8090434
62. Alharthi, AS, Al-Baadani, HH, Abdelrahman, MM, and Alhidary, IA. Effects of feeding different levels of sprouted barley on fermentation characteristics, bacterial quantification, and rumen morphology of growing lambs. Vet Sci. (2023) 10:15. doi: 10.3390/vetsci10010015
63. Fazaeli, H, Golmohammadi, HA, Tabatabayee, SN, and Asghari-Tabrizi, M. Productivity and nutritive value of barley green fodder yield in hydroponic system. World Appl Sci J. (2012) 16:531–9.
64. Hafla, AN, Soder, KJ, Brito, AF, Rubano, MD, and Dell, CJ. Effect of sprouted barley grain supplementation of an herbage-based or haylage-based diet on ruminal fermentation and methane output in continuous culture1. J Dairy Sci. (2014) 97:7856–69. doi: 10.3168/jds.2014-8518
65. Naik, PK, Swain, BK, and Singh, NP. Production and utilisation of hydroponics fodder. Indian J Anim Nutr. (2015) 32:1–9.
66. Farghaly, MM, Abdullah, MAM, Youssef, IMI, Abdel-Rahim, IR, and Abouelezz, K. Effect of feeding hydroponic barley sprouts to sheep on feed intake, nutrient digestibility, nitrogen retention, rumen fermentation and ruminal enzymes activity. Livest Sci. (2019) 228:31–7. doi: 10.1016/j.livsci.2019.07.022
67. Bevilacqua, PD, Bastos, RKX, and Mara, DD. An evaluation of microbial health risks to livestock fed with wastewater-irrigated forage crops. Zoonoses Public Health. (2014) 61:242–9. doi: 10.1111/zph.12063
68. Puccinelli, M, Carmassi, G, Pardossi, A, and Incrocci, L. Wild edible plant species grown hydroponically with crop drainage water in a Mediterranean climate: crop yield, leaf quality, and use of water and nutrients. Agric Water Manag. (2023) 282:108275. doi: 10.1016/j.agwat.2023.108275
69. Elahi, E, Abid, M, Zhang, L, and Alugongo, GM. The use of wastewater in livestock production and its socioeconomic and welfare implications. Environ Sci Pollut Res. (2017) 24:17255–66. doi: 10.1007/s11356-017-9263-3
70. Ines, BS, Imed, M, Frikha, D, Mohamed, C, and Adele, M. Reclaimed municipal wastewater for forage production. Water Sci Technol. (2017) 75:1784–93. doi: 10.2166/wst.2017.048
71. Kranjac-Berisavljevic, SCKS. Wastewater use in west African urban agriculture: benefits and risks In: WL Filho, AM Azul, L Brandli, M Lange Salvia, and T Wall, editors. Clean water and sanitation. Encyclopedia of the UN sustainable development goals. Cham: Springer International Publishing (2022). 707–16. doi: 10.1007/978-3-319-95846-0_169
72. Ines, BS, Muscolo, A, Mezghani, I, and Chaieb, M. Treated municipal wastewater as option to the use of fresh water for the cultivation of valuable pastoral species Buffel grass (Cenchrus ciliaris L.). Water Environ J. (2023) 37:549–60. doi: 10.1111/wej.12858
73. Pompilio, CGN, Francisco, CS, Tulio, FDMTM, Samuel, SMS, and Elisa, GJF. Heavy metals in blood, milk and cow's urine reared in irrigated areas with wastewater. Heliyon. (2021) 7:e06693. doi: 10.1016/j.heliyon.2021.e06693
74. Iqbal, Z, Abbas, F, Ibrahim, M, Qureshi, TI, Gul, M, and Mahmood, A. Human health risk assessment of heavy metals in raw milk of buffalo feeding at wastewater-irrigated agricultural farms in Pakistan. Environ Sci Pollut Res. (2020) 27:29567–79. doi: 10.1007/s11356-020-09256-4
75. Castro–González NP, Calderón–Sánchez F, Pérez–Sato M, Soní–Guillermo E, Reyes–Cervantes E. Health risk due to chronic heavy metal consumption via cow’s milk produced in Puebla, Mexico, in irrigated wastewater areas. Food Addit Contam Part B Surveill. (2019) 12:38–44. doi: 10.1080/19393210.2018.1520742
76. Castro-González, NP, Calderón-Sánchez, F, Castro de Jesús, J, Moreno-Rojas, R, Tamariz-Flores, JV, Pérez-Sato, M, et al. Heavy metals in cow’s milk and cheese produced in areas irrigated with waste water in Puebla, Mexico. ood Addit Contam Part B Surveill. (2018) 11:33–6. doi: 10.1080/19393210.2017.1397060
77. Jones, ML, and Allison, RW. Evaluation of the ruminant complete blood cell count. Vet Clin N Am Food Anim Pract. (2007) 23:377–402. doi: 10.1016/j.cvfa.2007.07.002
78. Puppel, K, and Kuczyńska, B. Metabolic profiles of cow’s blood; a review. J Sci Food Agric. (2016) 96:4321–8. doi: 10.1002/jsfa.7779
79. Pickering, EC. The role of the kidney in sodium and potassium balance in the cow. Proc Nutr Soc. (1965) 24:73–80. doi: 10.1079/PNS19650013
80. Al-Qudah, KM, Rousan, LM, and Ereifej, KI. Nitrate/nitrite poisoning in dairy cattle associated with consumption of forages irrigated with municipally treated wastewater. Toxicol Environ Chem. (2009) 91:163–70. doi: 10.1080/02772240802051205
81. Naik, PK, Dhuri, RB, Karunakaran, M, Swain, BK, and Singh, NP. Effect of feeding hydroponics maize fodder on digestibility of nutrients and milk production in lactating cows. Indian J Anim Sci. (2014) 84:880–3. doi: 10.56093/ijans.v84i8.43275
82. Nugroho, HD, and Permana, IG. Utilization of bioslurry on maize hydroponic fodder as a corn silage supplement on nutrient digestibility and milk production of dairy cows. Media Peternakan. (2015) 38:70–6. doi: 10.5398/medpet.2015.38.1.70
83. Kaouche-Adjlanea, S, Bafdelc, AASM, and Benhacined, R. Techno-economic approach to hydroponic forage crops: use for feeding dairy cattle herd. J Appl Environ Biol Sci. (2016) 6:83–7.
84. Agius, A, Pastorelli, G, and Attard, E. Cows fed hydroponic fodder and conventional diet: effects on milk quality. Arch Anim Breed. (2019) 62:517–25. doi: 10.5194/aab-62-517-2019
85. Bari, MS, Islam, MN, Islam, MM, Siddiki, MSR, Habib, MR, and Islam, MA. Partial replacement of conventional concentrate mixture with hydroponic maize and its effect on milk production and quality of crossbred cow. J Bangladesh Agric Univ. (2020) 18:1–635. doi: 10.5455/JBAU.98462
86. Kolesnikov, R, Morozov, V, Matserushka, A, Talalay, G, and Kolesnokova, M. Resistance of dairy cows during the use of new production fodder. KnE Life Sci. (2021) 6:419–29. doi: 10.18502/kls.v0i0.8973
87. Peer, DJ, and Leeson, S. Nutrient content of hydroponically sprouted barley. Anim Feed Sci Technol. (1985) 13:191–202. doi: 10.1016/0377-8401(85)90022-7
88. Peer, DJ, and Leeson, S. Feeding value of hydroponically sprouted barley for poultry and pigs. Anim Feed Sci Technol. (1985) 13:183–90. doi: 10.1016/0377-8401(85)90021-5
89. Heins, B. Evaluation of fodder systems for organic dairy cattle to improve livestock efficiency. Final project report submitted to the Ceres Trust. University of Minnesota West Central Research and outreach center, Morris, MN (2017). Available at:https://cerestrust.org/evaluation-fodder-systems-organic-dairy-cattle-improve-livestock-efficiency/ (Accessed July 31, 2023).
90. Fazaeli, H, Golmohammadi, HA, and Tabatatbaei, SN. Effect of replacing dietary corn silage with hydroponic barley green fodder on Holstein dairy cows performance. Iranian J Appl Anim Sci. (2021) 11:47–57.
91. Fink-Gremmels, J. The role of mycotoxins in the health and performance of dairy cows. Vet J. (2008) 176:84–92. doi: 10.1016/j.tvjl.2007.12.034
92. Fatima, D. Fungal diseases of bovines In: A Gupta and N Pratap Singh, editors. Fungal diseases in animals: From infections to prevention. Fungal biology. Cham: Springer International Publishing (2021). 1–14. doi: 10.1007/978-3-030-69507-1_1
93. Esposito, G, Masucci, F, Napolitano, F, Braghieri, A, Romano, R, Manzo, N, et al. Fatty acid and sensory profiles of Caciocavallo cheese as affected by management system. J Dairy Sci. (2014) 97:1918–28. doi: 10.3168/jds.2013-7292
94. Cavallini, D, Raffrenato, E, Mammi, LME, Palmonari, A, Canestrari, G, Costa, A, et al. Predicting fiber digestibility in Holstein dairy cows fed dry-hay based rations through machine learning. Animal. (2023):101000. doi: 10.1016/j.animal.2023.101000
95. Cavallini, D, Palmonari, A, Mammi, LME, Ghiaccio, F, Canestrari, G, and Formigoni, A. Evaluation of fecal sampling time points to estimate apparent nutrient digestibility in lactating Holstein dairy cows. Frontiers in Veterinary Science. (2023) 9:1065258. doi: 10.3389/fvets.2022.1065258
96. Spanghero, M, and Kowalski, ZM. Critical analysis of N balance experiments with lactating cows. Livest Prod Sci. (1997) 52:113–22. doi: 10.1016/S0301-6226(97)00138-3
97. Castillo, AR, Kebreab, E, Beever, DE, and France, J. A review of efficiency of nitrogen utilisation in lactating dairy cows and its relationship with environmental pollution. J Anim Feed Sci. (2000) 9:1–32. doi: 10.22358/jafs/68025/2000
98. Kebreab, E, France, J, Beever, DE, and Castillo, AR. Nitrogen pollution by dairy cows and its mitigation by dietary manipulation. Nutr Cycl Agroecosyst. (2001) 60:275–85. doi: 10.1023/A:1012668109662
99. Arriaga, H, Pinto, M, Calsamiglia, S, and Merino, P. Nutritional and management strategies on nitrogen and phosphorus use efficiency of lactating dairy cattle on commercial farms: an environmental perspective. J Dairy Sci. (2009) 92:204–15. doi: 10.3168/jds.2008-1304
100. Powell, JM, Gourley, CJP, Rotz, CA, and Weaver, DM. Nitrogen use efficiency: a potential performance indicator and policy tool for dairy farms. Environ Sci Pol. (2010) 13:217–28. doi: 10.1016/j.envsci.2010.03.007
101. Foskolos, A, and Moorby, JM. Evaluating lifetime nitrogen use efficiency of dairy cattle: a modelling approach. PLoS One. (2018) 13:e0201638. doi: 10.1371/journal.pone.0201638
102. Swensson, C. Relationship between content of crude protein in rations for dairy cows, N in urine and ammonia release. Livest Prod Sci. (2003) 84:125–33. doi: 10.1016/j.livprodsci.2003.09.009
103. Tucker, WE, and Hogue, JF. Influence of sodium chloride or potassium chloride on systemic Acid-Base status, Milk yield, and mineral metabolism in lactating dairy Cows1. J Dairy Sci. (1990) 73:3485–93. doi: 10.3168/jds.S0022-0302(90)79048-0
Keywords: hydroponic barley forage, reclaimed urban wastewater, dairy cows, hematochemical profile, rumen pH, milk traits, digestibility, nitrogen metabolism
Citation: Ceci L, Cavalera MA, Serrapica F, Di Francia A, Masucci F and Carelli G (2023) Use of reclaimed urban wastewater for the production of hydroponic barley forage: water characteristics, feed quality and effects on health status and production of lactating cows. Front. Vet. Sci. 10:1274466. doi: 10.3389/fvets.2023.1274466
Edited by:
Fazul Nabi, Southwest University, ChinaReviewed by:
Ravikanth Reddy Poonooru, University of Missouri, United StatesMarco Tassinari, University of Bologna, Italy
Copyright © 2023 Ceci, Cavalera, Serrapica, Di Francia, Masucci and Carelli. This is an open-access article distributed under the terms of the Creative Commons Attribution License (CC BY). The use, distribution or reproduction in other forums is permitted, provided the original author(s) and the copyright owner(s) are credited and that the original publication in this journal is cited, in accordance with accepted academic practice. No use, distribution or reproduction is permitted which does not comply with these terms.
*Correspondence: Felicia Masucci, masucci@unina.it
†These authors have contributed equally to this work and share first authorship