- 1National Engineering Research Center for Breeding Swine Industry and College of Animal Science, South China Agricultural University, Guangzhou, China
- 2National Engineering Research Center for Breeding Swine Industry, Wens Foodstuff Group Co., Ltd., Yunfu, China
Duroc pigs are popular crossbred terminal sires, and accurate assessment of genetic parameters in the population can help to rationalize breeding programmes. The principle aim of this study were to evaluate the genetic parameters of production (birth weight, BW; age at 115 kg, AGE; feed conversion ratio, FCR) and body size (body length, BL; body height, BH; front cannon circumference, FCC) traits of Duroc pigs. The second objective was to analyze the fit of different genetic assessment models. The variance components and correlations of BW (28,348 records), AGE (28,335 records), FCR (11,135 records), BL (31,544 records), BH (21,862 records), and FCC (14,684 records) traits were calculated by using DMU and AIREMLF90 from BLUPF90 package. In the common environment model, the heritability of BW, AGE, FCR, BL, BH, and FCC traits were 0.17 ± 0.014, 0.30 ± 0.019, 0.28 ± 0.024, 0.16 ± 0.013, 0.14 ± 0.017, and 0.081 ± 0.016, with common litter effect values of 0.25, 0.20, 0.18, 0.23, 0.19, and 0.16, respectively. According to the results of the Akaike information criterion (AIC) calculations, models with smaller AIC values have a better fit. We found that the common environment model with litter effects as random effects for estimating genetic parameters had a better fit. In this Model, the estimated genetic correlations between AGE with BW, FCR, BL, BH, and FCC traits were −0.28 (0.040), 0.76 (0.038), −0.71 (0.036), −0.44 (0.060), and −0.60 (0.073), respectively, with phenotypic correlations of −0.17, 0.52, −0.22, −0.13 and −0.24, respectively. In our analysis of genetic trends for six traits in the Duroc population from 2012 to 2021, we observed significant genetic trends for AGE, BL, and BH. Particularly noteworthy is the rapid decline in the genetic trend for AGE, indicating an enhancement in the pig's growth rate through selective breeding. Therefore, we believe that some challenging-to-select traits can benefit from the genetic correlations between traits. By selecting easily measurable traits, they can gain from synergistic selection effects, leading to genetic progress. Conducting population genetic parameter analysis can assist us in devising breeding strategies.
1 Introduction
As one of the world's most popular commercial pig breeds, the Duroc pig has undergone intense artificial selection to improve its commercial productivity and product quality (1). In recent years, Duroc pigs have gained popularity as the terminal sire in many mixed commercial lean types due to their superior performance in growth, feed conversion efficiency and conformation, along with carcass and other beneficial characteristics (2, 3). For example, Duroc × (Landrace × Yorkshire) (DLY) commercial pigs account for the majority of the current hog production market in China. During the process of breeding selection, managers preferred pigs that consume less feed, exhibit faster growth rates, possess a more robust body shape, and produce leaner meat; thereby, increasing breeding value (4). Therefore, pig breeding strategies prioritize production rates that enhance animal growth and reduce production costs (5). In this regard, birth weight (BW), age at 115 kg (AGE), and feed conversion ratio (FCR) traits are of particular interest. Among these traits, feed efficiency, which is challenging to measure directly, is often indicated indirectly by AGE (6). Moreover, body size traits such as breeding boar body length (BL), body height (BH), and front cannon circumference (FCC) play a crucial role in body size selection, whereas BL and BH are tightly connected to growth rate and physical condition (7).
Genetic improvement is a key technology for producing high-quality breeding pigs for optimal quality and sustainable production. The most valuable approach to assess the genetic worth of animals is by estimating genetic parameters such as individual breeding values (8), as they reveal the potential genetic value of traits within an animal that can be exploited through breeding and selection (5). Improving our understanding of the genetic relationships of production, growth, and body size traits in breeding pigs is necessary for updating selection indices and complementing breeding objectives (8), in addition to helping predict relevant selection responses and avoid possible undesirable side effects (9).
In contemporary breeding programs, animal models are frequently employed to calculate breeding values and variance components (10). Fixed effects, direct additive genetic effects, maternal effects, and numerous environmental effects can all be included in animal models (11). General animal, repeatability, maternal effect model, common environmental effect (litter effect), and random regression models are examples of common animal models. Choosing the most appropriate models based on the characteristics of the trait is the key to genetic parameter estimation. If some essential aspects of the assessment model are overlooked when evaluating the model, the variance may be inflated or understated (10). Thus, a well-fitting model guarantees accurate estimation of variance components, in turn producing reliable breeding value predictions. Therefore, this study analyzed the heritability, breeding values, and genetic and phenotypic correlation of BW, AGE, FCR, BL, BH, and FCC in a Duroc pig population using general animal and common environment models, respectively, to investigate (1) the influence of common environment effects on production and body size traits and (2) the genetic relationships among the traits, and (3) assess the progress of genetic improvement in this Duroc population.
2 Material and methods
2.1 Phenotypic and pedigree data sets
Phenotypic and pedigree data were collected from a great grandparent Duroc pig farm managed by WENS Foodstuff Group Co., Ltd. Phenotypic, located in Qingyuan City, Guangdong Province, People's Republic of China. The Duroc herd consists of American Duroc pigs introduced in 2010, with a current base herd size of 1,500 pigs. Phenotypic and pedigree records were collected for the period between 2012 and 2021, comprising over 130,000 phenotypic records and over 70,000 pedigree records. The study herd is distributed across six farms, with the number of individuals in each farm ranging from 144 to 24,829 pigs. The pigs were group housed in half-open cement floor pens (10 animals in each pen, with an average of 2 m2 per pig). Three production traits (BW, AGE, and FCR from 30 to 115 kg) and three body size traits (BL, BH, and FCC) were analyzed. Piglet birth weight was measured within 24 h after birth. The feed conversion ratio from 30 to 115 kg was assessed using an automatic feeder system called the Osborne FIRE Pig Performance Testing System (Kansas, American). Measurements were taken on the test day and adjusted to 115 kg using correction formulae derived from those independently created by WENS Foodstuff Group Co., Ltd. (formula omitted). The measurements of BL, BH, and FCC were taken when the pigs reached 115 ± 5 kg. BL was measured from the base of the ears to the base of the tail, while BH was determined from the shoulders to the ground. FCC represents the circumference of the left forelimb at its narrowest point. All three of these traits were measured using tapes. Outliers exceeding three standard deviations from the mean were eliminated prior to statistical analysis.
2.2 Statistical analysis
The influence of non-genetic factors, including the fixed effects of farm (six levels), year-season (42 levels), sex (two levels), and parity (11 levels), was tested for significance (P < 0.05) using R v4.1.2 software. Only significant effects (P < 0.05) were considered in subsequent mixed model analyses. DMU v6.0 (12) and AIREMLF90 program from the BLUPF90 software package were used to variance components and genetic correlation of traits using single/multiple-trait animal model. The fitness of different models was assessed using the Akaike information criterion (AIC), which is calculated as AIC = 2k - 2logL, where k represents the number of parameters used in the model and logL is the log-likelihood value estimated by AIREMLF90. The following general animal and common environment effect models were used:
Model 1: The general animal model:
Model 2: The common environment model:
where y is a vector of observations; b is a vector of fixed effects; a is a vector of direct additive genetic effects; c is a vector of common litter effects; e is a vector of residual effects. In this study, we define piglets born from the same mother during the same period as “common litter piglets.” Individual litter effects were recorded using the individual number of their mother along with the birth year and month as components. X, Za, and Zc are the incidence matrices for effects b, a, and c, respectively. It is assumed that random effects are independent and normally distributed:
where A is the numerator relationship matrix; I is an identity matrix; , , and are the variances of random additive genetic, common litter and residual effects, respectively. Common litter effect value (c2) is the proportion of litter effect in the total variance, which was computed using the follow equation, .
The total phenotypic variance () was calculated as , and heritability (h2) was computed using the following equation, (Model 1). The total phenotypic variance () was calculated as , and heritability (h2) was computed using the equation, (Model 2).
The general animal model for two traits:
All elements are consistent with the single-trait model described above. The formulae for calculating the genetic correlation (rg) and phenotypic correlation (rp) between two traits were estimated according to the method of Alam et al. (5), with the genetic correlation calculated as , where the and parameters are genetic variance estimates of traits 1 and 2, respectively, and σa1a2 is the genetic covariance between two traits. The formula for the phenotypic correlation between two traits is denoted as , where covp1p2 represents the phenotypic covariance between the traits, and and denote the phenotypic variances of trait 1 and trait 2, respectively. In addition, we calculated breeding values for various traits of the Duroc population using the DMU v6.0 software's DMU4 module and visualized the trends in mean breeding values for all traits across birth years.
3 Results
3.1 Descriptive statistics
Table 1 presents a descriptive summary of the BW, AGE, FCR, BL, BH, and FCC traits in the Duroc population. The average values for these traits in the Duroc pigs were as follows: BW (1.66 ± 0.27 kg), AGE (185.40 ± 15.00 days), FCR (2.33 ± 0.24), BL (116.36 ± 8.30 cm), BH (62.11 ± 3.38 cm), and FCC (18.86 ± 1.09 cm). All traits had a substantial number of phenotypic records, with over 10,000 records available. Notably, the BW trait had collected 28,348 records, but also exhibited the largest coefficient of variation of 16.27%. The phenotypic coefficients of variation for the other traits ranged from 5.44 to 10.30%, indicating relatively smaller variations.
3.2 Fixed effects analysis
Table 2 indicates that the effects of year-season, sex, farm, and parity on traits, including BW, AGE, BL, BH, and FCC were highly significant (P < 0.05) and thus, were incorporated into the genetic variance statistical model.
3.3 Variance components and genetic parameter estimates
When Model 1 was applied in the genetic estimation model in this study, the heritability estimates for BW, AGE, FCR, BL, BH, and FCC were 0.34 ± 0.015, 0.45 ± 0.016, 0.36 ± 0.024, 0.29 ± 0.016, 0.30 ± 0.018, and 0.21 ± 0.020, respectively (Table 3), all of which were of medium heritability. However, when the common litter effect was included as a random effect in the genetic assessment model (Model 2), the estimated heritability for BW, AGE, FCR, BL, BH, and FCC decreased to 0.17 ± 0.014, 0.30 ± 0.019, 0.28 ± 0.024, 0.16 ± 0.013, 0.14 ± 0.017, and 0.081 ± 0.016, respectively (Table 4). Besides that, BW, AGE, FCR, BL, BH, and FCC exhibited common litter effect values of 0.25, 0.20, 0.18, 0.23, 0.19, and 0.16, respectively. Notably, the common litter effect values for body size traits were all higher than the heritability, indicating a large influence of the common litter effect on both production and body size traits.
3.4 Genetic and phenotypic correlation among traits
The genetic correlations (Model 1) between AGE with BW, FCR, BL, BH, and FCC traits were −0.22, 0.62, −0.54, −0.28, and −0.31, respectively, while the phenotypic correlations were −0.17, 0.49, −0.41, −0.28 and −0.23, respectively (Table 5). The genetic and phenotypic correlations revealed negative associations between AGE and body size traits, while AGE and FCR showed highly significantly positive correlations. Additionally, BW exhibited negative correlation with production traits such as AGE and FCR, and positive correlations with body size traits including BL, BH, and FCC.
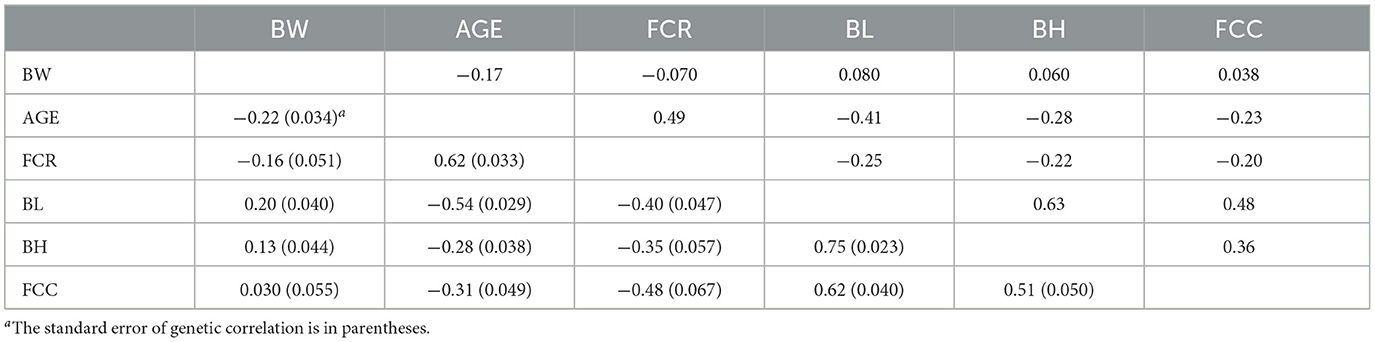
Table 5. Genetic (below diagonal) and phenotypic (above diagonal) correlations among traits for Model 1.
However, we found that the estimated genetic correlations (Model 2) between AGE with BW, FCR, BL, BH, and FCC were −0.28, 0.76, −0.71, −0.44, and −0.60, respectively, while the phenotypic correlations were −0.17, 0.52, −0.22, −0.13, and −0.24, respectively (Table 6). Similar to Model 1, AGE and FCR traits showed negative correlations with body size traits, while AGE and FCR traits exhibited significant positive correlations. Furthermore, strong positive correlations were observed among all body size traits.
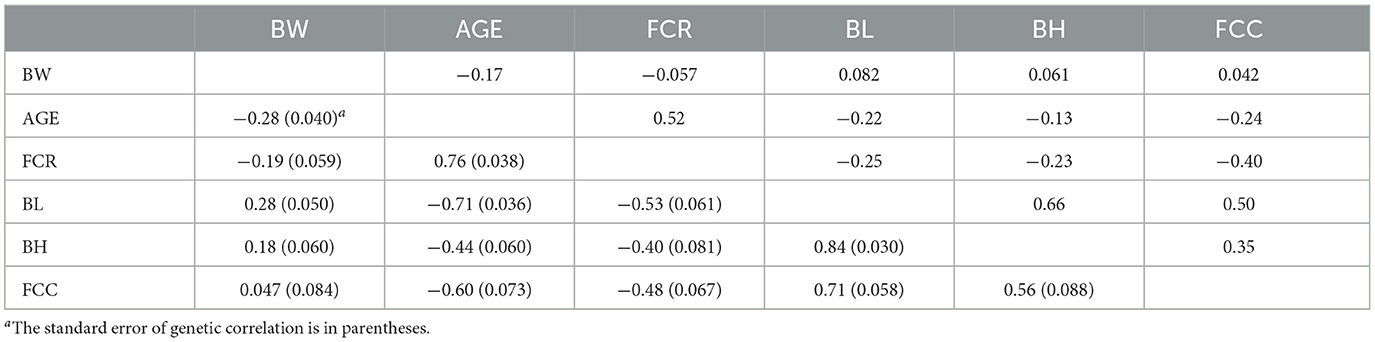
Table 6. Genetic (below diagonal) and phenotypic (above diagonal) correlations among traits for Model 2.
3.5 Genetic trends of production and body size traits
Figure 1 presents a comparison of the annual mean EBV for productivity and body size traits in the Duroc population estimated using models 1 and 2. Starting from 2012, the EBV for AGE traits showed a consistent decline, decreasing from −0.75 to −15.9 in Model 1 and from −0.009 to −3.55 in Model 2. Additionally, regarding the selection process for this trait, the EBV for BL traits exhibited a notable increase, ranging from −0.09 to 2.48 in Model 1 and from −0.006 to 0.45 in Model 2. Similarly, there was a consistent upward trend in BH_EBV, particularly since 2019, with values ranging from −0.09 to 0.5. However, the genetic responses for the remaining three traits displayed overall consistency between the two models, without apparent visual trends. Notably, Model 1 exhibited more pronounced predicted trends compared to Model 2.
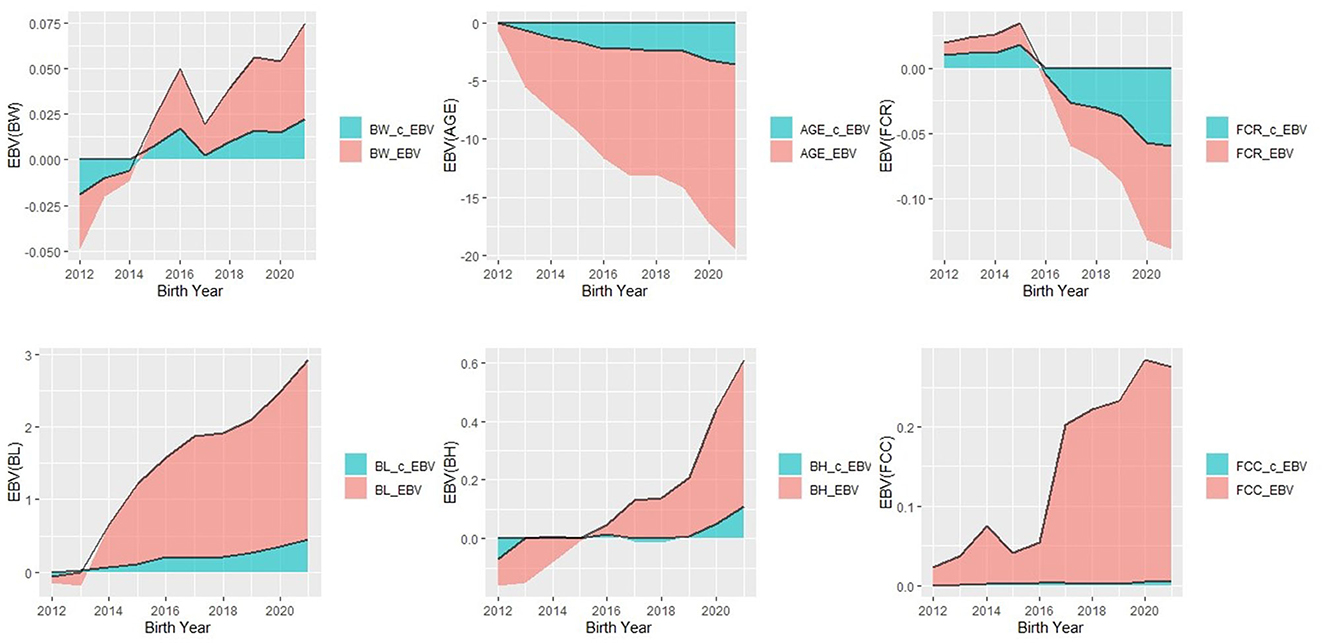
Figure 1. Trend of estimated breeding value (EBV) of all six traits in Duroc pigs by year of births analyzed by Model 1 and Model 2.
4 Discussion
4.1 Heritability of production traits
In this study, Model 1 estimated the heritability of BW, AGE, and FCR as 0.34 ± 0.015, 0.45 ± 0.016, and 0.36 ± 0.024, respectively, while in Model 2, these values were 0.17 ± 0.014, 0.30 ± 0.019, and 0.28 ± 0.024, respectively. Furthermore, we observed that the common litter effect values of BW, AGE, and FCR were 0.25, 0.20, and 0.18, respectively. Model 2 exhibited smaller AIC values for BW and AGE compared to Model 1, while for FCR, Model 2 yielded a higher AIC. Thu s, our findings suggest that (1) the common litter effects strongly influence the aforementioned traits, and (2) Model 2 provides a better fit for analyzing genetic parameters in BW and AGE. It is worth noting that previous studies have reported a range of heritability for BW, from 0.04 to 0.44 (13–20). Notably, the finding by Jiao et al. (21) align with our study. Similarly, the heritability estimates for AGE have been reported to range from 0.25 to 0.52 (22–25). For FCR, the heritability range is reported to be 0.20 to 0.42 (16, 22, 26–29). Although our study's results are consistent with previous reports, some discrepancies exist, which may be attributed to variations in species, sample size, analytical models, and population structure. Therefore, we conclude that the heritability of the production traits is moderate to low, and selecting solely based on phenotypic measurements results in slow genetic progress. However, integrating genomic selection techniques has the potential to greatly enhance the breeding progress.
4.2 Heritability of body size traits
We analyzed the heritability of BL, BH, and FCC using Model 1, which were estimated as 0.29 ± 0.016, 0.30 ± 0.018, and 0.21 ± 0.020, respectively. These estimates were higher than those reported by Ogawa et al. (30) for BH but lower than their BL and FCC values. Model 2 analysis revealed heritability estimates of 0.16 ± 0.013, 0.14 ± 0.017, and 0.081 ± 0.016 for BL, BH, and FCC, respectively. These estimates were higher than those identified by Hong et al. (7) for BH but lower than their BL findings. Additionally, previous studies by Johnson and Nugent 3rd (31) and Ohnishi and Satoh (32) reported a heritability range of 0.16 to 0.38 for BL. The common litter effect values for BL, BH, and FCC were 0.23, 0.19, and 0.16, respectively. Notably, the common litter effect values exceeded the heritability estimates, indicating their significant influence on body size traits. Furthermore, Model 2 consistently yielded lower AIC values for body size traits compared to Model 1, similar to BW and AGE. Thus, we found that incorporating the common litter effect as a random factor improves the fitting of the body size trait estimation model. However, an important challenge in practical production is the precise calculation of the common litter effect. Given the disparity between postpartum sow nurturing capability and piglet physical conditions, piglet fostering is a common practice in production. Consequently, full-sib piglets may exhibit varying within-litter effects due to fostering, which cannot be determined solely by pedigree information. Therefore, we recommend that foster care should be avoided in production as much as possible. In addition to the common litter effects, factors such as maternal effects and gene-environment interactions can also enhance the accuracy of genetic evaluation for these traits. However, further research is needed to provide more support for this perspective. We consider the body size traits to have low heritability, making it challenging to achieve satisfactory genetic progress through direct selection based on phenotypic measurements. Nonetheless, by integrating genomic selection techniques, there is a great potential to significantly enhance the breeding progress.
4.3 Genetic correlations and phenotypic correlations
The findings of this study indicated that the BW exhibits a negative genetic and phenotypic correlation with AGE and FCR. In contrast, it shows a positive correlation with BL and BH, and a slight correlation with FCC. Furthermore, the genetic and phenotypic correlations between AGE and FCR, as determined by models 1 and 2, were found to be 0.62 and 0.49, 0.76 and 0.52, respectively. These correlations were higher than the results reported by Cheng et al. (22), providing further support for the utilization of AGE traits as auxiliary indicators in estimating FCR across different countries. Conversely, AGE was strongly negatively correlated with body size traits, such as BL, BH, and FCC. The genetic correlation between AGE and BL was observed to be −0.54 (Model 1) and −0.71 (Model 2), consistent with the findings of Nikkilä et al. (33). Additionally, this study reveals highly significant positive genetic and phenotypic correlations among body size traits, aligning with the results reported by Hong et al. (7), Zhang et al. (34), and Liu et al. (35). Therefore, in the breeding process, the incorporation of simple and cost-effective auxiliary traits such as BL, BH, and AGE can effectively aid in the selection of FCR. This approach has the potential to significantly accelerate the genetic improvement of FCR in breeding companies. Moreover, elucidating the genetic correlations among traits is essential for breeders to make necessary adjustments and specifications in breeding programs, strategically plan breeding goals, and mitigate potential antagonistic effects among traits.
5 Conclusion
In conclusion, both production and body size traits exhibited moderate to low heritability in this study. It is crucial to consider the influence of common litter on these traits in genetic parameter analysis. The incorporation of a common litter effect model improved the model fit and enhanced the accuracy of breeding value estimation. Furthermore, our analysis of genetic and phenotypic correlations revealed significant positive associations between FCR and AGE traits. Conversely, FCR displayed a strong negative correlation with body size traits. These findings support the use of AGE traits as auxiliary indicators for selecting FCR and suggest that pigs with larger bodies exhibit higher feeding efficiency under equivalent feeding conditions. Additionally, this study demonstrates that selecting for the BL trait alone may lead to coordinated selection of other body size traits due to strong positive genetic correlations.
Data availability statement
The raw data supporting the conclusions of this article will be made available by the authors, without undue reservation.
Ethics statement
The animal study was approved by South China Agricultural University. The study was conducted in accordance with the local legislation and institutional requirements.
Author contributions
FZ: Validation, Visualization, Writing – original draft. DL: Validation, Writing – original draft. LD: Writing – review & editing. YH: Formal analysis, Writing – review & editing. HZ: Investigation, Resources, Writing – review & editing. GC: Funding acquisition, Supervision, Writing – review & editing. JY: Methodology, Project administration, Writing – review & editing. ZW: Conceptualization, Writing – review & editing.
Funding
The author(s) declare financial support was received for the research, authorship, and/or publication of this article. This research was supported by the Guangdong Provincial Key R&D Program (2022B0202090002). The funders had no role in study design, data collection and analysis, decision to publish, or preparation of the manuscript.
Acknowledgments
We thank the WENS Foodstuff Group Co., Ltd. who contributed data on their herds.
Conflict of interest
LD, HZ, GC, JY, and ZW were employed by company Wens Foodstuff Group Co., Ltd.
The remaining authors declare that the research was conducted in the absence of any commercial or financial relationships that could be construed as a potential conflict of interest.
Publisher's note
All claims expressed in this article are solely those of the authors and do not necessarily represent those of their affiliated organizations, or those of the publisher, the editors and the reviewers. Any product that may be evaluated in this article, or claim that may be made by its manufacturer, is not guaranteed or endorsed by the publisher.
References
1. Shu-qi D, Yuan-yu L, Yun-long M, Xi D, Ying-ting H, Ning G, et al. Genome-wide detection of selective signatures in a Duroc pig population. J Integr Agric. (2018) 17:2528–35. doi: 10.1016/S2095-3119(18)61984-7
2. Ma Y, Zhang S, Zhang K, Fang C, Xie S, Du X, et al. Genomic analysis to identify signatures of artificial selection and loci associated with important economic traits in Duroc pigs. G3. (2018) 8:3617–25. doi: 10.1534/g3.118.200665
3. Wang S, Yang J, Li G, Ding R, Zhuang Z, Ruan D, et al. Identification of homozygous regions with adverse effects on the five economic traits of Duroc pigs. Front Vet Sci. (2022) 9:855933. doi: 10.3389/fvets.2022.855933
4. Jiao S, Maltecca C, Gray KA, Cassady JP. Feed intake, average daily gain, feed efficiency, and real-time ultrasound traits in Duroc pigs: I. genetic parameter estimation and accuracy of genomic prediction. J Anim Sci. (2014) 92:2377–86. doi: 10.2527/jas.2013-7338
5. Alam M, Chang HK, Lee SS, Choi TJ. Genetic analysis of major production and reproduction traits of Korean Duroc, Landrace and Yorkshire pigs. Animals. (2021) 11:1321. doi: 10.3390/ani11051321
6. Hoque MA, Suzuki K, Kadowaki H, Shibata T, Oikawa T. Genetic parameters for feed efficiency traits and their relationships with growth and carcass traits in Duroc pigs. J Anim Breed Genet. (2007) 124:108–16. doi: 10.1111/j.1439-0388.2007.00650.x
7. Hong Y, Ye J, Dong L, Li Y, Yan L, Cai G, et al. Genome-wide association study for body length, body height, and total teat number in large white pigs. Front Genet. (2021) 12:650370. doi: 10.3389/fgene.2021.650370
8. Oliveira Junior GA, Schenkel FS, Alcantara L, Houlahan K, Lynch C, Baes CF. Estimated genetic parameters for all genetically evaluated traits in Canadian Holsteins. J Dairy Sci. (2021) 104:9002–15. doi: 10.3168/jds.2021-20227
9. Schmidtmann C, Segelke D, Bennewitz J, Tetens J, Thaller G. Genetic analysis of production traits and body size measurements and their relationships with metabolic diseases in German Holstein cattle. J Dairy Sci. (2022) 106:421–38. doi: 10.3168/jds.2022-22363
10. Dong L, Tan C, Cai G, Li Y, Wu D, Wu Z. Estimates of variance components and heritability using different animal models for growth, backfat, litter size, and healthy birth ratio in large white pigs. Can J Anim Sci. (2019) 100:330–6. doi: 10.1139/cjas-2019-0136
11. Henderson CR. Theoretical basis and computational methods for a number of different animal models. J Dairy Sci. (1988) 71:1–16. doi: 10.1016/S0022-0302(88)79974-9
12. Madsen P, Sørensen P, Su G, Damgaard L, Thomsen H, Labouriau R. “DMU-a package for analyzing multivariate mixed models,” In: Proceedings of 8th World Congress on Genetics Applied to Livestock Production. Belo Horizonte (2006).
13. Darfour-Oduro KA, Naazie A, Ahunu BK, Aboagye GS. Genetic parameter estimates of growth traits of indigenous pigs in Northern Ghana. Livest Sci. (2009) 125:187–91. doi: 10.1016/j.livsci,0.2009.04.007
14. Kapell DNRG, Ashworth CJ, Knap PW, Roehe R. Genetic parameters for piglet survival, litter size and birth weight or its variation within litter in sire and dam lines using Bayesian analysis. Livest Sci. (2011) 135:215–24. doi: 10.1016/j.livsci.2010.07.005
15. Dufrasne M, Misztal I, Tsuruta S, Holl J, Gray KA, Gengler N. Estimation of genetic parameters for birth weight, preweaning mortality, and hot carcass weight of crossbred pigs. J Anim Sci. (2013) 91:5565–71. doi: 10.2527/jas.2013-6684
16. Miar Y, Plastow G, Bruce H, Moore S, Manafiazar G, Kemp R, et al. Genetic and phenotypic correlations between performance traits with meat quality and carcass characteristics in commercial crossbred pigs. PLoS ONE. (2014) 9:e110105. doi: 10.1371/journal.pone.0110105
17. Serão N, Knauer M. Genetic parameters for age at puberty and associated traits in swine. J Anim Sci. (2017) 95:20. doi: 10.2527/asasmw.2017.043
18. Alves K, Schenkel FS, Brito LF, Robinson A. Estimation of direct and maternal genetic parameters for individual birth weight, weaning weight, and probe weight in Yorkshire and Landrace pigs. J Anim Sci. (2018) 96:2567–78. doi: 10.1093/jas/sky172
19. Ahmad SF, Gaur GK, Sahoo NR, Bharti PK, Naha BC. Genetic parameters of pre-weaning weights in crossbred piglets using multi-trait animal model. Trop Anim Health Prod. (2020) 52:109–14. doi: 10.1007/s11250-019-01985-0
20. Cedano-Castro JI, Jiménez R, Huamán A, Fuerst-Waltl B, Wurzinger M, Gutiérrez G. Estimation of genetic parameters for four Peruvian guinea pig lines. Trop Anim Health Prod. (2020) 53:34. doi: 10.1007/s11250-020-02473-6
21. Jiao S, Maltecca C, Gray KA, Cassady JP. Feed intake, average daily gain, feed efficiency, and real-time ultrasound traits in Duroc pigs: II. genomewide association. J Anim Sci. (2014) 92:2846–60. doi: 10.2527/jas.2014-7337
22. Cheng J, Newcom D, Schutz M, Muir W, Cui Q, Li B, et al. Selection for feed efficiency in Duroc pigs with the addition of daily feed intake data in a 2-stage selection procedure. Appl Anim Sci. (2019) 35:20–9. doi: 10.15232/aas.2018-01771
23. Tang Z, Xu J, Yin L, Yin D, Zhu M, Yu M, et al. Genome-wide association study reveals candidate genes for growth relevant traits in pigs. Front Genet. (2019) 10:302. doi: 10.3389/fgene.2019.00302
24. Ruan D, Zhuang Z, Ding R, Qiu Y, Zhou S, Wu J, et al. Weighted single-step GWAS identified candidate genes associated with growth traits in a Duroc pig population. Genes. (2021) 12:117. doi: 10.3390/genes12010117
25. Zhang Z, Ma P, Zhang Z, Wang Z, Wang Q, Pan Y. The construction of a haplotype reference panel using extremely low coverage whole genome sequences and its application in genome-wide association studies and genomic prediction in Duroc pigs. Genomics. (2022) 114:340–50. doi: 10.1016/j.ygeno.2021.12.016
26. Kadarmideen HN, Schwörer D, Ilahi H, Malek M, Hofer A. Genetics of osteochondral disease and its relationship with meat quality and quantity, growth, and feed conversion traits in pigs. J Anim Sci. (2004) 82:3118–27. doi: 10.2527/2004.82113118x
27. Do DN, Strathe AB, Jensen J, Mark T, Kadarmideen HN. Genetic parameters for different measures of feed efficiency and related traits in boars of three pig breeds. J Anim Sci. (2013) 91:4069–79. doi: 10.2527/jas.2012-6197
28. Ding R, Yang M, Wang X, Quan J, Zhuang Z, Zhou S, et al. Genetic architecture of feeding behavior and feed efficiency in a Duroc pig population. Front Genet. (2018) 9:220. doi: 10.3389/fgene.2018.00220
29. Homma C, Hirose K, Ito T, Kamikawa M, Toma S, Nikaido S, et al. Estimation of genetic parameter for feed efficiency and resilience traits in three pig breeds. Animal. (2021) 15:100384. doi: 10.1016/j.animal.2021.100384
30. Ogawa S, Yazaki N, Ohnishi C, Ishii K, Uemoto Y, Satoh M. Maternal effect on body measurement and meat production traits in purebred Duroc pigs. J Anim Breed Genet. (2021) 138:237–45. doi: 10.1111/jbg.12505
31. Johnson ZB, Nugent 3rd RA. Heritability of body length and measures of body density and their relationship to backfat thickness and loin muscle area in swine. J Anim Sci. (2003) 81:1943–9. doi: 10.2527/2003.8181943x
32. Ohnishi C, Satoh M. Estimation of genetic parameters for performance and body measurement traits in Duroc pigs selected for average daily gain, loin muscle area, and backfat thickness. Livest Sci. (2018) 214:161–6. doi: 10.1016/j.livsci.2018.05.022
33. Nikkilä MT, Stalder KJ, Mote BE, Rothschild MF, Gunsett FC, Johnson AK, et al. Genetic parameters for growth, body composition, and structural soundness traits in commercial gilts. J Anim Sci. (2013) 91:2034–46. doi: 10.2527/jas.2012-5722
34. Zhang H, Zhuang Z, Yang M, Ding R, Quan J, Zhou S, et al. Genome-wide detection of genetic loci and candidate genes for body conformation traits in Duroc × Landrace × Yorkshire crossbred pigs. Front Genet. (2021) 12:664343. doi: 10.3389/fgene.2021.664343
Keywords: Duroc pigs, common litter effect, animal model, genetic parameters, production traits, body size traits
Citation: Zhou F, Lin D, Dong L, Hong Y, Zeng H, Cai G, Ye J and Wu Z (2023) Genetic evaluation for production and body size traits using different animal models in purebred-Duroc pigs. Front. Vet. Sci. 10:1274266. doi: 10.3389/fvets.2023.1274266
Received: 08 August 2023; Accepted: 27 November 2023;
Published: 18 December 2023.
Edited by:
Martino Cassandro, University of Padua, ItalyReviewed by:
Ligang Wang, Chinese Academy of Agricultural Sciences (CAAS), ChinaNing Gao, Hunan Agricultural University, China
Copyright © 2023 Zhou, Lin, Dong, Hong, Zeng, Cai, Ye and Wu. This is an open-access article distributed under the terms of the Creative Commons Attribution License (CC BY). The use, distribution or reproduction in other forums is permitted, provided the original author(s) and the copyright owner(s) are credited and that the original publication in this journal is cited, in accordance with accepted academic practice. No use, distribution or reproduction is permitted which does not comply with these terms.
*Correspondence: Zhenfang Wu, d3pmZW1haWwmI3gwMDA0MDsxNjMuY29t; Jian Ye, anllMTk5MiYjeDAwMDQwOzEyNi5jb20=
†These authors have contributed equally to this work and share first authorship