- 1Department of Animal Science, Faculty of Agriculture, Khon Kaen University, Khon Kaen, Thailand
- 2Network Center of Animal Breeding and Omics Research, Khon Kaen University, Khon Kaen, Thailand
The objective was to compare and analyze the relationship between growth, purine content, uric acid, and superoxide dismutase (SOD) in purebred and crossbred Thai native chickens. A total of 300 Thai native chickens were divided into 3 groups. Group 1 was purebred Thai native chickens (100%TN), Group 2 was 50% Thai native chickens (50%TN), and Group 3 was 25% Thai native chickens (25%TN). Data included the body weight (BW), average daily gain (ADG), and breast circumference (BrC). At 6, 8, and 10 weeks of age, 10 chickens from each group were randomly euthanized to collect breast meat, liver, and blood samples to analyze the purine content consisting of total purine, adenine, guanine, xanthine, and hypoxanthine, and uric acid, in breast meat and liver and SOD in blood. A general linear model, Pearson correlation and principal component analysis were used to analyze the significant differences and relationship between variables. The results showed the 25%TN group had the highest growth traits at every age, while the 100%TN group had the lowest (p < 0.05). Consistent with the analysis results of purine values, purine content and uric acid in breast meat and liver and SOD in blood decreased with age (p < 0.05). The correlations between purine content (total purine, adenine, guanine, xanthine, and hypoxanthine) and growth traits (BW, ADG, and BrC) ranged from moderate negative to moderate positive (−0.542 to 0.253)(p < 0.05). The correlations between uric acid and growth traits (0.348–0.760) and SOD and growth traits (0.132–0.516) were low to moderate positive with significant differences (p < 0.05). The principal component plot, which highlighted three principal components (PC 1, PC 2, and PC 3), explained 86.44 and 86.53% of the total information in breast meat and liver for selecting animals for optimal balance of the variation in the growth traits, purine content, uric acid, and SOD. Although purebred Thai native chickens showed the lowest growth traits, purine content, uric acid, and SOD were also lowest compared to crossbred Thai native chickens. Therefore, the development of genetics in Thai native chickens to produce healthy food could be possible.
1. Introduction
Growth characteristics are one of the most economically important traits and have been continuously improved genetically in both commercial strains and native chickens (1–3) to produce more food for the world’s population, which is growing steadily (4, 5). While fast-growing chickens allow farmers to speed up production cycles, the other effect is an increase in undesirable bioactive compounds in the meat, especially increased accumulation of purine content and uric acid levels in meat and organs (6, 7).
Purines are nitrogen-containing compounds in many foods, including meat, seafood, and poultry (8–10). When the body metabolizes purines, they can be broken down into uric acid, accumulating in the blood and tissues and causing health problems, such as gout, hyperuricemia, renal dysfunction, and other cardiovascular diseases (11). Several studies have shown that fast-growing chickens could have higher levels of purines and uric acid in their meat and organs than slow-growing chickens (12, 13), and the levels of purines and uric acid are different from organ to organ, particularly in the liver, which is the most important organ to generate purines and uric acid (14, 15). This is because purines are essential components of deoxyribonucleic acid (DNA) and ribonucleic acid (RNA), which are necessary for cell division and protein synthesis (16, 17). As chickens grow rapidly, their muscle development and other metabolic processes require significant protein synthesis. Purines are essential for forming nucleotides, the building blocks of DNA and RNA, and play a role in energy metabolism (7, 18). However, chickens cannot synthesize purines de novo (19, 20); consequently, they must obtain purines through their diet or by recycling purines from other metabolic processes. Therefore, to improve their fast growth rate, chickens require more purines to support their metabolic needs (7). As a result, chickens that grow more quickly may have higher levels of purines in their tissues, which can be transferred to consumers who consume their meat or chicken products (13, 21, 22).
At the same time, fast-growing chickens are more prone to stress than slow-growing chickens due to their rapid growth rate and increased metabolic demands; the consequences are that fast-growing chickens have health and welfare problems (23, 24). In addition, fast-growing chickens are more susceptible to stressors such as overcrowding, high temperatures, and poor air quality (25, 26), which leads to increased mortality rates, reduced immune and antioxidant enzymes [catalase (CAT), superoxide dismutase (SOD), and glutathione peroxidase (GPX)], physiological parameters (27–30), and behavioral problems such as feather pecking and cannibalism (31). In contrast, slow-growing chickens may have a more robust immune system, better adaptability to environmental stressors, and lower susceptibility to behavioral problems (32, 33).
As mentioned above, this aligns with the popularity of consumers, who are more interested in good quality and healthy food (34, 35). Slow-growing chicken meat produced from native chickens is becoming increasingly interesting because it has several distinctive properties compared to commercial broiler meat products, including a unique flavor, firm and tender meat (36, 37), lower fat (38) and high levels of bioactive compounds, such as angiotensin-converting enzyme inhibitors (ACE), anserine, and carnosine, which have health benefits for consumers (13, 39–42).
However, the limitation of the slow growth rate in native chickens compared to commercial strains makes it challenging to raise native chickens for business. For this reason, farmers have used a crossbreeding mating system between commercial strains and native chickens to solve this problem (43–45). In this regard, it is necessary to have academic supporting information, especially the association between body weight traits, purines, uric acid, and SOD in native chicken meat and organs. Therefore, to ensure the development of crossbred native chicken genetics with both commercial potential and good properties of being a healthy food, the aim of this study was to compare and analyze the relationship between growth, purine content, uric acid, and SOD in purebred and crossbred Thai native chickens. Our results will benefit genetic selection in the slow-growing chicken breeding program.
2. Materials and methods
2.1. Ethics statement and animal management
All animal procedures were approved by the Institutional Animal Care and Use Committee, based on the Ethics of Animal Experimentation of the National Research Council of Thailand (record no. IACUC-KKU-14/65). This study was conducted at the experimental farm of the Network Center for Animal Breeding and Omics Research (NCAB), Faculty of Agriculture, Khon Kaen University, Thailand. A total of 300 Thai native chickens (Shee breed), which have been genetically selected from previous breed improvement program, were divided into 3 groups of 100 birds each. Group 1 was purebred Thai native (TN) chickens (100%TN), Group 2 was 50% female Thai native with 50% male broiler chickens (Ross 308 breed; 50%TN), and Group 3 was 25% female Thai native with 75% male broiler chickens (25%TN). Housing and management were processed under Thai native chicken rearing standards, and all birds were reared in an open-environmental system. The birds were fed ad libitum with commercial diets divided into 2 phases: starting phase (21% crude protein, 5% crude fiber, 3,100 kcal of ME/kg) for the first 4 weeks after hatching (0–4 weeks of age) and growing phase (19% crude protein, 5% crude fiber, 3,200 kcal of ME/kg) from 4 weeks old to the end of the experiment (10 weeks of age). When all chicks were born, they were numbered with legs tagged for identification until 4 weeks of age, then they were numbered with wings tagged. All chicken groups were raised using warming with a 100-watt lamp for 2 weeks. The lightening program consisted of two stages: the first stage was from hatching to 4 weeks with 24 h light/0 h dark; the second stage was from 5 to 10 weeks with natural light.
2.2. Data collection
Growth traits were recorded for each chicken from all groups. Body weights were individually collected using a weighing scale every 2 weeks from hatch to 10 weeks old. Breast circumferences were individually collected using measuring tape by inserting the tape beneath both wings and measuring the circumference at the largest part of the breast. The growth traits consisted of body weight at hatch (BW0), body weight at 2, 4, 6, 8, and 10 weeks of age (BW2, BW4, BW6, BW8, and BW10), average daily gain (ADG) during 0–2, 2–4, 4–6, 6–8, and 8–10 weeks of age (ADG0–2, ADG2–4, ADG4–6, ADG6–8 and ADG8–10), and breast circumference at 6, 8, and 10 weeks of age (BrC6, BrC8, and BrC10). At 6, 8, and 10 weeks of age, in each chicken group (100%TN, 50%TN, and 25%TN), 10 chickens (5 males and 5 females) were randomly euthanized to collect breast meat, liver, and blood samples. Breast meat samples were randomly collected from 6 locations from both sides of the breast, right side (upper, middle, lower) and left side (upper, middle, lower) using lancets and keeping the samples in plastic bags. Livers were collected for the whole piece and kept in plastic bags. The samples were prepared for the next step. The purine contents adenine, guanine, xanthine, and hypoxanthine, and uric acid (calculated from purine content), in breast meat and liver and superoxide dismutase (SOD) in blood were analyzed. The breast meat and liver were preserved using snap-freezing in liquid nitrogen and stored at −20°C for estimation of purine content and uric acid. Blood samples (approximately 1 mL) were collected for serum from the brachial vein and then stored at −20°C for future analysis of SOD.
2.3. Contents of purine and its derivative analysis
The contents of purine (adenine, guanine, hypoxanthine, and xanthine) and uric acid in breast meat and liver were determined using high-performance liquid chromatography (HPLC) (Shimadzu modelLC20A, Tokyo, Japan). The breast meat and liver samples were minced separately, and approximately 500 mg of the samples were homogenized in 10 mL of deionized water containing 35% perchloric acid. The homogenate was incubated at 95°C, shaken at 180 rpm for 1 h in a water bath, and then immediately neutralized with 30% potassium hydroxide. The mixture was centrifuged at 3,500 × g for 15 min at 4°C. Finally, the supernatant was filtered through 0.45 μm filtration membranes and injected into an HPLC for analysis. The analytical column used in the experiment was an Asahipak GS-HQ 320HQ, 300 mm × 7.5 mm, 6 μm column (Showa Denko K.K., Tokyo, Japan) at a temperature of 35°C. HPLC was performed using a mobile phase of 150 mM sodium phosphate buffer (pH 2.5) at a flow rate of 0.6 mL min−1, and the running time was 35 min. All samples were measured twice, and the values were averaged. The total purine content was calculated from the combined amounts of each derivative (14, 46).
2.4. Superoxide dismutase analysis
The activity of the antioxidant enzyme SOD was determined following the instructions described by Ratchamak et al. (47). Briefly, 10 μL of plasma was mixed with 835 μL of a solution containing cytochrome C (1 mM) and xanthine (50 mM), and 155 μL of xanthine oxidase was diluted in sodium phosphate/EDTA buffer (50 and 100 mM, respectively, pH 7.8). Then, the absorbance was determined every 5 min in a spectrophotometer fitted with a temperature regulator maintained at 25°C. The concentration of xanthine oxidase was calculated to generate the optimum amount of O2−, with a consequent reduction of cytochrome C that was calculated as the rate of cytochrome C reduction of 0.025 units of absorbance/min (at a wavelength of 550 nm); the basis of this calculation is that 1 unit of total SOD activity corresponded to 50% of this value. Therefore, SOD activity in the sample decreased the rate of cytochrome reduction compared to the blank.
2.5. Statistical analyses
In order to check the normality of the data, the growth traits, purine content, and uric acid [calculated according to Kaneko et al. (14)] in breast meat and liver, and SOD in blood were subjected to the Shapiro–Wilk test. Levene’s test was used to assess the homogeneity of variance across treatments (chicken groups). Where a significant deviation from a normal distribution and/or homogeneity of variance was observed, the nonparametric Kruskal–Wallis ANOVA rank test was applied to determine the differences between the chicken groups (called treatments). Data (growth traits, purine content, uric acid, and SOD) were subjected to multifactor (sex, chicken hatch set, and breed group) analysis of variance (ANOVA) using a general linear model for unbalanced data (GLM procedure) of SAS package v.9.0. Where significant differences were detected, multiple pairwise comparisons were conducted using Scheffe’s test (p < 0.05). The treatment effects were significant at p < 0.05 using the Dwass–Steel–Critchlow–Fligner test. All data are expressed as the mean values ± standard deviations. For correlation analysis, the data of all three chicken groups were combined. Data (growth traits, purine contents, uric acid, and SOD) were analyzed by Pearson correlation coefficients using PROC CORR in the SAS program to characterize the relationship among variables, and the results were presented as a heatmap correlation separated by chicken group using the Microsoft Excel v.2021. After that, principal component analysis (PCA) for all data separated by breast meat and liver was used to analyze the intercorrelation. PCA was then used to extract the most important information from the data table, compress the size of the dataset by keeping only the important information, simplify the description of the dataset, and analyze the structure of the observations and the variables to express this information as a set of new orthogonal variables by displaying them as points in maps. The PCA dataset was further tested using the Kaiser Meyer-Olkin (KMO) measure of sampling adequacy. A KMO measure of 0.60 and above is considered adequate (48) using the factor program of the SPSS 22 statistical package.
3. Results
3.1. Comparison of growth traits
A comparison of body weight (BW), average daily gain (ADG), breast circumference (BrC), feed intake (FI), and feed conversion ratio (FCR) in purebred and crossbred Thai native chickens is presented in Table 1. Statistical differences between BW and ADG in all three chicken groups were found from 2 to 10 weeks of age (p < 0.05). The highest BW and ADG were found in 25%TN chickens (258.19 g and 36.83 g/day), followed by 50%TN chickens (163.50 g and 18.45 g/day) and 100%TN chickens (138.33 g and 15.60 g/day). For breast circumference traits, significant differences were found at 6, 8, and 10 weeks of age (p < 0.05). Crossbred chickens with 25%TN had the highest breast circumference (28.03, 31.12, and 36.62 cm), while purebred chickens had the lowest circumference (19.64, 21.56, and 23.14 cm). For FI and FCR, the results were consistent with body weight and average daily gain (p < 0.05).
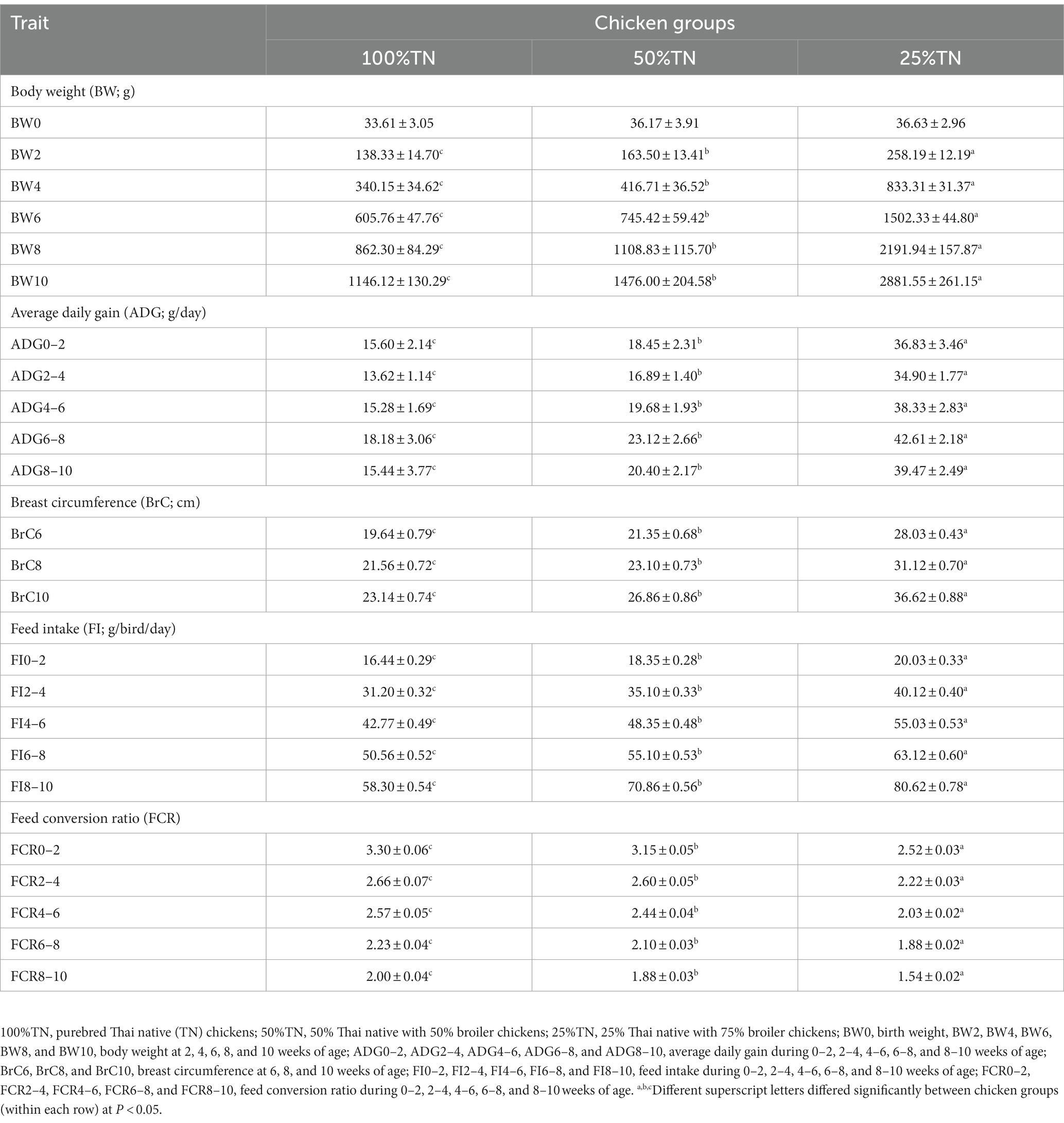
Table 1. Characteristics of growth traits of purebred and crossbred Thai native chickens (mean ± SD).
3.2. Comparison of purine content, uric acid, and SOD
Purine content and uric acid in breast meat and liver and SOD in the blood of purebred and crossbred Thai native chickens are shown in Table 2. The quantity of purine content and uric acid in the liver were higher than in breast meat in all chicken groups, except hypoxanthine, which was more abundant in breast meat than in the liver. The lowest total purine, adenine, guanine, xanthine, hypoxanthine, and uric acid levels were found in 100%TN chickens, and the highest values were found in 25%TN chickens (p < 0.05). However, the purine content and uric acid in 100%TN and 50%TN chickens were not significantly different (p > 0.05). In addition, the highest values for each parameter and each chicken group were found at 6 weeks of age. Afterward, values for each parameter decreased with age. For SOD, the results were in the same direction as the results of purine content and uric acid; 25%TH had the highest SOD, while 100%TH had the lowest SOD.
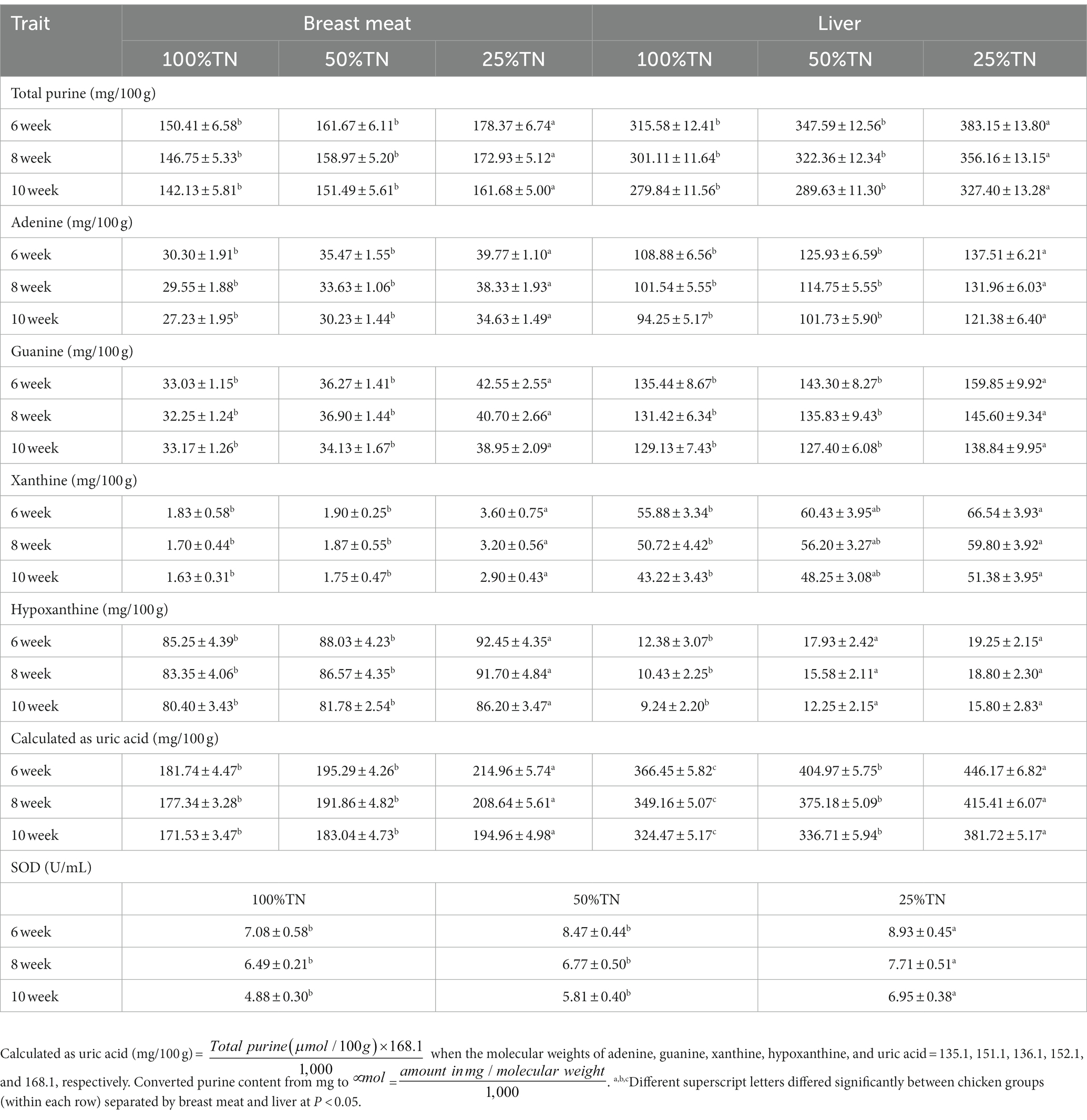
Table 2. Characteristics of purine content and uric acid in breast meat and liver and SOD in the blood of purebred and crossbred Thai native chickens (mean ± SD).
3.3. Correlations between purine content, uric acid, SOD, and growth traits
Correlation coefficients between purine content, uric acid, SOD, and growth traits are illustrated in Figure 1 using a heatmap. In the breast meat (Figure 1A), the correlations between total purine and uric acid and total purine and SOD were low positive correlations with values of 0.221 and 0.154, respectively, with significant differences (p < 0.05). However, when considering the contents of purine individually, low to moderate negative correlations were found between adenine, xanthine, and hypoxanthine and uric acid (−0.373, −0.197, and − 0.166) and SOD (−0.568, −0.298, and − 0.172) (p < 0.05), except correlations between guanine and uric acid (0.377) and guanine and SOD (0.354) were moderate positive correlations with significant differences (p < 0.05). The correlations between purine content (total purine, adenine, guanine, xanthine, and hypoxanthine) and growth traits (BW, ADG, and BrC) ranged from moderate negative to moderate positive (−0.542 to 0.253) with significant differences (p < 0.05). The correlations between uric acid and growth traits (0.348–0.760) and SOD and growth traits (0.132–0.516) were low to moderate positive with significant differences (p < 0.05). The correlation between uric acid and SOD was 0.685, with significant differences (p < 0.05). Finally, the correlations between and within BW, ADG, and BrC traits were highly positive and significant (p < 0.05). For the correlation in the liver (Figure 1B), it was found that most correlations between traits and within traits were in the same relationship as those found in the breast meat (p < 0.05), except for the correlations between growth traits and adenine, xanthine, and hypoxanthine, which were opposite in breast meat (negative correlations and significant, p < 0.05).
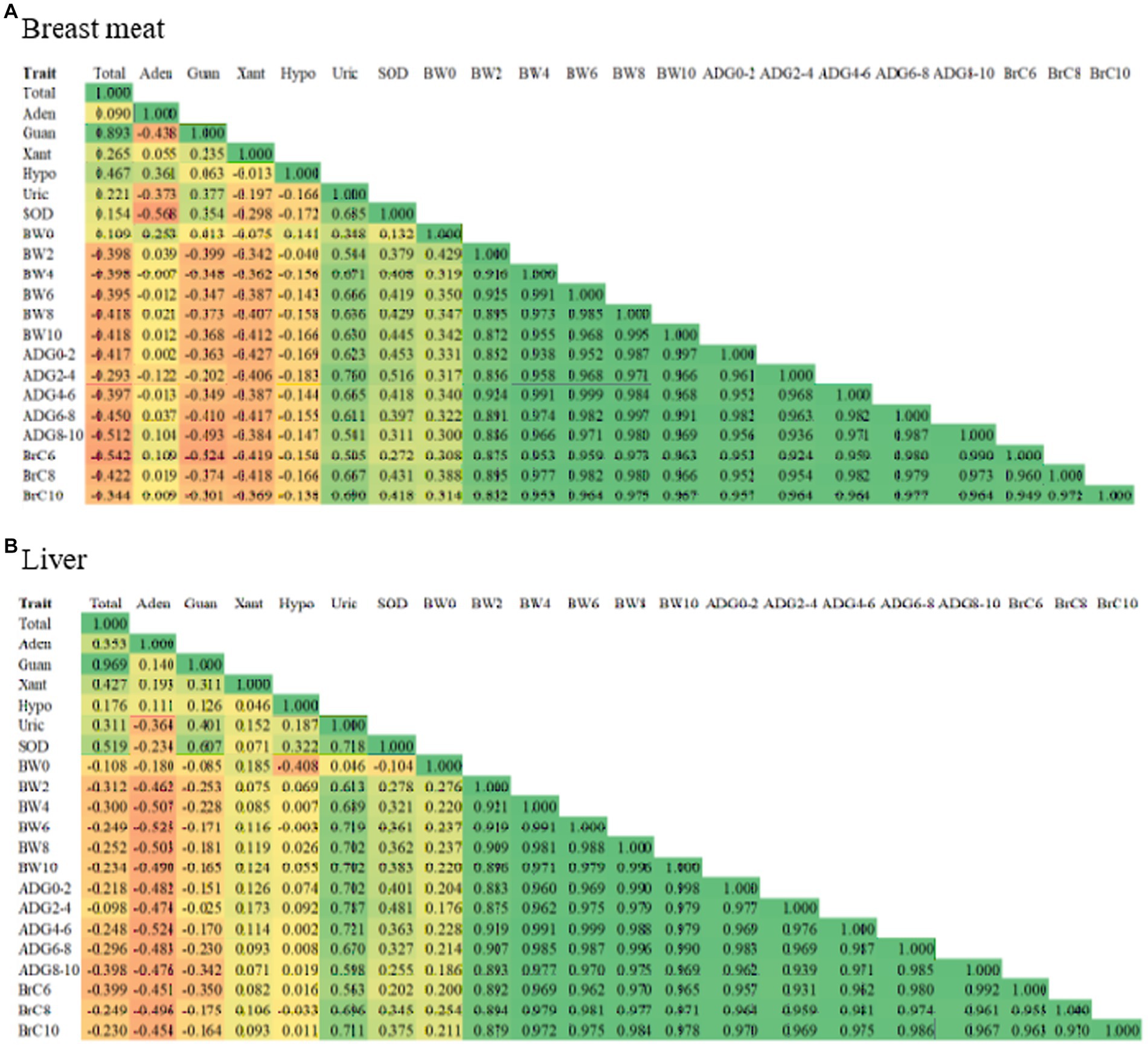
Figure 1. Heatmap illustrating correlation coefficient between purine content, uric acid, SOD, and growth traits in (A) breast meat, and (B) liver of purebred and crossbred Thai native chickens. Orange color means negative correlation, whereas green color represents positive correlation. Total, total purine; Aden, adenine; Guan, guanine; Xant, xanthine; Hypo, hypoxanthine; Uric, uric acid; SOD, superoxide dismutase; BW0, birth weight, BW2, BW4, BW6, BW8; BW, body weight at 2, 4, 6, 8, 10 weeks of age; ADG0–2, ADG2–4, ADG4–6, ADG6–8, ADG8–10, average daily gain during 0–2, 2–4, 4–6, 6–8, 8–10 weeks of age; BrC6, BrC8, BrC10, breast circumference at 6, 8, 10 weeks of age.
3.4. Principal component analysis results
The principal component (PC) plot of purine content and uric acid in breast meat and liver, SOD in blood, and growth traits is presented in Figure 2. The statistical analysis highlighted three principal components (PC1, PC2, and PC3), explaining 86.44% in breast meat (Figure 2A) and 86.53% in liver (Figure 2B) of the total information. The PC plot showed clusters of samples based on their similarity, which provided a good separation between purine content, uric acid, SOD, and growth traits. In breast meat (Figure 2A), the first principal component (PC1) accounted for 65.05% (eigenvalue = 13.04) of the total variance and was related to the overall 14 growth traits (BW, ADG, and BrC). The second component (PC2) contributed to 13.24% (eigenvalue = 2.79) of the total variance and was related to total purine, guanine, uric acid, and SOD. The third component (PC3) accounted for 8.15% (eigenvalue = 1.86) of the total variance and was related to xanthine and hypoxanthine. In the liver (Figure 2A), PC1 accounted for 64.94% (eigenvalue = 13.64) of the total variance and was related to uric acid and all growth traits (BW, ADG, and BrC), except birth weight (BW0). PC2 contributed 14.64% (eigenvalue = 3.08) to the total variance and was related to total purine, guanine, and SOD. PC3 accounted for 6.95% (eigenvalue = 1.46) of the total variance and was related to birth weight and hypoxanthine. Adenine and xanthine were not categorized in PC1 to PC3.
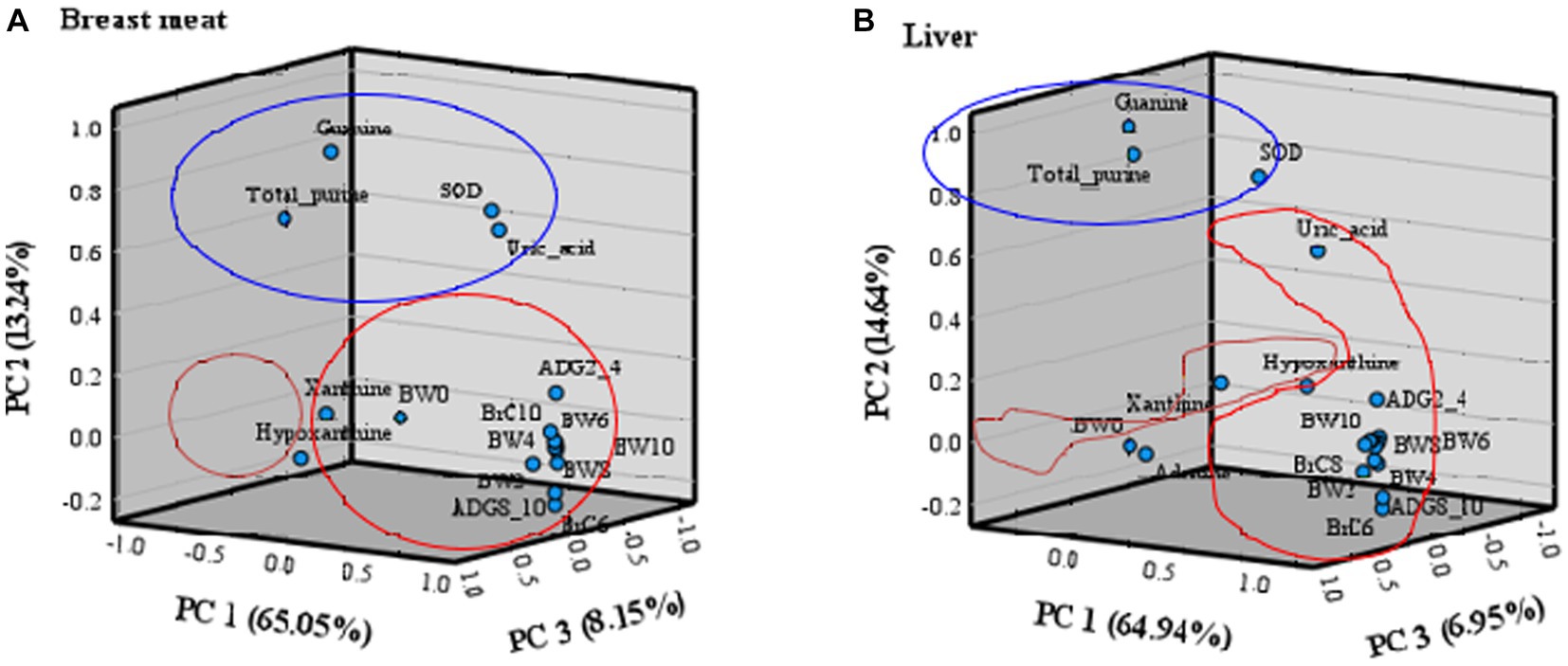
Figure 2. Three-dimensional component plot of purine content, uric acid, SOD, and growth traits in (A) breast meat and (B) liver by principal component analysis; PC 1 (red group), PC 2 (blue group), and PC 3 (orange group).
4. Discussion
This study provides researchers with more genetic information about the relationship between bioactive compounds, antioxidant enzymes, and growth traits in Thai native chickens and its crossbred, which is the first to be reported. Purine-free diets do not exist; therefore, consuming high-purine chicken meat may have disadvantages and clinical risks, especially in individuals susceptible to gout, hyperuricemia, and kidney stones. Also, this findings of our study can be applied in chicken breeding programs (selected low purine content genetic line) for the development and genetic improvement of purebred and crossbred native chickens to have good growth characteristics and be suitable for developing a healthy food from chicken meat.
For growth traits, the 25%TN (crossbred) group had the highest growth traits compared to the 50%TN (crossbred) and 100%TN (purebred) groups, which were expected results. This is due to the genes of broiler chickens in large quantities resulting in 25%TN crossbred native chickens having average body weight, average daily gain, and breast circumference in each age range being the highest. Raising crossbred native chickens like those in the 25%TN group will increase the production cycles per year by two times the rearing time of purebred native chickens. However, even though the chickens in the 100%TN (Pure Thai native chicken) group had an average body weight, average daily gain, and breast circumference that were the lowest, their BW8 was better than other Thai native chicken breeds, such as Lueng Hang Kao Kabinburi (49) at 642.08 g at 8 weeks of age, and Pradu Hang dum (1) at 808.01 g at 8 weeks of age. Importantly, this study demonstrates that the genetic improvement of growth traits is more accurate and better than those studied in other Thai native chicken breeds. For purine content and uric acid, the results in this study were consistent with other studies; for example, Potue et al. (13) found that the total purine and purine contents (adenine, guanine, and hypoxanthine) of commercial broilers were 15 and 41% higher than those of purebred and crossbred Thai native chickens (Pradu Hang dum), respectively. Meanwhile, Kaneko et al. (14) and Hou et al. (50) showed that the purine content in chicken liver was more than twice as high as that in chicken breast meat. In addition, a study by Hou et al. (50) showed that the purine content in chicken liver was greater than that in other parts. In addition, Kubota et al. (46) showed that the purine content decreased with age in Korat chickens as found in this study.
A positive relationship between purine content and uric acid is biologically significant because elevated purine content is often converted to higher uric acid levels (51, 52). Also, higher purine content and uric acid in the chicken liver compared with chicken breast meat can be attributed to several factors. For instance, the liver is responsible for various metabolic and recycling processes, including the breakdown of purine into uric acid. As a result, the liver contains higher concentrations of purines and their metabolic byproduct, uric acid (53). The diet of the chicken can also influence the purine content in its organs. Chickens that consume foods rich in purines, such as insects or plant materials high in purine content, may have higher purine levels in their liver than chickens with a different diet (51, 52). Hou et al. (50) showed that one-third of uric acid is produced exogenously and two-thirds endogenously. Different tissues of an animal can have varying compositions. The chicken liver is a highly metabolic organ with a unique cellular structure compared to chicken breast meat (54, 55). The liver contains higher concentrations of nucleic acids, which are purine-rich compounds, contributing to the overall purine content. Moreover, purebred Thai native chickens are considered to be slow-growing chickens (100%TN) with lower purine and uric acid levels than crossbred Thai native chickens, which are classified as fast-growing chickens (25%TN and 50%TN). This is due to the following factors. Firstly, fast-growing chickens are bred to have rapid muscle growth and high feed efficiency (56, 57). Their metabolism is geared toward quick energy production and protein synthesis, which can result in increased purine metabolism (7, 19, 20, 58). This elevated metabolic rate can lead to higher purine turnover and, subsequently, higher uric acid levels in the body. Secondly, slow-growing chicken breeds are generally selected for different traits compared to fast-growing breeds. The genetic makeup of slow-growing chickens may result in lower purine production or less purine metabolism, leading to reduced purine and uric acid levels (59). It is important to note that while slow-growing chickens may have lower purine and uric acid levels, the total purine in chicken meat is not exceptionally high (<200 mg/100 g) (13, 50).
As chickens age, their metabolic rate slow down, and their organs, including the liver and kidneys, become less efficient. To compensate for this, older chickens naturally produce lower levels of purines, resulting in lower uric acid levels in their bodies. This is thought to occur because the enzymes involved in purine synthesis and metabolism are downregulated or inhibited in older chickens, thereby reducing the overall production of purines and uric acid. Additionally, older chickens may also have changes in their gut microbiome, which can affect the metabolism of purines and other compounds. For example, changes in the gut microbiome can lead to a reduction in the availability of certain nutrients, which can, in turn, affect the synthesis of purines and other compounds. Overall, the metabolic mechanisms that result in lower levels of purines and uric acid in older chickens are complex and multifactorial and involve changes in enzyme activity, gut microbiome composition, and overall metabolic rate.
In the study of relationships, understanding correlation heatmaps can help us identify patterns and relationships between multiple variables. The positive relationship between total purine and uric acid content in chicken meat can be attributed to the fact that uric acid is a purine metabolite. When purines are broken down in the body, one of the end products is uric acid. Therefore, a higher concentration of purines in chicken meat would naturally result in a higher concentration of uric acid (13, 21, 22). Chickens, like humans and many other animals, metabolize purines into uric acid. The metabolic process involves the breakdown of dietary purines and endogenous purines (nucleic acids) within the chicken’s body. The uric acid formed during this process is excreted via the kidneys. An imbalance in purine metabolism or excretion can lead to elevated uric acid levels in meat. When the purine and uric acid levels in chicken meat increase significantly, several problems can occur within the chicken’s body, such as metabolic stress on the chicken’s body as it tries to process and eliminate excess purines, imbalance in the chicken’s immune system, more susceptible to infections and diseases causing inflammation, discomfort, and impaired mobility, finally reduced growth rates and decreased overall productivity. In addition, the synthesis of fatty acids, especially triglycerides, in the liver is associated with the de novo synthesis of purine, accelerating uric acid production (19, 20, 60).
The relationship between total purine and superoxide dismutase (SOD) activity in chicken meat may exhibit a positive correlation due to the antioxidant properties of purines and the role of SOD in the antioxidant defense system. Purines, including adenine and guanine, possess antioxidant properties (61). They can act as scavengers of free radicals and reactive oxygen species (ROS) that are generated during cellular metabolism. ROS can cause oxidative damage to cells and tissues (62). The presence of purine content in chicken meat with higher total purine content could indicate a higher antioxidant capacity, potentially helping to counteract oxidative stress (63). The positive correlation between total purine content and SOD activity in chicken meat could indicate that higher purine levels contribute to an enhanced antioxidant defense system. This suggests that chicken meat with higher total purine may exhibit increased levels of SOD activity, helping to neutralize ROS and reduce oxidative stress. It is important to note that various factors, including genetics, diet, and environmental conditions, can influence both purine content and SOD activity in chicken meat. Additionally, other antioxidant enzymes and compounds may also play a role in the overall antioxidant capacity of the meat. Therefore, the relationship between total purine and SOD activity should be considered within the broader context of antioxidant defenses in chicken meat.
The relationship between uric acid and SOD can be both positive and negative relationship, as the analysis suggests, because the two variables are very different in their mechanisms of action. Uric acid is a natural antioxidant present in the blood and tissues of humans and certain animals, including chickens. It acts as a scavenger of free radicals and reactive oxygen species (ROS), protecting cells from oxidative damage (64). Uric acid can directly neutralize certain ROS and indirectly contribute to the antioxidant defense system. At the same time, SOD is an enzyme that plays a vital role in the antioxidant defense system by catalyzing the conversion of superoxide radicals into less harmful molecules (65). SOD helps neutralize superoxide radicals, reducing oxidative stress and potential damage to cells. While both uric acid and SOD are involved in antioxidant processes, their relationship may not necessarily exhibit a consistent positive correlation in chicken meat. Several factors can influence the relationship between uric acid and SOD activity, including gene expression, enzymatic regulation, and cellular signaling pathways. The levels of SOD activity can be influenced by various factors, such as diet, genetic factors, and environmental conditions. These factors may not necessarily align with the levels of uric acid present in chicken meat. Although uric acid and SOD represent different components of this system, while they both contribute to the overall antioxidant capacity, their relationship can be influenced by other antioxidant enzymes and molecules present in chicken meat.
The relationship between purine content and growth characteristics is consistent due to the fundamental role that purine content plays in various biological processes. Conservation of basic cellular processes: purine content is involved in fundamental cellular processes such as DNA replication, RNA synthesis, and protein synthesis (16, 17). These processes are highly conserved across living organisms, from bacteria to plants and animals (66). The need for purine content in these processes ensures that they remain crucial for growth and development throughout the evolutionary spectrum (67). Biochemical pathways: purine content is synthesized through a series of enzymatic reactions in organisms. These pathways are conserved across species, ensuring the production of purine content for essential cellular functions (68). The consistency in purine biosynthesis pathways contributes to the consistent relationship between purine content and growth traits. Regulatory mechanisms: purine metabolism and utilization are tightly regulated in cells. Feedback mechanisms and regulatory enzymes control the levels of purine content to maintain the balance between synthesis and degradation (15). This regulation ensures that adequate purine levels are available for growth-related processes while preventing excessive accumulation or depletion (69). The consistency in these regulatory mechanisms contributes to the consistent relationship between purines and growth traits. Evolutionary significance: efficient utilization of resources is critical for the survival and reproduction of organisms (70). Purine content, which is vital for growth and development, has likely been under strong evolutionary pressure to maintain a consistent relationship with growth characteristics (71). Organisms that can effectively synthesize, regulate, and utilize purine content are more likely to exhibit consistent growth traits, providing them with a selective advantage. While the relationship between purine content and growth characteristics is generally consistent, it is important to recognize that there can still be variations due to genetic factors, environmental influences, and specific physiological contexts. Nonetheless, the central role of purines in critical cellular processes ensures a consistent relationship with growth traits across different organisms.
The principal component (PC) analysis technique can help to elucidate the relationship of various traits in growth characteristics (BW, ADG, and BrC), purine content, uric acid, and SOD in purebred and crossbred Thai native chickens. The PC plot expressed how the same PC explained correlated variables, and less correlated variables were explained by different PCs (72, 73). The results found this time, the PC1 was primarily associated with growth traits, with a positive correlation between traits evident from body weight at 2 weeks of age onwards. Thus, when choosing growth traits to analyze the selection indexes, one trait of body weight at 2 weeks (BW2) may be sufficient for evaluating growth genetics. It also enables faster animal selection without waiting for growth data to be recorded until maturity. The PC2 is related to total purine, guanine, uric acid, and SOD, with a mutually positive correlation in each trait. It is worth noting that guanine data collection can very well describe changes in total purine, uric acid, and SOD and can save time and budget for other data collection. For PC3, the relation to xanthine and hypoxanthine in both chicken breast meat and liver is unclear due to its low relationship; therefore, in the authors’ opinion, it is suggested that selective use of PC1 and PC2 is sufficient to explain the relationship between growth characteristics, bioactive compounds, and antioxidants to stress. The results led to an objective simultaneous analysis of these growth traits and bioactive compounds rather than an individual analysis of each. Additionally, the use of three-dimensional components (PC1, PC2, and PC3) was more appropriate than the use of the original interrelated parameters for predicting the growth characteristics and bioactive compounds of native chickens. In addition, three principal components in breast meat and liver could aid in selection and breeding programs in terms of the selection index and genetic evaluation (74) of native chickens. The present findings are consistent with those reported by Pinto et al. (75) in broilers, Yakubu et al. (76) in Nigerian indigenous chickens, and Mussa et al. (77) in Tanzanian indigenous chickens, where PC1 was termed overall body size, general weight, and meat quality. However, despite the potential benefit given by principal components for multitrait evaluation, its use in animal breeding programs has been relatively scarce, perhaps partly because it is not always possible to explain high variance percentages with few principal components, as occurred in this study. However, in circumstances when a good adjustment occurs, its practical use can bring great benefits to animal breeding programs because it can greatly facilitate evaluations. Principal component analysis is an interesting tool for evaluating and understanding the total variance originating in a group of correlated traits, allowing for a drastic reduction in the number of traits to be considered in the selection index of poultry breeding programs. The selection of animals for any principal component does not cause correlated responses in terms of other principal components (75). This is buttressed by the report of Yamaki et al. (78), who used principal component analysis to identify independent and informative variables, thereby eliminating redundant information for the purpose of reducing the costs of quail genetic programs.
In conclusion, purine content, uric acid, and SOD of Thai native chickens differed depending on the genetic make-up of Thai chickens’ breed groups ranging from purebred to their crossbreds. We found from our study that increased levels of purine and uric acid are associated with fast and improved growth performance in Thai chicken Crossbreds than in pure Thai chickens. However, exaggerated levels of purines and uric acid in chicken may permit negative biological consequences, such as metabolic stress, which may lead to the accumulation of more free radicals; this may cause an imbalance in the immune system, susceptibility to infections and disease conditions, reduced growth rates, and decreased overall productivity. The development of improved growth characteristics in chicken leads to an increase in uric acid which subsequently increases SOD values in terms of antioxidant potentials (scavenging of free radicals). Earlier studies concluded that consuming high-purine chicken meat may pose public health challenges and clinical risks, especially in individuals susceptible to gout, hyperuricemia, and kidney stones. Finally, this study provides biological information to livestock farmers, particularly for poultry farmers, particularly Thai farmers to produce crossbred native chickens with faster production cycles.
Data availability statement
The original contributions presented in the study are included in the article/supplementary material, further inquiries can be directed to the corresponding author.
Ethics statement
The animal studies were approved by the Institutional Animal Care and Use Committee, based on the Ethics of Animal Experimentation of the National Research Council of Thailand (record no. IACUC-KKU-14/65). The studies were conducted in accordance with the local legislation and institutional requirements. Written informed consent was obtained from the owners for the participation of their animals in this study.
Author contributions
WB: Funding acquisition, Methodology, Project administration, Writing – original draft, Writing – review & editing, Conceptualization, Data curation, Formal analysis. VT: Conceptualization, Data curation, Formal analysis, Methodology, Writing – original draft. KC: Conceptualization, Data curation, Writing – original draft. WL: Writing – original draft. VC: Formal analysis, Funding acquisition, Project administration, Writing – original draft.
Funding
The author(s) declare financial support was received for the research, authorship, and/or publication of this article. This research was funded by the Research Program of Khon Kaen University for scholarships (grant no. RP66-3-001).
Acknowledgments
The authors gratefully thank the Network Center for Animal Breeding and Omics Research (NCAB), Faculty of Agriculture, Khon Kaen University, Thailand for the data and generous support.
Conflict of interest
The authors declare that the research was conducted in the absence of any commercial or financial relationships that could be construed as a potential conflict of interest.
Publisher’s note
All claims expressed in this article are solely those of the authors and do not necessarily represent those of their affiliated organizations, or those of the publisher, the editors and the reviewers. Any product that may be evaluated in this article, or claim that may be made by its manufacturer, is not guaranteed or endorsed by the publisher.
References
1. Chaikuad, N, Loengbudnark, W, Chankitisakul, V, and Boonkum, W. Genetic comparisons of body weight, average daily gain, and breast circumference between slow-growing Thai native chickens (Pradu hang dum) raised on-site farm and on-station. Vet Sci. (2023) 10:1–14. doi: 10.3390/vetsci10010011
2. Goto, T, Ishikawa, A, Nishibori, M, and Tsudzuki, M. A longitudinal quantitative trait locus mapping of chicken growth traits. Mol Genet Genomics. (2018) 294:243–52. doi: 10.1007/s00438-018-1501-y
3. Manjula, P, Park, HB, Seo, D, Choi, N, Jin, S, Ahn, SJ, et al. Estimation of heritability and genetic correlation of body weight gain and growth curve parameters in Korean native chicken. Asian Australas J Anim Sci. (2018) 31:26–31. doi: 10.5713/ajas.17.0179
4. Godfray, HC, Beddington, JR, Crute, IR, Haddad, L, Lawrence, D, Muir, JF, et al. Food security: the challenge of feeding 9 billion people. Science. (2010) 28:1185383. doi: 10.1126/science.1185383
5. Pawar, SS, Sajjanar, B, Lonkar, VD, Kurade, NP, Kadam, AS, Nirmal, AV, et al. Assessing and mitigating the impact of heat stress in poultry. Adv Anim Vet Sci. (2016) 4:332–41. doi: 10.14737/journal.aavs/2016/4.6.332.341
6. Jinnah, HA, Sabina, RL, and Van Den Berghe, G. Metabolic disorders of purine metabolism affecting the nervous system. Handb Clin Neurol. (2013) 113:1827–36. doi: 10.1016/B978-0-444-59565-2.00052-6
7. Welch, MM, and Rudolph, FB. Regulation of purine biosynthesis and interconversion in the chick. J Biol Chem. (1982) 257:13253–6. doi: 10.1016/S0021-9258(18)33438-0
8. Aihemaitijiang, S, Zhang, Y, Zhang, L, Yang, J, Ye, C, Halimulati, M, et al. The association between purine-rich food intake and hyperuricemia: a cross-sectional study in Chinese adult residents. Nutrients. (2020) 12:3835. doi: 10.3390/nu12123835
9. Pan, H, Rong, S, Zou, L, Wang, Z, and Yang, Y. The contents of purine in common animal foods in China. Acta Nutr Sin. (2012) 34:74–8.
10. Souci, SW, Fachmann, W, and Kraut, H. Food composition and nutrition table. 7th edn. Stuttgart: MedPharm (2006).
11. Cleophas, MC, Joosten, LA, Stamp, LK, Dalbeth, N, Woodward, OM, and Merriman, TR. ABCG2 polymorphisms in gout: insights into disease susceptibility and treatment approaches. Pharmacogenomics Pers Med. (2017) 10:129–42. doi: 10.2147/PGPM.S105854
12. Daneshmand, A, Kermanshahi, H, Mesgaran, MD, King, AJ, and Ibrahim, SA. Effect of purine nucleosides on growth performance, gut morphology, digestive enzymes, serum profile and immune response in broiler chickens. Br Poult Sci. (2017) 58:536–43. doi: 10.1080/00071668.2017.1335859
13. Potue, P, Chiangsaen, P, Maneesai, P, Khamseekaew, J, Pakdeechote, P, Chankitisakul, V, et al. Effects of Thai native chicken breast meat consumption on serum uric acid level, biochemical parameters, and antioxidant activities in rats. Sci Rep. (2022) 12:14056. doi: 10.1038/s41598-022-18484-2
14. Kaneko, K, Aoyagi, Y, Fukuuchi, T, Inazawa, K, and Yamaoka, N. Total purine and purine base content of common foodstuffs for facilitating nutritional therapy for gout and hyperuricemia. Biol Pharm Bull. (2014) 37:709–21. doi: 10.1248/bpb.b13-00967
15. Maiuolo, J, Oppedisano, F, Gratteri, S, Muscoli, C, and Mollace, V. Regulation of uric acid metabolism and excretion. Int J Cardiol. (2016) 213:8–14. doi: 10.1016/j.ijcard.2015.08.109
16. Angstadt, CN. Purine and pyrimidine metabolism The University of Utah (1997) Available at: http://library.med.utah.edu/NetBiochem/pupyr/pp.htm.
17. Garrett, R, and Grisham, C. Nucleotides and nucleic acids In: R Garrett ed. Biochemistry. 3rd edn. Singapore: Thomson Brooks/Cole (2005). 309–34.
18. Henderson, JF, and Paterson, ARP. Nucleotide metabolism, an introduction. New York, NY: Academic Press (1973).
19. Burns, RA, and Buttery, PJ. Purine metabolism and urate biosynthesis in isolated chicken hepatocytes. Arch Biochem Biophy. (1984) 233:507–14. doi: 10.1016/0003-9861(84)90474-0
20. Lipstein, B, Boer, P, and Sperling, O. Regulation of de novo purine synthesis in chick liver slices: role of phosphoribosylpyrophosphate availability and of salvage purine nucleotide synthesis. Biochim Biophys Acta Nucleic Acids Protein Synth. (1978) 521:45–54. doi: 10.1016/0005-2787(78)90247-2
21. Wu, M, Zhang, W, Shen, X, and Wang, W. Simultaneous determination of purines and uric acid in Chinese chicken broth using TFA/FA hydrolysis coupled with HPLC-VWD. Foods. (2021) 10:2814. doi: 10.3390/foods10112814
22. Yang, H, Zhang, J, Liu, G, and Zhang, P. Measurement of purines and uric acid simultaneous in meat with high performance liquid chromatographys. J Hyg Res. (2012) 41:303–6.
23. Rayner, AC, Newberry, RC, Vas, J, and Mullan, S. Slow growing broilers are healthier and express more behavioural indicators of positive welfare. Sci Rep. (2020) 10:15151. doi: 10.1038/s41598-020-72198-x
24. Zhang, J, Schmidt, CJ, and Lamont, SJ. Transcriptome analysis reveals potential mechanisms underlying differential heart development in fast- and slow-growing broilers under heat stress. BMC Genomics. (2017) 18:295. doi: 10.1186/s12864-017-3675-9
25. Boonkum, W, Duangjinda, M, Kananit, S, Chankitisakul, V, and Kenchaiwong, W. Genetic effect and growth curve parameter estimation under heat stress in slow-growing Thai native chickens. Vet Sci. (2021) 8:297. doi: 10.3390/vetsci8120297
26. Gomes, AV, Quinteiro-Filho, WM, Ribeiro, A, Ferraz-de-Paula, V, Pinheiro, ML, Baskeville, E, et al. Overcrowding stress decreases macrophage activity and increases Salmonella Enteritidis invasion in broiler chickens. Avian Pathol. (2014) 43:82–90. doi: 10.1080/03079457.2013.874006
27. Cengiz, Ö, Köksal, BH, Tatl, O, Sevim, Ö, Ahsan, U, Üner, AG, et al. Effect of dietary probiotic and high stocking density on the performance, carcass yield, gut microflora, and stress indicators of broilers. Poult Sci. (2015) 94:2395–403. doi: 10.3382/ps/pev194
28. Farag, MR, and Alagawany, M. Physiological alterations of poultry to the high environmental temperature. J Therm Biol. (2018) 76:101–6. doi: 10.1016/j.jtherbio.2018.07.012
29. Roushdy, EM, Zaglool, AW, and El-Tarabany, MS. Effects of chronic thermal stress on growth performance, carcass traits, antioxidant indices and the expression of HSP70, growth hormone and superoxide dismutase genes in two broiler strains. J Therm Biol. (2018) 74:337–43. doi: 10.1016/j.jtherbio.2018.04.009
30. Wasti, S, Sah, N, and Mishra, B. Impact of heat stress on poultry health and performances, and potential mitigation strategies. Animals. (2020) 10:1266–86. doi: 10.3390/ani10081266
31. Nielsen, BL, Thomsen, MG, Sørensen, P, and Young, JF. Feed and strain effects on the use of outdoor areas by broilers. Br Poult Sci. (2003) 44:161–9. doi: 10.1080/0007166031000088389
32. Duangjinda, M, Tunim, S, Duangdaen, C, and Boonkum, W. Hsp70 genotypes and heat tolerance of commercial and native chickens reared in hot and humid conditions. Braz J Poult Sci. (2017) 19:7–18. doi: 10.1590/1806-9061-2016-0245
33. Ghayas, A, Hussain, J, Mahmud, A, Jaspal, MH, Ishaq, HM, and Hussain, A. Behaviour, welfare, and tibia traits of fast- and slow-growing chickens reared in intensive and free range systems. S Afr J Anim Sci. (2021) 51:22–32. doi: 10.4314/sajas.v51i1.3
34. Lim, SY, Lee, KW, Seow, WL, Mohamed, NA, Devaraj, NK, and Amin-Nordin, S. Effectiveness of integrated technology apps for supporting healthy food purchasing and consumption: A systematic review. Foods. (2021) 10:1861. doi: 10.3390/foods10081861
35. Meeprom, S, Jaratmetakul, P, and Boonkum, W. Examining the effect of consumer experience on co-creation and loyalty for healthy meat consumption. Front Sustain Food Syst. (2023) 7:1123984. doi: 10.3389/fsufs.2023.1123984
36. Jaturasitha, S, Chaiwang, N, and Kreuzer, M. Thai native chicken meat, an option to meet the demands for specific meat quality by certain groups of consumers, a review. Anim Product Sci. (2017) 57:1582–7. doi: 10.1071/AN15646
37. Wattanachant, S, Benjakul, S, and Ledward, DA. Composition, color, and texture of Thai indigenous and broiler chicken muscles. Poult Sci. (2004) 83:123–8. doi: 10.1093/ps/83.1.123
38. Tunim, S, Phasuk, Y, Aggrey, SE, and Duangjinda, M. Gene expression of fatty acid binding protein genes and its relationship with fat deposition of Thai native crossbreed chickens. Anim Biosci. (2021) 34:751–8. doi: 10.5713/ajas.20.0020
39. Ali, M, Lee, SY, Park, JY, Jung, S, Jo, C, and Nam, KC. Comparison of functional compounds and micronutrients of chicken breast meat by breeds. Food Sci Anim Resour. (2019) 39:632–42. doi: 10.5851/kosfa.2019.e54
40. Charoensin, S, Laopaiboon, B, Boonkum, W, Phetcharaburanin, J, Villareal, MO, Isoda, H, et al. Thai native chicken as a potential functional meat source rich in anserine, anserine/carnosine, and antioxidant substances. Animals. (2021) 11:902. doi: 10.3390/ani11030902
41. Kojima, S, Saegusa, H, and Sakata, M. Histidine-containing dipeptide concentration and antioxidant effects of meat extracts from silky fowl, comparison with meat-type chicken breast and thigh meats. Food Sci Technol Res. (2014) 20:621–8. doi: 10.3136/fstr.20.621
42. Sangsawad, P, Roytrakul, S, and Yongsawatdigul, J. Angiotensin converting enzyme (ACE) inhibitory peptides derived from the simulated in vitro gastrointestinal digestion of cooked chicken breast. J Funct Foods. (2017) 29:77–83. doi: 10.1016/j.jf.2016.12.005
43. Chomchuen, K, Tuntiyasawasdikul, V, Chankitisakul, V, and Boonkum, W. Comparative study of phenotypes and genetics related to the growth performance of crossbred Thai indigenous (KKU1 vs. KKU2) chickens under hot and humid conditions. Vet Sci. (2022) 9:263. doi: 10.3390/vetsci9060263
44. Islam, MA, and Nishibori, M. Crossbred chicken for poultry production in the tropics. J Poult Sci. (2010) 47:217–79. doi: 10.2141/jpsa.010033
45. Ndofor-Foleng, HM, Oleforuh-Okoleh, V, Musongong, GA, Ohageni, J, and Duru, UE. Evaluation of growth and reproductive traits of Nigerian local chicken and exotic chicken. Indian J Anim Res. (2015) 49:155–60. doi: 10.5958/0976-0555.2015.00046.1
46. Kubota, S, Vandee, A, Keawnakient, P, Molee, W, Yongsawatdikul, J, and Molee, A. Genetics and genomics effects of the MC4R, CAPN1, and ADSL genes on body weight and purine content in slow-growing chickens. Poult Sci. (2019) 98:4327–37. doi: 10.3382/ps/pez262
47. Ratchamak, R, Thananurak, P, Boonkum, W, Semaming, Y, and Chankitisakul, V. The melatonin treatment improves the ovarian responses after superstimulation in Thai-Holstein crossbreeds under heat stress conditions. Front. Vet. Sci. (2022) 9:888039. doi: 10.3389/fvets.2022.888039
48. Eyduran, E, Topal, M, and Sonmez, AY. Use of factor scores in multiple regression analysis for estimation of body weight by several body measurements in brown trouts (Salmo trutta fario). Int J Agri Biol. (2010) 12:611–5.
49. Tongsiri, S, Jeyaruban, GM, Hermesch, S, Vander Werf, JHJ, Li, L, and Chormai, T. Genetic parameters and inbreeding effects for production traits of Thai native chickens. Asian-Australas J Anim Sci. (2019) 32:930–938. doi: 10.5713/ajas.18.0690
50. Hou, C, Xiao, G, Amakye, WK, Sun, J, Xu, Z, and Ren, J. Guidelines for purine extraction and determination in foods. Food Frontiers. (2021) 2:557–73. doi: 10.1002/fft2.100
51. Choi, HK, Liu, S, and Cruhan, G. Intake of purine-rich foods, protein, and dairy products and relationship to serum levels of uric acid: the third National Health and nutrition examination survey. Arthritis Rheum. (2005) 52:283–9. doi: 10.1002/art.20761
52. Zgaga, L, Theodoratou, E, Kyle, J, Farrington, SM, Agakov, F, Tenesa, A, et al. The association of dietary intake of purine-rich vegetables, sugar-sweetened beverages and dairy with plasma urate, in a cross-sectional study. PLoS One. (2012) 7:e38123. doi: 10.1371/journal.pone.0038123
53. El Ridi, R, and Tallima, H. Physiological functions and pathogenic potential of uric acid: A review. J Adv Res. (2017) 8:487–93. doi: 10.1016/j.jare.2017.03.003
54. Li, H, Wang, T, Xu, C, Wang, D, Ren, J, Li, Y, et al. Transcriptome profile of liver at different physiological stages reveals potential mode for lipid metabolism in laying hens. BMC Genomics. (2015) 16:763. doi: 10.1186/s12864-015-1943-0
55. Wang, X, Yang, L, Wang, H, Shao, F, Yu, J, Jiang, H, et al. Growth hormone-regulated mRNAs and miRNAs in chicken hepatocytes. PLoS One. (2014) 9:e112896. doi: 10.1371/journal.pone.0112896
57. Siegel, PB. Evolution of the modern broiler and feed efficiency. Annu Rev Anim Biosci. (2014) 2:375–85. doi: 10.1146/annurev-animal-022513-114132
58. Kondo, M, Yamaoka, T, Honda, S, Miwa, Y, Katashima, R, Moritani, M, et al. The rate of cell growth is regulated by purine biosynthesis via ATP production and G1 to S phase transition. J Biochem. (2000) 128:57–64. doi: 10.1093/oxfordjournals.jbchem.a022730
59. Poompramun, C, Hennequet-Antier, C, Thumanu, K, Sinpru, P, Pengsanthia, S, Molee, W, et al. Revealing pathways associated with feed efficiency and meat quality traits in slow-growing chickens. Animals. (2021) 11:2977. doi: 10.3390/ani11102977
60. de Oliveira, EP, and Burini, RC. High plasma uric acid concentration: causes and consequences. Diabetol Metab Syndr. (2012) 4:1–7. doi: 10.1186/1758-5996-4-12
61. Melzer, MS, and Tomlinson, RV. Antioxidative effects of purine bases on hydrogen peroxide oxidation of pyrimidine bases. Arch Biochem Biophys. (1966) 115:226–9. doi: 10.1016/S0003-9861(66)81061-5
62. Surai, PF, Kochish, II, Fisinin, VI, and Kidd, MT. Antioxidant defence systems and oxidative stress in poultry biology: an update. Antioxidants. (2019) 8:235. doi: 10.3390/antiox8070235
63. Keenan, RT. The biology of urate. Semin Arthritis Rheum. (2020) 50:S2–S10. doi: 10.1016/j.semarthrit.2020.04.007
64. Sautin, YY, and Johnson, RJ. Uric acid: the oxidant-antioxidant paradox. Nucleosides Nucleotides Nucleic Acids. (2008) 27:608–19. doi: 10.1080/15257770802138558
66. Huynh, TN, and Stewart, V. Purine catabolism by enterobacteria. Adv Microb Physiol. (2023) 82:205–66. doi: 10.1016/bs.ampbs.2023.01.001
67. Daneshmand, A, Kermanshahi, H, Mesgaran, MD, King, AJ, Ibrahim, SA, and Klasing, KC. Combination of purine and pyrimidine nucleosides influences growth performance, gut morphology, digestive enzymes, serum biochemical indices and immune functions in broiler chickens. Anim Feed Sci Technol. (2017) 228:186–93. doi: 10.1016/j.anifeedsci.2017.04.011
68. Sowerby, SJ, and Heckl, WM. The role of self-assembled monolayers of the purine and pyrimidine bases in the emergence of life. Orig Life Evol Biosph. (1998) 28:283–310. doi: 10.1023/a:1006570726326
69. Itakura, M, Sabina, RL, Heald, PW, and Holmes, EW. Basis for the control of purine biosynthesis by purine ribonucleotides. J Clin Invest. (1981) 67:994–1002. doi: 10.1172/jci110150
70. López, JM, Outtrimc, EL, Fu, R, Sutcliffe, DJ, Torresh, RJ, and Jinnah, HA. Physiological levels of folic acid reveal purine alterations in Lesch-Nyhan disease. Biochemist. (2020) 117:12071–9. doi: 10.1073/pnas.2003475117
71. Wegelin, I, Manzol, FA, and Pane, G. Enzymes involved in amp metabolism in chick embryo muscles. Comp Biochem Physiol. (1978) 59:55–7. doi: 10.1016/0305-0491(78)90270-5
72. Belhaj, K, Mansouri, F, Ben Moumen, A, Sindic, M, Fauconnier, ML, Boukharta, M, et al. Proximate composition, amino acid profile, and mineral content of four sheep meats reared extensively in Morocco: a comparative study. Sci World J. (2021) 2021:6633774. doi: 10.1155/2021/6633774
73. Michalczuka, M, Marzecb, A, Damaziaka, K, Zdanowska-Sąsiadekc, Ż, Bogdańskaa, K, Slósarza, J, et al. Application of the support sensory system and principal component analysis to compare meat of chickens of two genotypes. CyTA J Food. (2018) 16:667–71. doi: 10.1080/19476337.2018.1448457
74. Van Vleck, LD. Selection index and introduction to mixed model methods. Boca Raton, FL: CRC Press (1993).
75. Pinto, LFB, Dacker, IU, De Melo, CMR, Ledur, MC, and Coutinho, LL. Principal components analysis applied to performance and carcass traits in the chicken. Anim Res. (2006) 55:419–25. doi: 10.1051/animres:2006022
76. Yakubu, A, Kuje, D, and Okpeku, M. Principal components as measures of size and shape in Nigerian indigenous chickens. Thai J Agric Sci. (2009) 42:167–76.
77. Mussa, NJ, Kibonde, SF, Boonkum, W, and Chankitisakul, V. The Comparison between Tanzanian Indigenous (Ufipa Breed) and Commercial Broiler (Ross Chicken) Meat on the Physicochemical Characteristics, Collagen and Nucleic Acid Contents. Food Sci. Anim. Resour. (2022) 42:833–848. doi: 10.5851/kosfa.2022.e35
Keywords: body weight, SOD, purine, correlation, indigenous chickens
Citation: Tantiyasawasdikul V, Chomchuen K, Loengbudnark W, Chankitisakul V and Boonkum W (2023) Comparative study and relationship analysis between purine content, uric acid, superoxide dismutase, and growth traits in purebred and crossbred Thai native chickens. Front. Vet. Sci. 10:1263829. doi: 10.3389/fvets.2023.1263829
Edited by:
Abdulmojeed Yakubu, Nasarawa State University, NigeriaReviewed by:
Teketay Anley, Oregon Health and Science University, United StatesGbolabo Olaitan Onasanya, Federal University, Dutse, Nigeria
Copyright © 2023 Tantiyasawasdikul, Chomchuen, Loengbudnark, Chankitisakul and Boonkum. This is an open-access article distributed under the terms of the Creative Commons Attribution License (CC BY). The use, distribution or reproduction in other forums is permitted, provided the original author(s) and the copyright owner(s) are credited and that the original publication in this journal is cited, in accordance with accepted academic practice. No use, distribution or reproduction is permitted which does not comply with these terms.
*Correspondence: Wuttigrai Boonkum, d3V0dGJvQGtrdS5hYy50aA==