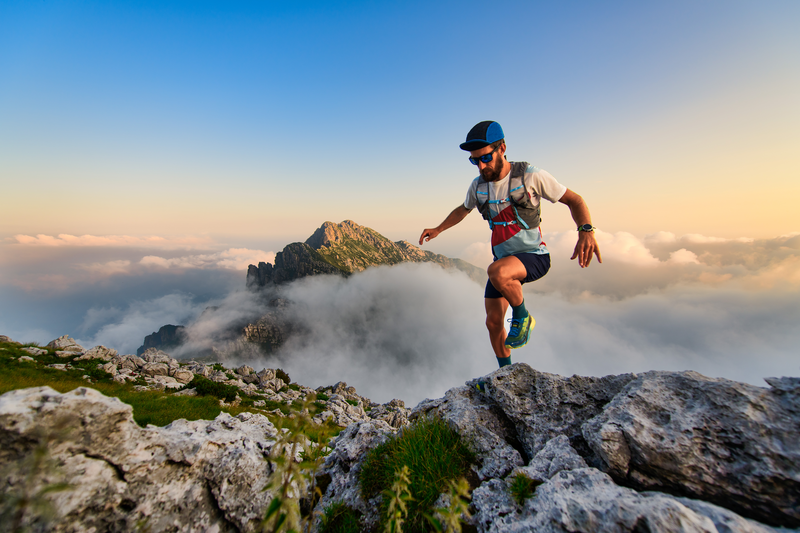
94% of researchers rate our articles as excellent or good
Learn more about the work of our research integrity team to safeguard the quality of each article we publish.
Find out more
ORIGINAL RESEARCH article
Front. Vet. Sci. , 16 November 2023
Sec. Animal Nutrition and Metabolism
Volume 10 - 2023 | https://doi.org/10.3389/fvets.2023.1256903
This article is part of the Research Topic Gut Microbiota: Allied with Livestock Nutrition, Health, And Welfare View all 19 articles
Objective: The objective of this study was to compare the effects of Leymus chinensis hay and alfalfa hay as the roughage on the rumen bacterial and the meat metabolomics in lambs.
Methods: Fourteen male lambs were randomly assigned to two dietary treatments (one group was fed with concentrate and Leymus chinensis hay; another was fed with concentrate and alfalfa hay) with seven replicates per treatment. The feeding experiment lasted for 60 days. Lambs were slaughtered at the end of the feeding experiment. Growth performance, carcass performance, and weights of various viscera were determined. The longissimus dorsi and rumen contents were collected for untargeted metabolomics and 16S rDNA amplicon sequencing analysis, respectively.
Results: The lambs fed with alfalfa hay showed a significantly increased in average daily gain, carcass weight, dressing percentage, loin-eye area, and kidney weight. Feeding Leymus chinensis hay and alfalfa hay diets resulted in different meat metabolite deposition and rumen bacterial communities in the lambs. The relative abundance of phyla Fibrobacteres, Bacteroidetes, and Spirochaetes were greater in the Leymus Chinensis hay group, while, the relative abundance of Firmicutes, Proteobacteria, Fusobacteria, and Verrucomicrobia were greater in the alfalfa hay group. Based on untargeted metabolomics, the main altered metabolic pathways included alanine, aspartate and glutamate metabolism, D-glutamine and D-glutamate metabolism, phenylalanine metabolism, nitrogen metabolism, and tyrosine metabolism. Several bacteria genera including BF31, Alistipes, Faecalibacterium, Eggerthella, and Anaeroplasma were significantly correlated with growth performance and meat metabolites.
Conclusion: Alfalfa hay improved growth performance and carcass characteristics in lambs. Leymus chinensis hay and alfalfa hay caused different meat metabolite deposition by modifying the rumen bacterial community. These findings will be beneficial to future forage utilization for sheep growth, carcass performance, and meat quality improvement.
Mutton is one of the most widely consumed meats around the world due to its high protein and low cholesterol (1). With the growing requirement for high-quality meat, meat quality has induced more and more attention. Improving animal diets is one of the most effective ways to ameliorate animal growth performance, carcass traits, and meat quality (2, 3). Roughage is a necessary nutrient source for ruminants. In particular, types and quality of forage are key factors affecting ruminant productivity, carcass composition, rumen microbiota, and quality of meat nutrition such as amino acids, fatty acids, and mineral elements (4, 5).
Leymus chinensis, a perennial species of Gramineae, is widely distributed in the Eurasian Steppe including the eastern Inner Mongolian Plateau and the Songnen Plain in China (6). Leymus chinensis has been one of the main forages due to its high yield, appropriate nutritional values, and palatability (7). It has been reported that adding Leymus chinensis hay in the diet to replace part of the corn silage and alfalfa hay facilitated the improvement of milk yield, milk fat and protein yield, and milk fat concentration (8). Enhancing the ratio of Leymus chinensis silage decreased dry matter and neutral detergent fiber degradability but promoted crude protein degradability in the combinations of Leymus chinensis silage and corn silage in beef cattle (7). Moreover, the Leymus chinensis hay diet increased the C15:0 fatty acid contents in lamb meat compared to the mixed forage diet (5). As the average daily gain (ADG) of ewes was higher in the Leymus chinensis hay treatment group, Leymus chinensis hay was of better quality for ewes than Vigna radiata stalk (9).
Alfalfa hay is famous for its high quality and widely used as an important dietary roughage for ruminants. Feeding with alfalfa hay increased the growth performance in lambs, such as the ADG, compared with wheat straw diets (10). Feeding lactating ewes with alfalfa hay promoted milk production compared with the wheat straw diets (10). A forage diet mixture of alfalfa hay and maize stover in a ratio of 60:40 optimized the growth and carcass traits of lambs (11). Additionally, alfalfa hay as an ingredient to supplement the low-energy diet increased omega-3 fatty acids and lowered the omega-6: omega-3 ratio in lamb meat (12), indicating that alfalfa affects the meat metabolites and nutritional quality.
The rumen is a complex ecosystem containing functional microbiota responsible for the rumen fermentation and an important part in producing nutrient substances and calories in ruminants (13, 14). The ruminal bacteria community is closely correlated with diets (15). Interestingly, altering the diet has a cascading effect on the rumen microbiota, which affects animal growth performance and meat quality (15). Previous reports have revealed that various forages have a great effect on rumen and fecal microbiota composition (16–18). For example, lambs fed with alfalfa had a higher relative abundance of Akkermansia and Asteroleplasma than the mix of purple prairie clover and alfalfa treatment group (18). The alfalfa hay diet elevated the proportion of Prevotella and Selenomonas compared with the cornstalk diet, while cornstalk feeding increased the proportion of Thermoactimoyces, Bacillus, Papillibacter, Anaerotruncus, and Streptomyces compared with the Leymus chinensis hay or alfalfa hay feeding in dairy cows (19). It has also been revealed associations between the rumen bacterial community with metabolite deposition. For instance, Bacteroidales_UCG-001_norank was negatively related to fatty acids including C18:2 and C20:4 in the longissimus dorsi (LD) of sheep (20). Amino acids including isoleucine and glycine were positively correlated with Anaeroplasma and negatively associated with Parabacteroides and Alloprevotella (21). Moryella was positively associated with fatty acids such as C16:0 and C18:1n9c, and negatively related to C20:4 n6, C20:3 n6, and C20:5 in lamb lumborum muscle (22). Moryella also exhibited a positive relationship with meat metabolites including L-carnosine, N-acetyl-L-histidine, and negatively related to N-acetylaspartylglutamate, L-carnitine, L-citrulline, and Pro-Glu (22). Therefore, it is important to characterize the relationship between rumen bacteria and meat metabolites.
To our knowledge, the comparison of growth performance, carcass characteristics, and meat metabolites of sheep fed with Leymus chinensis hay and alfalfa hay remains poorly defined. The alterations in the rumen bacterial community and interactions between bacteria and metabolites are also lacking. The lack of in-depth evaluation may affect the application of forages in ruminant farming. Therefore, this study focused on investigating the effects of Leymus chinensis hay and alfalfa hay on growth and carcass traits, meat metabolite deposition, and rumen bacterial community in lambs. This study will facilitate assessing growth and carcass performance, and meat quality of sheep fed with forages different forage types, and provide an important reference for improving ruminant growth and carcass performance through manipulating diets in the future.
The animal protocol in this study was approved by the Animal Care and Use Committee of Inner Mongolia University (Approval No. IMU-sheep-2020-041).
Healthy male East Friesian × Small-tail Han lambs were purchased from Inner Mongolia Lark Biotechnology Co., Ltd. Fourteen lambs with an average age of 60 days and with body weight of about 22.03 ± 1.08 kg were selected. The feeding trials were conducted at the Inner Mongolia University Meat Sheep Nutritional Base, where each lamb was housed individually in the same pens. Fourteen lambs were randomly allocated to two dietary treatment groups: one group was feed with concentrate and Leymus chinensis hay (Lc group); another was fed with concentrate and alfalfa (Medicago sativa) hay (Ms group) with free access to water. The Lc group consumed about 325 g of concentrate and 803 g of (Leymus chinensis hay each sheep per day, while the Ms group consumed about 325 g of concentrate and 925 g of alfalfa hay each sheep per day. Ingredient and chemical compositions of diets are shown in Supplementary Table S1. Before the experiment, lambs were acclimatized to the environment for about 1 week. The experiment lasted for 60 days. At the end of the experiment, lambs were selected for slaughter after fasting for about 12 h, and the LD and rumen contents samples were collected. Muscle samples were collected using a disposable scalpel at the same location in the LD of each lamb into 50 mL RNAase-free centrifuge tubes and stored at −80°C for metabolite analysis. After the rumen was opened, the rumen contents were filtered using 4 layers of sterile gauze, and the rumen contents were collected in a 50 mL RNAase-free centrifuge tube and stored at −80°C for 16S rDNA amplicon analysis.
Dry matter and crushed ash of Leymus chinensis hay and alfalfa hay samples were determined according to Ran et al. (23). Crude protein, acid detergent fiber, neutral detergent fiber, and crude fat in the Leymus chinensis hay and alfalfa hay samples were determined using an Automatic Kjeldahl Protein/Nitrogen Analyzer (K1160, Hanon Advanced Technology Group, China), Automatic Fiber Analyser (F2000, Hanon Advanced Technology Group, China) and Automatic Soxhlet Extractor (SOX606, Hanon Advanced Technology Group, China) respectively with reference to Shi et al. (24). The content of mineral elements was determined with using microwave (REVO, LabTech, China) and inductively coupled plasma-optical emission spectrometer (PQ 9000, analytikjena, German) (25).
Body weight and carcass weight were measured following overnight fasting and evisceration, respectively. Loin-eye area between the 12th and 13th ribs was traced on sulphate papers and calculated (26). Dressing percentage = carcass weight/body weight. Organ index = organ weight/body weight (27). Total weight = final body weight (FBW) − initial body weight (IBW). Average daily gain (ADG) = total weight gain/total days. Feed to gain ratio (F/G) = average daily feed intake (ADFI)/ADG.
The rumen contents were collected and stored at −80°C. Microbial DNA was extracted using a TIANGEN kit. The V3–V4 region of the bacterial 16S rDNA gene was amplified by PCR (95°C for 3 min, followed by 30 cycles at 95°C for 30 s, 50°C for 30 s, 72°C for 45 s, and an extension at 72°C for 10 min) using the F3 (ACTCCTACGGGAGGCAGCAG) and R4 (GGACTACHVGGGTWTCTAAT) primer pair (28). DNA Library Prep Kit for Illumina following manufacturer’s recommendations and index codes were added. High throughput sequencing was performed utilizing the Illumina MiSeq PE300 platform to detect the 16S rDNA amplicons according to standard protocols.
After sequencing, paired-end reads from sequencing were merged by FLASH, and low-quality reads were filtered by Trimmomatic. UPARSE was used to align operational taxonomic units (OTUs) at 97% identity. Taxonomy was assigned to OTUs by searching against the Greengenes database version 13.8. α- and β- diversities were calculated using QIIME2. The biomarkers with statistical differences were screened by Linear discriminant Effect Size (LEfSe) analysis, and the screening criteria were LDA threshold ≥4.0 and p-value <0.05. The redundancy analysis (RDA) and Spearman’s rank correlation analysis were performed using the R packages: vegan and pheatmap, respectively.
Untargeted metabolomics analysis was referenced to the previous method (29, 30). Briefly, 200 mg LD samples were mixed with 2-chlorophenylalanine (4 ppm) methanol (−20°C) and ground by a high-throughput tissue grinder for 90 s at 60 Hz. The samples were centrifuged at 4°C for 10 min at 12,000 rpm, and the supernatant was filtered through 0.22 μm membrane to obtain the prepared samples for LC-MS. Twenty microlitre from each sample were taken to the quality control samples, and the rest of the samples were used for LC-MS detection.
LC-MS detection was referenced against the previous method (31). Chromatographic separation was accomplished in a Thermo Ultimate 3000 system equipped with an ACQUITY UPLC® HSS T3 column maintained at 40°C. The temperature of the autosampler was 8°C. Gradient elution of analytes was carried out with 0.1% formic acid in water (C) and 0.1% formic acid in acetonitrile (D) or 5 mM ammonium formate in water (A) and acetonitrile (B) at a flow rate of 0.25 mL/min. Injection of 2 μL of each sample was performed after equilibration. An increasing linear gradient of solvent B (v/v) was used as follows: 0–1 min, 2% B/D; 1–9 min, 2%–50% B/D; 9–12 min, 50%–98% B/D; 12–13.5 min, 98% B/D; 13.5–14 min, 98% B/D; 14–20 min, 2% D-positive model (14–17 min, 2% B-negative model) (31).
The ESI-MSn experiments were executed on the Thermo Q Exactive Focus mass spectrometer with the spray voltage of 3.8 kV and −2.5 kV in positive and negative modes, respectively. Sheath gas and auxiliary gas were set at 30 and 10 arbitrary units, respectively. The capillary column temperature was 325°C. The analyzer scanned over a mass range of m/z 81–1,000 for a full scan at a mass resolution of 70,000. Data-dependent acquisition (DDA) MS/MS experiments were performed with an HCD scan. The normalized collision energy was 30 eV. Raw data were converted to the mzXML format by Proteowizard (v3.0.8789), and then processed by the MetaboAnalystR package (32).
GraphPad Prism was used for statistical analysis. The results of growth performance, carcass performance, and organ index are presented as means ± standard error (SE). All data were considered statistically significant at p < 0.05. Statistical significance of organ index, growth and carcass performance, and metabolites were determined by t-test. The statistical significance of α-diversity was performed with Wilcoxon tests.
To investigate the effects of Leymus chinensis hay and alfalfa hay on growth and carcass performance, we first compared IBW, FBW, ADG, carcass weight, and loin-eye area between the two groups. IBW was not significantly distinct, while total weight gain, FBW, and ADG were significantly greater in the Ms. group. Carcass weight, dressing percentage, and loin-eye area were also obviously increased in the Ms. group. However, lambs in the Ms. group had higher ADFI. The F/G was similar between the two groups (Table 1). In addition, we analyzed the organ index in lambs. Kidney index was increased in the Ms. group, while others including spleen, lung, liver, and heart index were not significantly affected (Table 2). These data indicated that compared to Leymus chinensis hay, alfalfa hay improved growth and carcass traits in lambs.
Table 1. Effects of Leymus chinensis hay and alfalfa hay on growth and carcass performance of sheep (n = 7).
To investigate the effects of Lemus chinensis hay and alfalfa hay on rumen microbiota, we performed and analyzed bacterial 16S rDNA sequencing of rumen contents between two groups. The observed OTU and Shannon curves reached the saturation phase, suggesting the sufficient and reliable sequence depth captured in this study (Figure 1A). A total of 2,140 OTUs were identified in both groups, among which 3,491 and 3,861 specific OTUs were observed in the Lc and Ms. groups, respectively (Figure 1B). Shannon and Simpson indexes reflected the bacterial community richness and diversity. No significant differences were observed in the Shannon and Simpson index between the two groups (Figure 1C). The β-diversity presenting as Principal Co-ordinates Analysis (PCoA), Non-metric multidimensional scaling (NMDS), Principal Components Analysis (PCA), and Partial Least Squares Discrimination Analysis (PLS-DA) was further applied to analyze the variation of bacterial structure. The NMDS, PCA, and PLS-DA are in Supplementary Figure S3. The Lc group rumen contents (LcRc) formed a different rumen bacterial community clustered separately from the Ms group rumen contents (MsRc) (Figure 1D).
Figure 1. The diversity of the rumen microbiota of lambs fed a diet with Lemus chinensis hay and alfalfa hay. (A) Observed OTUs and Shannon curves of rumen microbiota. (B) The Venn diagram illustrates the overlap of microbial OTUs between the two groups. (C) The α-diversity includes Shannon and Simpson index. (D) The β-diversity presents as PCoA. n = 7 in each group. LcRc and MsRc indicate rumen contents from lambs fed with Leymus chinensis hay and alfalfa hay, respectively.
To further define the composition of rumen microbiota in the LcRc and MsRc, the percentages of bacterial community abundance were analyzed at phylum and genus levels. At the phylum level, Bacteroidetes, Firmicutes, Spirochaetes, and Fibrobacteres were the dominant phyla (Figure 2A). The relative abundance of Fibrobacteres, Bacteroidetes, and Spirochaetes were obviously greater in the Lc group (Supplementary Table S2); the relative abundance of Firmicutes, Actinobacteria, Fusobacteria, Verrucomicrobia, and Proteobacteria were obviously greater in the MsRc (Supplementary Table S2). At the genus level, the top 20 genera were present with relatively high abundance (Figure 2B). The 17 genera significantly altered between two groups, among which only 5 genera, i.e., Clostridiu, Fibrobacter, Selenomonas, BF311, and Treponema, were greater in the LcRc. Most of the altered bacteria in the MsRc, including Moryella, Blautia, p_75_a5, Faecalibacterium, Mogibacteriaceae, Anaerostipes, Bifidobacterium, Eggerthella, and Collinsella belong to Firmicutes and Actinobacteria (Supplementary Table S3).
Figure 2. The composition of the rumen microbiota of lambs fed a diet with Lemus chinensis hay and alfalfa hay. Taxonomic distribution of rumen bacterial communities of different groups at phylum level (A) and genus level (B). LcRc and MsRc indicate rumen contents from lambs fed with Leymus chinensis hay and alfalfa hay, respectively. “p_” denotes phylum-level classification, “f_” denotes phylum-level classification, and “g_” denotes genus-level classification.
The rumen bacterial composition was further compared through LEfSe analysis with 4.0 as the threshold on the LDA score to identify specific species in each group. The constitution of rumen bacteria changed between LcRc and MsRc. A total of 17 taxa sequences were enriched in the LcRc and mainly belonged to Fibrobacteres, Bacteroidetes, and Spirochaetes (Figure 3). A total of 7 rumen bacteria mainly belonging to Firmicute were determined as enriched in the MsRc (Figure 3). Taken together, lambs in Lc and Ms. groups had distinct compositions of rumen bacterial community, which may lead to different growth performance, carcass performance, and metabolic profiles.
Figure 3. The effect of Lemus chinensis hay and alfalfa hay on the taxonomic diversity of the rumen bacteria. The cladogram (A) and histogram (B) show rumen bacterial taxa with a linear discriminant analysis (LDA) score >4.0 by LEfSe analysis. LcRc and MsRc indicate rumen contents from lambs fed with Leymus chinensis hay and alfalfa hay, respectively.
To further investigate the potential relationship between rumen microbiota variation and ADG, carcass weight, and body weight, we performed the RDA and Spearman’s correlation between differential rumen bacteria and host parameters. According to RDA, the Lemus chinensis hay and alfalfa hay treatments were distinct and two different clusters were observed. ADG, carcass weight, and body weight showed a significant correlation with bacterial community (Figures 4A,B). The Spearman’s correlation data showed that the relative abundance of BF311 and Pseudobutyrivibrio were negatively related to ADG, carcass weight, and body weight (Figure 4C). Additionally, most genus-level bacteria such as Enterococcus, Lactobacillus, Acidaminococcus, and Moryella belonging to Firmicutes showed a strongly positive correlation with ADG, carcass weight, and body weight (Figures 4C). Alistipes belonging to Bacteroidetes, Eggerthella and Bifidobacterium belonging to Bacteroidetes, and Anaeroplasma belonging to Tenericutes, were also positively associated with ADG, carcass weight, and body weight (Figure 4C).
Figure 4. Relationship between key rumen bacteria with ADG, carcass weight, and body weight. (A,B) RDA was performed on genus-level taxonomic profile and host parameters including ADG, carcass weight, and body weight. Arrows indicate the correlation between community structure and host parameters. (C) Correlation analysis of key rumen microbiota with ADG, carcass weight, and body weight. Statistical significance was calculated by Spearman’s correlation analysis. *p ≤ 0.05, **p ≤ 0.01, ***p ≤ 0.001.
To define similarities and differences between lamb meat fed with Leymus chinensis hay and alfalfa hay, we performed and analyzed the untargeted metabolome profiles of LD samples. PCA score plots with positive and negative ionization modes revealed no significant clustering of the two treatment groups (Figure 5A), but further analysis revealed significant differences in metabolite composition. These metabolites mainly consisted of peptides, lipids, organic acids, steroids, carbohydrates, nucleic acids, vitamins and cofactors, hormones, and transmitters (Figure 5B). These two comparisons identified 96 significant differential metabolites (67 in the positive mode and 29 in the negative mode), among which 52 and 44 differential metabolites were elevated in the Lc and Ms. groups, respectively. In detail, organic acid and derivatives including N-Acetyl-L-leucine, Pantetheine, Cysteinyl glycine, Alanyl tyrosine, and Ala-Ile were greater in the Ms group longissimus dorsi (MsLD), while Cinnamoyl glycine, L-arginosuccinate, and Glu-Thr were greater in the Lc group longissimus dorsi (LcLD). Lipids and lipid-like molecules such as 2-methylbutyroylcarnitine, Glycoursodeoxycholic acid, and 2-Hydroxymyristic acid were higher in the LcLD, while Hexadecanediouc acid, prostaglandin F2alpha, and tetradecanedioic acid were higher in the MsLD. Interestingly, LcLD contained higher organoheterocyclic compounds including Momocrotaline, (+)-alpha-lipoic acid, Methyl indole-3-acetate, benzenoids (i.e., hippuric acid), phenylpropanoids, and polyketides (i.e., 2-phenylpropionic acid). MsLD had a higher relative abundance of alkaloids and derivates including ecgonine methyl ester (Figures 5C,D). Between Leymus chinensis hay and alfalfa hay treatment, peptides including Ala-Ile, Glu-Thr, N-Acetyl-Asp-Glu, gamma-glutamyl-cysteine, and gamma-glutamyl-L-leucine, significantly changed (Figures 5C,D). Furthermore, based on the pathway analysis, we found that these differential metabolites were mainly enriched in alanine, aspartate and glutamate metabolism, D-glutamine and D-glutamate metabolism, phenylalanine metabolism, nitrogen metabolism, and tyrosine metabolism (Figures 6A,B). Changed metabolites in these pathways were shown in Table 3, indicating that different forages affected amino acid composition and contents, and its metabolism, leading to various meat nutritional qualities.
Figure 5. Effects of Lemus chinensis hay and alfalfa hay on metabolites of LD in lambs. (A) Scatter plots of the PCA model based on identified metabolite features. (B) Composition of metabolites in LcLD and MsLD. (C) Volcano plot of differential metabolites between LcLD and MsLD. (D) Boxplot of selected differential metabolites. n = 7 in each group. *p ≤ 0.05, **p ≤ 0.01, ***p ≤ 0.001. LcLD and MsLD indicate LD from lambs fed with Leymus chinensis hay and alfalfa hay, respectively.
Figure 6. Pathway analysis of differential metabolites. (A) Enriched KEGG pathways of the comparison between LcLD and MsLD. (B) Pathway enrichment and topology analysis.
Table 3. KEGG pathway enrichment of the changed metabolites for LcLD vs. MsLD.a
To facilitate the identification of potential biomarkers between the two groups, PLS-DA and OPLS-DA were applied to identify the major differences in metabolites. PLS-DA score plots showed the best separation of LcLD and MsLD samples (Supplementary Figure S2A). Several potential biomarkers with VIP >2 including Monocrotaline, Hippuric acid, 6-Hydroxytestosterone, N-Acetyl-L-leucine, LysoPE 18:0, Ala-Ile, Methadone-d9, Cysteinylglycine, Cinnamoylglycine, Pilocarpine, and 2-Phenylpropionic acid and were highlighted in the volcano plots (Supplementary Figure S2B). To minimize overfitting in the PLS-DA model, we further explored potential markers using OPLS-DA between groups. The analysis presented a cross-validated score plot (R2X = 0.311, R2Y = 0.993, Q2 = 0.737, p-value = 0.01) of the discriminating model between LcLD and MsLD in positive ionization mode. The cross-validated score plot (R2X = 0.454, R2Y = 0.995, Q2 = 0.526, p-value = 0.05) was shown in negative ionization mode. Identified biomarkers were the same as those identified by PLS-DA as having a trend toward being distinct between the two groups (Supplementary Figure S3). Additionally, random forest showed top differential metabolites including N-Acetyl-L-leucine, Hippuric acid, (+)-alpha-lipoic acid, and 2-Phenylpropionic acid (Supplementary Figure S4).
Differential metabolites and potential biomarkers have been clarified as mentioned above. The correlation of potential biomarkers with growth performance was further analyzed. A total of 30 differential metabolites were significantly associated with ADG, carcass weight, and body weight. Among them, 14 metabolites including N-Acetyl-L-leucine, Methadone-d9, Hexadecanedioic acid, and alanyltyrosine were positively related to ADG, carcass weight, and body weight. Sixteen metabolites such as LysoPE 18:0, Cinnamoylglycine, and 2-Methylbutyroylcarnitine exhibited a negative relationship with growth performance (Figure 7).
Figure 7. Correlation analysis of differential metabolites with ADG, carcass weight, and body weight. Statistical significance was calculated by Spearman’s correlation analysis. *p ≤ 0.05, **p ≤ 0.01, ***p ≤ 0.001.
The 16S rDNA sequencing revealed that lambs with the consumption of dietary Leymus chinensis hay and alfalfa hay exhibited distinct rumen microbiota communities. The metabolomics analysis indicated that exposure to Leymus chinensis hay and alfalfa hay resulted in different metabolic profiles of LD. Therefore, we explored the correlations between the rumen microbiota and the altered LD metabolites through Spearman’s correlation analysis, revealing high correlations between key rumen microbiota with differential metabolites (Figure 8). In detail, Fibrobacter, Selenomonas, BF311, Treponema, and Clostridium exhibited a strong positive relationship with organic acid and derivatives such as Glu-Thr, benzenoids including hippuric acid, phenylpropanoids and polyketides including 2-phenylpropionic acid, and organoheterocyclic compounds including Methyl indole-3-acetate, Momocrotaline, and (+)-alpha-lipoic acid. And they were negatively associated with other differential metabolites including Ala-Ile, N-Acetyl-L-leucine, Tetradecanedioic acid, and Methadone-d9. Other genera including Bifidobacterium, Faecalibacterium, Blautia, Roseburia, Moryella, Streptococcus, [Ruminococcus], and Odoribacter, showed an obviously opposite correlation trend with the five genera mentioned above (Figure 8). These results indicated that the rumen bacterial compositions obviously and specifically impacted host LD metabolites.
Figure 8. Correlation analysis of key rumen microbiota with differential metabolites. Statistical significance was calculated by Spearman’s correlation analysis. *p ≤ 0.05, **p ≤ 0.01, ***p ≤ 0.001.
The forage is one of the main factor affecting ruminant production, rumen microbiota community, and meat quality. Previous studies explored ratios of various forages such as alfalfa hay, Leymus chinensis hay, Vigna radiata stalk, and corn stalk, which had a greater effect on the ruminants (5, 9, 12). To our knowledge, few studies have systematically compared the growth and carcass traits of lambs fed Leymus chinensis hay and alfalfa hay. In this study, we found that feeding with alfalfa hay increased lamb carcass weight, FBW, ADG, loin-eye area, and dressing percentage compared to those in the Lc group, supporting that those lambs fed with the alfalfa hay exhibited better carcass characteristics and growth performance.
The composition and diversity of rumen bacteria exhibit a close relationship with the host. Diet is one of the key factors that triggers changes in rumen microbial communities, alongside different environmental factors (33). To investigate the impacts of Leymus chinensis hay and alfalfa hay on the host rumen bacterial community, 16S rDNA amplicon sequencing was performed and analyzed. Obviously, there was no significant difference in Shannon and Simpson indexes in the LcRc and MsRc. This indicates no significant altered in the diversity of rumen bacteria.
Exploring changes in rumen microbiota, which are closely related to muscle metabolites, can help to investigate the mechanisms for improving lamb meat quality under different roughage conditions. At the phylum level, Firmicutes and Bacteroidetes were the major bacterial phyla in the rumen as reported (20, 22), whose relative abundances were significantly distinct in the LcRc and MsRc. Firmicutes are dominant across rumen bacterial communities and consist of various fibrolytic and cellulolytic bacterial genera (34). Bacteroidetes are beneficial for digesting carbohydrates (35). Then, we analyzed differences in key rumen bacteria at the genus level between the two groups and their correlation with metabolite deposition in the LD. Unspecified bacteroidales and Prevotella were the dominant bacterial genera in both groups. Based on the LEfSe analysis, lambs in the Lc group have a higher relative abundance of Prevotella, which plays an important role in polysaccharide and protein metabolism, and utilization of hemicelluloses (20). Fibrobacter was also higher in the LcRc, which is a cellulolytic bacteria in the rumen of ruminants (36). This may be related to the higher fiber intake of lambs in the Lc group, while we found that they positively regulate Glu-Thr levels in the LD. Dipeptides are important flavor precursors in lamb meat (37), suggesting that rumen microorganisms may influence lamb meat quality by regulating flavor substances in muscle after feeding Leymus chinensis hay. It has been reported that Fibrobacter was able to synthesize oligosaccharides and contributed to rapidly adapting to sudden environmental changes (33). In addition, three genera mainly belonging to the fibrolytic bacteria, such as Selenomonas, BF311, and Treponema were obviously elevated in the LcRc. Treponema is closely related to pectin treatments due to its ability to degrade pectin, which shares mutual interaction with Fibrobacter (33, 38). Selenomonas can oxidize lactate and produce propionic acid as a main fermentation product (39). And the correlation between these three fibrolytic bacteria and the metabolite levels of the LD had the same trend as Fibrobacter, suggesting that they may have the same mechanism of action. Most of the significant differential bacteria, such as Bifidobacterium, Faecalibacterium, Mogibacterium, Anaerostipes, Alistipes, and Blautia, were elevated in the MsRc. A previous study has shown that Bifidobacterium is saccharolytic bacteria can generate acetate and lactate (10). Faecalibacterium is one of the butyrate-producing bacteria linked to higher weight gain, and displays anti-inflammatory action (40). Anaerostipes is also a butyrate-producing bacterium (41). In the present study also the relative abundance of Faecalibacterium was found to be positively correlated with body weight and carcass weight. Changes in Mogibacterium are associated with feed efficiency in ruminants (42). Additionally, Blautia has been proven to enhance beneficial anti-inflammatory effects of hosts (43). These results suggested that alfalfa hay may have altered feed efficiency, influenced volatile fatty acid production, and also facilitated rumen resistance to inflammation. Taken together, the forage types, i.e., Leymus chinensis hay and alfalfa hay, resulted in different rumen bacterial compositions of lambs.
The compositions of nutrient metabolites have a direct impact on meat quality (44). Thus, meat metabolic profiles aroused great public concern. Amino acids are important ingredients for the nutritional value of mutton (45). According to the analysis of untargeted metabolomics, we found significant differences in amino acid-related metabolites in meat between Lc and Ms. groups. Some amino acid metabolism, including alanine, aspartate, and glutamate metabolism, phenylalanine metabolism, D-glutamine, and D-glutamate metabolism, and tyrosine metabolism were changed, suggesting forages regulated amino acid contents and metabolism, and thus affected the nutritional quality of meat. Free amino acids and dipeptides are important flavor precursors in meat (37). Importantly, some peptides have bioactive properties (37). Cysteinylglycine belonging to the dipeptide is a structural component of glutathione (46), which was higher in the MsLD. Between Leymus chinensis hay and alfalfa hay treatment, peptides including Ala-Ile, Glu-Thr, N-Acetyl-Asp-Glu, gamma-glutamyl-cysteine and gamma-glutamyl-L-leucine changed significantly. The gamma-glutamyl peptide, gamma-glutamyl-L-leucine, has been recognized as a kokumi-imparting molecule (47). Another gamma-glutamyl peptide, gamma-glutamyl-cysteine, can activate the calcium-sensing receptor and then impart the kokumi peptide-induced responses (48).
Rumen microorganisms provide energy for muscle metabolism to the host mainly in the form of volatile fatty acids (49). Feeding Leymus chinensis hay and alfalfa hay not only altered the substrates available for rumen fermentation but may have also altered the distribution of various fatty acids in the rumen, thereby affecting the composition of the rumen microbiota and the levels of LD muscle metabolites (50). For example, Du et al. (51) found that Ruminiclostridium_6 and U29-B03 might be participating in carbohydrate metabolism to produce volatile fatty acids, which promote IMF deposition affecting tenderness in muscles. The significant changes in the relative abundance of the associated bacteria in the Lc and Ms. groups in the present study may similarly cause alterations in the levels of metabolites in muscle via the volatile fatty acid pathway. However, more research would be required to reveal the connection between different roughages and changes in rumen microbiota and muscle metabolites.
Compared to lamb with the Leymus chinensis hay diet, lambs fed with alfalfa hay exhibited better growth performance and carcass performance. Leymus chinensis hay led to the enrichment of the genera Fibrobacter, Treponema, Selenomonas, and BF311. Alfalfa hay led to the enrichment of Blautia, Anaerostipes, Faecelibacterium, Alistipes, Bifidobacterium, and other genera. Ruminal bacteria after feeding different roughages affect the quality of lamb meat by influencing the metabolism of several amino acids and polypeptides in lamb meat. It was also demonstrated that alanine, aspartate and glutamate metabolism, D-glutamine and D-glutamate metabolism, phenylalanine metabolism, nitrogen metabolism, and tyrosine metabolism were the key metabolic pathways involved after feeding Leymus chinensis hay and alfalfa hay. In addition, conjoint analysis of rumen microbes and metabolomics indicated a close relationship between rumen microbial composition and muscle metabolites. These results have important significance for the future adequate and rational utilization of pasture to improve the quality of lamb meat.
The datasets presented in this study can be found in online repositories. The names of the repository/repositories and accession number(s) can be found at: https://www.ncbi.nlm.nih.gov/bioproject; PRJNA995904.
The animal study was approved by Animal Care and Use Committee of Inner Mongolia University (Approval No. IMU-sheep-2020-041). The study was conducted in accordance with the local legislation and institutional requirements.
HW: Writing – original draft. LiM: Data curation, Writing – review & editing. LaM: Writing – review & editing, Data curation.
The author(s) declare financial support was received for the research, authorship, and/or publication of this article. This work was supported by the Inner Mongolia Autonomous Region Department of Science and Technology (zdzx2018016 and zdzx2018065), and the Grassland talent innovative team of Inner Mongolia Autonomous Region of China (12000-12102621).
We express our gratitude to the diligent and committed researchers at our laboratories for their unwavering dedication and hard work. Additionally, we extend our sincere appreciation to all individuals who have contributed to the realization of this thesis.
The authors declare that the research was conducted in the absence of any commercial or financial relationships that could be construed as a potential conflict of interest.
All claims expressed in this article are solely those of the authors and do not necessarily represent those of their affiliated organizations, or those of the publisher, the editors and the reviewers. Any product that may be evaluated in this article, or claim that may be made by its manufacturer, is not guaranteed or endorsed by the publisher.
The Supplementary material for this article can be found online at: https://www.frontiersin.org/articles/10.3389/fvets.2023.1256903/full#supplementary-material
Lc, Leymus chinensis; Ms, Medicago sativa; LD, Longissimus dorsi; OTUs, Operational taxonomic units; ADG, Average daily gain; FBW, Final body weight; IBW, Initial body weight; F/G, Feed to gain ratio; ADFI, Average daily feed intake.
1. Wang, B, Ma, T, Deng, KD, Jiang, CG, and Diao, QY. Effect of urea supplementation on performance and safety in diets of Dorper crossbred sheep. J Anim Physiol Anim Nutr. (2016) 100:902–10. doi: 10.1111/jpn.12417
2. Qin, X, Zhang, T, Cao, Y, Deng, B, Zhang, J, and Zhao, J. Effects of dietary sea buckthorn pomace supplementation on skeletal muscle mass and meat quality in lambs. Meat Sci. (2020) 166:108141. doi: 10.1016/j.meatsci.2020.108141
3. Knight, MI, Butler, KL, Linden, NP, Burnett, VF, Ball, AJ, McDonagh, MB, et al. Understanding the impact of sire lean meat yield breeding value on carcass composition, meat quality, nutrient and mineral content of Australian lamb. Meat Sci. (2020) 170:108236. doi: 10.1016/j.meatsci.2020.108236
4. Sun, HX, Zhong, RZ, Liu, HW, Wang, ML, Sun, JY, and Zhou, DW. Meat quality, fatty acid composition of tissue and gastrointestinal content, and antioxidant status of lamb fed seed of a halophyte (Suaeda glauca). Meat Sci. (2015) 100:10–6. doi: 10.1016/j.meatsci.2014.09.005
5. Zhang, XQ, Jiang, C, Jin, YM, Li, P, and Zhong, JF. The effect of substitution of mixed grass hay with Urtica cannabina hay and/or Leymus chinensis hay on blood biochemical profile, carcass traits, and intramuscular fatty acid composition in finishing lambs. Anim Feed Sci Technol. (2021) 272:114780. doi: 10.1016/j.anifeedsci.2020.114780
6. Chen, S, Huang, X, Yan, X, Liang, Y, Wang, Y, Li, X, et al. Transcriptome analysis in sheepgrass (Leymus chinensis): a dominant perennial grass of the Eurasian Steppe. PLoS One. (2013) 8:e67974. doi: 10.1371/journal.pone.0067974
7. Zhang, X, Wang, H, You, W, Zhao, H, Wei, C, Jin, Q, et al. In vitro degradability of corn silage and Leymus chinensis silage and evaluation of their mixed ratios on performance, digestion and serum parameters in beef cattle. J Anim Physiol Anim Nutr. (2020) 104:1628–36. doi: 10.1111/jpn.13392
8. Yan, R, Chen, S, Zhang, X, Han, J, Zhang, Y, and Undersander, D. Short communication: effects of replacing part of corn silage and alfalfa hay with Leymus chinensis hay on milk production and composition. J Dairy Sci. (2011) 94:3605–8. doi: 10.3168/jds.2010-3536
9. Yang, Z, Wang, Y, Yuan, X, Wang, L, and Wang, D. Forage intake and weight gain of ewes is affected by roughage mixes during winter in northeastern China. Anim Sci J. (2017) 88:1058–65. doi: 10.1111/asj.12747
10. Obeidat, BS, Subih, HS, Taylor, JB, and Obeidat, MD. Alfalfa hay improves nursing performance of Awassi ewes and performance of growing lambs when used as a source of forage compared with wheat straw. Trop Anim Health Prod. (2019) 51:581–8. doi: 10.1007/s11250-018-1735-z
11. Sun, L, Yin, Q, Gentu, G, Xue, YL, Hou, ML, Liu, LY, et al. Feeding forage mixtures of alfalfa hay and maize stover optimizes growth performance and carcass characteristics of lambs. Anim Sci J. (2018) 89:359–66. doi: 10.1111/asj.12928
12. Ponnampalam, EN, Dunshea, FR, and Warner, RD. Use of lucerne hay in ruminant feeds to improve animal productivity, meat nutritional value and meat preservation under a more variable climate. Meat Sci. (2020) 170:108235. doi: 10.1016/j.meatsci.2020.108235
13. Morgavi, DP, Rathahao-Paris, E, Popova, M, Boccard, J, Nielsen, KF, and Boudra, H. Rumen microbial communities influence metabolic phenotypes in lambs. Front Microbiol. (2015) 6:1060. doi: 10.3389/fmicb.2015.01060
14. Vasta, V, Yáñez-Ruiz, DR, Mele, M, Serra, A, Luciano, G, Lanza, M, et al. Bacterial and protozoal communities and fatty acid profile in the rumen of sheep fed a diet containing added tannins. Appl Environ Microbiol. (2010) 76:2549–55. doi: 10.1128/AEM.02583-09
15. Zened, A, Combes, S, Cauquil, L, Mariette, J, Klopp, C, Bouchez, O, et al. Microbial ecology of the rumen evaluated by 454 Gs Flx pyrosequencing is affected by starch and oil supplementation of diets. FEMS Microbiol Ecol. (2013) 83:504–14. doi: 10.1111/1574-6941.12011
16. Petri, RM, Forster, RJ, Yang, W, McKinnon, JJ, and McAllister, TA. Characterization of rumen bacterial diversity and fermentation parameters in concentrate fed cattle with and without forage. J Appl Microbiol. (2012) 112:1152–62. doi: 10.1111/j.1365-2672.2012.05295.x
17. Huws, SA, Lee, MRF, Muetzel, SM, Scott, MB, Wallace, RJ, and Scollan, ND. Forage type and fish oil cause shifts in rumen bacterial diversity. FEMS Microbiol Ecol. (2010) 73:396–407. doi: 10.1111/j.1574-6941.2010.00892.x
18. Huang, QQ, Holman, DB, Alexander, T, Hu, TM, Jin, L, Xu, ZJ, et al. Fecal microbiota of lambs fed purple prairie clover (Dalea purpurea vent.) and alfalfa (Medicago sativa). Arch Microbiol. (2018) 200:137–45. doi: 10.1007/s00203-017-1427-5
19. Zhang, RY, Zhu, WY, Zhu, W, Liu, JX, and Mao, SY. Effect of dietary forage sources on rumen microbiota, rumen fermentation and biogenic amines in dairy cows. J Sci Food Agr. (2014) 94:1886–95. doi: 10.1002/jsfa.6508
20. Wang, B, Luo, Y, Wang, Y, Wang, D, Hou, Y, Yao, D, et al. Rumen bacteria and meat fatty acid composition of Sunit sheep reared under different feeding regimens in China. J Sci Food Agric. (2021) 101:1100–10. doi: 10.1002/jsfa.10720
21. Qu, W, Nie, C, Zhao, J, Ou, X, Zhang, Y, Yang, S, et al. Microbiome-metabolomics analysis of the impacts of long-term dietary advanced-glycation-end-product consumption on C57bl/6 mouse fecal microbiota and metabolites. J Agric Food Chem. (2018) 66:8864–75. doi: 10.1021/acs.jafc.8b01466
22. Wang, B, Wang, Y, Zuo, S, Peng, S, Wang, Z, Zhang, Y, et al. Untargeted and targeted metabolomics profiling of muscle reveals enhanced meat quality in artificial pasture grazing tan lambs via rescheduling the rumen bacterial community. J Agric Food Chem. (2021) 69:846–58. doi: 10.1021/acs.jafc.0c06427
23. Ran, T, Saleem, AM, Beauchemin, KA, Penner, GB, and Yang, W. Processing index of barley grain and dietary undigested neutral detergent Fiber concentration affected chewing behavior, ruminal pH, and total tract nutrient digestibility of heifers fed a high-grain diet. J Anim Sci. (2021) 99:skab011. doi: 10.1093/jas/skab011
24. Shi, B, He, W, Su, G, Xu, X, and Shan, A. The effect of increasing neutral detergent Fiber level through different fiber feed ingredients throughout the gestation of sows. Animals. (2021) 11:415. doi: 10.3390/ani11020415
25. Rubio-Armendáriz, C, Gutiérrez, ÁJ, Gomes-Furtado, V, González-Weller, D, Revert, C, Hardisson, A, et al. Essential metals and trace elements in cereals and their derivatives commercialized and consumed in Cape Verde. Biol Trace Elem Res. (2023) 201:444–54. doi: 10.1007/s12011-022-03158-x
26. Xiang, J, Zhong, L, Luo, H, Meng, L, Dong, Y, Qi, Z, et al. A comparative analysis of carcass and meat traits, and rumen bacteria between Chinese Mongolian sheep and Dorper × Chinese Mongolian crossbred sheep. Animal. (2022) 16:100503. doi: 10.1016/j.animal.2022.100503
27. Fan, L, He, ZZ, Ao, X, Sun, WL, Xiao, X, Zeng, FK, et al. Effects of residual superdoses of phytase on growth performance, tibia mineralization, and relative organ weight in ducks fed phosphorus-deficient diets. Poult Sci. (2019) 98:3926–36. doi: 10.3382/ps/pez114
28. Takahashi, S, Tomita, J, Nishioka, K, Hisada, T, and Nishijima, M. Development of a prokaryotic universal primer for simultaneous analysis of bacteria and archaea using next-generation sequencing. PLoS One. (2014) 9:e105592. doi: 10.1371/journal.pone.0105592
29. Ponnusamy, K, Choi, JN, Kim, J, Lee, SY, and Lee, CH. Microbial community and metabolomic comparison of irritable bowel syndrome faeces. J Med Microbiol. (2011) 60:817–27. doi: 10.1099/jmm.0.028126-0
30. Sangster, T, Major, H, Plumb, R, Wilson, AJ, and Wilson, ID. A pragmatic and readily implemented quality control strategy for Hplc-Ms and Gc-Ms-based metabonomic analysis. Analyst. (2006) 131:1075–8. doi: 10.1039/b604498k
31. Want, EJ, Wilson, ID, Gika, H, Theodoridis, G, Plumb, RS, Shockcor, J, et al. Global metabolic profiling procedures for urine using Uplc-Ms. Nat Protoc. (2010) 5:1005–18. doi: 10.1038/nprot.2010.50
32. Chong, J, and Xia, J. Metaboanalystr: an R package for flexible and reproducible analysis of metabolomics data. Bioinformatics. (2018) 34:4313–4. doi: 10.1093/bioinformatics/bty528
33. Xie, X, Yang, C, Guan, LL, Wang, J, Xue, M, and Liu, JX. Persistence of cellulolytic bacteria Fibrobacter and Treponema after short-term corn stover-based dietary intervention reveals the potential to improve rumen Fibrolytic function. Front Microbiol. (2018) 9:1363. doi: 10.3389/fmicb.2018.01363
34. Evans, NJ, Brown, JM, Murray, RD, Getty, B, Birtles, RJ, Hart, CA, et al. Characterization of novel bovine gastrointestinal tract Treponema isolates and comparison with bovine digital dermatitis Treponemes. Appl Environ Microbiol. (2011) 77:138–47. doi: 10.1128/AEM.00993-10
35. Spence, C, Wells, WG, and Smith, CJ. Characterization of the primary starch utilization operon in the obligate anaerobe Bacteroides fragilis: regulation by carbon source and oxygen. J Bacteriol. (2006) 188:4663–72. doi: 10.1128/JB.00125-06
36. Qi, M, Nelson, KE, Daugherty, SC, Nelson, WC, Hance, IR, Morrison, M, et al. Genomic differences between Fibrobacter succinogenes S85 and Fibrobacter intestinalis Dr7, identified by suppression subtractive hybridization. Appl Environ Microbiol. (2008) 74:987–93. doi: 10.1128/Aem.02514-07
37. Kaczmarska, K, Taylor, M, Piyasiri, U, and Frank, D. Flavor and metabolite profiles of meat, meat substitutes, and traditional plant-based high-protein food products available in Australia. Foods. (2021) 10:801. doi: 10.3390/foods10040801
38. Liu, J, Pu, YY, Xie, Q, Wang, JK, and Liu, JX. Pectin induces an in vitro rumen microbial population shift attributed to the pectinolytic Treponema group. Curr Microbiol. (2015) 70:67–74. doi: 10.1007/s00284-014-0672-y
39. Vidra, A, and Nemeth, A. Bio-produced propionic acid: a review. Period Polytech Chem Eng. (2018) 62:57–67. doi: 10.3311/PPch.10805
40. Oikonomou, G, Teixeira, AG, Foditsch, C, Bicalho, ML, Machado, VS, and Bicalho, RC. Fecal microbial diversity in pre-weaned dairy calves as described by pyrosequencing of metagenomic 16s rDNA. Associations of Faecalibacterium species with health and growth. PLoS One. (2013) 8:e63157. doi: 10.1371/journal.pone.0063157
41. Xie, F, Xu, L, Wang, Y, and Mao, S. Metagenomic sequencing reveals that high-grain feeding alters the composition and metabolism of cecal microbiota and induces cecal mucosal injury in sheep. mSystems. (2021) 6:e0091521. doi: 10.1128/mSystems.00915-21
42. McLoughlin, S, Spillane, C, Claffey, N, Smith, PE, O’Rourke, T, Diskin, MG, et al. Rumen microbiome composition is altered in sheep divergent in feed efficiency. Front Microbiol. (2020) 11:1981. doi: 10.3389/fmicb.2020.01981
43. Jenq, RR, Taur, Y, Devlin, SM, Ponce, DM, Goldberg, JD, Ahr, KF, et al. Intestinal Blautia is associated with reduced death from graft-versus-host disease. Biol Blood Marrow Transplant. (2015) 21:1373–83. doi: 10.1016/j.bbmt.2015.04.016
44. Huang, Y, Zhou, L, Zhang, J, Liu, X, Zhang, Y, Cai, L, et al. A large-scale comparison of meat quality and intramuscular fatty acid composition among three Chinese indigenous pig breeds. Meat Sci. (2020) 168:108182. doi: 10.1016/j.meatsci.2020.108182
45. Cai, Z-W, Zhao, X-F, Jiang, X-L, Yao, Y-C, Zhao, C-J, Xu, N-Y, et al. Comparison of muscle amino acid and fatty acid composition of castrated and uncastrated male pigs at different slaughter ages (2010) Ital J Anim Sci. 9, e33. doi: 10.4081/ijas.2010.e33
46. Oestreicher, J, and Morgan, B. Glutathione: subcellular distribution and membrane transport1. Biochem Cell Biol. (2019) 97:270–89. doi: 10.1139/bcb-2018-0189
47. Lee, YC, Chi, MC, Lin, MG, Chen, YY, Lin, LL, and Wang, TF. Biocatalytic synthesis of γ-glutamyl-L-leucine, a Kokumi-Imparting Dipeptide, by Bacillus licheniformis γ-Glutamyltranspeptidase. Food Biotechnol. (2018) 32:130–47. doi: 10.1080/08905436.2018.1444636
48. Ohsu, T, Amino, Y, Nagasaki, H, Yamanaka, T, Takeshita, S, Hatanaka, T, et al. Involvement of the calcium-sensing receptor in human taste perception. J Biol Chem. (2010) 285:1016–22. doi: 10.1074/jbc.M109.029165
49. Nathani, NM, Patel, AK, Mootapally, CS, Reddy, B, Shah, SV, Lunagaria, PM, et al. Effect of roughage on rumen microbiota composition in the efficient feed converter and sturdy Indian Jaffrabadi Buffalo (Bubalus bubalis). BMC Genomics. (2015) 16:1116. doi: 10.1186/s12864-015-2340-4
50. Ghimire, S, Kohn, RA, Gregorini, P, White, RR, and Hanigan, MD. Representing interconversions among volatile fatty acids in the Molly cow model. J Dairy Sci. (2017) 100:3658–71. doi: 10.3168/jds.2016-11858
Keywords: alfalfa hay, growth performance, lamb, Leymus chinensis hay, metabolomics, rumen bacteria
Citation: Wang H, Meng L and Mi L (2023) Effects of Leymus chinensis hay and alfalfa hay on growth performance, rumen microbiota, and untargeted metabolomics of meat in lambs. Front. Vet. Sci. 10:1256903. doi: 10.3389/fvets.2023.1256903
Received: 11 July 2023; Accepted: 24 October 2023;
Published: 16 November 2023.
Edited by:
Wen-Chao Liu, Guangdong Ocean University, ChinaReviewed by:
Maghsoud Besharati, University of Tabriz, IranCopyright © 2023 Wang, Meng and Mi. This is an open-access article distributed under the terms of the Creative Commons Attribution License (CC BY). The use, distribution or reproduction in other forums is permitted, provided the original author(s) and the copyright owner(s) are credited and that the original publication in this journal is cited, in accordance with accepted academic practice. No use, distribution or reproduction is permitted which does not comply with these terms.
*Correspondence: Lan Mi, bGFubWlfOTBAMTI2LmNvbQ==
†These authors have contributed equally to this work and share first authorship
Disclaimer: All claims expressed in this article are solely those of the authors and do not necessarily represent those of their affiliated organizations, or those of the publisher, the editors and the reviewers. Any product that may be evaluated in this article or claim that may be made by its manufacturer is not guaranteed or endorsed by the publisher.
Research integrity at Frontiers
Learn more about the work of our research integrity team to safeguard the quality of each article we publish.