- 1Cellular Biology Laboratory, Carlos Chagas Institute, Oswaldo Cruz Foundation (FIOCRUZ-PR), Curitiba, Brazil
- 2Auditora Fiscal Federal Agropecuária do Ministério da Agricultura Pecuária e Abastecimento (MAPA), Curitiba, Brazil
- 3Programa de Pós-Graduação Ciências da Saúde, Universidade Federal de São João Del Rei, Divinópolis, Brazil
- 4Trypanosomatid Molecular Biology Laboratory, Carlos Chagas Institute, Oswaldo Cruz Foundation (FIOCRUZ-PR), Curitiba, Brazil
- 5Laboratory for Applied Science and Technology in Health, Carlos Chagas Institute, Oswaldo Cruz Foundation (FIOCRUZ-PR), Curitiba, Brazil
- 6Parana Institute of Molecular Biology, Curitiba, Brazil
Fasciola hepatica can cause problems in both animals and humans. Fasciolosis can be diagnosed through the indirect ELISA immunodiagnostic test. Serological diagnosis of Fasciola is based on recombinant antigens secreted by this worm. We used PubMed and Google Scholar databases to review the published literature on ‘antigens with immunogenic potential’ used in serological tests to identify antibodies against F. hepatica in humans, cattle, and sheep. Studies that investigated diagnostic tests with common reference standards were included in the sensitivity and/or specificity bivariate meta-analysis. In the quality and susceptibility to bias analysis of the 33 included studies, 26 fulfilled at least six (75%) of the eight QUADAS criteria and were considered good-quality papers. We found that most of the studies used native excretory-secretory antigens and recombinant cathepsin in ELISA tests for serological diagnosis of fascioliasis in humans, cattle, and sheep. The meta-analysis revealed that all antigens demonstrated good accuracy. The best results in terms of sensitivity [0.931–2.5% confidence interval (CI) and 0.985–97.5% CI] and specificity (0.959–2.5% CI and 0.997–97.5% CI) were found in human FhES. FhrCL-1, FhES, and FhrSAP-2 antigens gave the best results for the serum diagnosis of human and animal fasciolosis.
1. Introduction
In recent years, there has been a high level of concern worldwide about the incidence of foodborne trematode (FBT) infections. The parasites responsible for this infection include Fasciola hepatica and F. gigantica flatworms, which pose a major problem for animals and humans (1). They have a complex life cycle, using Lymnaeidae snails as an intermediate host, a carrier (aquatic plants), and a final mammalian host (cattle, sheep, or even humans). In humans, this parasitosis is acknowledged to be a (re-)emerging disease in several countries that has spread in close association with climatic conditions (2). Almost 80 species of intestinal flukes infect humans and animals worldwide (3, 4). However, in South America, only F. hepatica has been identified in humans and cattle (3). Fasciolosis is considered an important endemic disease in this part of the American continent (2, 5–7).
Bovine fasciolosis occurs on every continent except Antarctica, and over 700 million animals are estimated to be at risk of infection. The cost to the farming and industry of F. hepatica infection in cattle is estimated to be over 3 billion USD per year worldwide (8, 9). This cost is largely unquantified at national or regional levels, and it has been reported that fluke affects milk yield and carcass composition, prolonging the time required to reach slaughter weight (10–12). It is therefore important to develop methods to identify liver fluke infections.
The gold standard for diagnosing trematode infection involves examining fecal eggs, which can be performed through ether concentration, sedimentation techniques, or the Kato–Katz method. In the case of visceral inspection, the presence of worms in the liver can also be used (13–16). FBT infections are usually diagnosed through imaging, immunodiagnostic, and molecular techniques (humans), as well as parasitological methods (animals). Immunodiagnostic testing commonly includes the indirect enzyme-linked immunosorbent assay (ELISA), indirect hemagglutination, indirect fluorescent antibody test, and intradermal testing. For serological diagnosis, Fasciola ELISA tests use different antigens for antibody detection in humans, sheep, and cattle. These antigens include a series of proteolytic enzymes, such as proteases and glutathione S-transferases, which the parasite uses to survive in the host body (17–19). These enzymes have been implicated in several aspects of helminth development (18).
Initially, serologic tests used to diagnose fasciolosis relied on a somatic antigen (SA) obtained from adult flukes collected from the bile ducts of cows at slaughterhouses (20). This method was less specific than other, more modern tests. Subsequently, ELISA tests were developed to detect antibodies in human and animal sera, using excretory-secretory (ES) Fasciola sp. antigens. These antigens, which are excreted and secreted by liver fluke, are immunogenic and can modulate host immune responses. More recent testing methods have used recombinant Fasciola antigens and ELISA tests have been developed to detect antibodies in human and animal sera (21). Standardization in recombinant protein preparation is important in these cases to increase production. Recombinant antigen production is also more cost-effective than ES preparation (21, 22).
In recent years, a wide range of targeted F. hepatica genes has been chosen, cloned, and produced in various expression host systems (bacteria and yeast) using different expression conditions to achieve an ideal diagnostic test for human fasciolosis. Recombinant saposin-like protein 2 antigen (rSAP-2) (23–25), recombinant leucine aminopeptidase (rLAP) (26), recombinant glutathione S-transferase (27, 28), and recombinant cathepsin L1 (rCL-1) (21, 22, 29) are the most immunodominant antigens. Trematodes secrete a large family of cysteine proteases (30) that include cathepsin L1 (CL-1), cathepsin L2 (CL-2), cathepsin L3 (CL-3), and cathepsin L5 (CL-5) (30, 31).
Proteomic analysis of F. hepatica secretions identified cathepsin L1 enzymes as the main components involved in virulence (32). They can cleave several host substrates in the host blood for parasite feeding, migration through host tissues, formation of eggshells, and excystment (30). Cathepsin proteins can be found in juvenile and adult liver flukes (18, 30). Cathepsin L proteases are the most predominant components of ES antigens, which are used globally as immunodiagnostic tools for diagnosing liver fluke infections in humans and animals (33).
Understanding the role principal proteases involved in F. hepatica host invasion is the first step toward developing serologic diagnostic tests for humans and animals. In the case of humans, lateral flow immunoassay (LFI) tests have already been developed for fasciolosis (33). ELISA and LFI tests use F. hepatica proteins as antigens to identify antibodies in human and animal sera or feces (22, 29, 34–36). We therefore conducted a meta-analysis of the literature using the terms “antigens (native and recombinant) with immunogenic potential” used in serological tests to identify antibodies against F. hepatica in humans, cattle, and sheep. Our principal aims were to evaluate the quality of the selected papers and then perform a meta-analysis to identify the best antigen options.
2. Materials and methods
2.1. Information sources and selection of studies
For the systematic review (SR), the Google Scholar and PubMed databases were used up to November 2022. No restrictions were placed on study publication dates. Chart 1 shows the search strategy, index terms, and inclusion and exclusion criteria used. The references of the chosen publications were also analyzed to identify additional papers. The protocol was included in the PROSPERO registry (ID:412565).
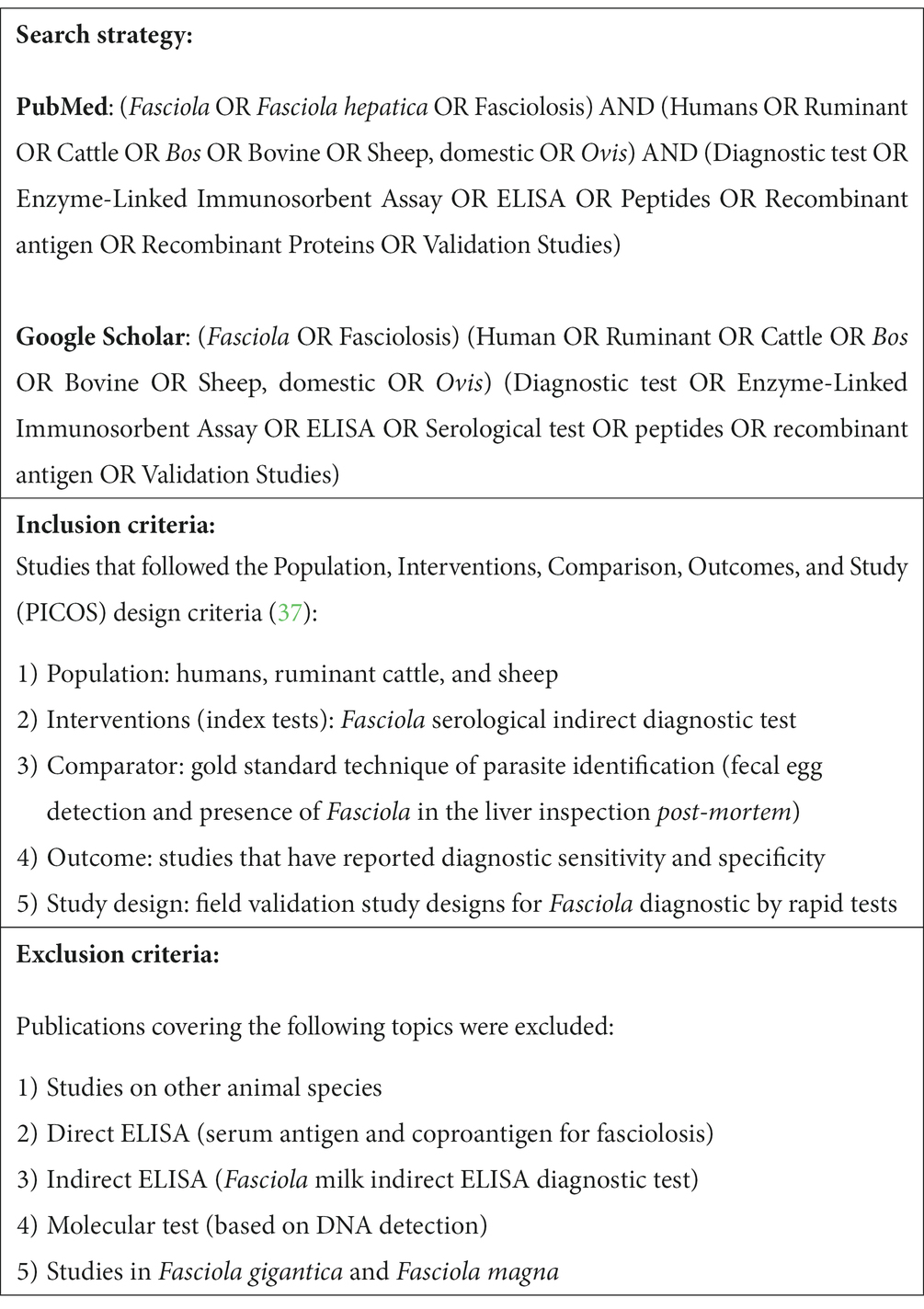
CHART 1. Search strategies and inclusion and exclusion criteria applied in the SR of peptides with immunogenic potential used in serological tests to identify antibodies against F. hepatica in humans and ruminants.
2.2. Evaluation of limitations and potential bias
The Quality Assessment of Diagnostic Accuracy Studies (QUADAS) tool was used to evaluate the publication quality (38, 39). This tool contains 14 criteria, with eight considered applicable to this study (see Chart 2). Three additional questions from the Standards for Reporting of Diagnostic Accuracy (STARD) checklist (40) were included to provide essential information when evaluating epidemiological studies and methods, as suggested by several authors (38, 39). Therefore, the selected articles were read and analyzed using the combination of eight QUADAS and three STARD criteria.
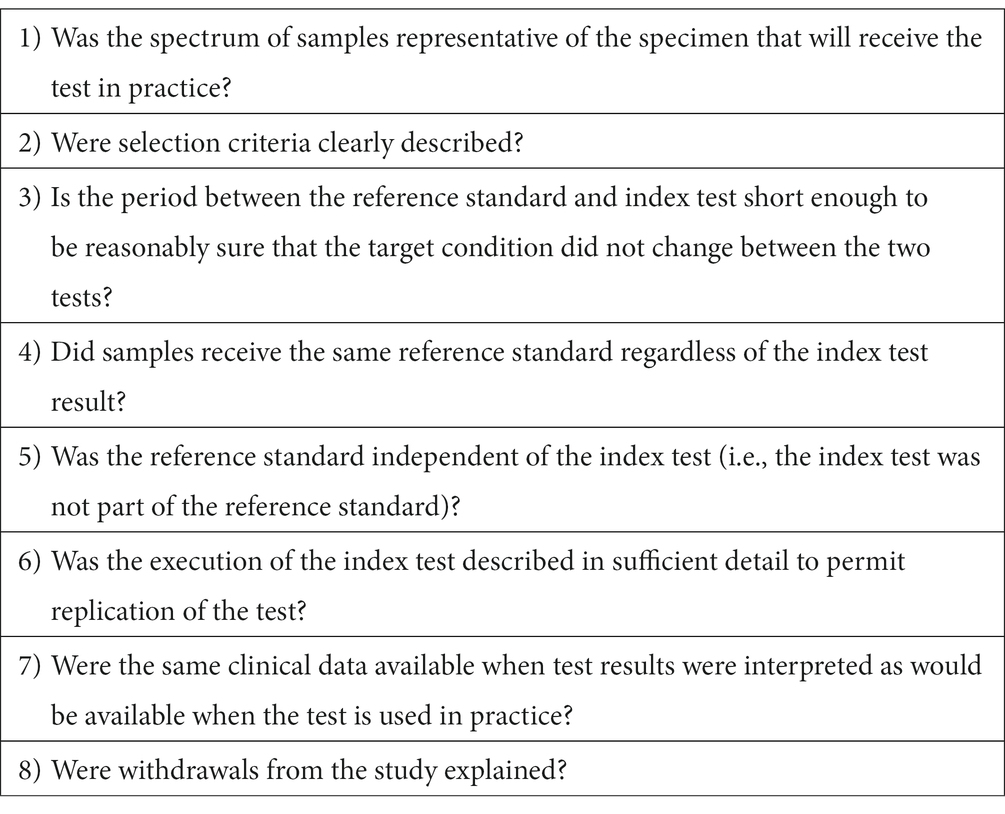
CHART 2. QUADAS criteria for assessing the quality of the studies included in this SR on peptides or recombinant proteins with immunogenic potential used in serological tests to identify antibodies against F. hepatica in humans and ruminants.
The QUADAS and STARD criteria are presented in Charts 2, 3, respectively:
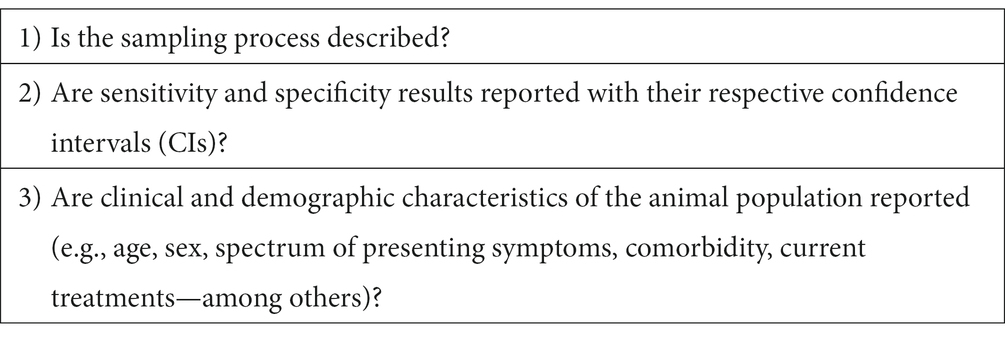
CHART 3. STARD criteria for assessing the quality of the studies included in this SR on peptides or recombinant proteins with immunogenic potential used in serological tests to identify antibodies against F. hepatica in humans and ruminants.
For both analyses, the responses to the questions were categorized as “yes,” “no,” or “unclear.” The quality analysis method followed that described by De Oliveira et al. (38), with some modifications. In the QUADAS analysis, studies that met four to five criteria (corresponding to 50–60% “yes” answers)—were considered to be of “regular” or “good” quality. A cutoff point of 75%, where at least six criteria were met, was used to define a “good” quality study. For STARD, good-quality studies were considered those meeting all three STARD criteria. The quality criteria were applied independently by two researchers—disagreements were resolved by a third reviewer who participated in the analysis of the specific criteria in question.
2.3. Meta-analysis
The articles were organized into 13 groups according to host species and antigen parasite characteristics to verify the possibility of performing a meta-analysis. This included six human groups: humans using F. hepatica excretory-secretory proteins (FhES), human somatic antigens (FhSA), human recombinant ferritin (FhrFtn-1), human tegument-associated protein (FhTP16.5), human recombinant saposin (FhrSAP-2) and recombinant cathepsin (FhrCL-1); two cattle groups: cattle FhES and cattle FhrCL-1; and five sheep groups: sheep FhES, sheep FhSA, sheep FhrCL-1, sheep fatty acid binding recombinant protein (FhrFAB), and sheep glutathione S-transferase recombinant protein (FhrGST). Meta-analyses were performed only if there were more than two eligible studies in each group.
The bivariate binomial random effects model of Chu and Cole (41) was applied to the meta-analysis. Sensitivity and specificity were jointly modeled with the estimates from each study. It was assumed they varied but came from a common underlying distribution with an unstructured between-study covariance matrix (42). All models were fitted without covariates. The hierarchical summary receiver operating characteristic (HSROC) parameters were used to draw the summary receiver operating characteristic (SROC) plots. The percentage of the study weights was calculated using the methodology of Burke et al. (43). Sensitivity analyses were conducted by removing studies assessed as having low quality. This did not result in any significant changes in the pattern of the results obtained.
Considering the number of studies, heterogeneity was assessed by visual inspection of forest plots and confidence intervals (CI) of sensitivity and specificity of primary studies. The analyses were performed in the MetaDTA program (44). Chart 4 lists the papers used for the meta-analysis in each group.
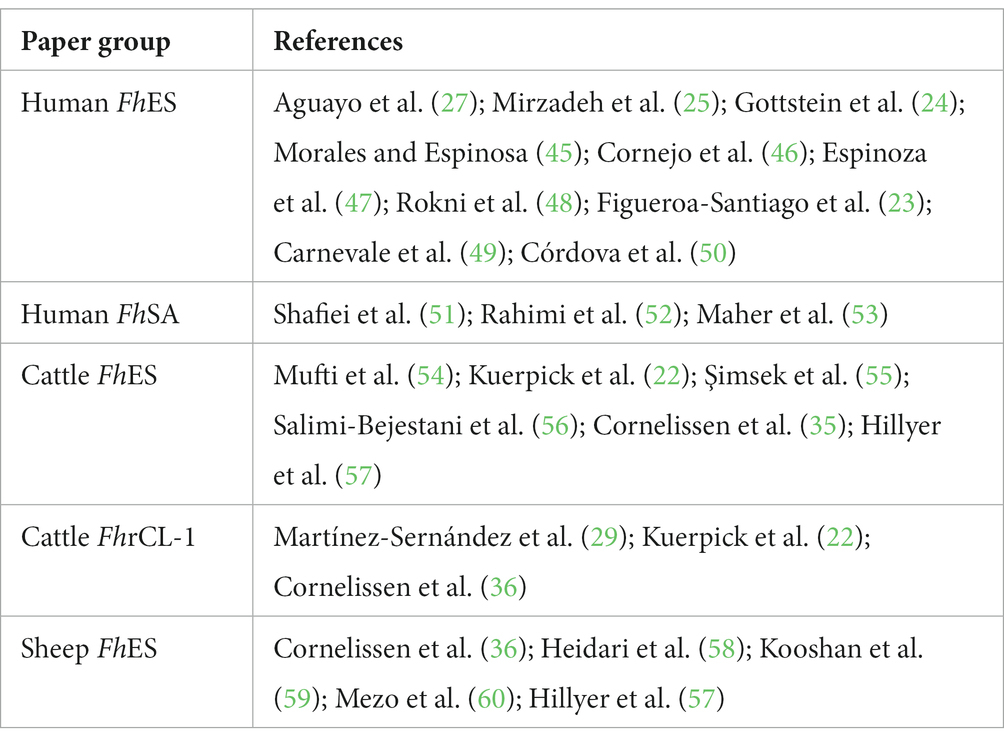
CHART 4. Articles included in meta-analysis performance in relation to sensitivity, specificity, and diagnostic odds ratio by groups, related to host species and antigen parasite characteristics—human Fasciola hepatica excretory-secretory (FhES) proteins and human Fasciola hepatica Somatic Antigen (FhSA); cattle FhES and cattle Fasciola hepatica recombinant Cathepsin L-1 (FhrCL-1); and sheep FhES.
3. Results
The first search yielded 1,073 from PubMed and 1,436 articles from Google Scholar. After reviewing the titles, 612 studies met the inclusion criteria. These papers were analyzed according to the search strategy, and after removing duplicates (93), 519 titles remained, 249 of which were excluded after reading the abstract, leaving 270 papers. Of these, 169 were disregarded as they discussed F. gigantica, and another two studies were excluded for discussing F. magna. Thirty-three papers discussed other animal species, and eight publications could not be accessed online. Of the remaining 58 full-text articles assessed for eligibility, 25 were excluded for not using an ELISA test. Accordingly, 33 studies were included in the qualitative synthesis, and 27 papers were included in the meta-analysis (Figure 1).
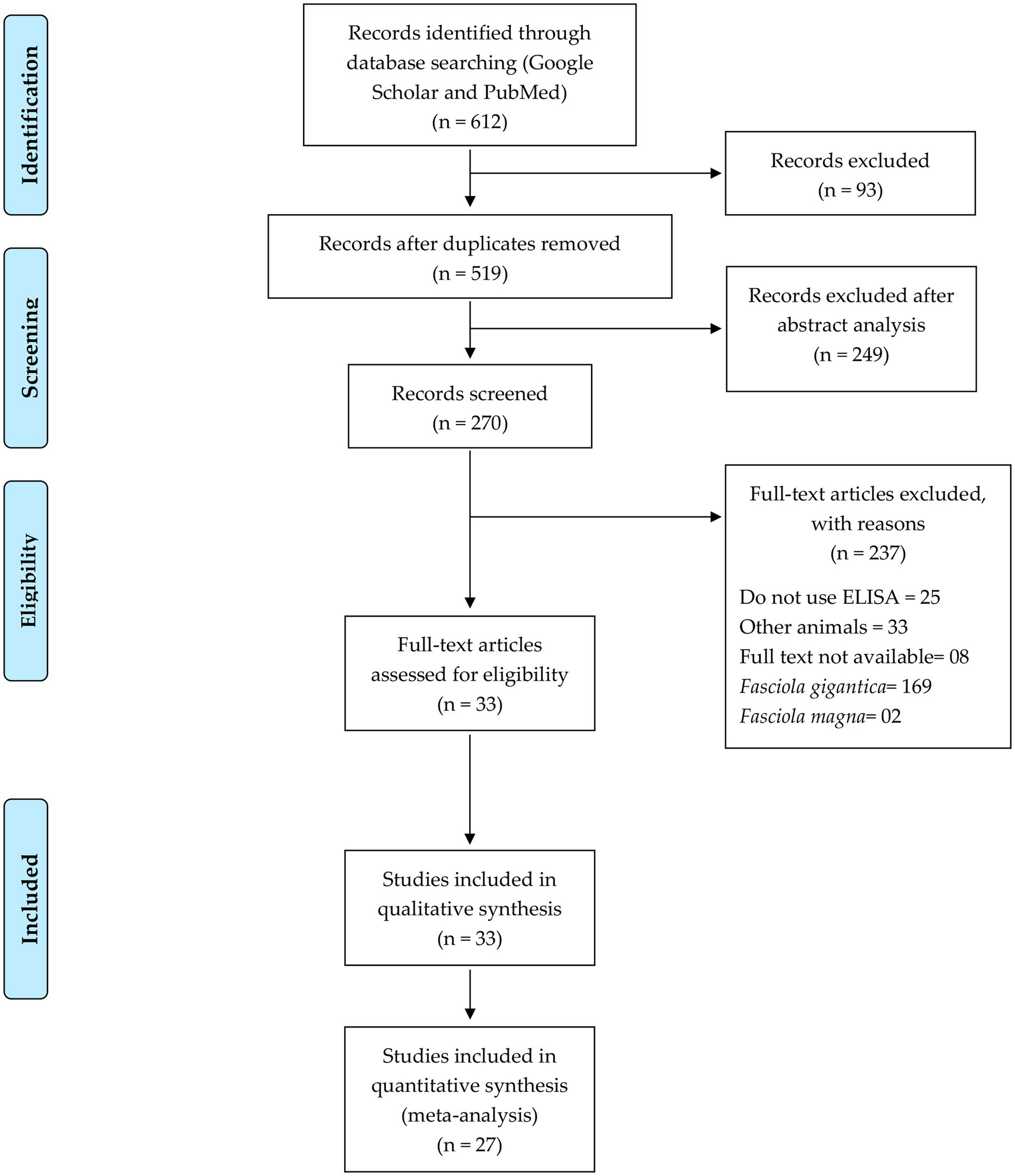
Figure 1. Flow diagram of the study selection process (37).
3.1. Quality assessment of included studies
Twenty-six articles met at least six (75%) of the eight QUADAS criteria and were considered to be good-quality papers. Six articles met 4–5/8 criteria (50–60%) and were categorized as regular studies. Only one reached 4/8 QUADAS criteria, suggesting that it was of lower quality.
For STARD, only two studies met all three criteria and were considered good-quality studies. Fifteen were categorized as having met two STARD criteria, and 16 studies were classified as having met just one STARD criterion (Supplementary Charts S1, S2).
3.2. Antigen target from ELISA
The meta-analysis considered seven native and recombinant antigens used for serological diagnosis of fasciolosis in different animals, including humans. The selected studies showed that the most common antigen used in ELISA tests was FhES. The second most common ones were recombinant cathepsin L1 and recombinant saposin. Other proteins also appeared in this review, such as native SAs of F. hepatica as well as other recombinant antigens, namely recombinant ferritin, fatty acid binding recombinant protein, and glutathione S-transferase recombinant protein.
Most studies focused on humans (20 papers) using FhES, FhrCL-1, and other antigens. Nine articles discussed the FhES antigens for serological diagnosis of human fasciolosis. Several tests targeting antibodies against the previously described FhrCL-1 (n = 3) in humans and cattle have been developed. Other recombinant proteins (FhrFtn-1, FhrFAB, and FhrGST) were also used for serological diagnosis of fasciolosis in humans, cattle and sheep.
The highest sensitivities and specificities for diagnosing fasciolosis were obtained using human samples. Serological ELISA tests that used FhES, FhrCL-1, FhSA, and FhrSAP-2 proteins gave similar results for humans, cattle, and sheep. False positives were reported for all antigens (native and recombinant) and were not linked to any particular parasitic infection. No cross-reaction was reported for any antigen (native or recombinant) for any of the analyzed species.
3.3. Meta-analysis results
Of the 13 groups, only five contained more than two studies that could be used for meta-analysis. These were human FhSA and FhES, cattle FhES and FhrCL-1, and sheep FhES (Supplementary Chart S3).
Tables 1–5 show the sensitivity, specificity, and diagnostic odds ratio (DOR) values combined in the bivariate meta-analyses by paper group. For each group, SROC plots, forest plots of sensitivity, and forest plots of specificity (Figures 2–5) are presented, except for the FhSA and cattle FhrCL-1 groups, for which no SROC plots could be produced, as only they only contained three papers each.
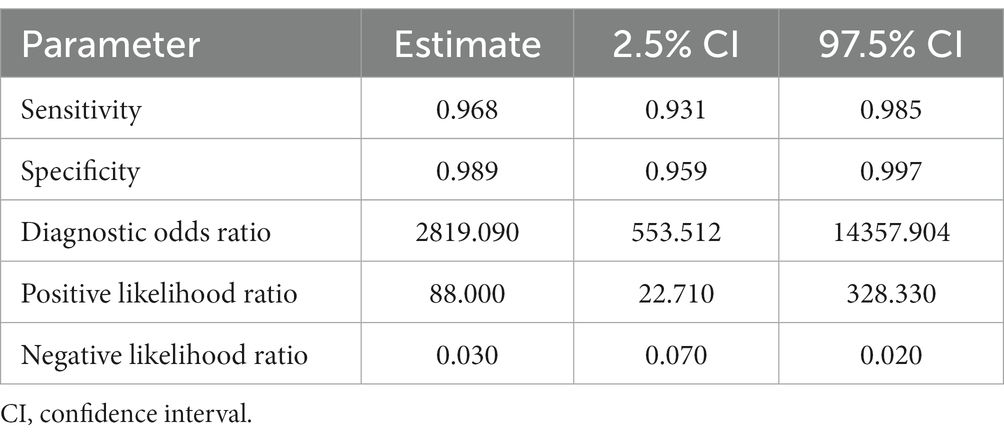
Table 1. Sensitivity, specificity, and diagnostic odds ratio values combined in the bivariate meta-analyses for human FhES proteins paper group.
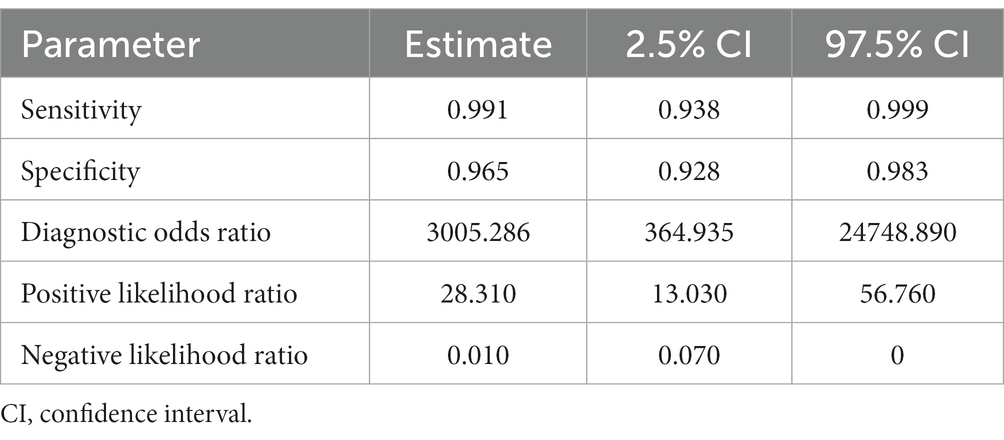
Table 2. Sensitivity, specificity, and diagnostic odds ratio values combined in the bivariate meta-analyses for human FhSA proteins paper group.
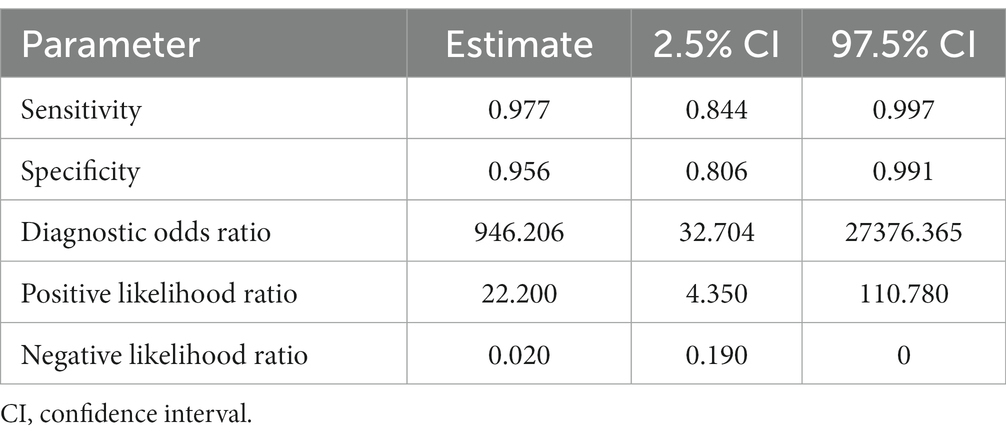
Table 3. Sensitivity, specificity, and diagnostic odds ratio values combined in the bivariate meta-analyses for cattle FhES proteins paper group.
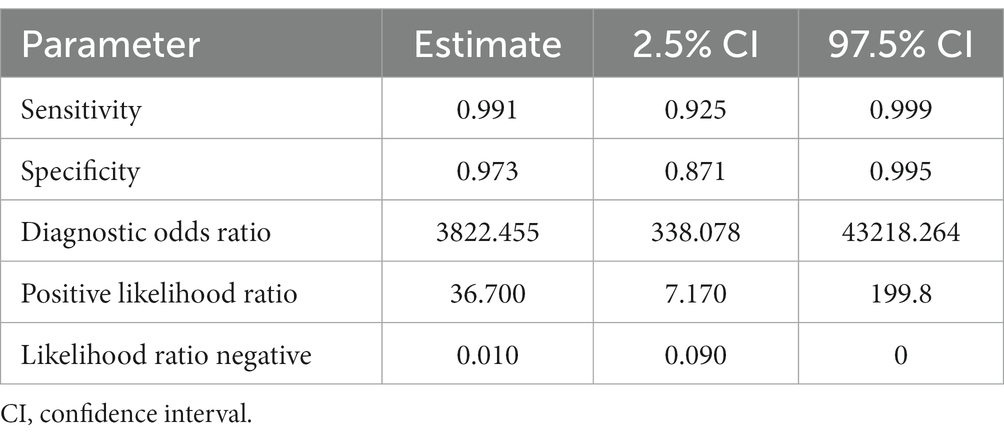
Table 4. Sensitivity, specificity, and diagnostic odds ratio values combined in the bivariate meta-analyses for cattle FhrCL-1 proteins paper group.
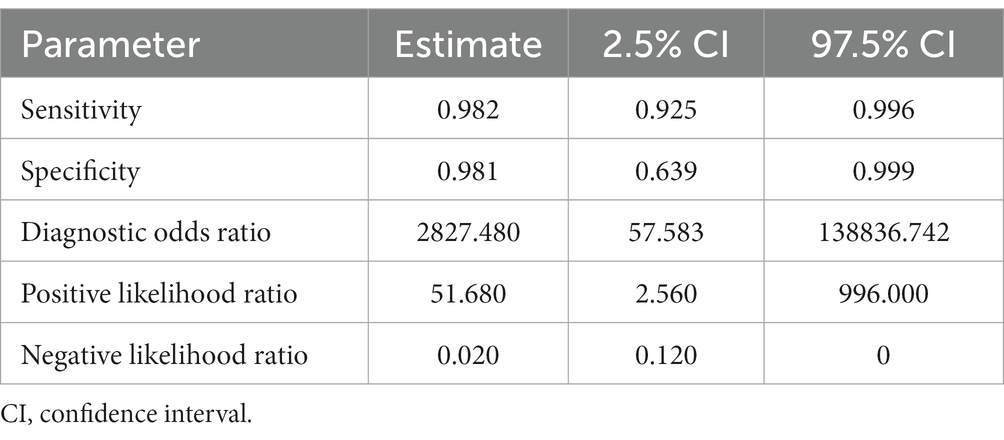
Table 5. Sensitivity, specificity, and diagnostic odds ratio values combined in the bivariate meta-analyses for sheep FhES proteins paper group.
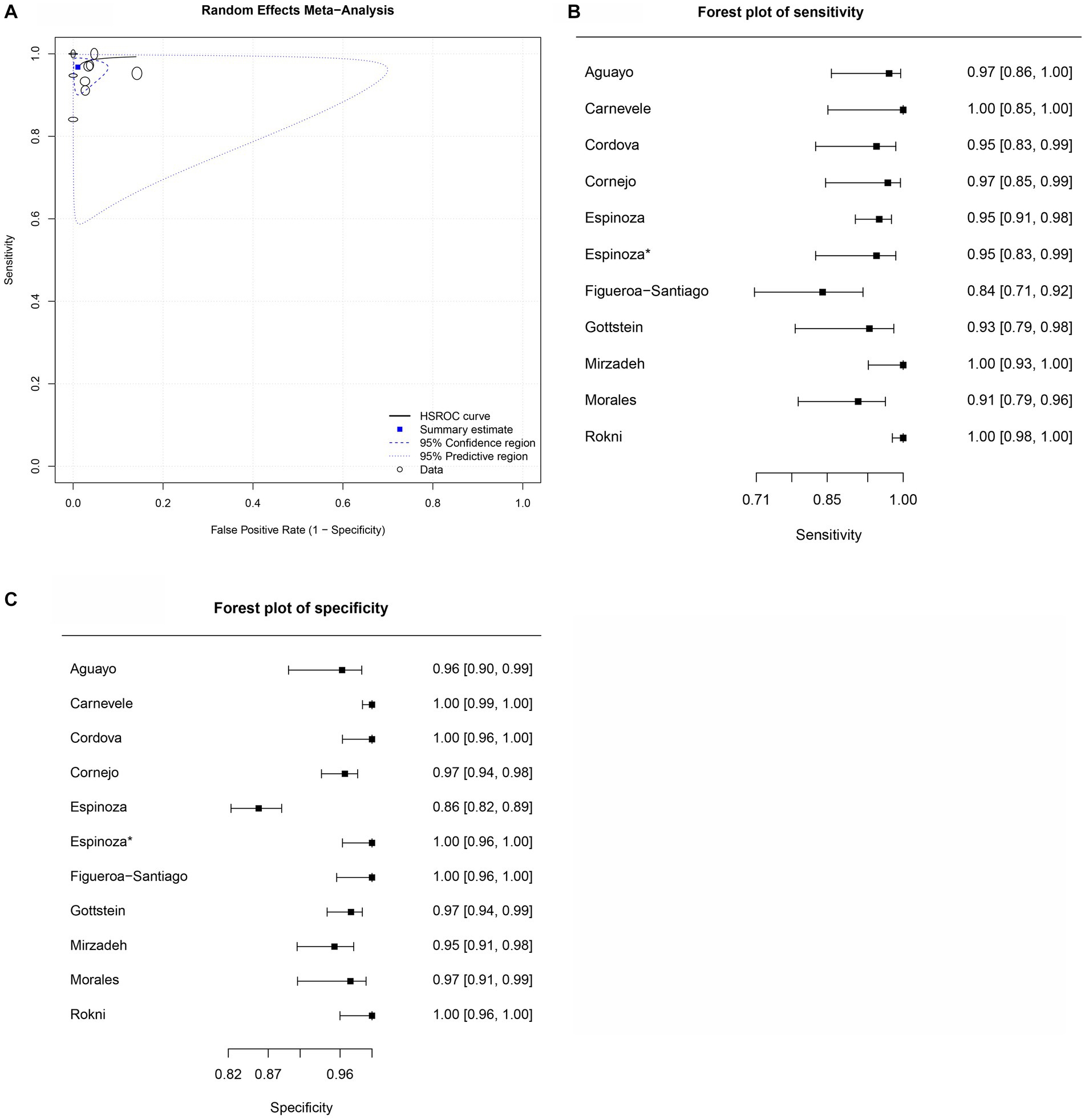
Figure 2. Forest plots for the human FhES proteins paper group. (A) Random effects meta-analysis for human excretory-secretory proteins paper group. The size of the circles determines the weight of the study. (B) Forest plot sensitivity for human excretory-secretory proteins paper group. *This corresponds to a second approach by Espinosa et al. (47), in the same article, in field research. (C) Forest plot specificity for human excretory-secretory proteins paper group.
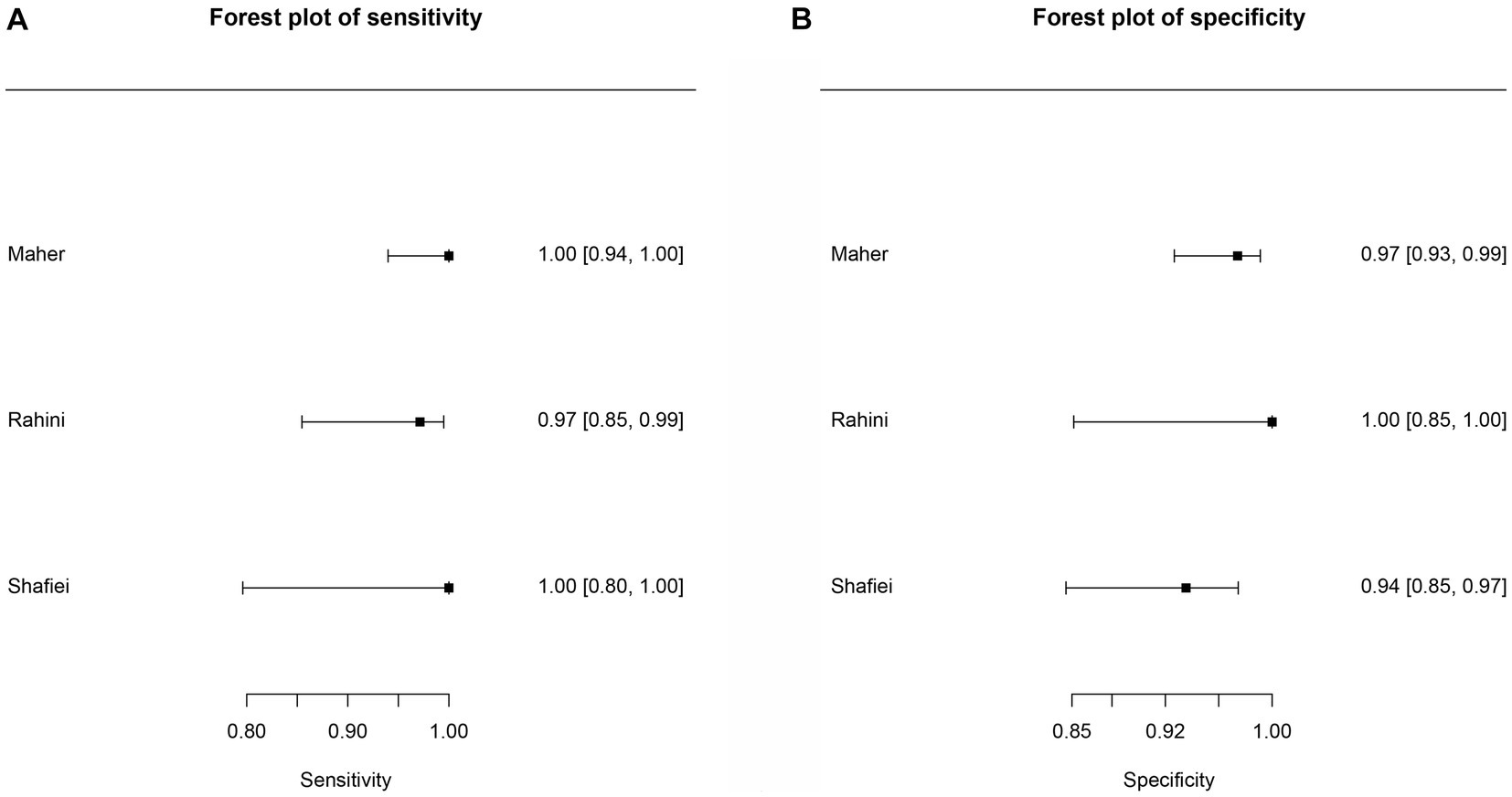
Figure 3. Forest plots for the human FhSA proteins paper group. (A) Forest plot sensitivity for human somatic antigens paper group. (B) Forest plot specificity for human somatic antigens paper group.
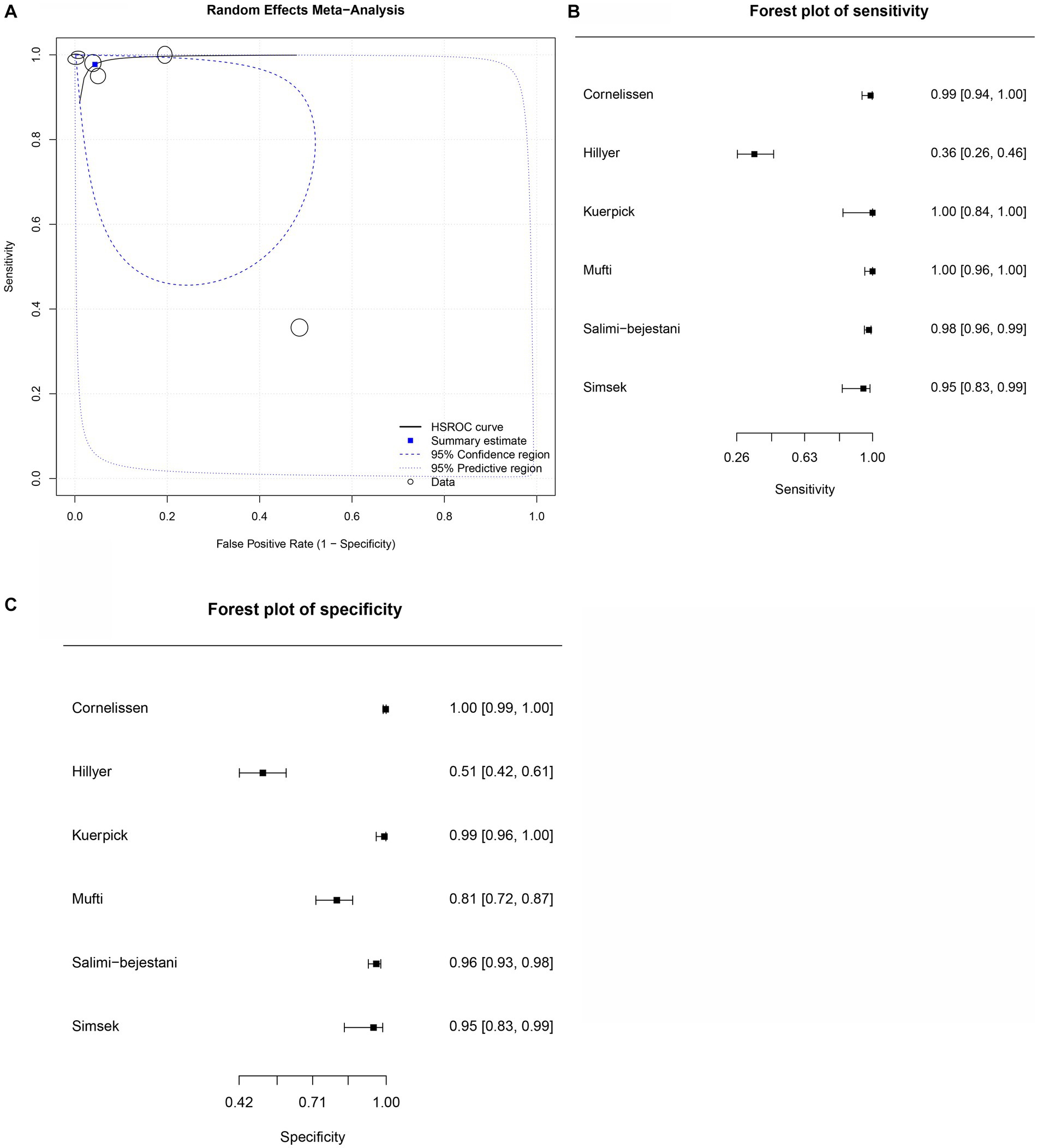
Figure 4. Forest plots for the cattle FhES proteins paper group. (A) Random effects meta-analysis for cattle excretory-secretory proteins paper group. The size of the circles determines the weight of the study. (B) Forest plot sensitivity for cattle excretory-secretory paper group. (C) Forest plot specificity for cattle excretory-secretory proteins paper group.
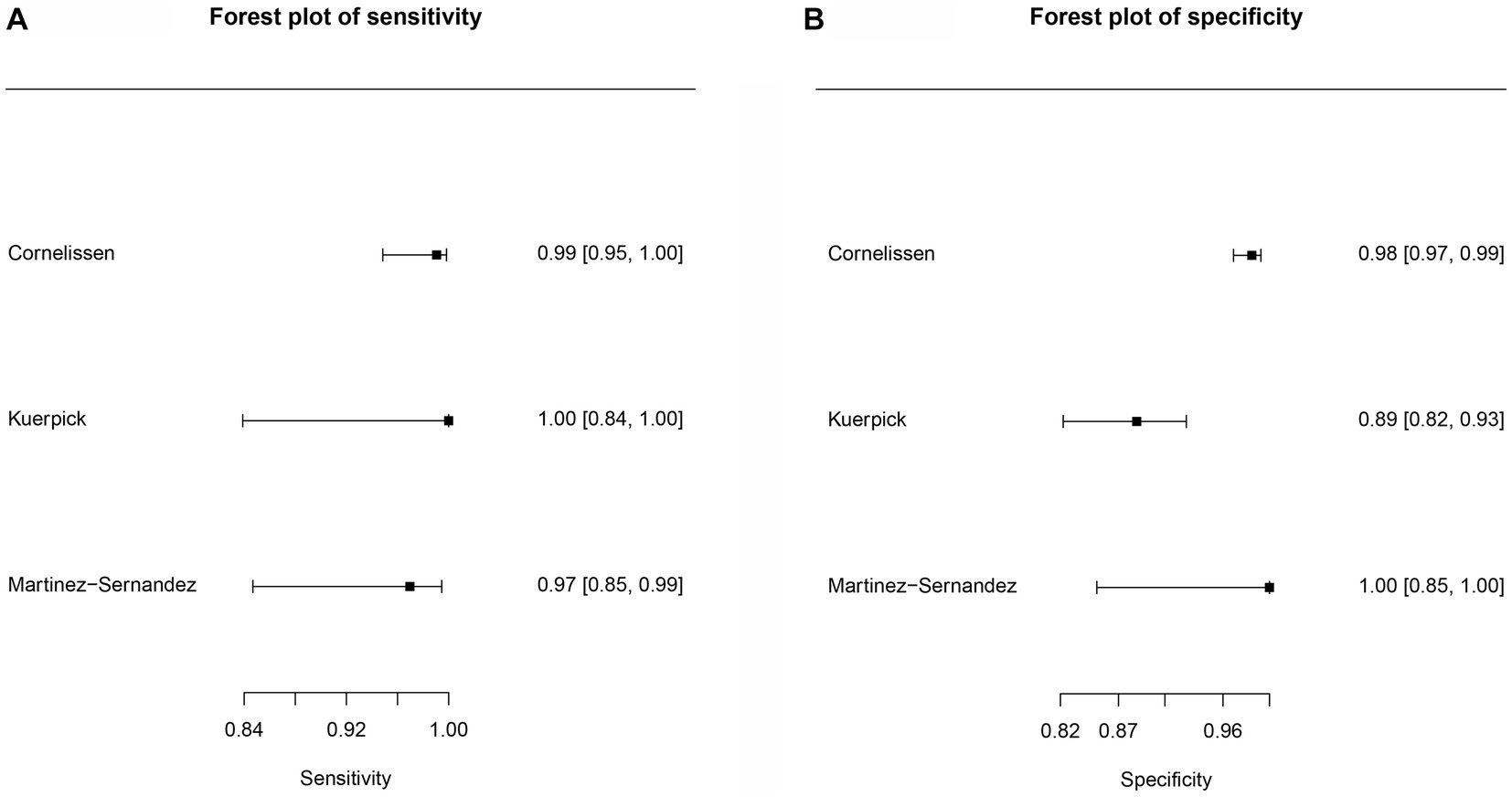
Figure 5. Forest plots for the cattle FhrCL-1 protein paper group. (A) Forest plot sensitivity for cattle recombinant cathepsin antigens paper group. (B) Forest plot specificity for cattle recombinant cathepsin paper group.
3.3.1. Diagnostic accuracy of antigens selected in meta-analysis
Ten studies were included for the human FhES protein group (Figures 2B,C). Sensitivity estimates for the FhES group were high and similar across the analyzed articles (0.968), and the 2.5% CI and (97.5%) values were 0.931 and 0.985, respectively. For specificity estimates, the 2.5% CI and 97.5% CI were 0.989, 0.959, and 0.997, respectively. Table 1 shows the DOR. Two studies from the human FhES antigen group showed 100% sensitivity and specificity with the ELISA test. All papers that discussed the FhES antigen and ELISA tests showed a high level of sensitivity, and four articles had 100% sensitivity. In one paper, the specificity was lower than 90%. Four studies showed 100% specificity when using the FhES antigen for serum diagnosis of F. hepatica in humans (Figures 2B,C). The estimated positive summary Likelihood Ratio for this group was 88.000, which stands as the highest value among the analyzed antigens in the meta-analysis (Table 1).
Figure 2 summarizes the overall diagnostic accuracy of the human FhES protein group. The HSROC curve had a curvilinear shape (Figure 2A), indicating similarity among the papers included in the meta-analysis, with circles showing a similar format. The SROC point is located near the upper left corner of the curve.
For the human FhSA protein group (human FhSA), three studies were included in the meta-analysis (Figure 3). In this case, no SROC plots were not produced. The sensitivity estimates for the human FhSA group were 0.991, and the 2.5% CI and 97.5% CI values were 0.938 and 0.999, respectively. The 2.5% CI and 97.5% CI values for specificity estimates were 0.965, 0.928, and 0.983, respectively. The DOR is shown in Table 2. Among the studies encompassed in the analysis, the groups focusing on the human FhSA protein and the cattle FhrCL-1 protein exhibited the most minimal negative summary Likelihood Ratio values, which suggests a favorable likelihood of accurate negative diagnosis (Tables 2, 4).
Six studies were included in the cattle FhES protein group (Figures 4B,C). Figure 4A provides an overview of the overall diagnostic accuracy of the cattle FhES protein group. The summary HSROC curve was not curvilinear, suggesting a heterogeneous distribution between papers. Circles show a similar format. The sensitivity estimates for the cattle FhES group were 0.977, and the 2.5% CI and 97.5% CI values were 0.844 and 0.997, respectively. The 2.5% CI and 97.5% CI values for specificity estimates were 0.956, 0.806, and 0.991, respectively. Two papers that used the FhES antigen for serum diagnosis of F. hepatica in cattle showed a sensitivity of 100%, and the specificity varied between 85 and 99% (Figures 4B,C). Four studies presented more variation in sensitivity and specificity. Table 3 shows the DOR. The cattle FhES protein group demonstrated positive and negative summary Likelihood Ratio values of 22.200 and 0.020, respectively (Table 3).
Three studies were included (Figure 5) for the cattle FhrCL-1 protein group. In this case, no ROC curves were produced. The sensitivity estimate for the FhrCL-1 group was 0.991, and the 2.5% CI and 97.5% CI values were 0.925 and 0.999, respectively. For the specificity estimate, the 2.5% CI and 97.5% CI values were 0.973, 0.871, and 0.995, respectively. DOR can be observed in Table 4.
Seven studies were included for the sheep FhES protein group (Figures 6B,C); the overall diagnostic accuracy is summarized in Figure 6A. The summary HSROC curve was curvilinear, suggesting a homogeneous distribution between papers in the meta-analysis, with circles showing similar formats. The sensitivity estimates for the FhES group were 0.982, and the 2.5% CI and 97.5% CI values were 0.925 and 0.996, respectively. The 2.5% CI and 97.5% CI values for specificity estimates were 0.981, 0.639, and 0.999, respectively. Table 5 shows the DOR. According to the DOR results, the papers that used FhES antigens for serological diagnosis of fasciolosis in sheep showed similar results to those of other species. Table 5 presents the positive and negative summary Likelihood Ratio values as 51.680 and 0.020, respectively, further emphasizing the elevated accuracy of the antigen.
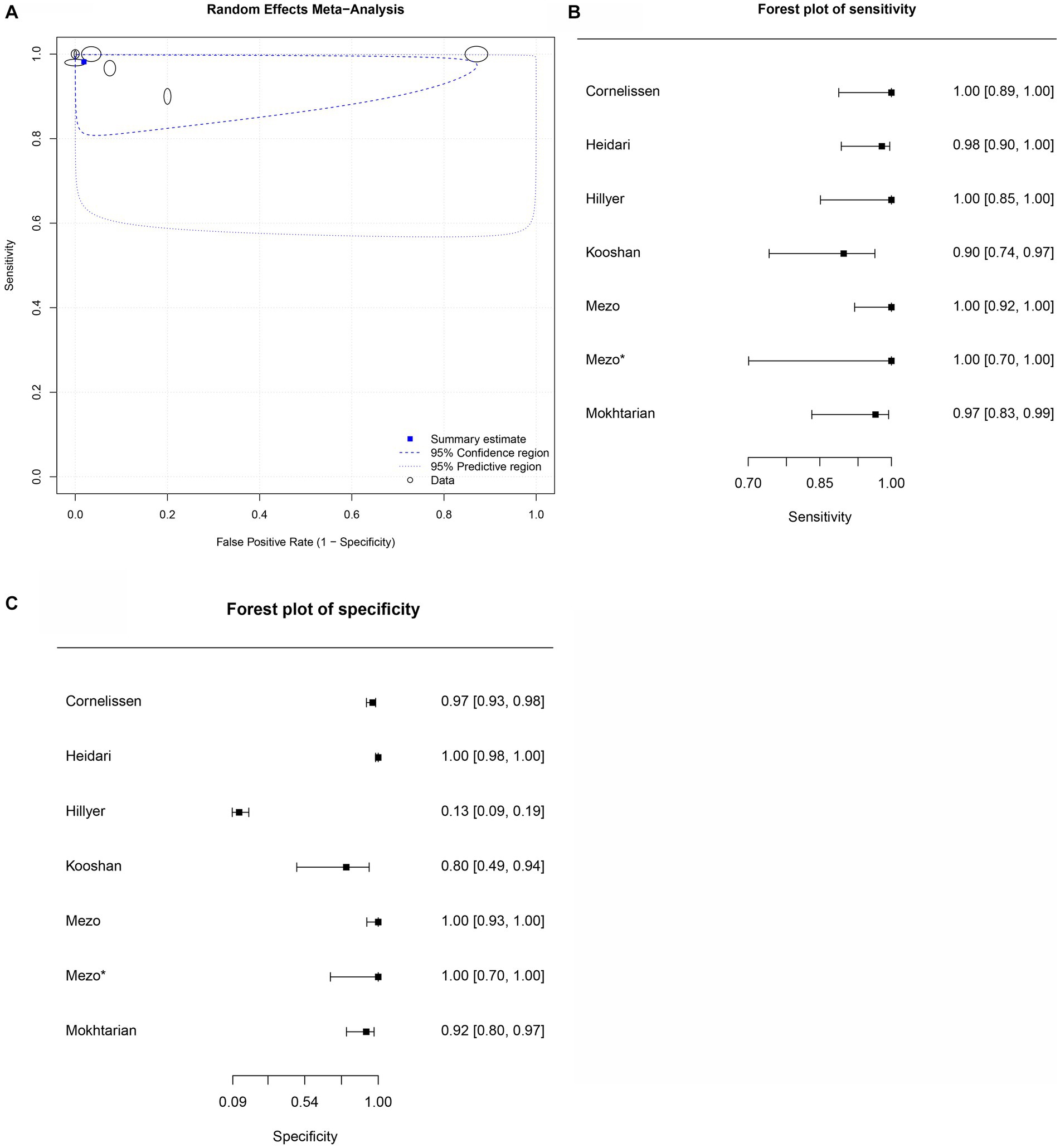
Figure 6. Forest plots for the sheep FhES proteins paper group. (A) Random effects meta-analysis for sheep excretory-secretory proteins paper group. The size of the circles determines the weight of the study. (B) Forest plot sensitivity for sheep excretory-secretory proteins paper group. *This corresponds to a second approach by Mezo et al. (60), in the same article, in field research. (C) Forest plot specificity for sheep excretory-secretory proteins paper group.
Within the five selected groups, the ES antigens of F. hepatica were the most extensively studied. Despite the sheep FhES group having just one study and the lowest specificity, the overall results were good. This group displayed a large variation in terms of specificity. The sheep FhES group presented similar results between studies as other species.
In general, the reviewed articles gave consistent results, with some studies indicating similarities between serological ELISA tests using different antigens and coprological detection of F. hepatica eggs in human, cattle, and sheep feces. Among the analyzed antigens, the cattle FhrCL-1 exhibited homogeneous results, with good accuracy for the serological diagnosis of fasciolosis using ELISA tests. Other native antigens included in the meta-analysis were the human FhSA antigen group, which showed low variation in sensitivity and specificity compared to the identification of F. hepatica eggs in human and cattle feces. For the sheep FhES antigen group, the random effects meta-analysis was more heterogeneous than other native and recombinant antigen groups. Based on the DOR results, studies that used human and cattle FhES as antigens for serological diagnosis of fasciolosis in humans, cattle, and sheep showed good results compared to coprological detection of the F. hepatica eggs in feces.
4. Discussion
To our knowledge, this is the first meta-analysis based on serodiagnosis of hepatic fasciolosis in humans, cattle, and sheep. Thirty-three studies were included, and a meta-analysis was performed on 27 of these. In general, the studies were of moderate methodological quality and were clinically heterogeneous. All studies analyzed in this meta-analysis used cattle serum samples and confirmed fasciolosis through fecal analysis, which is considered the gold standard test for diagnosing this disease. Overall, FhrCL-1, FhES, and FhSAP-2 antigens presented the best sensitivity and specificity results for the serum diagnosis of animal and human fasciolosis. The quality of the articles was assessed based on the criteria outlined in QUADAS or STARD. These criteria encompassed the characterization of the samples, the time elapsed between the reference standard and index test, and the demographic characteristics of the animal population under study. After evaluating the results, it was found that none of the articles fulfilled all the QUADAS and STARD criteria.
Fasciolosis is a neglected tropical disease diagnosed through coprology and serological methods (1). A large number of antigens (both native and recombinant) were used in the ELISA tests described in the articles. Among the papers included in the meta-analysis studied the human FhES antigen group. FhES has been employed for diagnosing human and bovine fasciolosis in ELISA tests and has proven highly effective (47–50). FhES antigens play a role in assisting the parasite’s migration through the host tissue. Thus, immunoglobulins for this antigen can be detected in early F. hepatica infection (21, 24, 36). Serological tests for the diagnosis of human fasciolosis showed good efficacy when human and animal antibodies for F. hepatica were detected using the FhES antigen in the ELISA tests. However, purification of cysteine proteinase is a complex and time-consuming process (18, 22, 34) that can make the production of a commercial ELISA test difficult.
4.1. Native Fasciola hepatica antigen
The antigenic preparations used in this study, including the human FhSA protein group, were primarily sourced from adult worm extracts and excretion products, as well as partially purified fractions (51–53). Antibody detection assays were preferred for the immune diagnosis of fasciolosis (27, 29) due to their relative simplicity and early seroconversion during primary infections (3). Since F. hepatica is the main cause of human and animal fasciolosis, most of the studies investigating the diagnosis of this disease focused on subunits purified from either FhSA or FhES antigens specific to this fluke species (27, 51, 54). For the human antigen groups (FhSA and FhES), only three articles provide a thorough characterization of the study population (46, 47, 52). Most articles that investigate FhSA and FhES antigens in humans utilize samples from hospitals (23–25, 27, 48–51, 53). As a result, it is challenging to determine the timing of infection, but it is likely that these are chronic infections. Only one article mentions the detection of acute infection by F. hepatica (47).
Six studies were included in the meta-analysis evaluating the cattle FhES protein group. Excretory-secretory products (ESPs) are the antigens that were most commonly used together with ELISA methods for antibody detection. The FhES antigens of F. hepatica used in ELISA tests are known to be immunodominant in cattle naturally exposed to F. hepatica infection (3, 35, 57). Native antigens of F. hepatica can be collected at bovine abattoirs and used in the laboratory for ELISA tests. This meta-analysis is in line with previous studies, which showed that a cattle FhES protein group plays a valuable role in an ELISA system for the serodiagnosis of bovine fasciolosis (22, 54, 55). Based on the cattle FhES antigen group, ELISA tests have been used to detect experimental infections in cattle from the third to the fifth week after infection (22, 35, 56). However, although these studies have good experimental designs, they are limited by the lack of clinical and epidemiological information on the animals. For the cattle FhES proteins paper group, one article had good sample characterization (22). Articles with natural and experimental infections in cattle were selected. Approximately 100 metacercariae were used in experimental infection studies on cattle (22, 35, 56). In papers with experimental infections, antibody detection occurred between 2 and 4 weeks post-infection (22, 56).
For the FhSA sheep group, only two papers were selected, and a meta-analysis was not performed. These papers reported a sensitivity of 80% and specificity of 90% (59, 60), which was relatively low compared to other antigens (21, 24, 25). Another study that used FhSA as an antigen in an ELISA test reported a sensitivity and specificity of close to 100% (20). The use of the FhSA and FhES native antigens for routine diagnostic laboratory testing presents some challenges, including the dependence on the availability of living flukes and the fact that it is an antigen mixture subjected to variations due to natural conditions (24, 25). However, laboratories can obtain recombinant antigens, and it has been shown that large quantities of highly pure recombinant F. hepatica antigens with correct folding play a vital role in improving the antigenicity and accuracy of serodiagnosis methods (22, 24, 25, 36). For the sheep FhES proteins, articles were found on both natural and experimental infections. The samples were obtained from farms and abattoirs (57–59). However, none of the studies provided a thorough sample characterization. In the papers with experimental infections, antibody detection occurred between 1 and 3 weeks post-infection (60). Sheep were the hosts where the antibody was identified earliest (36, 60).
4.2. Recombinant Fasciola hepatica antigen
In terms of human groups using the FhrFtn-1 antigen, just one paper was selected. In this paper, the sensitivity and specificity were close to 100% (61). FhrFtn-1 is expressed during parasite development and has been shown to be highly reactive with sera from experimental animals with acute or chronic infections. However, it is important to highlight that the FhrFtn-1 antigen presented cross-reactivity for other parasites (61), therefore compromising the effectiveness of the ELISA test.
The FhrGST antigen has high antibody titers during active sheep infections, indicating that these molecules are repeatedly and effectively exposed to the host immune system. Cross-reactivity between fasciolosis and echinococcosis can be observed with the FhrGST and FhrFAB antigens used in ELISA tests (28); consistent results and adjustments are necessary for the ELISA test using the FhrGST and FhrFAB antigens for commercialization.
The human group using FhrTP 16.5, a small antigen of the tegument of F. hepatica expressed in bacteria, showed sensitivity and specificity that were close to 90% (61). The tegumental surface of F. hepatica is a unique syncytial structure that serves as an interface between the parasite and host. The FhrTP antigens are easily released to stimulate the host immune response and are therefore considered diagnostic antigens (3, 61). The FhrTP antigen is located on the parasite’s surface and has cross-reactivity with other parasites (62), compromising the quality of ELISA tests. Furthermore, current parasitological methods depend on the technician’s expertise, as F. hepatica eggs can be confused with those of other helminths (4, 13).
Among the subunit antigens, cathepsin-L, a component of Fasciola ES antigens, garnered significant attention. Serological tests have shown that they are highly accurate in diagnosing human, cattle, and sheep fasciolosis. The recombinant cathepsin L1 test uses recombinant pro-cathepsin L1 and targets antibodies against cathepsin, a cysteine protease, to diagnose fasciolosis caused by F. hepatica. Similarly, other studies have not found cross-reactions in cathepsin-based ELISA tests (21) and have reported good performance. The ELISA test yielded better results with FhES, a native antigen collected from F. hepatica obtained in a bovine abattoir. The second most important antigen used in ELISA tests was FhrCL-1, a recombinant antigen expressed in bacteria and yeast. One article had good sample characterization for the cattle FhrCL-1 protein paper group (22). In the experimental infection in cattle, antibodies against F. hepatica were identified 3 weeks post-infection (22, 36).
Only two papers were selected for human groups using FhrSAP-2, and it was therefore impossible to conduct a meta-analysis. These antigens are expressed in E. coli (24, 25). The papers that used the FhrSAP-2 antigen for serum diagnosis of F. hepatica in humans showed a sensitivity of 100% and a specificity higher than 95% (24, 25). Previous studies have also shown that FhrSAP-2 is highly immunogenic and can detect the acute phase of fasciolosis (24, 25, 28). In sheep FhrCL-1, two studies were selected, and no meta-analysis was performed. The sensitivity and specificity were very high for FhrCL-1 (28, 29). Analysis of different cloning and variations of purification methods has shown diverse levels of sensitivity, specificity, and accuracy in diagnostic tests. For the last human group using FhrCL-1, just two papers were selected, and a meta-analysis was not carried out (21, 24); in these articles, the sensitivity and specificity were close to 100%. The FhrCL-1 antigen is localized in excretory and secretory proteins and has no cross-reactivity with other parasites (21, 24). A good diagnostic test must distinguish between F. hepatica and other parasitic diseases.
Nine of the 33 studies analyzed used recombinant antigens in the ELISA test. FhrCL-1, FhES, and FhrSAP-2 antigens gave the best results, with high sensitivity and specificity values for fasciolosis serodiagnosis in humans and animals. The recombinant antigen can be used in ELISA tests for non-invasive or bulk tank milk samples for epidemiological studies. Serological studies are now the main diagnostic method in use, enabling disease diagnosis even during the acute stage and before the parasite eggs can be identified in feces. Serology has the advantage of identifying infections much earlier than fecal egg identification (around 4–5 weeks) (21, 25, 29). The serological methods, especially the ELISA test, are highly sensitive and specific compared to diagnosing F. hepatica by coprological methods (25, 27, 29). Recombinant proteins allow for increased mass screening, facilitating fasciolosis serodiagnosis in humans and animals.
Our meta-analysis has shown that early antibodies against F. hepatica can be detected in both animals and humans. This early detection is made possible through the use of native and recombinant antigens in ELISA tests (21, 22, 51, 54, 58). However, the studies included in the meta-analysis did not adequately distinguish between acute and chronic fasciolosis infections. Despite this, the results obtained indicated high sensitivity and specificity values for various antigens in both animals and humans. By employing these antigens in ELISA tests, it becomes possible to accurately identify F. hepatica antibodies, thereby reducing the occurrence of false positives or false negatives. Nevertheless, despite the promising findings from the meta-analysis, the availability of antigens in the form of ELISA tests for the systematic identification of fasciolosis in animals and humans remains limited (36, 47, 49). Currently, only a small number of native and recombinant antigens are commercially accessible in the form of ELISA tests for widespread use (22, 47, 51, 52).
Our study has some limitations that need to be addressed. Firstly, the number of studies included in the meta-analysis is relatively small, which restricts our ability to conduct relevant subgroup analyses, such as age and time of infection. This limitation is caused by the lack of consistent data found in the available literature. However, this limitation highlights the importance of further research in the literature to gather more data, aiming to provide high-quality scientific evidence for the incorporation of these tests in disease screening and early diagnosis. Despite these limitations, it is crucial to acknowledge the robustness and low heterogeneity of the data obtained in our study. These factors have allowed us to draw sound conclusions from the results we have obtained so far. Future research with a larger and more diverse pool of studies will be valuable to expand and corroborate our findings.
5. Conclusion
The meta-analysis results showed eight antigen types for serum diagnosis of fasciolosis in humans, cattle and sheep. Most articles analyzed used ES antigens in humans. It is therefore suggested that FhrCL-1, FhES, and FhrSAP-2 could be considered ideal diagnostic antigens for the earliest serum diagnosis of human and animal fasciolosis. We recommend future studies with F. hepatica antigens for serological diagnosis in other animal species and the need for the literature to include more robust and well-characterized studies.
Author contributions
GD: conceptualization, methodology, and manuscript writing. TV: conceptualization, methodology, manuscript writing and reviewing. VB: data curation, meta-analysis, and manuscript reviewing. MP: meta-analysis and manuscript reviewing. JR and LM: manuscript reviewing. FF: manuscript writing, reviewing, and editing. All authors contributed to the article and approved the submitted version.
Acknowledgments
The authors would like to thank the Fundação Osvaldo Cruz (FIOCRUZ–PR), Coordenação de Aperfeiçoamento de Pessoal de Nível Superior (CAPES), and Conselho Nacional de Pesquisa (CNPq) for the possibility of developing this research.
Conflict of interest
The authors declare that the research was conducted in the absence of any commercial or financial relationships that could be construed as a potential conflict of interest.
Publisher’s note
All claims expressed in this article are solely those of the authors and do not necessarily represent those of their affiliated organizations, or those of the publisher, the editors and the reviewers. Any product that may be evaluated in this article, or claim that may be made by its manufacturer, is not guaranteed or endorsed by the publisher.
Supplementary material
The Supplementary material for this article can be found online at: https://www.frontiersin.org/articles/10.3389/fvets.2023.1252454/full#supplementary-material
References
1. Mas-Comas, S , Bargues, MD , and Valero, MA . Human fascioliasis infection sources, their diversity, incidence factors, analytical methods and prevention measures. Parasitology. (2018) 145:1665–99. doi: 10.1017/S0031182018000914
2. Mas-Coma, S , Bargues, MD , and Valero, MA . Fascioliasis and other plant-borne trematode zoonoses. Int J Parasitol. (2005) 35:1255–78. doi: 10.1016/j.ijpara.2005.07.010
3. Mas-Coma, S , Valero, MA , and Bargues, MD . Chapter 2 Fasciola, Lymnaeids and human fascioliasis, with a global overview on disease transmission, epidemiology, evolutionary genetics, molecular epidemiology and control. Adv Parasitol. (2009) 69:41–146. doi: 10.1016/S0065-308X(09)69002-3
4. Fürst, T , Sayasone, S , and Odermatt, P . Manifestation, diagnosis, and management of foodborne trematodiasis. Br Med J. (2012) 344:1–11. doi: 10.1136/bmj.e4093
5. Carmona, C , and Tort, JF . Fasciolosis in South America: epidemiology and control challenges. J Helminthol. (2017) 91:99–109. doi: 10.1017/S0022149X16000560
6. Molento, MB , Bennema, S , Bertot, J , Pritsch, IC , and Arenal, A . Bovine fascioliasis in Brazil: economic impact and forecasting. Vet Parasitol Reg Stud Rep. (2018) 12:1–3. doi: 10.1016/j.vprsr.2017.12.004
7. Américo, L , Padilha, MAC , Arruda, PM , Drescher, G , de Moura, AB , and Chryssafidis, AL . Epidemiological survey and confirmation of autochthonous cases of bovine fasciolosis in the Serrana mesoregion of Santa Catarina, Brazil. Front Vet Sci. (2022) 9:1–8. doi: 10.3389/fvets.2022.93346
8. Spithill, TW , Smooker, PM , and Coperman, DB . “Fasciola gigantica”: epidemiology, control, immunology and molecular biology In: JP Dalton , editor. Fasciolosis. CABI Publisher: Wallingford, Oxon (1999). 465–525.
9. Hayward, AD , Skuce, PJ , and McNeilly, TN . The influence of liver fluke infection on production in sheep and cattle: a meta-analysis. Int J Parasitol. (2021) 51:913–24. doi: 10.1016/j.ijpara.2021.02.006
10. Howell, A , Baylis, M , Smith, R , Pinchbeck, G , and Williams, D . Epidemiology and impact of Fasciola hepatica exposure in high-yielding dairy herds. Prev Vet Med. (2015) 121:41–8. doi: 10.1016/j.prevetmed.2015.05.013
11. Mehmood, K , Zhang, H , Sabir, AJ , Abbas, RZ , Ijaz, M , Durrani, AZ, et al. A review on epidemiology, global prevalence and economical losses of fasciolosis in ruminants. Microb Pathog. (2017) 109:253–62. doi: 10.1016/j.micpath.2017.06.006
12. Kouam, MK , Meningue, R , and Fon, DE . Parasitic causes of organ condemnation in cattle slaughtered in Fako abattoirs, south-west region of Cameroon, and estimate of financial losses. J Helminthol. (2018) 93:367–71. doi: 10.1017/S0022149X18000391
13. Arifin, MI , Höglund, J , and Novobilský, A . Comparison of molecular and conventional methods for the diagnosis of Fasciola hepatica infection in the field. Vet Parasitol. (2016) 232:8–11. doi: 10.1016/j.vetpar.2016.11.003
14. Maciel, MG , Lima, WDS , Almeida, FLM , De Coelho, LIARC , Araújo, GAN , Lima, MG, et al. Cross-sectional serological survey of human fascioliasis in Canutama municipality in Western Amazon, Brazil. J Parasitol Res. (2018) 2018:1–8. doi: 10.1155/2018/6823638
15. Daniel, AZ . Comparison of Kato-Katz thick smear, Mini-FLOTAC, and fluke finder for the detection and quantification of Fasciola hepatica eggs in artificially spiked human stool. Am J Trop Med Hyg. (2019) 101:59–61. doi: 10.4269/ajtmh.18-0988
16. Fürst, T , Keiser, J , and Utzinger, J . Global burden of human food-borne trematodiasis: a systematic review and meta-analysis. Lancet Infect Dis. (2012) 12:210–21. doi: 10.1136/bmj.e4093
17. Kang, J , Bahk, Y , Cho, P , Hong, S , Kim, T , Sohn, W, et al. A family of cathepsin F cysteine proteases of Clonorchis sinensis is the major secreted proteins that are expressed in the intestine of the parasite. Mol Biochem Parasitol. (2010) 170:7–16. doi: 10.1016/j.molbiopara.2009.11.006
18. Norbury, LJ , Beckham, S , Pike, RN , Grams, R , Spithill, TW , Fecondo, JV, et al. Adult and juvenile Fasciola cathepsin L proteases: different enzymes for different roles. Biochimie. (2011) 93:604–11. doi: 10.1016/j.biochi.2010.12.004
19. Meemon, K , and Sobhon, P . Juvenile-specific cathepsin proteases in Fasciola spp.: their characteristics and vaccine efficacies. Parasitol Res. (2015) 114:2807–13. doi: 10.1007/s00436-015-4589-6
20. Cornelissen, JB , de Leeuw, WA , and van der Heijden, PJ . Comparison of an indirect haemagglutination assay and an ELISA for diagnosing Fasciola hepatica in experimentally and naturally infected sheep. Vet Q. (1992) 14:152–6. doi: 10.1080/01652176.1992.9694354
21. Gonzales Santana, B , Dalton, JP , Vasquez Camargo, F , Parkinson, M , and Ndao, M . The diagnosis of human fascioliasis by enzyme-linked immunosorbent assay (ELISA) using recombinant Cathepsin L protease. PLoS Negl Trop Dis. (2013) 7:e2414–9. doi: 10.1371/journal.pntd.0002414
22. Kuerpick, B , Schnieder, T , and Strube, C . Evaluation of a recombinant cathepsin L1 ELISA and comparison with the Pourquier and ES ELISA for the detection of antibodies against Fasciola hepatica. Vet Parasitol. (2013) 193:206–13. doi: 10.1016/j.vetpar.2012.11.021
23. Figueroa-Santiago, O , Delgado, B , and Espino, AM . Fasciola hepatica saposin-like protein-2-based ELISA for the serodiagnosis of chronic human fascioliasis. Parasitology. (2011) 70:355–61. doi: 10.1016/j.diagmicrobio.2011.03.016
24. Gottstein, B , Schneeberger, M , Boubaker, G , Merkle, B , Huber, C , Spiliotis, M, et al. Comparative assessment of ELISAs using recombinant Saposin-like protein 2 and recombinant Cathepsin L-1 from Fasciola hepatica for the Serodiagnosis of human Fasciolosis. PLoS Negl Trop Dis. (2014) 8:1–10. doi: 10.1371/journal.pntd.0002860
25. Mirzadeh, A , Valadkhani, Z , Yoosefy, A , Babaie, J , Golkar, M , Esmaeili Rastaghi, AR, et al. Expression, purification and in vitro refolding of the recombinant truncated Saposin-like protein 2 antigen for development of diagnosis of human fascioliasis. Acta Trop. (2017) 171:163–71. doi: 10.1016/j.actatropica.2017.03.002
26. Villa-Mancera, A , Molina-Mendoza, P , Hernández-Guzmán, K , Olivares-Pérez, J , Sarracent-Pérez, J , and Zumaquero-Ríos, J . Comparative diagnosis of serum IgG1 and Coproantigen ELISA for fasciolosis detection of goats in Mexico. Biomed Res Int. (2016) 2016:1–7. doi: 10.1155/2016/3860928
27. Aguayo, V , Valdes, B , and Espino, AM . Assessment of Fasciola hepatica glutathione S-transferase as an antigen for serodiagnosis of human chronic fascioliasis. Acta Trop. (2018) 186:41–9. doi: 10.1016/j.actatropica.2018.07.002
28. Mokhtarian, K , Meamar, AR , Khoshmirsafa, M , Razmjou, E , Masoori, L , Khanmohammadi, M, et al. Comparative assessment of recombinant and native immunogenic forms of Fasciola hepatica proteins for serodiagnosis of sheep fasciolosis. Parasitol Res. (2018) 117:225–32. doi: 10.1007/s00436-017-5696-3
29. Martínez-Sernández, V , Perteguer, MJ , Hernández-González, A , Mezo, M , González-Warleta, M , Orbegozo-Medina, RA, et al. Comparison of recombinant cathepsins L1, L2, and L5 as ELISA targets for serodiagnosis of bovine and ovine fascioliasis. Parasitol Res. (2018) 117:1521–34. doi: 10.1007/s00436-018-5809-7
30. Tort, J , Brindley, PJ , Knox, D , Wolfe, KH , and Dalton, JP . Proteinases and associated genes of parasitic helminths. Adv Parasitol. (1999) 43:161–266. doi: 10.1016/s0065-308x(08)60243-2
31. Wang, S , Wei, W , Luo, X , Wang, S , Hu, S , and Cai, X . Comparative genomic analysis of aspartic proteases in eight parasitic platyhelminths: insights into functions and evolution. Gene. (2015) 559:52–61. doi: 10.1016/j.gene.2015.01.020
32. McVeigh, P , Maule, AG , Dalton, JP , and Robinson, MW . Fasciola hepatica virulence-associated cysteine peptidases: a systems biology perspective. Microbes Infect. (2012) 14:301–10. doi: 10.1016/j.micinf.2011.11.012
33. Ali, NM . Development and evaluation of a dipstick assay in diagnosis of human fasciolosis. Parasitol Res. (2012) 110:1649–54. doi: 10.1007/s00436-011-2678-8
34. Mazeri, S , Sargison, N , Kelly, RF , Bronsvoort, BMDC , and Handel, I . Evaluation of the performance of five diagnostic tests for Fasciola hepatica infection in naturally infected cattle using a Bayesian no gold standard approach. PLoS One. (2016) 11:1–22. doi: 10.1371/journal.pone.0161621
35. Cornelissen, JBWJ , Gaasenbeek, CPH , Boersma, W , Borgsteede, FHM , and Van Milligen, FJ . Use of a pre-selected epitope of cathepsin-L1 in a highly specific peptide-based immunoassay for the diagnosis of Fasciola hepatica infections in cattle. Int J Parasitol. (1999) 29:685–96. doi: 10.1080/01652176.1992.9694354
36. Cornelissen, JBWJ , Gaasenbeek, CP , Borgteede, FH , Holland, WG , Harmsen, MM , and Boersma, WJA . Early immunodiagnosis of fasciolosis in ruminants using recombinant Fasciola hepatica cathepsin L-like protease. Int J Parasitol. (2001) 31:728–37. doi: 10.1016/S0020-7519(01)00175-8
37. Moher, D , Liberati, A , Tetzlaff, J , Altman, DG , and Grp, P . Preferred reporting items for systematic reviews and Meta-analyses: the PRISMA statement (reprinted from annals of internal medicine). Phys Ther. (2009) 89:873–80. doi: 10.1371/journal.pmed.1000097
38. De Oliveira, MRF , De Castro Gomes, A , and Toscano, MG . QUADAS e STARD: avaliação da qualidade de estudos de acurácia de testes diagnósticos. Rev Saúde Pública. (2011) 45:416–22. doi: 10.1590/S0034-89102011000200021
39. Whiting, PF , Rutjes, AWS , Westwood, ME , Mallet, S , Deeks, JJ , Reitsma, JB, et al. QUADAS-2: a revised tool for the quality assessment of diagnostic accuracy studies. Ann Intern Med. (2011) 155:529–36. doi: 10.7326/0003-4819-155-8-201110180-00009
40. Bossuyt, PM , Reitsma, JB , Bruns, DE , Gatsonis, CA , Glasziou, PP , Irwig, LM, et al. The STARD statement for reporting studies of diagnostic accuracy: explanation and elaboration. Ann Intern Med. (2003) 138:W1–W12. doi: 10.7326/00034819138-1-200301070-00012-w1
41. Chu, H , and Cole, SR . Bivariate meta-analysis of sensitivity and specificity with sparse data: a generalized linear mixed model approach. J Clin Epidemiol. (2006) 59:1331–2. doi: 10.1016/j.jclinepi.2006.06.011
42. Freeman, SC , Kerby, CR , Patel, A , Cooper, NJ , Quinn, T , and Sutton, AJ . Development of an interactive web-based tool to conduct and interrogate meta-analysis of diagnostic test accuracy studies: MetaDTA. BMC Med Res Methodol. (2019) 19:1–11. doi: 10.1186/s12874-019-0724-x
43. Burke, DL , Ensor, J , Snell, KIE , van der Windt, D , and Riley, RD . Guidance for deriving and presenting percentage study weights in meta-analysis of test accuracy studies. Res Synth Methods. (2018) 9:163–78. doi: 10.1002/jrsm.1283
44. Patel, A , Cooper, N , Freeman, S , and Sutton, A . Graphical enhancements to summary receiver operating characteristic plots to facilitate the analysis and reporting of meta-analysis of diagnostic test accuracy data. Res Synth Methods. (2021) 12:34–44. doi: 10.1002/jrsm.1439
45. Morales, A , and Espino, AM . Evaluation and characterization of Fasciola hepatica tegument protein extract for serodiagnosis of human fascioliasis. Clin Vaccine Immunol. (2012) 19:1870–8. doi: 10.1128/CVI.00487-12
46. Cornejo, H , Oblitas, F , Cruzado, S , and Quispe, W . Evaluación de una prueba de ELISA con antígeno metabólico de Fasciola hepatica para el diagnóstico de fasciolosis humana en Cajamarca, Perú. Rev Peru Med Exp Salud Pública. (2010) 27:569–74. doi: 10.17843/rpmesp.2010.274.1529
47. Espinoza, JR , Timoteo, O , and Herrera-Velit, P . Fas2-ELISA in the detection of human infection by Fasciola hepatica. J Helminthol. (2005) 79:235–40. doi: 10.1079/joh2005303
48. Rokni, MB , Massoud, J , O’Neill, SM , Parkinson, M , and Dalton, JP . Diagnosis of human fasciolosis in the Gilan province of northern Iran: application of cathepsin L-ELISA. Diagn Microbiol Infect Dis. (2002) 44:175–9. doi: 10.1016/S0732-8893(02)00431-5
49. Carnevale, S , Rodríguez, MI , Santillán, G , Labbé, JH , Cabrera, MG , Bellegarde, EJ, et al. Immunodiagnosis of human fascioliasis by an enzyme-linked immunosorbent assay (ELISA) and a micro-ELISA. Clin Diagn Lab Immunol. (2001) 8:174–7. doi: 10.1128/CDLI.8.1.174-177.2001
50. Córdova, M , Reátegui, L , and Espinosa, JR . Immunodiagnosis of human fascioliasis with Fasciola hepatica cysteine proteinases. Trans R Soc Trop Med Hyg. (1999) 93:54–7. doi: 10.1016/S0035-9203(99)90178-5
51. Shafiei, R , Sarkari, B , and Sadjjadi, SM . Performance of a 27 kDa Fasciola hepatica antigen in the diagnosis of human fascioliasis. J Lab Phys. (2015) 2014:17–20. doi: 10.1155/2014/405740
52. Rahimi, MT , Ashrafi, K , Koosha, S , Abdi, J , and Rokni, MB . Evaluation of fast-ELISA versus standard-ELISA to diagnose human fasciolosis. Arch Iran Med. (2011) 14:18–21.
53. Maher, K , El Ridi, R , El Hoda, AN , El-Ghannam, M , Shaheen, H , Shaker, Z, et al. Parasite-specific antibody profile in human fascioliasis: application for immunodiagnosis of infection. Am. J. Trop. Med. Hyg. (1999) 61:738–42. doi: 10.4269/ajtmh.1999.61.738
54. Mufti, S , Afshan, K , Khan, IA , Irum, S , Qureshi, IZ , Rizvi, SSR, et al. Serological and coprological studies of bovine fasciolosis in the Pothwar region. Pakistan Pak Vet J. (2015) 35:178–82.
55. Şimşek, S , Köroǧlu, E , Ütük, AE , and Altay, K . Use of indirect excretory/secretory enzyme-linked immunosorbent assay (ES-ELISA) for the diagnosis of natural Fasciola hepatica infection in eosinophilic and non-eosinophilic cattle from eastern Turkey. Turkish J Vet Anim Sci. (2006) 30:411–5.
56. Salimi-Bejestani, M , McGarry, JW , Felstead, S , Ortiz, P , Akca, A , and Willliams, DJL . Development of an antibody-detection ELISA for Fasciola hepatica and its evaluation against a commercially available test. Res Vet Sci. (2005) 78:177–81. doi: 10.1016/j.rvsc.2004.08.005
57. Hillyer, GV , Soler De Galanes, M , Buchón, P , and Bjorland, J . Herd evaluation by enzyme-linked immunosorbent assay for the determination of Fasciola hepatica infection in sheep and cattle from the Altiplano of Bolivia. Vet Parasitol. (1996) 61:211–20. doi: 10.1016/0304-4017(95)00831-4
58. Heidari, H , Zahiri, H , Gharekhani, J , Hosseini, A , and Aeineh, S . Comparison of dot-ELISA and ELISA techniques for detection of Fasciola hepatica in sheep using excretory-secretory antigens. Istanbul Univ Vet Fak Derg. (2015) 41:21–5. doi: 10.16988/iuvfd.2015.14154
59. Kooshan, M , Hashemi, T , and Naghibi, A . Use of somatic and excretory-secretory antigens of Fasciola hepatica in diagnosis of sheep by ELISA. Am-Eurasian J Agric Environ Sci. (2010) 7:170–5.
60. Mezo, M , González-Warleta, M , and Ubeira, FM . Optimized serodiagnosis of sheep fascioliasis by fast-D protein liquid chromatography fractionation of Fasciola hepatica excretory-secretory antigens. J Parasitol. (2003) 89:843–9. doi: 10.1645/GE-74RI.1
61. Cabán-Hernández, K , Gaudier, JF , Ruiz-Jiménez, C , and Espino, AM . Development of two antibody detection enzyme-linked immunosorbent assays for serodiagnosis of human chronic fascioliasis. J Clin Microbiol. (2014) 52:766–72. doi: 10.1128/JCM.02875-13
Keywords: meta-analysis, Fasciola hepatica , native antigen, recombinant antigen, human and animals
Citation: Drescher G, Vasconcelos TCBd, Belo VS, Pinto MMdG, Rosa JdO, Morello LG and Figueiredo FB (2023) Serological diagnosis of fasciolosis (Fasciola hepatica) in humans, cattle, and sheep: a meta-analysis. Front. Vet. Sci. 10:1252454. doi: 10.3389/fvets.2023.1252454
Edited by:
Vikrant Sudan, Guru Angad Dev Veterinary and Animal Sciences University, IndiaReviewed by:
Niranjan Kumar, Kamdhenu University, IndiaSiju Susan Jacob, Indian Council of Agricultural Research (ICAR), India
Sheila Donnelly, University of Technology Sydney, Australia
Copyright © 2023 Drescher, Vasconcelos, Belo, Pinto, Rosa, Morello and Figueiredo. This is an open-access article distributed under the terms of the Creative Commons Attribution License (CC BY). The use, distribution or reproduction in other forums is permitted, provided the original author(s) and the copyright owner(s) are credited and that the original publication in this journal is cited, in accordance with accepted academic practice. No use, distribution or reproduction is permitted which does not comply with these terms.
*Correspondence: Guilherme Drescher, guidrescher@yahoo.com.br; Fabiano Borges Figueiredo, fabiano.figueiredo@fiocruz.br
†The statements in this article must be considered as the author’s own opinion and do not necessarily reflect the official position of the Brazilian Ministry of Agriculture, Livestock and Supply