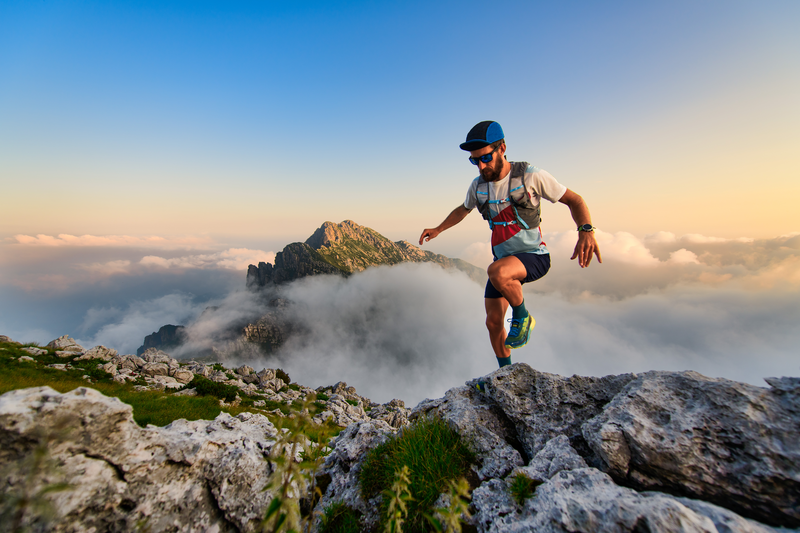
94% of researchers rate our articles as excellent or good
Learn more about the work of our research integrity team to safeguard the quality of each article we publish.
Find out more
ORIGINAL RESEARCH article
Front. Vet. Sci. , 14 September 2023
Sec. Parasitology
Volume 10 - 2023 | https://doi.org/10.3389/fvets.2023.1247552
This article is part of the Research Topic Wildlife Parasitology: Emerging Diseases and Neglected Parasites View all 20 articles
Lipoptena insects are important ectoparasites of cervids and may affect humans that are incidentally bitten. The presence of zoonotic pathogen DNA, such as Anaplasma, and Bartonella, raises the importance of Lipoptena insects in veterinary and human medicine. Eld’s deer (Rucervus eldii thamin), an endangered wild ruminant in Thailand, are bred and raised in the open zoo. The semi-wild zoo environment suggests ectoparasite infestation and potential risk for mechanical transmission of pathogens to visitors, zoo workers, or other animals. However, epidemiology knowledge of pathogens related to endangered wild ruminants in Thailand is limited. This study aims to determine the prevalence and diversity of Anaplasma and Bartonella in the L. fortisetosa collected from captive Eld’s deer in Chon Buri, Thailand. Of the 91 Lipoptena DNA samples obtained, 42 (46.15%) and 25 (27.47%) were positive for Anaplasma and Bartonella by molecular detection, respectively. Further, 42 sequences of Anaplasma (4 nucleotide sequence types) showed 100% identity to those detected in other ruminants and blood-sucking ectoparasites. Twenty-five sequences of Bartonella (8 nucleotide sequence types) showed 97.35–99.11% identity to the novel Bartonella species from sika deer and keds in Japan. Phylogenetic trees revealed Anaplasma sequences were grouped with the clusters of A. bovis and other ruminant-related Anaplasma, while Bartonella sequences were clustered with the novel Bartonella species lineages C, D, and E, which originated from Japan. Interestingly, a new independent lineage of novel Bartonella species was found in obtained specimens. We report the first molecular detection of Anaplasma and Bartonella on L. fortisetosa, which could represent infectious status of captive Eld’s deer in the zoo. Wild animals act as reservoirs for many pathogens, thus preventive measures in surrounding areas should be considered to prevent pathogen infection among animals or potential zoonotic infection among humans.
Deer keds of the genus Lipoptena spp. (Diptera: Hippoboscidae) are hematophagous insects that infest mammals (1). The insects become wingless after finding a suitable host and attach to a single host throughout their life span (2–4). Of over 30 species of Lipoptena insects worldwide, L. fortisetosa along with L. cervi, L. depressa, and L. mazamae are the most prevalent and threaten to wildlife, livestock, and pets (5–8). Lipoptena fortisetosa are found on sika deer (Cervus nippon), roe deer (Capreolus capreolus), and red deer (Cervus elaphus) in many countries and incidentally found on dogs (2, 9–12). In addition, humans can be bitten by Lipoptena insects (13). Several molecular epidemiological studies show L. fortisetosa harbors DNA of various pathogens, including Anaplasma phagocytophilum, Babesia spp., Bartonella spp., Borrelia spp., Coxiella-like endosymbionts, Francisella tularensis, Mycoplasma spp., Rickettsia spp., and Theileria spp. (14–16).
The genus Anaplasma includes intracellular gram-negative bacteria transmitted by ixodid ticks (17). Several Anaplasma spp., such as A. marginale, A. centrale, A. ovis, and A. bovis, are obligate bacteria parasitizing blood cells of many ruminants, while A. platys is mainly a pathogen of dogs (17). Anaplasma phagocytophilum is a pathogenic bacterium of a wide range of hosts, including humans and domestic and wild animals (18). In addition, A. phagocytophilum has been detected worldwide in wild ruminants and their ectoparasites (19–23). Although the role of wildlife in circulation of Anaplasma spp. is yet to be clearly defined, several species of wild ruminants are considered important reservoirs (24). In Thailand, studies found evidence of A. platys and A. bovis detection in Dermacentor auratus ticks collected from sambar deer (Cervus unicolor) (25). Because D. auratus ticks are found on humans in Thailand (26, 27), humans infected with Anaplasma bacteria via infected tick bites in addition to other deer ectoparasites is also possible.
Bartonella spp. are intra-erythrocytic gram-negative bacteria mainly transmitted among hosts by arthropod vectors, such as cat fleas (Ctenocephalides felis), body lice (Pediculus humanus), and sand flies (Lutzomyia verrucarum) (28–30). There are 45 Bartonella spp./subspp. Detected or isolated from various animals (31). Bartonella schoenbuchensis, B. capreoli, and B. bovis are detected in wild ruminants in several countries, which are strongly suspected to be transmitted by Lipoptena spp. (32–39). In Thailand, the novel Bartonella spp. was detected and isolated from captive Rusa deer (Rusa timorensis) blood samples (40). Since no ectoparasites are found on these deer, further studies are needed to determine whether ectoparasites transmit Bartonella among deer throughout Thailand. For zoonotic issues, human cases of bartonellosis caused by ruminant-related species, B. schoenbuchensis and B. melophagi, have been previously reported (41, 42). These findings highlight that although Bartonella bacterial infection in animals does not result in serious diseases, this wide range of infected animals could be a reservoir for potential zoonotic infection.
Khao Kheow Open Zoo is located within a wildlife sanctuary in Chon Buri province, eastern Thailand. Several endangered wildlife, as well as Eld’s deer (Rucervus eldii thamin), are bred and raised in the open zoo to increase population numbers. Here, wild animals can freely roam the open zoo and sanctuary areas, increasing the possibility of pathogen transmission among wild animals. Recent evidence shows ruminant-related blood pathogens, including Anaplasma, Babesia, Ehrlichia, and Theileria, in various species of ticks in this surrounding environment (43). Moreover, Tiawsirisup et al. (44) reported the presence of L. fortisetosa on Eld’s deer with Theileria capreoli and T. cervi in these insects in Thailand. Although DNA presence does not guarantee pathogen transmission, it may highlight the potential risk for mechanical transmission of pathogens to humans and healthy animals via bites of infected ectoparasites. Currently, knowledge surrounding epidemiology of pathogens related to endangered wild ruminants in Thailand is limited. This study aims to determine the prevalence and diversity of Anaplasma and Bartonella in the ectoparasite collected from captive Eld’s deer. Our findings may be used to understand the current status of pathogens among Eld’s deer and their ectoparasite, formulate animal welfare policies, and provide valuable information to prevent and control pathogens related to endangered wildlife species in the country.
From May to November 2021, 91 blood-sucking insects were collected from 12 Eld’s deer at the wildlife animal hospital, Khao Kheow Open Zoo. The Eld’s deer were admitted to the hospital for various reasons, such as disease diagnosis or regular health examination. Insect sample collection was done by veterinarians and zoo staff during an anesthetized stage of animals. Each specimen was kept in a microcentrifuge tube with RNA stabilization solution and transported to the Parasitology Unit, Faculty of Veterinary Science, Chulalongkorn University, for morphological identification by using a taxonomic key (45). All specimens were identified as L. fortisetosa (44). In addition, 38 males and 53 females were also defined during morphological identification.
DNA was extracted from each Lipoptena specimen using the IndiSpin Pathogen Kit (Indical Bioscience, Germany), according to the manufacturer’s instructions. For molecular identification of Lipoptena specimens, we examined DNA samples using PCR assay with primers LCO1490 and HCO2198 (46). PCR mixture and condition were described by Tiawsirisup et al. (44) and the product size was 658 bp, which was confirmed using DNA sequencing. Using the nucleotide BLAST tool, all representative and validated sequences showed the closest similarity (94.28–94.45%) to L. fortisetosa (OL850869) from China. Moreover, L. fortisetosa can be classified into two clades: “clade I” based on sequences already deposited in the GenBank database and “clade II” based on sequences of Lipoptena specimens collected in Thailand (44).
All DNA samples were used for Anaplasma and Bartonella detection using the PCR assay. Primers EHR16SD and EHR16SR were used to amplify a 345 bp segment of the 16S rRNA gene of Anaplasmataceae members (47). Primers BhCS781p and BhCS1137n were used to amplify a 380 bp segment of the citrate synthase gene (gltA) of Bartonella spp. (48). The PCR mixture was performed in a 25 μL reaction volume containing a DNA template, 10x PCR buffer (KOD One, TOYOBO Co., Ltd., Japan), 10 μM of forward and reverse primers, and sterile distilled water. PCR conditions were adapted by following the manufacturer’s instructions for PCR buffer and annealing temperatures were followed according to relevant studies (47, 48). DNA from A. marginale and B. henselae isolates (positive control) and distilled water (negative control) were used as controls for the PCR assay. The Anaplasma and Bartonella PCR-positive products from Lipoptena specimens were purified using a GenepHlow Gel/PCR cleanup kit (Geneaid Biotech Ltd., Taiwan) and sent for nucleotide sequencing (U2Bio Co., Ltd., South Korea).
Forty-two sequences from Anaplasma PCR-positive and 25 sequences from Bartonella PCR-positive samples were analyzed for the closest similarity with reference nucleotide sequences in the GenBank database using the NCBI nucleotide BLAST tool. All sequences were validated, aligned, and compared for genetic similarity using MegAlign (DNASTAR, Inc., United States). The number of nucleotide sequence types (ntSTs) of Anaplasma and Bartonella sequences were analyzed using DnaSP version 6.12.03 (49).
We analyzed the best-fit models for constructing phylogenetic trees using the Find Best DNA/Protein Model in MEGA X. Phylogenetic trees were generated using MEGA X with the maximum likelihood (ML) algorithm on the Kimura 2-parameter model plus gamma distribution (K2 + G) for Anaplasma sequences and Tamura-Nei parameter model plus gamma distribution (TN93 + G) for Bartonella sequences applied bootstrap method with 1,000 replications. ntST networks were constructed using the Median-joining (MJ) network in PopART version 1.7 (50, 51).
Pathogen infection rates in different genders of Lipoptena specimens were calculated and compared using Fisher’s exact test and p < 0.05 was considered statistically significant (GraphPad Prism 8.4.2 software, CA).
The PCR results showed that 46.15% (42/91) and 27.47% (25/91) of L. fortisetosa harbored Anaplasma and Bartonella DNA, respectively (Table 1). Based on the collecting date, Lipoptena specimens collected in June 2021 showed the highest prevalence of Anaplasma infection (66.67%; 24/36), while specimens collected in May 2021 showed the highest prevalence of Bartonella infection (39.28%; 11/28) and co-infection (28.57%; 8/28) (Table 1). However, no Anaplasma and Bartonella DNAs were detected from Lipoptena specimens collected in November 2021 (Table 1). Anaplasma infection rate in female specimens (47.16%; 25/53) was higher than in males (44.73%, 17/38; p = 0.8346). In addition, we also found a higher Bartonella infection rate in female specimens (33.96%, 18/53) than in males (18.42%, 7/38; p = 0.1525). Of the 91 specimens, 11 (12.08%) were co-infected with Anaplasma and Bartonella spp. (Table 1).
Table 1. Prevalence of Anaplasma and Bartonella spp. infection in Lipoptena fortisetosa detected by PCR.
Among 67 validated sequences in this study, 42 sequences (primer cut; 305 bp) were from Anaplasma PCR-positive samples, while the other 25 were from Bartonella PCR-positive (primer cut; 337 bp) samples (Table 2). We aligned and compared the validated sequences of each pathogen, then grouped these into nucleotide sequence types (ntSTs) by using DnaSP version 6.12.03 (Table 2). The validated Anaplasma sequences were grouped into four ntSTs and representative sequence from each ntST was submitted to the GenBank database, including ntST1 (37 sequences; Acc. No. OQ692407), ntST2 (three sequences; Acc. No. OQ692408), ntST3 (one sequence; Acc. No. OQ692409), and ntST4 (one sequence; Acc. No. OQ692410) (Table 2). Among four ntSTs of the Anaplasma sequences, BLAST results showed ntST1 had 100% identity with various ruminant-related Anaplasma, including A. capra (ON872236) from horse in Iraq, A. marginale (OP851751) from cattle in India, A. ovis (OM282854) from sheep in Russia, and Anaplasma spp. (KY766240) from Rhipicephalus microplus tick in Thailand. The ntST2 shared 100% identity with Anaplasma spp. (AF497579) from the Haemaphysalis lagrangei tick in Thailand and A. bovis (OQ132528) from H. hystricis tick in China. The ntST3 and 4 shared 100% identity with the Anaplasma spp. (MH589424) from a mountain bongo in Kenya and A. bovis (KP062954) from a goat in China, respectively (Table 2).
Table 2. Nucleotide sequence types (ntSTs), NCBI BLAST results, and accession number of the representative nucleotide sequences obtained in this study.
Twenty-five sequences obtained from Bartonella PCR-positive samples were grouped into eight ntSTs and representative sequence from each ntST was submitted to the GenBank database, including ntST5 (10 sequences; Acc. No. OQ716819), ntST6 (one sequence; Acc. No. OQ716820), ntST7 (three sequences; Acc. No. OQ716821), ntST8 (six sequences; Acc. No. OQ716822), ntST9 (one sequence; Acc. No. OQ716823), ntST10 (two sequences; Acc. No. OQ716824), ntST11 (one sequence; Acc. No. OQ716825), and ntST12 (one sequence; Acc. No. OQ716826) (Table 2). The BLAST results showed ntST5 and 8 shared the highest similarity to Bartonella spp. (LP485116) from deer ked in Japan with 99.11 and 98.52%, respectively. The ntST6 and 10 had the highest similarity to Bartonella spp. (CP019781) from sika deer in Japan with 97.65%, while ntST7, 11, and 12 showed the highest similarity to Bartonella spp. (LC485115) from deer ked in Japan, with 98.22, 98.52, and 97.63%, respectively. Lastly, ntST9 showed the highest similarity to the uncultured bacterium (JX416234) with 97.35% from a bat fly in the USA (Table 2).
The phylogenetic tree of Anaplasma sequences showed samples in ntST2 and 4 clustered in the same clade as A. bovis, while samples in ntST1 and 3 grouped with clade of other ruminant-related Anaplasma spp. (Figure 1). Figure 2 shows the ntST network of 16S rRNA gene of Anaplasma spp. from a total of 12 ntSTs (68 sequences). ntST1 to ntST4 represented Anaplasma sequences obtained in this study. From the ntST network, ntST2 and 4 were classified into A. bovis group, which differed by one mutation step from ntST of the A. bovis clade (KY766234, MK028574, MH255937, KP314248, AB983376, and KP062958) found in ruminants and ticks in several countries (Figure 2). Furthermore, two mutation steps separated ntST2 from ntST4 (Figure 2). The samples in ntST1 were grouped with clade of other ruminant-related Anaplasma from GenBank; Anaplasma spp. from tick (KY766240), A. capra (ON872236), A. marginale (FJ226454, OP851751), A. ovis (KJ639880, OM282854; Figure 2). The samples in ntST3 were grouped with a sequence from mountain bongo in Kenya (MH589424), which differed by two (MN611757, MT371255, and MW899038) and three (OL690556) mutation steps from the clade of A. phagocytophilum (Figure 2).
Figure 1. ML tree of 16 s rRNA gene of Anaplasma sequences (305 bp) computed with the K2 + G model. The phylogenetic relationships among sequences obtained in this study (black dot) and reference sequences from the GenBank database. Ehrlichia sequence isolated from dog (KR920044) represents as an out group.
Figure 2. ntST network of 16S rRNA gene of Anaplasma spp. ntST1 to ntST4 represented sequences obtained in this study, while other sequences obtained from the GenBank database relating to the reference sequences shown in Figure 1. The size of circle represents the frequency of each ntST, whereas the color represents the gender of specimens (A) and collecting date (B). “Other” refers to the reference sequences.
Figure 3 shows phylogenetic tree of Bartonella sequences and ntST5 to 12 referred sequences obtained in this study. Except for the ntST9 that represents a novel Bartonella species, all obtained sequences were grouped into a distinct Bartonella phylogenic lineages C, D, and E, representing a novel Bartonella species (15, 39) (Figure 3). Samples in ntST6 and 10 belong to a distinct phylogenetic branch within lineage C, while lineage D is represented in the current study by samples in ntST7, 11, and 12 (Figure 3). Samples in ntST5 and 8 belong to the distinct phylogenetic lineage E (Figure 3). Notably, the phylogenic branch of ntST9 was separated from the clade of lineage C, representing a new independent lineage of a novel Bartonella species (Figure 3). The ntST network of gltA gene of Bartonella spp. from a total of 23 ntSTs (32 sequences) was showed in Figure 4. Based on the distinct phylogenic lineages of a novel Bartonella spp., the ntSTs of the Bartonella sequences obtained in this study could divided into three lineages: C (ntST6 and 10), D (ntST7, 11, and 12), and E (ntST5 and 8; Figure 4). Both ntST6 and 10 differed by eight mutation steps from clade of lineage C found from sika deer in Japan (CP019781 and AB703131; Figure 4). The ntST7, 11, and 12 were separated from the clade of lineage D found in L. fortisetosa collected in Japan (LC485115) by six, five, and eight mutation steps, respectively (Figure 4). The samples in ntST5 differed by three mutation steps from clade of lineage E found in L. fortisetosa collected in Japan (LC485116), while samples in ntST8 differed by five mutation steps (Figure 4). Furthermore, ntST9, respectively, differed from the designated novel Bartonella sequence lineages B, C, D, and E by 12, 10, 18, and 13 mutation steps, which suggests a new independent lineage of a novel Bartonella species (Figure 4).
Figure 3. ML tree of gltA gene of Bartonella sequences (337 bp) computed with the TN93 + G model. The phylogenetic relationships among sequences obtained in this study (black dot) and ruminant-related Bartonella sequences from the GenBank database. Lineages B, C, D, and E were determined by Sato et al. (15, 39). Bartonella bacilliformis sequence isolated from human (KR920044) represents as an out group.
Figure 4. ntST network of gltA gene of Bartonella spp. ntST5 to ntST12 represented sequences obtained in this study, while other sequences obtained from the GenBank database relating to the reference sequences shown in Figure 3. The size of circle represents the frequency of each ntST, whereas the color represents the gender of specimens (A) and collecting date (B). “Other” refers to the reference sequences. Lineages B, C, D, and E were determined by Sato et al. (15, 39).
Lipoptena fortisetosa is a crucial ectoparasite infesting cervids worldwide. This insect is primarily found in sika deer (Cervus nippon) in Japan and has also been reported in Siberian roe deer (Capreolus pygargus) in Korea, Kazakhstan, and Russia (1, 9, 52). The distribution of L. fortisetosa in European countries has been hypothesized by climate change, introduction of alien cervid species into new areas, and adaptation of the insect to different hosts (11, 12). In Thailand, L. fortisetosa was first found on captive Eld’s deer in Chon Buri, as previously reported (44). Lipoptena insects may cause anemia, skin irritation, itching, restlessness, and hair loss in animal hosts (53). However, there were no skin or other symptoms on infested Eld’s deer in Thailand.
To our knowledge, this is the first report on Anaplasma and Bartonella detection in L. fortisetosa in Thailand. The prevalence of Anaplasma spp. detection in L. fortisetosa (46.15%) in the present study was higher than that reported in Poland (8.00%) (54). Although no previous evidence exists of Anaplasma harbored by this insect in the country, the presence of ticks, the primary Anaplasma vector, in the same area of wildlife habitat may enhance the possibility of bacterial infection in other blood-feeding ectoparasites, including Lipoptena insects. This possibility is supported by the results where ntST2 was identical to Anaplasma detected in H. lagrangei ticks in a previous study (43). In addition to Anaplasma, various pathogens, such as Babesia, Ehrlichia, Theileria, and Wolbachia, have also been detected in questing ticks in wildlife habitat in Chon Buri, Thailand (43). This finding highlights that, in the same surrounding area, various ectoparasites may harbor, or transmit the pathogen. Moreover, Anaplasma DNA is found in other species of Lipoptena insects, such as L. cervi and L. depressa (19, 23, 55).
Anaplasma DNA fragments from this study can be clustered with the clades of A. bovis and other ruminant-related Anaplasma. A. bovis has previously been detected in domestic goats (56), but no report on wildlife in Thailand. Interestingly, A. bovis DNA has been found in ticks collected from the Malayan sun bear (Helarctos malayanus), sambar deer (Cervus unicolor), and questing ticks dragged in wildlife habitat in Thailand (25, 43, 47). These findings suggest wild animals may act as a natural reservoir and their ectoparasites may be associated with Anaplasma infection of domestic ruminants. This possibility is supported by the present study showing ntST2 and 4 showed similar genetic material with A. bovis detected in goats in China (OQ132528 and KP062954, respectively). Other ruminant-related Anaplasma, including A. marginale, A. ovis, and A. capra, have been detected in various wild animals, suggesting a broad host range and genetic diversity (57–59). In the present study, using primers for the partial 16S rRNA gene of Anaplasma did not distinguish obtained sequence data from other ruminant-related Anaplasma. Amplification and sequencing of full-length 16S rRNA gene or other specific genes of Anaplasma would be necessary to clarify genetic characterization.
Besides biological transmission of Anaplasma by ticks, other potential vectors have been reported to mechanically transfer bacteria to animal hosts, including biting flies (60) and syringophilid mites (61). For Lipoptena, the insects detach their wings after finding suitable hosts and can only be transferred among hosts via direct contact. Since the insects acquire Anaplasma-infected blood meal on bacteremic hosts, it is possible to transmit bacteria horizontally to noninfected animals via direct contact (55). However, further experimental studies are needed to confirm direct evidence of the vector ability for Anaplasma bacteria transmission.
The prevalence of Bartonella detection in L. fortisetosa (29.67%) in this study was lower than those collected from free-living cervids in Japan (87.87%) and Poland (75.67%) (15, 62). Possible reasons for low prevalence of Bartonella detection are that Eld’s deer are raised in captive areas in Thailand. The semi-wild conditions of deer may confer a lower infestation probability by pathogen-infected ectoparasites compared with free-living wild cervids in Japan and Poland. This possibility is supported by a previous study in Poland showing farm cervids had a lower prevalence of A. phagocytophilum infection than wild individuals (21). Myczka et al. (21) also mentioned the lack of Anaplasma detection in farmed cervids may be due to regular deworming, which strengthens their condition and makes them less susceptible to infection by the bacteria. Notably, novel Bartonella has been detected and isolated with low prevalence (3.60%) from captive Rusa deer in Thailand, including those being regularly dewormed (40). Secondly, despite prior tick presence in the same surrounding area (43), ticks may not be the essential vector for Bartonella transmission among cervids (15, 63). For this reason, we implied that since Eld’s deer are infested by ticks and Lipoptena insects, Bartonella can still be detected, merely not in high prevalence. However, Bartonella detected in captive Eld’s deer and ticks collected from surrounding areas should be analyzed to clarify these reasons.
Bartonella sequences obtained from this study can be grouped with novel Bartonella lineages C, D, and E, which originated from L. fortisetosa collected from deer in Japan (15, 39). In addition, the novel Bartonella lineage B, primarily derived from Japanese sika deer, has previously been found in L. cervi and L. fortisetosa collected from red deer in Poland (62, 64). These findings suggest novel Bartonella stains from wild ruminants in Japan may spread to other countries following Lipoptena vector introduction into these new areas. We also found a new independent lineage of novel Bartonella from collected Lipoptena insects. However, genetic characterization analysis is needed to determine whether these new lineages are Lipoptena insects-specific Bartonella. Obtained Bartonella sequences were clustered with ruminant-related Bartonella. In addition to the report of novel Bartonella isolated from captive Rusa deer in Thailand (40), further reports exist of Bartonella detected or isolated from domestic ruminants in the country. Bai et al. (65) revealed that B. bovis was isolated from water buffalo blood. In 2021, seroprevalence of antibodies against B. henselae, B. vinsonii subspp. Berkhoffii, and B. tamiae in water buffaloes has also been reported (66). These findings support the genetic diversity of Bartonella among ruminants in Thailand. Further molecular surveys of Bartonella in both wild and domestic ruminants and their ectoparasites are needed to clarify the role of bacterial infection among ruminants in the country.
The high prevalence of Bartonella DNA presence in Lipoptena insects raises the question that insects may play an essential role in Bartonella transmission among hosts (5, 15, 34, 37, 38). The evidence of Bartonella survival and propagation in Lipoptena specimens has been reported from previous studies using bacterial isolation from the insects and immunohistochemical analysis (15, 35). In addition, the bacterial DNA detected in both wingless L. cervi females and their offspring suggests the potential for vertical Bartonella transmission (34). However, both in vitro and in vivo studies are required to verify how vector competence of Lipoptena insects facilitates Bartonella transmission.
Co-infections occur in Lipoptena insects but pathogen diversity may vary by species, hosts, and geographic distribution. In the USA, 6.25% of L. cervi removed from white-tailed deer carried both B. burgdorferi s.l. and A. phagocytophilum DNA (19). Further, 50% of L. fortisetosa collected from Korean water deer harbored Coxiella, T. ovis, and T. luwenshuni DNA, but no Rickettsia, Babesia, Bartonella, Borrelia, or Hepatozoon were detected (67). In this study, 11 Lipoptena specimens (12.08%) harbored both Anaplasma and Bartonella DNA. We also found four specimens were additionally infected with Theileria spp. as previously reported by Tiawsirisup et al. (44). The prevalence of more than one pathogen in Lipoptena insects emphasizes their medical and veterinary importance.
In the present study, our findings provide the first molecular detection of Anaplasma and Bartonella on L. fortisetosa in Eld’s deer in the country. Despite no blood samples from Eld’s deer, pathogen DNA detected in insects could represent the health status of animal hosts. Further studies on molecular genetic characterization of related pathogens are needed to investigate correlations of vectors, hosts, and pathogens. In addition, visitors are allowed to have direct contact with animals through petting, feeding, or taking close photos with animals in the zoo. These activities can promote the risks of potential zoonotic infection. Preventive procedures, such as health monitoring, anti-parasite medication, and proper treatments for animals should be regularly conducted. Zoo staff should pay attention to regular hygiene measures before and after working with animals and surrounding areas, such as hand washing, wearing PPE, and foot bathing with an antiseptic solution. Finally, visitors should avoid direct contact with animals and be wary of insects or ectoparasites while visiting the zoo.
The datasets presented in this study can be found in online repositories. The names of the repository/repositories and accession number(s) can be found at: https://www.ncbi.nlm.nih.gov/, OQ692407 https://www.ncbi.nlm.nih.gov/, OQ692408 https://www.ncbi.nlm.nih.gov/, OQ692409 https://www.ncbi.nlm.nih.gov/, OQ692410 https://www.ncbi.nlm.nih.gov/, OQ716819 https://www.ncbi.nlm.nih.gov/, OQ716820 https://www.ncbi.nlm.nih.gov/, OQ716821 https://www.ncbi.nlm.nih.gov/, OQ716822 https://www.ncbi.nlm.nih.gov/, OQ716823 https://www.ncbi.nlm.nih.gov/, OQ716824 https://www.ncbi.nlm.nih.gov/, OQ716825 https://www.ncbi.nlm.nih.gov/, OQ716826.
The animal study was approved by the Chulalongkorn University Animal Care and Use Committee (Animal use protocol number: 2231011). The study was conducted in accordance with the local legislation and institutional requirements.
WW contributed data, performed the analysis, and wrote the manuscript. CS-i and KT collected the data and provided technical support. NY, CA, GR, NB, and NS collected samples and data. LB edited the manuscript. UM and PK provided professional support at the sample collecting sites. AS provided technical support. ST supervised the study, edited the manuscript, and corresponded. All authors contributed to the article and approved the submitted version.
This research was funded by the Thailand Science Research and Innovation (TSRI) Fund (CU_FRB640001_01_31_3), the Chulalongkorn University Research Unit (GRU 3310160009), and the Second Century Fund (C2F), Chulalongkorn University.
The authors would like to thank the staff at the Khao Kheow Open Zoo, Thailand, for their cooperation in insect sample collection.
The authors declare that the research was conducted in the absence of any commercial or financial relationships that could be construed as a potential conflict of interest.
All claims expressed in this article are solely those of the authors and do not necessarily represent those of their affiliated organizations, or those of the publisher, the editors and the reviewers. Any product that may be evaluated in this article, or claim that may be made by its manufacturer, is not guaranteed or endorsed by the publisher.
1. Maa, TC . A synopsis of the Lipopteninae (Diptera: Hippoboscidae). J Med Entomol. (1965) 2:233–48. doi: 10.1093/jmedent/2.3.233
2. Andreani, A, Sacchetti, P, and Belcari, A. Comparative morphology of the deer ked Lipoptena fortisetosa first recorded from Italy. Med Vet Entomol. (2019) 33:140–53. doi: 10.1111/mve.12342
3. Haarløv, N . Life cycle and distribution pattern of Lipoptena cervi (L.) (Dipt., Hippobosc.) on Danish deer. Oikos. (1964) 15:93–129.
4. Mysterud, A, Madslien, K, Herland, A, Viljugrein, H, and Ytrehus, B. Phenology of deer ked (Lipoptena cervi) host-seeking flight activity and its relationship with prevailing autumn weather. Parasit Vectors. (2016) 9:95. doi: 10.1186/s13071-016-1387-7
5. Halos, L, Jamal, T, Maillard, R, Girard, B, Guillot, J, Chomel, B, et al. Role of Hippoboscidae flies as potential vectors of Bartonella spp. infecting wild and domestic ruminants. Appl Environ Microbiol. (2004) 70:6302–5. doi: 10.1128/AEM.70.10.6302-6305.2004
6. Hermosilla, C, Pantchev, N, Bachmann, R, and Bauer, C. Lipoptena cervi (deer ked) in two naturally infested dogs. Vet Rec. (2006) 159:286–7. doi: 10.1136/vr.159.9.286
7. Kynkäänniemi, S-M, Kettu, M, Kortet, R, Härkönen, L, Kaitala, A, Paakkonen, T, et al. Acute impacts of the deer ked (Lipoptena cervi) infestation on reindeer (Rangifer tarandus tarandus) behaviour. Parasitol Res. (2014) 113:1489–97. doi: 10.1007/s00436-014-3790-3
8. Metelitsa, AK, and Veselkin, GA. Parasitism of the louse fly Lipoptena fortisetosa on cattle. Parazitologiia. (1989) 23:276–7.
9. Choi, CY, Lee, S, Moon, KH, Kang, CW, and Yun, YM. New record of Lipoptena fortisetosa (Diptera: Hippoboscidae) collected from Siberian roe deer on Jeju island, Korea. J Med Entomol. (2013) 50:1173–7. doi: 10.1603/ME12150
10. Galecki, R, Jaroszewski, J, Xuan, X, and Bakula, T. Temporal-microclimatic factors affect the phenology of Lipoptena fortisetosa in central European forests. Animals (Basel). (2020):10. doi: 10.3390/ani10112012
11. Kurina, O, Kirik, H, Ounap, H, and Ounap, E. The northernmost record of a blood-sucking ectoparasite, Lipoptena fortisetosa Maa (Diptera: Hippoboscidae), in Estonia. Biodivers Data J. (2019) 7:e47857. doi: 10.3897/BDJ.7.e47857
12. Mihalca, AD, Pastrav, IR, Sandor, AD, Deak, G, Gherman, CM, Sarmasi, A, et al. First report of the dog louse fly Hippobosca longipennis in Romania. Med Vet Entomol. (2019) 33:530–5. doi: 10.1111/mve.12395
13. Kortet, R, Harkonen, L, Hokkanen, P, Harkonen, S, Kaitala, A, Kaunisto, S, et al. Experiments on the ectoparasitic deer ked that often attacks humans; preferences for body parts, colour and temperature. Bull Entomol Res. (2010) 100:279–85. doi: 10.1017/S0007485309990277
14. Galecki, R, Jaroszewski, J, Bakula, T, and Xuan, X. Molecular characterization of Lipoptena cervi from environmental samples collected in Poland. Int J Parasitol Parasites Wildl. (2021) 14:41–7. doi: 10.1016/j.ijppaw.2020.12.005
15. Sato, S, Kabeya, H, Ishiguro, S, Shibasaki, Y, and Maruyama, S. Lipoptena fortisetosa as a vector of Bartonella bacteria in Japanese sika deer (Cervus nippon). Parasit Vectors. (2021) 14:73. doi: 10.1186/s13071-021-04585-w
16. Werszko, J, Steiner-Bogdaszewska, Z, Jezewski, W, Szewczyk, T, Kurylo, G, Wolkowycki, M, et al. Molecular detection of Trypanosoma spp. in Lipoptena cervi and Lipoptena fortisetosa (Diptera: Hippoboscidae) and their potential role in the transmission of pathogens. Parasitology. (2020) 147:1629–35. doi: 10.1017/S0031182020001584
17. Rymaszewska, A, and Grenda, S. Bacteria of the genus Anaplasma–characteristics of Anaplasma and their vectors: a review. Vet Med. (2008) 53:573–84. doi: 10.17221/1861-VETMED
18. Woldehiwet, Z . The natural history of Anaplasma phagocytophilum. Vet Parasitol. (2010) 167:108–22. doi: 10.1016/j.vetpar.2009.09.013
19. Buss, M, Case, L, Kearney, B, Coleman, C, and Henning, JD. Detection of Lyme disease and anaplasmosis pathogens via PCR in Pennsylvania deer ked. J Vector Ecol. (2016) 41:292–4. doi: 10.1111/jvec.12225
20. Masuzawa, T, Uchishima, Y, Fukui, T, Okamoto, Y, Muto, M, Koizumi, N, et al. Detection of Anaplasma phagocytophilum from wild boars and deer in Japan. Jpn J Infect Dis. (2011) 64:333–6. doi: 10.7883/yoken.64.333
21. Myczka, AW, Steiner-Bogdaszewska, Ż, Filip-Hutsch, K, Oloś, G, Czopowicz, M, and Laskowski, Z. Detection of Anaplasma phagocytophilum in wild and farmed cervids in Poland. Pathogens. (2021) 10:1190. doi: 10.3390/pathogens10091190
22. Tate, CM, Mead, DG, Luttrell, MP, Howerth, EW, Dugan, VG, Munderloh, UG, et al. Experimental infection of white-tailed deer with Anaplasma phagocytophilum, etiologic agent of human granulocytic anaplasmosis. J Clin Microbiol. (2005) 43:3595–601. doi: 10.1128/JCM.43.8.3595-3601.2005
23. Víchová, B, Majláthová, V, Nováková, M, Majláth, I, Čurlík, J, Bona, M, et al. PCR detection of re-emerging tick-borne pathogen, Anaplasma phagocytophilum, in deer ked (Lipoptena cervi) a blood-sucking ectoparasite of cervids. Biologia. (2011) 66:1082–6. doi: 10.2478/s11756-011-0123-1
24. Jaarsma, RI, Sprong, H, Takumi, K, Kazimirova, M, Silaghi, C, Mysterud, A, et al. Anaplasma phagocytophilum evolves in geographical and biotic niches of vertebrates and ticks. Parasit Vectors. (2019) 12:328. doi: 10.1186/s13071-019-3583-8
25. Sumrandee, C, Baimai, V, Trinachartvanit, W, and Ahantarig, A. Molecular detection of Rickettsia, Anaplasma, Coxiella and Francisella bacteria in ticks collected from Artiodactyla in Thailand. Ticks Tick Borne Dis. (2016) 7:678–89. doi: 10.1016/j.ttbdis.2016.02.015
26. Keirans, JE, and Clifford, CM. A checklist of types of Ixodoidea (Acari) in the collection of the Rocky Mountain laboratories. J Med Entomol. (1984) 21:310–20. doi: 10.1093/jmedent/21.3.310
27. Tanskul, P, Stark, HE, and Inlao, I. A checklist of ticks of Thailand (Acari: Metastigmata: Ixodoidea). J Med Entomol. (1983) 20:330–41. doi: 10.1093/jmedent/20.3.330
28. Chomel, BB, Kasten, RW, Floyd-Hawkins, K, Chi, B, Yamamoto, K, Roberts-Wilson, J, et al. Experimental transmission of Bartonella henselae by the cat flea. J Clin Microbiol. (1996) 34:1952–6. doi: 10.1128/jcm.34.8.1952-1956.1996
29. Ellis, BA, Rotz, LD, Leake, JA, Samalvides, F, Bernable, J, Ventura, G, et al. An outbreak of acute bartonellosis (Oroya fever) in the Urubamba region of Peru, 1998. Am J Trop Med Hyg. (1999) 61:344–9. doi: 10.4269/ajtmh.1999.61.344
30. Swift, HF . Trench fever. Arch Intern Med. (1920) 26:76–98. doi: 10.1001/archinte.1920.00100010079006
31. Okaro, U, Addisu, A, Casanas, B, and Anderson, B. Bartonella species, an emerging cause of blood-culture-negative endocarditis. Clin Microbiol Rev. (2017) 30:709–46. doi: 10.1128/CMR.00013-17
32. Bai, Y, Cross, PC, Malania, L, and Kosoy, M. Isolation of Bartonella capreoli from elk. Vet Microbiol. (2011) 148:329–32. doi: 10.1016/j.vetmic.2010.09.022
33. Bermond, D, Boulouis, HJ, Heller, R, Van Laere, G, Monteil, H, Chomel, BB, et al. Bartonella bovis Bermond et al. sp. nov. and Bartonella capreoli sp. nov., isolated from European ruminants. Int J Syst Evol Microbiol. (2002) 52:383–90. doi: 10.1099/00207713-52-2-383
34. de Bruin, A, van Leeuwen, AD, Jahfari, S, Takken, W, Foldvari, M, Dremmel, L, et al. Vertical transmission of Bartonella schoenbuchensis in Lipoptena cervi. Parasit Vectors. (2015) 8:176. doi: 10.1186/s13071-015-0764-y
35. Dehio, C, Sauder, U, and Hiestand, R. Isolation of Bartonella schoenbuchensis from Lipoptena cervi, a blood-sucking arthropod causing deer ked dermatitis. J Clin Microbiol. (2004) 42:5320–3. doi: 10.1128/JCM.42.11.5320-5323.2004
36. Izenour, K, Zikeli, S, Kalalah, A, Ditchkoff, SS, Starkey, LA, Wang, C, et al. Diverse Bartonella spp. detected in white-tailed deer (Odocoileus virginianus) and associated keds (Lipoptena mazamae) in the southeastern USA. J Wildl Dis. (2020) 56:505–11. doi: 10.7589/2019-08-196
37. Matsumoto, K, Berrada, ZL, Klinger, E, Goethert, HK, and Telford, SR 3rd. Molecular detection of Bartonella schoenbuchensis from ectoparasites of deer in Massachusetts. Vector Borne Zoonotic Dis. (2008) 8:549–54. doi: 10.1089/vbz.2007.0244
38. Reeves, WK, Nelder, MP, Cobb, KD, and Dasch, GA. Bartonella spp. in deer keds, Lipoptena mazamae (Diptera: Hippoboscidae), from Georgia and South Carolina, USA. J Wildl Dis (2006) 42:391–6. doi: 10.7589/0090-3558-42.2.391
39. Sato, S, Kabeya, H, Yamazaki, M, Takeno, S, Suzuki, K, Kobayashi, S, et al. Prevalence and genetic diversity of Bartonella species in sika deer (Cervus nippon) in Japan. Comp Immunol Microbiol Infect Dis (2012) 35:575–581. doi: 10.1016/j.cimid.2012.07.001
40. Pangjai, D, Intachinda, S, Maruyama, S, Boonmar, S, Kabeya, H, Sato, S, et al. Isolation and phylogenetic analysis of Bartonella species from Rusa deer (Rusa timorensis) in Thailand. Comp Immunol Microbiol Infect Dis. (2018) 56:58–62. doi: 10.1016/j.cimid.2017.12.005
41. Maggi, RG, Kosoy, M, Mintzer, M, and Breitschwerdt, EB. Isolation of candidatus Bartonella melophagi from human blood. Emerg Infect Dis. (2009) 15:66–8. doi: doi: 10.3201/eid1501.081080
42. Vayssier-Taussat, M, Moutailler, S, Femenia, F, Raymond, P, Croce, O, La Scola, B, et al. Identification of novel zoonotic activity of Bartonella spp., France. Emerg Infect Dis (2016) 22:457–62. doi: 10.3201/eid2203.150269
43. Wattanamethanont, J, Kaewthamasorn, M, and Tiawsirisup, S. Natural infection of questing ixodid ticks with protozoa and bacteria in Chonburi province, Thailand. Ticks Tick Borne Dis. (2018) 9:749–58. doi: 10.1016/j.ttbdis.2018.02.020
44. Tiawsirisup, S, Yurayart, N, Thongmeesee, K, Sri-In, C, Akarapas, C, Rittisornthanoo, G, et al. Possible role of Lipoptena fortisetosa (Diptera: Hippoboscidae) as a potential vector for Theileria spp. in captive Eld's deer in Khao Kheow open zoo, Thailand. Acta Trop. (2023) 237:106737. doi: 10.1016/j.actatropica.2022.106737
45. Salvetti, M, Bianchi, A, Marangi, M, Barlaam, A, Giacomelli, S, Bertoletti, I, et al. Deer keds on wild ungulates in northern Italy, with a taxonomic key for the identification of Lipoptena spp. of Europe. Med Vet Entomol. (2020) 34:74–85. doi: 10.1111/mve.12411
46. Folmer, O, Black, M, Hoeh, W, Lutz, R, and Vrijenhoek, R. DNA primers for amplification of mitochondrial cytochrome c oxidase subunit I from diverse metazoan invertebrates. Mol Mar Biol Biotechnol. (1994) 3:294–9.
47. Parola, P, Roux, V, Camicas, JL, Baradji, I, Brouqui, P, and Raoult, D. Detection of ehrlichiae in African ticks by polymerase chain reaction. Trans R Soc Trop Med Hyg. (2000) 94:707–8. doi: 10.1016/s0035-9203(00)90243-8
48. Norman, AF, Regnery, R, Jameson, P, Greene, C, and Krause, DC. Differentiation of Bartonella-like isolates at the species level by PCR-restriction fragment length polymorphism in the citrate synthase gene. J Clin Microbiol. (1995) 33:1797–803. doi: 10.1128/jcm.33.7.1797-1803.1995
49. Rozas, J, Ferrer-Mata, A, Sanchez-DelBarrio, JC, Guirao-Rico, S, Librado, P, Ramos-Onsins, SE, et al. DnaSP 6: DNA sequence polymorphism analysis of large data sets. Mol Biol Evol. (2017) 34:3299–302. doi: 10.1093/molbev/msx248
50. Bandelt, HJ, Forster, P, and Rohl, A. Median-joining networks for inferring intraspecific phylogenies. Mol Biol Evol. (1999) 16:37–48. doi: 10.1093/oxfordjournals.molbev.a026036
51. Leigh, JW, and Bryant, D. POPART: full-feature software for haplotype network construction. Methods Ecol Evol. (2015) 6:1110–6. doi: 10.1111/2041-210X.12410
52. Edwards, SJ, Hood, MW, Shaw, JH, Rayburn, JD, Kirby, MD, Hanfman, DT, et al. Index-catalogue of medical and veterinary zoology; supplement 21, part 5: Parasite-subject catalogue. Parasites: Arthropoda and miscellaneous Phyla. WA: USDA Government Printing Office (1978). 1–246.
53. Madslien, K, Ytrehus, B, Vikoren, T, Malmsten, J, Isaksen, K, Hygen, HO, et al. Hair-loss epizootic in moose (Alces alces) associated with massive deer ked (Lipoptena cervi) infestation. J Wildl Dis. (2011) 47:893–906. doi: 10.7589/0090-3558-47.4.893
54. Galecki, R, Jaroszewski, J, Bakula, T, Galon, EM, and Xuan, X. Molecular detection of selected pathogens with zoonotic potential in deer keds (Lipoptena fortisetosa). Pathogens. (2021):10. doi: 10.3390/pathogens10030324
55. Foley, JE, Hasty, JM, and Lane, RS. Diversity of rickettsial pathogens in Columbian black-tailed deer and their associated keds (Diptera: Hippoboscidae) and ticks (Acari: Ixodidae). J Vector Ecol. (2016) 41:41–7. doi: 10.1111/jvec.12192
56. Aung, A, Kaewlamun, W, Narapakdeesakul, D, Poofery, J, and Kaewthamasorn, M. Molecular detection and characterization of tick-borne parasites in goats and ticks from Thailand. Ticks Tick Borne Dis. (2022) 13:101938. doi: 10.1016/j.ttbdis.2022.101938
57. de la Fuente, J, Ruiz-Fons, F, Naranjo, V, Torina, A, Rodriguez, O, and Gortazar, C. Evidence of Anaplasma infections in European roe deer (Capreolus capreolus) from southern Spain. Res Vet Sci. (2008) 84:382–6. doi: 10.1016/j.rvsc.2007.05.018
58. Guillemi, EC, de la Fourniere, S, Orozco, M, Pena Martinez, J, Correa, E, Fernandez, J, et al. Molecular identification of Anaplasma marginale in two autochthonous south American wild species revealed an identical new genotype and its phylogenetic relationship with those of bovines. Parasit Vectors. (2016) 9:305. doi: 10.1186/s13071-016-1555-9
59. Yang, J, Liu, Z, Niu, Q, Mukhtar, MU, Guan, G, Liu, G, et al. A novel genotype of "Anaplasma capra" in wildlife and its phylogenetic relationship with the human genotypes. Emerg Microbes Infect. (2018) 7:210. doi: 10.1038/s41426-018-0212-0
60. de La Fuente, J, Naranjo, V, Ruiz-Fons, F, Hofle, U, Fernandez De Mera, IG, Villanua, D, et al. Potential vertebrate reservoir hosts and invertebrate vectors of Anaplasma marginale and A. phagocytophilum in Central Spain. Vector Borne Zoonotic Dis. (2005) 5:390–401. doi: 10.1089/vbz.2005.5.390
61. Skoracki, M, Michalik, J, Skotarczak, B, Rymaszewska, A, Sikora, B, Hofman, T, et al. First detection of Anaplasma phagocytophilum in quill mites (Acari: Syringophilidae) parasitizing passerine birds. Microbes Infect. (2006) 8:303–7. doi: 10.1016/j.micinf.2005.06.029
62. Werszko, J, Swislocka, M, Witecka, J, Szewczyk, T, Steiner-Bogdaszewska, Z, Wilamowski, K, et al. The new haplotypes of Bartonella spp. and Borrelia burgdorferi sensu lato identified in Lipoptena spp. (Diptera: Hippoboscidae) collected in the areas of North-Eastern Poland. Pathogens. (2022):11. doi: 10.3390/pathogens11101111
63. Tijsse-Klasen, E, Fonville, M, Gassner, F, Nijhof, AM, Hovius, EK, Jongejan, F, et al. Absence of zoonotic Bartonella species in questing ticks: first detection of Bartonella clarridgeiae and Rickettsia felis in cat fleas in the Netherlands. Parasit Vectors. (2011) 4:61. doi: 10.1186/1756-3305-4-61
64. Szewczyk, T, Werszko, J, Steiner-Bogdaszewska, Z, Jezewski, W, Laskowski, Z, and Karbowiak, G. Molecular detection of Bartonella spp. in deer ked (Lipoptena cervi) in Poland. Parasit Vectors. (2017) 10:487. doi: 10.1186/s13071-017-2413-0
65. Bai, Y, Malania, L, Alvarez Castillo, D, Moran, D, Boonmar, S, Chanlun, A, et al. Global distribution of Bartonella infections in domestic bovine and characterization of Bartonella bovis strains using multi-locus sequence typing. PLoS One. (2013) 8:e80894. doi: 10.1371/journal.pone.0080894
66. Boonmar, S, Saengsawang, P, Mitsuwan, W, Panjai, D, Kidsin, K, Sansamur, C, et al. The first report of the seroprevalence of antibodies against Bartonella spp. in water buffaloes (Bubalus bubalis) from South Thailand. Vet World. (2021) 14:3144–8. doi: 10.14202/vetworld.2021.3144-3148
Keywords: prevalence, Anaplasma , Bartonella , Lipoptena fortisetosa , Eld’s deer, Thailand
Citation: Wechtaisong W, Sri-in C, Thongmeesee K, Yurayart N, Akarapas C, Rittisornthanoo G, Bunphungbaramee N, Sipraya N, Bartholomay LC, Maikaew U, Kongmakee P, Saedan A and Tiawsirisup S (2023) Diversity of Anaplasma and novel Bartonella species in Lipoptena fortisetosa collected from captive Eld’s deer in Thailand. Front. Vet. Sci. 10:1247552. doi: 10.3389/fvets.2023.1247552
Received: 26 June 2023; Accepted: 30 August 2023;
Published: 14 September 2023.
Edited by:
Alireza Sazmand, Bu-Ali Sina University, IranReviewed by:
Giovanni Sgroi, Experimental Zooprophylactic Institute of Southern Italy (IZSM), ItalyCopyright © 2023 Wechtaisong, Sri-in, Thongmeesee, Yurayart, Akarapas, Rittisornthanoo, Bunphungbaramee, Sipraya, Bartholomay, Maikaew, Kongmakee, Saedan and Tiawsirisup. This is an open-access article distributed under the terms of the Creative Commons Attribution License (CC BY). The use, distribution or reproduction in other forums is permitted, provided the original author(s) and the copyright owner(s) are credited and that the original publication in this journal is cited, in accordance with accepted academic practice. No use, distribution or reproduction is permitted which does not comply with these terms.
*Correspondence: Sonthaya Tiawsirisup, c29udGhheWEudEBjaHVsYS5hYy50aA==
Disclaimer: All claims expressed in this article are solely those of the authors and do not necessarily represent those of their affiliated organizations, or those of the publisher, the editors and the reviewers. Any product that may be evaluated in this article or claim that may be made by its manufacturer is not guaranteed or endorsed by the publisher.
Research integrity at Frontiers
Learn more about the work of our research integrity team to safeguard the quality of each article we publish.