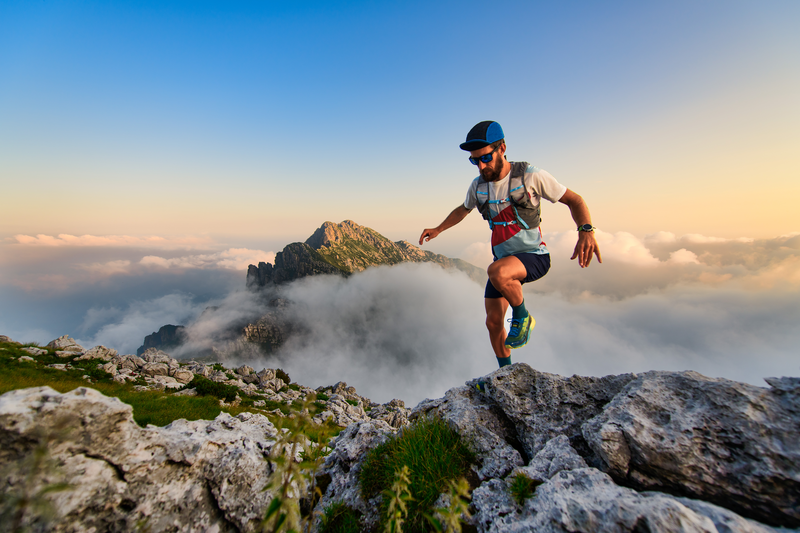
94% of researchers rate our articles as excellent or good
Learn more about the work of our research integrity team to safeguard the quality of each article we publish.
Find out more
ORIGINAL RESEARCH article
Front. Vet. Sci. , 02 October 2023
Sec. Veterinary Emergency and Critical Care Medicine
Volume 10 - 2023 | https://doi.org/10.3389/fvets.2023.1245864
Introduction: Domoic acid (DA) is a glutaminergic excitatory neurotoxin that causes the morbidity and mortality of California sea lions (Zalophus californianus; CSL) and other marine mammals due to a suite of effects mostly on the nervous and cardiac systems. Between 1998 and 2019, 11,737 live-stranded CSL were admitted to The Marine Mammal Center (TMMC; Sausalito, CA, USA), over 2,000 of which were intoxicated by DA. A plethora of clinical research has been performed over the past 20 years to characterize the range of toxic effects of DA exposure on CSLs, generating the largest dataset on the effects of natural exposure to this toxin in wildlife.
Materials and methods: In this study, we review published methods for diagnosing DA intoxication, clinical presentation, and treatment of DA-intoxicated CSL and present a practical, reproducible scoring system called the neuroscore (NS) to help assess whether a DA-affected CSL is fit for release to the wild following rehabilitation. Logistic regression models were used to assess the relationships between outcome (released vs. euthanized or died) and multiple variables to predict the outcome for a subset of 92 stranded CSLs.
Results: The largest proportion of DA-intoxicated CSLs was adult females (58.6%). The proportions of acute and chronic cases were 63.5 and 36.5% respectively, with 44% of affected CSL released and 56% either dying naturally or euthanized. The average time in rehabilitation was 15.9 days (range 0–169) for all outcomes. The best-performing model (85% accuracy; area under the curve = 0.90) assessing the relationship between outcome and predictor variables consisted of four variables: final NS, change in NS over time, whether the animal began eating in rehabilitation, and the state of nutrition on admission.
Discussion: Our results provide longitudinal information on the symptomatology of CSL intoxicated by domoic acid and suggest that a behavioral scoring system is a useful tool to assess the fitness for the release of DA-intoxicated CSL.
Domoic acid (DA) is a potent glutaminergic excitatory neurotoxin that is produced by some species of marine diatoms in the genus Pseudo-nitzschia (1, 2). The toxin accumulates in filter-feeding finfish, shellfish, and other prey items, which can cause toxicosis when consumed by vertebrates (1, 3). Domoic acid toxicosis in humans was first recognized in 1987, when over 100 people became ill after consuming DA-contaminated mussels (1, 2). Since then, many of the diverse effects of DA on mammals have been elucidated through experimental exposures of laboratory animals, including zebrafish, rodents, and non-human primates, and observations of naturally exposed marine wildlife (4–14). Among wildlife, most available information is derived from clinical and pathological studies of California sea lions (Zalophus californianus; CSL) that stranded along the California coast following natural exposure. Domoic acid intoxication was first documented in marine mammals in 1998, when over 400 CSL were stranded along the California coast showing neurological signs and acute mortality (5, 15). Since 1998, CSL have been intoxicated with DA annually, and a suite of characteristic clinical effects and histological lesions are consistently recognized. These lesions have also been described in southern sea otters (Enhydra lutris nereis) and northern fur seals (Callorhinus ursinus) stranded along the California coast (16, 17).
A decade after the first cases were recognized, a second clinical syndrome was described in CSL: chronic DA toxicosis. As opposed to acute toxicosis, which immediately follows ingestion and absorption, chronic toxicosis is due either to repeated, low-level exposure of the toxin or to the long-lasting effects of past sub-lethal exposure, as no toxin is present in the body at the time of clinical presentation (4, 18). Acutely affected CSL typically strands in good body condition with neurological signs consisting of any combination of seizures, ataxia, head weaving, muscle tremors, decreased response to stimuli, and/or coma that either resolve within 7 days once DA is cleared from the body or progress to a chronic epileptic state or mortality (5). The chronic syndrome is characterized by intermittent seizures and epilepsy associated with hippocampal atrophy, often accompanied by behavioral changes and disruptions of spatial memory (4, 13). Neuronal necrosis, particularly in the ventral hippocampal complex, amygdala, pyriform lobe, and olfactory cortex, is observed in acute cases that progresses to hippocampal atrophy in the chronic syndrome (12, 18). Degenerative cardiomyopathy attributed to the direct action of DA on cardiac glutamate receptors has been described in both syndromes (14).
Over recent years, the differentiation of these two syndromes has become increasingly difficult, presumably due to a combination of increased frequency of DA-producing harmful algal blooms in the California Current Ecosystem, and continued and/or repeated exposure of individual CSL (19). Following ingestion, DA can be detected in urine, serum, stomach contents, feces, amniotic fluid, and milk. However, DA is water-soluble, has a half-life of only hours in blood and 24 h in urine, and as the period from exposure to sample collection is often unknown, a negative result from tested body fluids does not rule out DA intoxication (20, 21). Importantly, the prognosis differs between acute and chronic syndromes; in acute cases, symptoms may resolve, whereas in chronic cases they will not, resulting in a poor prognosis for survival (13). Therefore, it is critical to define clinical signs and biomarkers that can be used as prognostic indicators in intoxicated animals outside of biological sampling, both for the management of CSL in rehabilitation and for the best assessment of their fitness for release (13, 22). Rapid determination of release potential allows for more humane management of animals, such as earlier euthanasia to relieve the suffering of animals with little to no chance of survival post-release.
In this study, we review the published methods for the diagnosis of DA toxicosis in CSL as well as the clinical presentation and treatment of DA toxicosis in CSL based on published literature and 20 years of data collected by The Marine Mammal Center (TMMC), a rescue and rehabilitation facility for stranded marine mammals along central and northern California, based in Sausalito (CA, USA). To aid future clinical management of DA toxicosis in CSL, we present a behavioral assessment tool to aid clinicians in determining whether a CSL affected by DA is fit for release.
Search criteria included peer-reviewed journal articles, conference proceedings, internal case definitions, and book chapters that investigated methods for the diagnosis of DA toxicosis in CSL and laboratory animals. Additionally, the effects of DA toxicosis in CSL, laboratory animals, and other marine species, as well as the clinical presentations of DA intoxication, were explored. A set time frame for the publication date was not included in the search criteria, and relevant published literature was included regardless of the publication date. Interrogated databases included Google Scholar and PubMed, as well as an internal list of DA-related publications and book chapters from TMMC. Keywords used to search online databases included “domoic acid,” “sea lion,” “zalophus,” “sea otter,” “cardiac,” “in utero,” “neurologic,” “fur seal,” “marine mammal.” and “ophthalmic”.
Inclusion criteria for antemortem diagnosis of DA toxicosis (acute or chronic) in CSLs were seizures, ataxia, head weaving, tremors, blindness, blepharospasm, reduced responsiveness, coma, and/or abnormal behavior that included disorientation, unusually human-oriented or inquisitive, aggressive behavior, or repetitive behaviors (i.e., frantic pacing, swimming in tight circles, rocking on the pen floor, and/or chewing on body parts or objects) (TMMC case definition). Relative eosinophilia (>0.68 × 109/L), elevated hematocrit (median = 49.5%), and/or reduced serum cortisol concentration (<20 mg/dL) on admission blood work provided further indication of DA intoxication (TMMC case definition) (23).
Antemortem differentiation between acute and chronic DA intoxication was based on stranding history, clinical signs, and imaging when available. Acute DA intoxication was presumed to occur when a group of ≥5 sea lions with neurological signs stranded within 48 h and 80 km of each other and, if available, documentation of an active Pseudo-nitzschia bloom or elevated DA levels from monitoring programs such as the Southern California Coastal Ocean Observing System (SCCOOS) and the Central and Northern California Ocean Observing System (CeNCOOS). When available, post-mortem lesions in at least one of the sea lions consistent with acute DA toxicosis in the hippocampus provided further evidence for an acute DA intoxication event (4). Acute DA intoxication was usually inapparent on gross necropsy examination but was confirmed on histology by the presence of neuronal necrosis/sclerosis in the Cornu Ammonis of the hippocampus that may also affect neurons of the dentate gyrus, parahippocampal gyrus, amygdaloid body, olfactory, and pyriform lobe cortex (12, 24).
Inclusion criteria for chronic DA intoxication were defined based on stranding histories in which a single animal stranded in an abnormal location (delta, urban area, etc.), typically in poor body condition, and as an isolated event with neurological abnormalities (4). Antemortem magnetic resonance imaging (MRI) showing hippocampal atrophy provided further evidence of chronic DA intoxication when available. The post-mortem histological diagnosis of chronic DA intoxication was based on the often asymmetric atrophy of the hippocampal complex, characterized histologically by neuronal loss and gliosis (12, 24).
Therapy was aimed at controlling symptoms as previously described in the literature and the TMMC pharmacopeia (5). Phenobarbital (Golden Gate Pharmacy, Novato, CA 94949, USA) was administered for seizure control during rehabilitation and was started upon the initial diagnosis of DA toxicosis (4 mg/kg IM q12h for 2 days followed by 2 mg/kg q12h for 5 more days (IM or PO if eating). Lorazepam (Golden Gate Pharmacy, Novato, CA 94949, USA), midazolam (Almaject, Morristown, NJ 07690, USA), and/or diazepam (Hospira, Lake Forest, Il 60045, USA) were used in conjunction with phenobarbital for the treatment of active, break-through seizures (0.2 mg/kg IM PRN).
Adjunct therapy to help minimize inflammation and cellular necrosis included an antioxidant, alpha lipoic acid (10 mg/kg SQ q24h; Golden Gate Pharmacy, Novato, CA 94949, USA) (25) and an anti-inflammatory dose of dexamethasone (Bimeda-MTC Animal Health Inc., Cambridge, Ontario N3C 2W4, Canada) or prednisone (Novitium Pharma LLC, East Windsor, NJ 08520, USA), unless contraindicated due to co-morbidities such as corneal ulceration. Supportive subcutaneous fluids (lactated Ringer's solution, LRS, Vetivex, Dechra Veterinary Products, Overland Park, Kansas 66211, USA; Normosol-R, PlasmaLyte A, Abbott Animal Health, Abbott Park, Illinois 60064, USA) were administered to anorexic animals at 20–25 ml/kg/day for 3–5 days or until animals started eating.
As DA is retained in fetal amniotic and allantoic fluids, leading to prolonged persistence in circulation with subsequent progressive disease and fetal developmental abnormalities (8, 26), abortion was induced in pregnant females by administering dexamethasone at 0.25 mg/kg IM q24h for 3 days. If abortion did not occur by day 3, a single 500-microgram dose of prostaglandin F2alpha (Lutalyse®, Zoetis, Parsippany-Troy Hills, NJ, 07054, USA) was administered. Prophylactic ceftiofur crystalline free acid (EXCEDE, Zoetis, Parsippany, New Jersey 07054, USA) was administered IM (6.6 mg/kg) to reduce the risk of pyometra in debilitated animals.
A retrospective analysis of the clinical presentation, demographics, and survival to release of 2, 447 CSL diagnosed with DA intoxication and admitted to TMMC between 1998 and 2019 was performed. A subset of 92 CSL affected by DA and admitted to TMMC between July 2015 and August 2017 was analyzed to determine the performance of a quantitative behavioral assessment tool, referred to as the neuroscore (NS), in predicting whether a CSL affected by DA was a candidate for release. The subset of CSL was chosen because they all had at least three complete NS performed with the most up-to-date NS and criteria used consistently by experienced TMMC veterinarians. The NS criteria have been adapted over time based on the changing symptomatology of DA intoxication. Stranding data and clinical presentations, a review of the current diagnostic tests available to differentiate and diagnose acute and chronic DA intoxication, and the performance of the NS in helping to predict whether a CSL is fit for release, as well as its limitations, are reported.
All animals were stranded along the California coast between approximately 34°22′23′′ N and 40°0′4′′ N and were transported to TMMC for assessment and possible rehabilitation. Physical examinations were performed on all animals upon admission under manual or chemical restraint. Age class was estimated based on body mass, straight body length, tooth development, and the development of the sagittal crest in males (27). The state of nutrition was estimated based on a body condition scale of 1 to 5, with 1 being severely malnourished and 5 being over-conditioned (TMMC internal scale). Blood samples were collected via the caudal gluteal vein directly into vacutainer collection tubes, and the complete blood count and serum chemistry were analyzed as previously published (5, 28). Urine was collected when possible via cystocentesis or catheterization, and an abdominal ultrasound examination was performed on a female CSL to assess for pregnancy. An MRI was performed under general anesthesia using published anesthetic protocols (29). Sea lions were housed with one or more conspecifics of similar age, in either dry pens (if non-responsive initially) or pens with access to a saltwater pool and concrete deck space. They were offered frozen, thawed herring two to three times daily, and records indicating whether the animal was eating or not were maintained. Sea lions were diagnosed with domoic acid intoxication using the earlier described inclusion criteria.
A quantitative behavioral assessment tool (NS) aimed at quantifying the severity of DA-associated effects on stranded CSL was developed at TMMC and was performed on CSL diagnosed with DA intoxication as a standardized method to assess the clinical response to treatment. This assessment is similar to the method previously reported (5), although the assessment has been gradually adjusted over the years based on the changing symptomatology (acute vs. chronic) observed in CSL. The NS (Figure 1) was modeled after pain scoring systems in human neonates such as the premature infant pain profile and the neonatal infant pain scale (30, 31) and is a sum of multiple components assessing posture, mentation, the presence of abnormal movements, response to auditory and tactile stimuli, and locomotor skills. The sum of these components is the NS, where a lower NS is consistent with an animal with a higher probability of survival after release (as determined by a low likelihood of re-stranding). The cutoff value used at TMMC to determine whether a CSL was a candidate for release was a NS of 10 or below. The NS assessments began 2 days after an animal finished the previously described course of treatment (i.e., 2 days after the final dose of phenobarbital) to minimize potential residual sedative effects following phenobarbital administration. A single NS assessment was performed every other day by experienced TMMC clinical veterinarians until three scores were obtained, upon which the NS-trend as well as the overall clinical progression of the animal were taken into consideration to determine whether to release the animal.
Figure 1. (A) Neuroscore criteria used at The Marine Mammal Center to assess the clinical responsiveness (or the clinical response to treatment) of stranded California sea lions intoxicated by domoic acid. Circles next to terms indicate that one option should be selected; squares indicate more than one option may be selected. The assessor chooses a single score based on the animal under assessment for the sections “Posture,” “Mentation,” “Responsiveness,” and “Locomotion” and marks all that apply for the “Presence of Abnormal Movements” section. An animal that receives a score of 10 or less is considered a candidate for release. (B) An example of a neuroscore from a California sea lion intoxicated by domoic acid would be a candidate for release with a total neuroscore of six. (C) An example of a neuroscore from a California sea lion intoxicated by domoic acid would not be a candidate for release with a total neuroscore of 14.
Differences in the proportion of CSL stranding due to DA between age classes, sex, and outcome (released vs. died/euthanized) and in the proportion of acute vs. chronic cases were tested using Pearson's chi-squared test on the full dataset (CSL stranding between 1998 and 2019). Correlations between variables were tested using generalized linear models and likelihood ratio tests, with model specifics provided in Section 3.
We tested whether it was possible to use different variables to predict the outcome (release vs. death) after stranding due to DA on a subset of 92 CSL strandings between 2015 and 2017. Candidate variables included the state of nutrition, straight body length, presence of circulating eosinophilia, diagnosed co-morbidities at admission, and whether the animal began eating while in rehabilitation, in conjunction with the NS results. We report which combination of variables was the best predictors of releasability to aid rehabilitation facilities in determining whether a DA-affected CSL is fit for release (as determined by the low likelihood of re-stranding). Generalized linear models with logit link functions and binomial error distributions were constructed for different combinations of candidate variables, and their performance was compared using 10-fold cross-validation error, AIC, and AUC (area under the curve) (32). Cross-validation was used to explicitly test the predictive performance of a model. (33) For the 10-fold cross-validation test, the dataset is randomly divided into 10 parts. For each of those 10 parts in turn, a model is fitted using the 9 parts (training data) not included in the current part, after which the fitted model is used to predict the outcome of the data in the current part (test data). This approach repeatedly simulates a situation where a model is constructed using observed data and is then used to predict the outcome of future observations that have not been used to construct the model. We compared mean eosinophil counts of DA-affected CSL between the dataset used in this study and previously reported results (23) using a two-tailed t-test. As those results were reported separately for acute and chronic cases, we first calculated the overall mean and standard error, considering sample size weighting.
Statistical results were reported in terms of the level of statistical support for a tested correlation or difference between models, as opposed to a binary “significant or not significant” classification based on an arbitrary P-value cutoff of 0.05. “No support” is used when P-values are high (~0.1 and above), “weak support” when P-values are close to 0.05 (~0.01–0.1), “moderate support” when P-values are small (~0.001–0.01), and “strong support” when P-values are very small (~<0.001). This reporting method emphasizes the fact that statistical significance is determined on a continuum and follows current reporting recommendations (34).
Analyses, data manipulation, and plotting were performed in R, using packages lme4, ggplot2, pROC, and dplyr (35–39).
A wide variety of tests have been evaluated to investigate and characterize the effects of DA toxicosis in CSL as alternatives to the detection of DA itself in body fluids or tissues, but few have been evaluated for sensitivity or specificity or have been applied to the clinical setting on a regular basis. Changes in circulating numbers of eosinophils, serum apolipoprotein E, and cerebrospinal fluid proteins have been detected in groups of sea lions with DA toxicosis compared to unaffected animals, and DA antibodies have been detected in sea lions with chronic toxicosis (23, 40–42). Antemortem MRI evaluation of hippocampal size (18, 43) and non-invasive diagnostic methods have also been explored. Behavioral studies in CSL affected by DA found a direct correlation between long-term spatial memory and right-sided hippocampal structure (45). Measurement of habituation rates to an auditory stimulus and the presence of persistent abnormal behaviors such as head weaving and muscle fasciculations can also help diagnose DA toxicosis (21, 46). As neurons in the olfactory bulb may also undergo DA-induced necrosis, olfactory testing has also been evaluated for the diagnosis of DA intoxication (47). A summary of these tests, their results, and potential limitations are listed by publication date in Table 1.
Table 1. Summary table of published methods to aid in the diagnosis of acute domoic acid toxicosis, chronic domoic acid toxicosis, or both.
Despite the variety of antemortem tests that have shown promising results as diagnostic tools to help characterize the effects of DA intoxication, not all have been applied clinically on a larger scale, either due to logistical reasons, test and reagent development, and/or availability, lack of resources, or low specificity. In addition to diagnostic tools, inexpensive, practical, and minimally invasive methods to aid clinicians in determining whether a CSL affected by DA is clinically fit for release are still needed.
Various clinical presentations previously described in the literature were observed in CSL with DA intoxication, including, but not limited to, head weaving, ataxia, seizures, and coma that varied in severity depending on the animal. Cardiomyopathy, blindness, and the presence of eosinophilia (diagnosed as an absolute eosinophil count >0.68 × 109/L) have also been well-documented in the literature (4, 12, 14, 23). Historically, eosinophilia was reported in both acute and chronically intoxicated CSL in 2009, with a median of 0.925 × 109/L (23). Similarly, we found this trend to be consistent in our full dataset of stranded CSL between 1998 and 2019. Data differentiating between acutely intoxicated CSL and chronically intoxicated CSL were available on 1,640 out of the 2,448 CSL within the full dataset. Of these 1,640 CSLs, eosinophil data were available on 642, where 63.91% (n = 540) had eosinophilia on admit blood work; 70.6% (n = 293) of acutely intoxicated CSL and 61.7% (n = 140) of chronically intoxicated CSL had an eosinophilia. We found a slightly higher mean eosinophil count of 1.07 × 109/L (acute and chronically intoxicated CSL combined) compared to previously published data. We found no statistical support for a difference in previously published eosinophil counts (mean = 1.01 × 109/L) and our current study (mean = 1.07 × 109/L), t-test, t = 1.471, df = 670, p-value = 0.142.
As DA-producing blooms are most common in spring and early summer, it is not uncommon for late pregnancy CSL to strand with DA intoxication (8). To date, clinical treatment has been aimed at saving the adult rather than the fetus, as fetal damage due to in utero exposure is suspected, making the offspring's prognosis poor (8, 11). The number and outcomes of all CSLs diagnosed as pregnant, blind, having eosinophilia on admission blood work, or with cardiomyopathy between 1998 and 2019 are reported in Table 2.
Table 2. Summary of California sea lions diagnosed as pregnant, blind, or with cardiomyopathy as well as diagnostics performed and the percent of sea lions that survived.
Between 1998 and 2019, TMMC rescued a total of 11, 737 CSL, 21% (n = 2, 447) of which were diagnosed with DA toxicosis (Figure 2). Strong statistical support for an increase in CSL stranding due to DA intoxication over time was found (generalized linear model with log link function and Poisson error distribution; ChiSq = 41.7, p-value < 0.001), where, on average, the number of DA-associated strandings increased by 2.1% each year, which may in part be due to an increase in the total CSL population over time (49). Of the DA-associated strandings, the proportions diagnosed with acute and chronic syndromes were 63.5% (n = 1,042) and 36.5% (n = 598), respectively, with strong statistical support for an annual increase of 7.4% of the average proportion of chronic DA stranding (ChiSq = 42.3, df = 1, p-value < 0.0001). We were unable to categorize a total of 807 cases due to the lack of use of the classification of acute or chronic DA intoxication at admission by the clinician.
Figure 2. Total number of stranded California sea lions at The Marine Mammal Center (TMMC) due to any cause (light gray) compared to the number of California sea lions stranded at TMMC due to domoic acid intoxication (black) between 1998 and 2019. In the years with elevated California sea lion strandings due to domoic acid intoxication and stranding due to any cause, the causes of stranding other than domoic acid intoxication were primarily due to leptospirosis infection (2000 and 2009) and malnutrition (2009 and 2015), with a high proportion of malnourished pups and yearlings stranding in 2009 and 2015.
Between 1998 and 2019, 44% (n = 1,074) of stranded CSL with DA intoxication (acute or chronic) were released, while 56% (n = 1,366) died or were euthanized. Of the animals that died or were euthanized, 42.4% (n = 579) died naturally, and 57.6% (n = 787) were euthanized. A total of 149 animals with DA intoxication re-stranded (6.1%) after being released, a rate that was significantly higher than the re-strand rate due to causes of stranding excluding DA intoxication during this same period (3.7%; ChiSq = 20.7, df = 1, p-value < 0.0001). This rate considers each re-strand as a separate event, even if the same animal re-strands multiple times.
Strong statistical support was found for age and sex effects (ChiSq = 3,698, df = 4, -value <0.001; ChiSq = 881, df = 1, p-value < 0.001, respectively). Most sea lions with DA toxicosis were adult females, followed by juvenile males, subadult males, subadult females, yearling females, and adult males (Table 2). The average rehabilitation times (number of days between admission and disposition date) were 24.9 days (range = 2–169) for animals that were released (excluding the animals that were placed in permanent human care), 8.3 days (range = 0–98) for those that died or were euthanized, with a combined (survived or died) average of 15.9 days for all outcomes. Demographic statistics are summarized in Table 3.
Table 3. Summary of age class and sex distribution of stranded California sea lions diagnosed with domoic acid intoxication (acute, chronic, or acute on chronic) between 1998 and 2019 at The Marine Mammal Center.
Records from a subset of 92 DA-intoxicated CSL that were stranded between July 2015 and August 2017 were reviewed to assess the predictive accuracy of the NS in determining whether a DA-affected CSL was fit for release. Other potential candidate variables that aid in determining releasability and components of the NS that were most important in determining whether a DA-intoxicated CSL was fit for release were also assessed. Table 4 lists all models tested along with their results. Of note, the best predictors of releasability were the third NS, whether the animal started eating while in rehabilitation, the difference in NS over time with a decreasing trend of NS values, and the state of nutrition at admission. Utilizing these four variables, the model correctly predicted whether a CSL was released or not 85% (AUC = 0.90) of the time. The presence of eosinophilia, co-morbidities, and straight body length had no significant correlation with the outcome of the animal and thus were not included in the models. Of the 92 animals in the subset, 46.7% died or were euthanized (n = 43), and 53.2% were released (n = 49). Nine animals that died or were euthanized had full histopathology collected on necropsy, and eight of the nine animals had brain and/or cardiac lesions consistent with domoic acid toxicity, ranging from unilateral to bilateral hippocampal atrophy, inflammation, gliosis, and/or neuronal degeneration; myocardial degeneration, necrosis, or edema. The animal that did not have lesions consistent with domoic acid toxicosis was diagnosed with meningitis (22). The remaining animals that died or were euthanized either did not have histopathology collected or did not have full histopathology collected to include the brain or heart.
Table 4. Performance of the top models predicting outcome (release vs. death) using different combinations of variables.
We also assessed which components of the NS were most important in determining fitness for release. In a situation where all combinations of the different NS subsections were possible (classification tree modeling), auditory approach response, tactile response, and mentation were the most important. When using only one subsection at a time in a logistic regression model, the subsections that correlated most strongly with outcome were auditory approach response, tactile response, and locomotion.
As the survivability of released CSL with DA intoxication is unknown and outside the scope of this study, the re-strand rate was used as an assessment of the effectiveness of the NS in aiding clinicians in determining whether an intoxicated CSL was fit for release. At TMMC, a NS of 10 for the third/final NS performed has been used since 2006 as a cutoff to differentiate between a releasable and non-releasable animal. Prior to the implementation of the NS in the release assessment (1998–2006), the average re-strand rate for DA-intoxicated CSL was 8.2%. In the time frame after the consistent use of the NS during the release assessment (2007–2019), the average re-strand rate was 6%. The differences between the re-strand rates were not statistically supported; however, the consistent implementation of the NS during the release assessment was associated with an improved average re-strand rate for released DA-intoxicated CSL.
Despite the extensive research and effort applied to further our understanding of DA intoxication in marine wildlife, humans, and laboratory animal models over the past 20 years, the clinical management of CSL and other marine mammals diagnosed with DA toxicosis remains challenging. Rehabilitation facilities have the responsibility to rehabilitate and release stranded marine mammals with a high “likelihood of survival” post-release, as per NOAA guidelines (22). We reviewed published literature to summarize diagnostic tests used to help detect the spectrum of lesions and effects associated with the acute and chronic syndromes as well as treatment strategies for CSL intoxicated with DA, presented stranding statistics of DA-affected CSL over the past 20 years, and evaluated a promising tool with candidate variables to aid rehabilitation facilities in determining whether a DA-affected CSL is fit for release (as determined by high likelihood of survival and a low likelihood of re-stranding).
Of the previously published diagnostic tests listed in Table 1, the presence of eosinophilia was the only previously published test evaluated in this study for correlation with “being released” with a low likelihood of re-stranding, and no correlation was found. Thus, the presence of eosinophilia on admission was not included in the models. To date, clinical signs, especially when observed in CSL that strand in spatial and temporal clusters, are the most useful initial guide for a clinician to determine that a CSL has DA toxicosis. Eosinophilia on blood work should increase the clinician's suspicion of DA intoxication, especially as there are multiple other diseases that mimic DA toxicosis, such as bacterial meningitis or protozoal encephalopathy. Additionally, cardiomyopathy and blindness have been well-documented and were found in 2.2 and 1.1% of DA-intoxicated CSL between 1998 and 2019, respectively. Cardiomyopathy and blindness are thought to be caused by DA binding to glutamate receptors on cardiac muscle fibers and to retinal neurons, whereas the cause of eosinophilia is unknown but is hypothesized to be related either to adrenal gland function or caused by exposure to DA itself, independent of adrenal gland function (12, 14, 23, 50). CSF proteomics have promise for aiding clinicians in diagnosing DA exposure as multiple CSF proteins were found to be elevated in DA-intoxicated CSL compared to control CSL (42), and the presence of DA antibodies shows promise as 11 of 17 CSL chronic DA cases had antibodies (30). A definitive diagnosis of hippocampal atrophy can be obtained with an MRI. Using the entire clinical picture of the animal, the state of nutrition at admission, the third and final NS, the change in NS over time, and whether the animal started eating while in rehabilitation can help guide clinicians on the decision to release an animal with DA intoxication if MRI is not possible.
To date, research has focused on the diagnosis and pathological effects of DA toxicosis in mature CSL. Over the past 20 years, 30 stranded pups were also diagnosed with DA intoxication at TMMC, and some affected pregnant females that do not strand may give birth to live neonates. The ability of DA to cross the placenta and recirculate between the fetus and amniotic fluid, as well as its presence in the milk of lactating females, present potential repercussions for the developing fetus and young, naïve pups (6, 8, 10, 11, 20, 51). Studies in non-human primates and rodents (mice and rats) exposed both in utero and in the early post-natal phase show that DA exposure causes abnormalities such as repetitive behaviors and altered spontaneous behaviors, a lower seizure threshold, and structural changes in areas of the brain including the hippocampus, cortex, and amygdala (2, 6, 12, 52–54). As such, rehabilitated neonates and pups born to dams intoxicated by DA may have a poor prognosis for survival if released or placed in permanent care. Recently, a delayed manifestation of neurological disease and acute death later in life following DA exposure during development were reported in CSLs (55). In addition to CSL, DA can affect other species in California waters and has even been found in species as far north as Alaska, posing a potentially significant risk to the recovery of threatened species such as the southern sea otter (Enhydra lutris) and Guadalupe fur seal (Arctocephalus townsendi) among others (9, 15–17, 56). Further studies are warranted to determine the long-term effects of DA exposure from both in utero and lactational exposure in CSL and other pinnipeds as DA exposure and strandings secondary to DA toxicosis become more frequent and expansive throughout the Pacific and other oceans.
The NS is a useful prognostic tool as it does not require specialized equipment, is a relatively quick assessment requiring potentially only one person, and is a cost-effective method that can objectively be performed without extensive training. At TMMC, a final NS ≤ 10 has been used since 2006 as a cutoff to differentiate between a releasable and non-releasable animal, and the consistent use of the NS has decreased the re-strand rate for DA-intoxicated CSL over time.
When discussing the re-strand rates in the period prior to and after consistent implementation of the NS, it is also important to discuss the potential differences in the management of CSL intoxicated with DA. Although the decision-making process for the management of each individual CSL is not discussed in this study, the differences in the proportion of released vs. euthanized CSL prior to and after the implementation of the NS likely reflect changes in the process. Prior to the implementation of the NS (1998–2006), the proportion of CSL with DA intoxication that were released was 62.6%, whereas after the implementation of the NS (2007–2019), the proportion of CSL with DA intoxication that were released decreased to 37.1%. Similarly, the proportion of CSLs that were euthanized prior to the implementation of the NS was 16%, which increased to 41.3% after the NS was implemented. These differences are likely a combination of an improved understanding of the disease process, differing case management styles, suspected repeat DA exposure to CSL over time, thus increasing the proportion of chronically affected CSL with a generally poorer prognosis for survival after release, and the utilization of better tools to help with release assessments.
We also assessed whether the NS could be improved based on the results from the subset of 92 CSL by adjusting the relative weights of the NS sections and re-analyzing how accurate the NS was in predicting whether an animal was fit for release. By removing the “Posture” section of the NS entirely and multiplying the “Locomotion” results by three, the NS accuracy improved a small amount from 85 to 87%, although there was no statistical support for this change. The NS has gradually been adjusted over time based on empirical observations of stranded CSL intoxicated by DA and the changing symptomatology over the years since its implementation as an assessment tool for release. However, this study was the first to statistically assess the effectiveness of the NS as a tool for release assessment as well as the optimal weightings between the components of the NS.
There were limitations in assessing the NS's accuracy to predict whether DA-intoxicated animals were fit for release, namely that the outcome variable (whether an animal was fit for release or not), was often determined by the same clinician treating the animal and performing the NS, and the decision to release an animal was partly based on the same diagnostics used to create the NS. This introduces bias into the current study, but this was unavoidable under the circumstances and does not mean the NS is not useful as it is a quantifiable measure of several variables that clinicians have been using to assess whether an animal is fit for release. Additionally, by analyzing variables outside of the NS, such as the state of nutrition at admission, whether an animal started eating while in rehabilitation, and changes in the NS final score, we aimed to help make the determination more objective for clinicians managing CSL with DA intoxication. Future clinical research could focus on determining the association of the NS with specific lesions in the CSL, such as hippocampal atrophy.
While we are continually improving our understanding of DA toxicosis in marine mammals, many challenges remain, including describing and understanding the long-term effects of in utero and neonatal exposure on the developing fetus and neonate, the pathophysiology of the effects on organ systems outside of the central nervous and cardiac systems, and the long-term effects of repeated non-lethal exposures, among others. This study illustrates the utility of detailed clinical record-keeping, and future work should focus on further refining clinical and diagnostic evaluations of naturally exposed marine mammals to clarify the pathogenesis and prognosis of DA toxicosis in these mammals.
The datasets presented in this article are not readily available because the current datasets used in this manuscript belong to the Marine Mammal Center and not the corresponding author. Requests can be made through the data request system directly to the Marine Mammal Center to obtain any data from this manuscript. Requests to access the datasets should be directed to AM, YWJieS5tY2NsYWluQG5tbWYub3Jn.
Ethical approval was not required for the study involving animals in accordance with the local legislation and institutional requirements because this study was performed using retrospective data collected during routine health care and release assessments for stranded California sea lions undergoing rehabilitation.
AM, CF, SJ, SW, and FG contributed to the conception and design of the manuscript. TN organized and collected the data. BB performed the statistical analysis. AM, CF, SJ, SW, LT-B, and FG contributed to the clinical care and management of cases as well as diagnostics. PD contributed to the histopathology and post-mortem review of cases. AM wrote the first draft of the manuscript. BB, CF, SJ, and FG wrote sections of the manuscript. All authors contributed to manuscript revision, read, and approved the submitted version.
Financial support for the writing of this study as well as partial funding for the article processing fee was provided by the Dr. Jeanette Fuller Ridgway Scientific Writing Fund.
The authors thank the vast number of people who have cared for stranded marine mammals, contributed to the understanding of the pathophysiology of domoic acid intoxication over the past 20 years, and have improved the lives of affected marine mammals. The authors particularly thank the incredible volunteers and staff at The Marine Mammal Center and other rehabilitation facilities for their tireless efforts caring for these patients, as well our many collaborators and NOAA. Lastly, the authors thank the Dr. Jeanette Fuller Ridgway Scientific Writing Fund for providing support in the writing of this study as well as support for the article processing charges. TMMC operates under NOAA permit 18786-04.
The authors declare that the research was conducted in the absence of any commercial or financial relationships that could be construed as a potential conflict of interest.
All claims expressed in this article are solely those of the authors and do not necessarily represent those of their affiliated organizations, or those of the publisher, the editors and the reviewers. Any product that may be evaluated in this article, or claim that may be made by its manufacturer, is not guaranteed or endorsed by the publisher.
1. Bates SS, Hubbard KA, Lundholm N, Montresor M, Leaw CP. Pseudo-nitzschia, Nitzschia, and domoic acid: new research since 2011. Harmful Algae. (2018) 79:3–43. doi: 10.1016/j.hal.2018.06.001
2. Petroff R, Hendrix A, Shum S, Grant KS, Lefebvre KA, Burbacher TM. Public health risks associated with chronic, low-level domoic acid exposure: a review of the evidence. Pharmacol Ther. (2021) 227:107865. doi: 10.1016/j.pharmthera.2021.107865
3. Anderson DM, Fensin E, Gobler CJ, Hoeglund AE, Hubbard KA, Kulis DM, et al. Marine harmful algal blooms (HABs) in the United States: history, current status and future trends. Harmful Algae. (2021) 102:101975. doi: 10.1016/j.hal.2021.101975
4. Goldstein T, Mazet JAK, Zabka TS, Langlois G, Colegrove KM, Silver M, et al. Novel symptomatology and changing epidemiology of domoic acid toxicosis in California sea lions (Zalophus californianus): an increasing risk to marine mammal health. Proc R Soc B Biol Sci. (2008) 275:267–76. doi: 10.1098/rspb.2007.1221
5. Gulland FMD, Haulena M, Fauquier D, Langlois G, Lander ME, Zabka T, et al. Domoic acid toxicity in Californian sea lions (Zalophus californianus): clinical signs, treatment and survival. Vet Rec. (2002) 150:475–80. doi: 10.1136/vr.150.15.475
6. Zuloaga DG, Lahvis GP, Mills B, Pearce HL, Turner J, Raber J. Fetal domoic acid exposure affects lateral amygdala neurons, diminishes social investigation and alters sensory-motor gating. Neurotoxicology. (2016) 53:132–40. doi: 10.1016/j.neuro.2016.01.007
7. Iverson F, Truelove J, Nera E, Tryphonas L, Campbell J, Lok E. Domoic acid poisoning and mussel-associated intoxication: preliminary investigations into the response of mice and rats to toxic mussel extract. Food Chem Toxicol. (1989) 27:377–84. doi: 10.1016/0278-6915(89)90143-9
8. Lefebvre KA, Hendrix A, Halaska B, Duignan P, Shum S, Isoherranen N, et al. Domoic acid in California sea lion fetal fluids indicates continuous exposure to a neuroteratogen poses risks to mammals. Harmful Algae. (2018) 79:53–7. doi: 10.1016/j.hal.2018.06.003
9. Moriarty ME, Tinker MT, Miller MA, Tomoleoni JA, Staedler MM, Fujii JA, et al. Exposure to domoic acid is an ecological driver of cardiac disease in southern sea otters?. Harmf Algae. (2021) 101:101973. doi: 10.1016/j.hal.2020.101973
10. Panlilio JM, Aluru N, Hahn ME. Developmental neurotoxicity of the harmful algal bloom toxin domoic acid: cellular and molecular mechanisms underlying altered behavior in the zebrafish model. Environ Health Perspect. (2020) 128:117002. doi: 10.1289/EHP6652
11. Shum S, Jing J, Petroff R, Crouthamel B, Grant KS, Burbacher TM, et al. Maternal-fetal disposition of domoic acid following repeated oral dosing during pregnancy in nonhuman primate. Toxicol Appl Pharmacol. (2020) 398:115027. doi: 10.1016/j.taap.2020.115027
12. Silvagni PA, Lowenstine LJ, Spraker T, Lipscomb TP, Gulland FMD. Pathology of domoic acid toxicity in California sea lions (Zalophus californianus). Vet Pathol. (2005) 42:184–91. doi: 10.1354/vp.42-2-184
13. Thomas K. Movement, dive behavior, and survival of California sea lions (Zalophus californianus) post-treatment for domoic acid toxicosis. Capstone Projects and Theses. (2008). p. 76. doi: 10.1111/j.1748-7692.2009.00314.x
14. Zabka TS, Goldstein T, Cross C, Mueller RW, Kreuder-Johnson C, Gill S, et al. Characterization of a degenerative cardiomyopathy associated with domoic acid toxicity in california sea lions (Zalophus californianus). Vet Pathol. (2009) 46:105–19. doi: 10.1354/vp.46-1-105
15. Scholin CA, Gulland F, Doucette GJ, Benson S, Busman M, Chavez FP, et al. Mortality of sea lions along the central California coast linked to a toxic diatom bloom. Nature. (2000) 403:80–4. doi: 10.1038/47481
16. Lefebvre KA, Robertson A, Frame ER, Colegrove KM, Nance S, Baugh KA, et al. Clinical signs and histopathology associated with domoic acid poisoning in northern fur seals (Callorhinus ursinus) and comparison of toxin detection methods. Harmful Algae. (2010) 9:374–83. doi: 10.1016/j.hal.2010.01.007
17. Miller MA, Moriarty ME, Duignan PJ, Zabka TS, Dodd E, Batac FI, et al. Clinical signs and pathology associated with domoic acid toxicosis in southern sea otters (Enhydra lutris nereis). Front Mar Sci. (2021) 8:585501. doi: 10.3389/fmars.2021.585501
18. Buckmaster PS, Wen X, Toyoda I, Gulland FMD, Van Bonn W. Hippocampal neuropathology of domoic acid-induced epilepsy in California sea lions (Zalophus californianus). J Comp Neurol. (2014) 522:1691–706. doi: 10.1002/cne.23509
19. McCabe RM, Hickey BM, Kudela RM, Lefebvre KA, Adams NG, Bill BD, et al. An unprecedented coastwide toxic algal bloom linked to anomalous ocean conditions. Geophys Res Lett. (2016) 43:10366–76. doi: 10.1002/2016GL070023
20. Brodie EC, Gulland FMD, Greig DJ, Hunter M, Jaakola J, Leger JS, et al. Domoic acid causes reproductive failure in California sea lions (Zalophus californianus). Mar Mamm Sci. (2006) 22:700–7. doi: 10.1111/j.1748-7692.2006.00045.x
21. Wittmaack C, Lahvis GP, Keith EO, Self-Sullivan C. Diagnosing domoic acid toxicosis in the California sea lion (Zalophus californianus) using behavioral criteria: a novel approach. Zoo Biol. (2015) 34:314–20. doi: 10.1002/zoo.21217
22. Whaley JE. Final Policies and Best Practices for Marine Mammal Response, Rehabilitation, and Release Standards for Release. NOAA (2009).
23. Gulland FM, Hall AJ, Greig DJ, Frame ER, Colegrove KM, Booth RK, et al. Evaluation of circulating eosinophil count and adrenal gland function in California sea lions naturally exposed t. J Am Vet Med Assoc. (2009) 241:943–9. doi: 10.2460/javma.241.7.943
24. Pulido OM. Domoic acid toxicologic pathology: a review. Mar Drugs. (2008) 6:180–219. doi: 10.3390/md6020180
25. Field CL, Whoriskey ST, Xianguo Z, Papich MG. Pharmacokinetics of subcutaneous alpha lipoic acid, a proposed therapeutic aid for domoic acid intoxication in California sea lions (Zalophus californianus). J Zoo Wildlife Med. (2021) 52:872–9. doi: 10.1638/2020-0223
26. Grant KS, Crouthamel B, Kenney C, McKain N, Petroff R, Shum S, et al. Preclinical modeling of exposure to a global marine bio-contaminant: effects of in utero domoic acid exposure on neonatal behavior and infant memory. Neurotoxicol Teratol. (2019) 73:1–8. doi: 10.1016/j.ntt.2019.01.003
27. Greig DJ, Gulland FMD, Kreuder C. A decade of live California sea lion (Zalophus californianus) strandings along the central california coast: causes and trends, 1991-2000. Aquat Mamm. (2005) 31:11–22. doi: 10.1578/AM.31.1.2005.11
28. Whitmer ER, Borremans B, Duignan PJ, Johnson SP, Lloyd-Smith JO, McClain AM, et al. Classification and regression tree analysis for predicting prognosis in wildlife rehabilitation: a case study of leptospirosis in california sea lions (Zalophus californianus). J Zoo Wildl Med. (2021) 52:38–48. doi: 10.1638/2020-0111
29. Cook PF, Hoard VA, Dolui S, deB Frederick B, Redfern R, Dennison SE, et al. An MRI protocol for anatomical and functional evaluation of the California sea lion brain. J Neurosci Methods. (2021) 353:109097. doi: 10.1016/j.jneumeth.2021.109097
30. Lawrence J, Alcock D, McGrath P, Kay J, MacMurray S, Dulberg C. The development of a tool to assess neonatal pain. Neonat Netw. (1993) 12:59–66.
31. Stevens B, Johnston C, Petryshen P, Taddio A. Premature infanct pain profile: development and initial validation. Clin J Pain. (1996) 12:13–22. doi: 10.1097/00002508-199603000-00004
32. Fawcett T. An introduction to ROC analysis. Pattern Recognit Lett. (2006) 27:861–74. doi: 10.1016/j.patrec.2005.10.010
33. Hastie T, Tibshirani R, Friedman J. The Elements of Statistical Learning: Data Mining, Inference, and Prediction. Springer.
34. Muff S, Nilsen EB, O'Hara RB, Nater CR. Rewriting results sections in the language of evidence. Trends Ecol Evol. (2022) 37:203–10. doi: 10.1016/j.tree.2021.10.009
35. Bates D, Maechler M, Bolker B, Walker S. Fitting linear mixed-effects models using lme4. J Stat Softw. (2015) 67:1–48. doi: 10.18637/jss.v067.i01
36. R Core Team. R: A Language and Environment for Statistical Computing. (2019). Available online at: https://www.r-project.org/ (accessed June 15, 2023).
37. Robin X, Turck N, Hainard A, Tiberti N, Lisacek F, Sanchez J-C, Mueller M. pROC: an open-source package for R and S+ to analyze and compare ROC curves. BMC Bioinformat. (2011) 12, 77. doi: 10.1186/1471-2105-12-77
39. Wickham H, François R, Henry L, Müller K. dplyr: A Grammar of Data Manipulation. (2021). Available online at: https://cran.r-project.org/package=dplyr (accessed June 15, 2023).
40. Lefebvre KA, Frame ER, Gulland F, Hansen JD, Kendrick PS, Beyer RP, et al. A novel antibody-based biomarker for chronic algal toxin exposure and sub-acute neurotoxicity. PLoS ONE. (2012) 7:1–7. doi: 10.1371/journal.pone.0036213
41. Neely BA, Soper JL, Greig DJ, Carlin KP, Favre EG, Gulland FMD, et al. Serum profiling by MALDI-TOF mass spectrometry as a diagnostic tool for domoic acid toxicosis in California sea lions. Proteome Sci. (2012) 10:1–12. doi: 10.1186/1477-5956-10-18
42. Neely BA, Soper JL, Gulland FMD, Bell PD, Kindy M, Arthur JM, et al. Proteomic analysis of cerebrospinal fluid in California sea lions (Zalophus californianus) with domoic acid toxicosis identifies proteins associated with neurodegeneration. Proteomics. (2015) 15:4051–63. doi: 10.1002/pmic.201500167
43. Montie EW, Wheeler E, Pussini N, Battey TWK, Barakos J, Dennison S, et al. Magnetic resonance imaging quality and volumes of brain structures from live and postmortem imaging of California sea lions with clinical signs of domoic acid toxicosis. Dis Aquat Organ. (2010) 91:243–56. doi: 10.3354/dao02259
44. Neely BA, Ferrante JA, Chaves JM, Soper JL, Almeida JS, et al. Proteomic analysis of plasma from California sea lions (Zalophus californianus) reveals apolipoprotein E as a candidate biomarker of chronic domoic acid toxicosis. PLoS ONE. (2015) 15:e0123295. doi: 10.1371/journal.pone.0123295
45. Cook PF, Reichmuth C, Rouse AA, Libby LA, Dennison SE, Carmichael OT, et al. Algal toxin impairs sea lion memory and hippocampal connectivity, with implications for strandings. Science. (2015) 350:1545–7. doi: 10.1126/science.aac5675
46. Cook P, Reichmuth C, Gulland F. Rapid behavioural diagnosis of domoic acid toxicosis in California sea lions. Biol Lett. (2011) 7:536–8. doi: 10.1098/rsbl.2011.0127
47. de Maio LM, Cook PF, Reichmuth C, Gulland FMD. The evaluation of olfaction in stranded California sea lions (Zalophus californianus) and its relevance to domoic acid toxicosis. Aquat Mamm. (2018) 44:231–8. doi: 10.1578/AM.44.3.2018.231
48. Barbosa L, Boor M, Greene R, Colegrove K, Johnson SP, Gulland F. Echocardiographic findings in domoic acid exposed California sea lions. In: IAAAM Conference Proceedings (2015).
49. Laake JL, Lowry MS, DeLong RL, Melin SR, Carretta JV. Population growth and status of california sea lions. J Wildlife Manag. (2018) 82:583–95. doi: 10.1002/jwmg.21405
50. Gill S, Goldstein T, Situ D, Zabka TS, Gulland FMD, Mueller RW. Cloning and characterization of glutamate receptors in Californian sea lions (Zalophus californianus). Mar Drugs. (2010) 8:1637–49. doi: 10.3390/md8051637
51. Rust L, Gulland F, Frame E, Lefebvre K. Domoic acid in milk of free living California marine mammals indicates lactational exposure occurs. Mar Mamm Sci. (2014) 30:1272–8. doi: 10.1111/mms.12117
52. Costa LG, Giordano G, Faustman EM. Domoic acid as a developmental neurotoxin. Neurotoxicology. (2010) 31:409–23. doi: 10.1016/j.neuro.2010.05.003
53. Jandová K, Kozler P, Langmeier M, Marešová D, Pokorn? J, Riljak V. Influence of low-dose neonatal domoic acid on the spontaneous behavior of rats in early adulthood. Physiol Res. (2014) 63:S521–8. doi: 10.33549/physiolres.932936
54. Krucik DDR, Cook P, Cathey M, Meegan JM, Gomez FM, Van Bonn W, Le-Bert C. Adult-onset epilepsy and hippocampal pathology in a California sea lion (Zalophus californianus): a case study of suspected in utero exposure to domoic acid. NeuroToxicol. (2023) 96:18. doi: 10.1016/j.neuro.2023.02.010
55. Simeone C, Fauquier D, Skidmore J, Cook P, Colegrove K, Gulland F, et al. Clinical signs and mortality of non-released stranded California sea lions housed in display facilities: the suspected role of prior exposure to algal toxins. Vet Rec. (2019) 185:304. doi: 10.1136/vr.105371
Keywords: domoic acid, sea lion, Zalophus californianus, toxicosis, prognosis, symptom
Citation: McClain AM, Field CL, Norris TA, Borremans B, Duignan PJ, Johnson SP, Whoriskey ST, Thompson-Barbosa L and Gulland FMD (2023) The symptomatology and diagnosis of domoic acid toxicosis in stranded California sea lions (Zalophus californianus): a review and evaluation of 20 years of cases to guide prognosis. Front. Vet. Sci. 10:1245864. doi: 10.3389/fvets.2023.1245864
Received: 26 June 2023; Accepted: 04 September 2023;
Published: 02 October 2023.
Edited by:
Peter Cook, New College of Florida, United StatesReviewed by:
Wendi D. Roe, Massey University, New ZealandCopyright © 2023 McClain, Field, Norris, Borremans, Duignan, Johnson, Whoriskey, Thompson-Barbosa and Gulland. This is an open-access article distributed under the terms of the Creative Commons Attribution License (CC BY). The use, distribution or reproduction in other forums is permitted, provided the original author(s) and the copyright owner(s) are credited and that the original publication in this journal is cited, in accordance with accepted academic practice. No use, distribution or reproduction is permitted which does not comply with these terms.
*Correspondence: Abby M. McClain, YWJieS5tY2NsYWluQG5tbWYub3Jn
†Present addresses: Abby M. McClain, National Marine Mammal Foundation, San Diego, CA, United States
Benny Borremans, Wildlife Health Ecology Research Organization, San Diego, CA, United States
Frances M. D. Gulland, One Health Institute, University of California, Davis, Davis, CA, United States
Lorraine Thompson-Barbosa, Oiled Wildlife Care Network, University of California, Davis, Davis, CA, United States
Shawn P. Johnson, Sea Change Health, Sunnyvale, CA, United States
Disclaimer: All claims expressed in this article are solely those of the authors and do not necessarily represent those of their affiliated organizations, or those of the publisher, the editors and the reviewers. Any product that may be evaluated in this article or claim that may be made by its manufacturer is not guaranteed or endorsed by the publisher.
Research integrity at Frontiers
Learn more about the work of our research integrity team to safeguard the quality of each article we publish.