- 1Wisconsin Veterinary Diagnostic Laboratory, University of Wisconsin-Madison, Madison, WI, United States
- 2Department of Medical Sciences, School of Veterinary Medicine, University of Wisconsin-Madison, Madison, WI, United States
- 3Department of Statistics, University of Wisconsin-Madison, Madison, WI, United States
- 4Land O'Lakes, Cottage Grove, WI, United States
Many dairy operations uses a Brix refractometer to assess the quality of first-milking colostrum. This study investigated whether a digital Brix refractometer could be used in a model to predict colostrum IgG concentration and whether more than one %Brix threshold could be used for different colostrum IgG concentrations. Colostrum from 182 animals was tested using a digital Brix refractometer and by single radial immunodiffusion. Statistical analysis, using simple linear regression to relate %Brix results with corresponding colostral IgG concentration, and receiver operating characteristic (ROC) analysis were used to identify %Brix cutoffs that had no false positive results. Colostral IgG concentrations from digital Brix refractometry had a R2 value of 0.818 and a S-value of 21.7 g/L. The large S-value shows that a digital Brix refractometer should not be used in a model to predict colostrum IgG concentration. However, %Brix scores of 19.0, 22.0, 25.0 and 30.0 percent can be used to estimate minimum colostral IgG concentrations of 25, 50, 75 and 100 g/L. These four cutoffs can be used to strategically feed smaller volumes of colostrum to newborn calves. Smaller volumes may reduce unwanted side effects and shorten the time interval in which calves refuse to nurse, while still delivering an adequate mass of IgG to have successful transfer of passive immunity.
1. Introduction
Newborn calves are born agammaglobulinemic and are dependent on the successful transfer of passive immunity (TPI) for optimal health and wellbeing (1, 2). Current best practices recommend that calves be fed 150–200 g of bovine colostral immunoglobulin G (IgG) within 2 h of birth, followed by an additional feeding of 75–100 g of IgG, 6–12 h after the first feeding (3). Larger calves (≥35 kg) are typically fed 300 g of IgG, and smaller calves are fed 225 g of IgG (3, 4). Dairy operations testing first-milking colostrum with a Brix refractometer often use a single threshold of ≥22% to ensure that the concentration of IgG is at least 50 g/L (3, 5, 6). The 50 g/L threshold has become the gold standard for first-milking colostrum (2–4). Feeding 4 L of first-milking colostrum within 2 h of birth, followed by an additional 2 L fed 6–12 h later, is a common practice in the industry. Very few calves will voluntarily drink 6 L of colostrum, which necessitates the use of an esophageal tube feeder for delivery (5, 6).
Feeding colostrum using an esophageal tube feeder to a newborn calf is not risk-free. One complication is aspiration pneumonia, which is caused by improper technique or excessive calf movement when the colostrum is delivered. Abdominal distension, colic, and occasionally death are other potential complications of feeding large volumes of colostrum in the first 24 h of life. As such, some dairy calf-care providers and practicing veterinarians have inquired about the possibility of implementing site-specific colostrum management programs. The calf-care providers and veterinarians wish to feed smaller volumes of first milking colostrum but still deliver a sufficient mass of colostral IgG to have excellent TPI. It is hypothesized that feeding smaller quantities of colostrum may improve abomasal health, reducing the likelihood of abdominal distension and colic post-colostrum feeding. The aim of this study was to compare %Brix results to the IgG concentrations of bovine colostrum at first milking and determine four different %Brix thresholds for 25, 50, 75, and 100 g/L of bovine colostral IgG.
2. Materials and methods
2.1. Colostrum sample collection and testing
Four Holstein dairy farms located in the Midwestern region of the United States submitted first-milking colostrum samples for testing and analysis at an accredited American Association of Veterinary Laboratory Diagnosticians (AAVLD) laboratory, the Wisconsin Veterinary Diagnostic Laboratory (WVDL). Power calculations based on the correlation between the Brix score and IgG concentration using the Power procedure in SAS (SAS 9.4, SAS Institute Inc., Cary, NC, USA) were used to calculate the sample size needed for each of the five different sampling strata. With an alpha of 0.05 and a power of 0.80, the projected minimum sample size for each of the five strata was 7 when the correlation was 0.65 or higher. Sampling kits were sent to each farm with instructions on how many samples should be collected from each of the five different sampling strata (Table 1). One hundred and eighty-two first-milking colostrum samples were collected from multi- and primiparous Holstein dairy cows. Colostrum samples were tested immediately after harvesting on-farm with a temperature-compensating digital Brix refractometer (Misco, Solon, Ohio) to obtain a %Brix reading. The refractometer was calibrated using distilled water at least once a week. If the samples met the predetermined sampling criteria, the %Brix result was recorded, and ~25–35 ml of colostrum were collected into 50 ml conical centrifuge tubes as a combined quarter sample and frozen immediately after collection at −20°C until shipped to the WVDL for testing and analysis. Samples were shipped with a sufficient number of cold packs to ensure the samples remained frozen. After receipt, each colostrum sample was assigned a unique bar-coded identification number and immediately frozen at −80°C.
Frozen (−80°C) colostrum samples were thawed in a bead bath. Testing commenced when the colostrum samples reached a temperature of 38.5°C to mimic the average normal bovine body temperature. The samples were thoroughly vortexed and tested in duplicate using a digital Brix refractometer, the same instrument model used in the on-farm collections. The Brix refractometer was calibrated by laboratory personnel using deionized water before testing commenced and repeated after 10 colostrum samples were tested. The samples were tested for IgG concentration using a commercially available single radial immunodiffusion (SRID) test (#72841, Triple-J Farms, Bellingham, WA) using the same methodology as previously described (7). Each SRID plate was set up with two internally developed bovine serum controls (high- and low-IgG concentration) in addition to the three standard solutions provided by the manufacturer. The samples were tested in duplicate. SRID agar plates were placed in a moist chamber and incubated at 23.5°C for 24 h. After incubation, the precipitin ring diameter for each sample, including the controls, was measured using a digital electronic caliper with a resolution of 0.01 mm. Samples with a coefficient of variation (CV) >5% were retested. Any colostrum sample in which the farm %Brix result differed by more than 0.5 %Brix from the WVDL was retested a second time to verify the results.
2.2. Statistical analysis
The %Brix results were compared with their matching SRID results using simple linear regression (GraphPad Prism ver 9.5, La Jolla, California). The %Brix was the independent variable, and the colostral IgG concentration, measured by SRID, was the dependent variable. The regression model was used to predict colostral IgG concentration from each of the 182 %Brix results. As previously described, the model was also used to calculate residual values for each of the 182 colostrum samples (8). All SRID results with a residual IgG value ± 10 g/L or higher were retested in duplicate. The coefficient of determination (R2) and standard error of the regression (S) were calculated, and a residual plot was constructed. Receiver operating characteristic (ROC) analysis using the ROCR package (R Foundation for Statistical Computing, ver. 4.04, Vienna, Austria) was used to calculate the cutoffs for colostral IgG concentrations of 25, 50, 75, and 100 g/L such that there were no false positive (FP) results for each %Brix value and the corresponding IgG result. An FP was defined as a %Brix value that caused the model to overestimate the true colostral IgG concentration.
3. Results
3.1. %Brix and colostrum SRID analysis
The linear regression model was estimated to be y = 8.40·X −110, where X was the %Brix score, and Y was the colostral IgG concentration. The R2 value for comparing %Brix and the corresponding colostral IgG concentration was 0.818 with an S-value of 21.7 g/L (Figure 1A). A graphical depiction of the residual plot (Figure 1B) shows the difference between the model's predicted IgG concentration (horizontal line) based on the %Brix score and the colostral IgG concentration when measured by SRID. The Brix cutoffs for estimating colostral IgG concentrations of 25, 50, 75, and 100 g/L were determined to be 18.7, 21.4, 24.7, and 30.1%, respectively. We propose using rounded values of 19.0, 22.0, 25.0, and 30.0 for simplicity (Table 2).
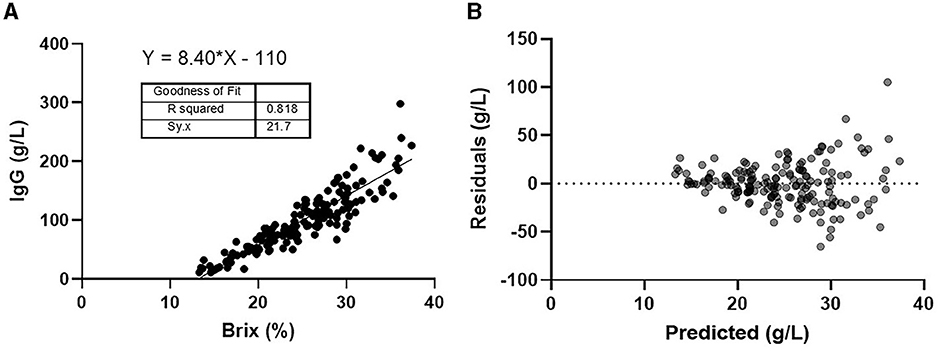
Figure 1. (A) Linear regression of Brix (%) vs. IgG g/L. (B) Residual plot of predicted colostral IgG concentration vs. actual concentration.
4. Discussion
This study shows that digital Brix refractometry cannot accurately estimate first-milking colostrum IgG concentration. However, it can be used to estimate the minimum amount of IgG for predetermined %Brix cutoffs of first-milking colostrum concentrations of 25, 50, 75, and 100 g/L. There is high confidence in the feasibility of the proposed %Brix cutoffs for on-farm use because the digital Brix refractometer has high precision and produces very reproducible results in the field (9–12). An examination of Figures 1A, B shows that as %Brix scores increase, the digital refractometer does not measure as well, particularly when the Brix scores are 30% or higher. This observation is likely caused by increasing concentrations of fat, casein, and other macromolecules that are insoluble in water, thus impacting the Brix refractometer reading (13).
A stratified sampling method was used to collect colostrum samples following screening with a digital Brix refractometer (14). This design differs from previous reports that collected colostrum samples without prior Brix refractometer screening (9–12). Stratified sampling has the advantage of ensuring a greater high to low range of %Brix scores naturally occurring in dairy herds, reducing testing costs. There were also stringent laboratory quality control measures employed that were not mentioned in previous reports (9–12). Bovine colostrum has a higher viscosity than milk (6), which makes accurate pipetting of small volumes especially difficult when the samples are cold/chilled to below body temperatures. This is the reason colostrum samples were thawed and warmed to 38.5°C (normal body temperature of cattle) and thoroughly mixed prior to pipetting. The commercial SRID test specifies that 5 μL of sample be pipetted into each well of an agar plate. Calibrated pipettes that are checked and verified semi-annually were used in the study because inaccurate pipetting of small volumes of analyte can greatly affect the accuracy of test results (15).
The R2 value of 0.818 suggests a strong relationship between %Brix and colostral IgG concentration. However, many reports have not calculated S-values or constructed a residual plot (9–12). When critically examined, this additional analysis causes a distinctly different picture to emerge for the usefulness of the %Brix score in predicting colostral IgG concentration. The S-value is an estimate of the average distance that the true measured colostrum IgG concentration falls from the concentration predicted by the model (8). For our model, S = 21.7 g/L. Thus, an approximate 95% prediction interval for a single new colostrum sample is ±1.96*S, for an estimate ±42.5 g/L. For example, if a %Brix value of 22% is used, the linear model predicts a colostral IgG concentration of 74.8 g/L, but the true value is only suspected to lie somewhere between 32.3 and 117.3 g/L with 95% confidence. This problem is illustrated graphically with the residual plot in Figure 1B. A prediction accuracy of ±42.5 g/L leads to the conclusion that model prediction of colostral IgG concentration based on %Brix score does not provide sufficient accuracy to be useful in predicting actual IgG concentration.
Several studies have shown both the short-term and long-term benefits of having adequate TPI in calves (16–19). It is well-established that calf health and wellbeing, future milk production, and longevity in the herd are highly correlated with successful TPI. For these reasons, using a model that often overestimates bovine IgG concentration based on %Brix scores when a fixed volume of colostrum is fed to newborn calves is unacceptable. The thresholds for the colostral IgG concentration of 25, 50, 75, and 100 g/L do not provide an estimate of the IgG concentration in the colostrum; it only informs the operator of the minimum amount of IgG that is likely to be present for each of the four different cutoffs. For example, a %Brix score of 30.0 only indicates that it is very likely that there is at least 100 g/L of bovine IgG in the colostrum sample. The Brix refractometer should only be used to estimate the minimum amount of IgG in a colostrum sample for the four different cutoffs and not be used in a model to predict individual colostral IgG concentrations.
This study was requested by some dairy calf-care providers and veterinarians due to their unhappiness with current first-milking colostrum feeding guidelines. On-farm observations by calf-care providers of calves with abdominal distension causing discomfort and/or colic, when coupled with the fact that newborn calves often refuse to nurse for an extended period of time, post-colostrum feeding, has caused concerns for calf health and wellbeing. A previous study has shown that first-milking-colostrum feedings can form a large curd that may be retained in the abomasum for more than 8 h after ingestion (20). It has also been reported that the volume of colostrum fed to newborn calves had less influence on the efficiency of immunoglobulin absorption than did the colostral IgG concentration itself (21). Calves fed 1 L of high-quality colostrum had more efficient immunoglobulin absorption than calves fed the same mass of immunoglobulin in 2 L of colostrum (21). Based on these observations and the results of this study, calf-care providers and veterinarians should consider feeding newborn calves a smaller volume of first milking colostrum (2–3 L) that contains at least 75–100 g/L of IgG. The first feeding should be followed by a second feeding of 1.0–1.5 liters of colostrum that contains at least 75–100 g/L of IgG. Colostrum that contains <75–100 g/L of bovine IgG can be fortified with a high-quality colostrum replacement product (22, 23). The concept is to feed a smaller but more concentrated volume of colostrum to newborn calves that delivers an adequate mass of immunoglobulins to have successful TPI. Feeding a smaller colostrum volume will reduce the number of newborn calves that refuse to nurse for at least 18–36 h post-colostrum feeding. Smaller colostrum volumes may also reduce the incidence of aspiration pneumonia, improve forestomach and abomasal health, and perhaps eliminate colic caused by over-distension of the abomasum with the formation of a large colostrum curd (20). Finally, it will increase the farm supply of colostrum that can be used to fortify newborn calf liquid feed diets for the first 2–14 days of life. Daily feeding of small volumes of first-milking colostrum for the first 2–14 days of a calf's life has improved gut health, increased average daily gain, and reduced neonatal calf diarrhea and pneumonia (24, 25).
5. Conclusion
This study shows that models that use digital Brix refractometry cannot accurately predict first-milking colostrum IgG concentration. Knowing this, calf-care providers and veterinarians should be aware that they should only use %Brix predetermined cutoffs when managing colostrum feeding programs. First-milking colostrum with higher %Brix scores (≥25%) when used to feed smaller volumes of colostrum to newborn calves is an intriguing concept that warrants further study.
Data availability statement
The original contributions presented in the study are included in the article/supplementary material, further inquiries can be directed to the corresponding author.
Ethics statement
Ethical review and approval was not required for the study on animals in accordance with the local legislation and institutional requirements.
Author contributions
DS: study design, data analysis, testing, writing—original draft, and editing. RB: writing—review, editing, and assistance with document submission. LS: laboratory testing supervision, writing—review, and editing. NK: statistical and data analysis, writing—review, and editing. TE: study design, writing—review, and editing. All authors contributed to the article and approved the submitted version.
Funding
The Wisconsin Veterinary Diagnostic Laboratory provided intramural funds for the study. The intramural funds paid for supplies, testing costs, publication fees, and the use of a statistical consultant (NK).
Conflict of interest
TE was employed by the company Land O' Lakes.
The remaining authors declare that the research was conducted in the absence of any commercial or financial relationships that could be construed as a potential conflict of interest.
Publisher's note
All claims expressed in this article are solely those of the authors and do not necessarily represent those of their affiliated organizations, or those of the publisher, the editors and the reviewers. Any product that may be evaluated in this article, or claim that may be made by its manufacturer, is not guaranteed or endorsed by the publisher.
References
1. Lombard J, Urie N, Garry F, Godden S, Quigley J, Earleywine T, et al. Consensus recommendations on calf- and herd-level passive immunity in dairy calves in the United States. J Dairy Sci. (2020) 103:7611–24. doi: 10.3168/jds.2019-17955
2. Uyama T, Kelton DF, Winder CB, Dunn J, Goetz HM, LeBlanc SJ, et al. Colostrum management practices that improve the transfer of passive immunity in neonatal dairy calves: a scoping review. PLoS ONE. (2022) 17:e0269824. doi: 10.1371/journal.pone.0269824
3. McGuirk SM, Collins M. Managing the production, storage, and delivery of colostrum. Vet Clin North Am Food Anim Pract. (2004) 20:593–603. doi: 10.1016/j.cvfa.2004.06.005
4. Ahmann J, Steinhoff-Wagner J, Buscher W. Determining immunoglobulin content of bovine colostrum and factors affecting the outcome: a review. Animals. (2021) 11:12. doi: 10.3390/ani11123587
5. Urday K M, Chigerwe M, Tyler JW. Voluntary colostrum intake in holstein heifer calves. Bovine Pract. (2008) 42:198–200. doi: 10.21423/bovine-vol42no2p198-200
6. Godden SM, Lombard JE, Woolums AR. Colostrum management for dairy calves. Vet Clin North Am Food Anim Pract. (2019) 35:535–56. doi: 10.1016/j.cvfa.2019.07.005
7. Gelsinger SL, Smith AM, Jones CM, Heinrichs AJ. Technical note: Comparison of radial immunodiffusion and ELISA for quantification of bovine immunoglobulin G in colostrum and plasma. J Dairy Sci. (2015) 98:4084–9. doi: 10.3168/jds.2014-8491
8. Weiss RE, Lazaro CG. Residual plots for repeated measures. Stat Med. (1992) 11:115–24. doi: 10.1002/sim.4780110110
9. Bielmann V, Gillan J, Perkins NR, Skidmore AL, Godden S, Leslie KE. An evaluation of Brix refractometry instruments for measurement of colostrum quality in dairy cattle. J Dairy Sci. (2010) 93:3713–21. doi: 10.3168/jds.2009-2943
10. Buczinski S, Vandeweerd JM. Diagnostic accuracy of refractometry for assessing bovine colostrum quality: a systematic review and meta-analysis. J Dairy Sci. (2016) 99:7381–94. doi: 10.3168/jds.2016-10955
11. Quigley JD, Lago A, Chapman C, Erickson P, Polo J. Evaluation of the Brix refractometer to estimate immunoglobulin G concentration in bovine colostrum. J Dairy Sci. (2013) 96:1148–55. doi: 10.3168/jds.2012-5823
12. Elsohaby I, McClure JT, Cameron M, Heider LC, Keefe GP. Rapid assessment of bovine colostrum quality: how reliable are transmission infrared spectroscopy and digital and optical refractometers? J Dairy Sci. (2017) 100:1427–35. doi: 10.3168/jds.2016-11824
13. Schalich KM, Selvaraj V. Contradictions on colostrum IgG levels and Brix values are real and can be explained. Response to letter by Lombard et al. (2022). J Ani Sci. (2022) 100:skac120. doi: 10.1093/jas/skac120
14. Kernan WN, Viscoli CM, Makuch RW, Brass LM, Horwitz RI. Stratified randomization for clinical trials. J Clin Epidemiol. (1999) 52:19–26. doi: 10.1016/S0895-4356(98)00138-3
15. Sturgeon CM, Viljoen A. Analytical error and interference in immunoassay: minimizing risk. Ann Clin Biochem. (2011) 48(Pt 5):418–32. doi: 10.1258/acb.2011.011073
16. Abuelo A, Cullens F, Hanes A, Brester JL. Impact of 2 versus 1 colostrum meals on failure of transfer of passive immunity, pre-weaning morbidity and mortality, and performance of dairy calves in a large dairy herd. Animals. (2021) 11:3. doi: 10.3390/ani11030782
17. Besser TE, Gay CC. The importance of colostrum to the health of the neonatal calf. Vet Clin North Am Food Anim Pract. (1994) 10:107–17. doi: 10.1016/S0749-0720(15)30591-0
18. Hammon HM, Liermann W, Frieten D, Koch C. Review: Importance of colostrum supply and milk feeding intensity on gastrointestinal and systemic development in calves. Animal. (2020) 14:s133–s43. doi: 10.1017/S1751731119003148
19. Kindlein L, Moretti DB, Pauletti P, Bagaldo AR, Rodrigues APO, Machado-Neto R. Bovine colostrum enriched with lyophilized bovine colostrum stimulates intestinal epithelium renewal of Holstein calves in the first days of life. J Anim Physiol Anim Nutr. (2018) 102:514–24. doi: 10.1111/jpn.12780
20. Miyazaki T, Okada K, Miyazaki M. Short communication: Neonatal calves coagulate first-milking colostrum and produce a large curd for efficient absorption of immunoglobulins after first ingestion. J Dairy Sci. (2017) 100:7262–70. doi: 10.3168/jds.2017-12808
21. Stott GH, Fellah A. Colostral immunoglobulin absorption linearly related to concentration for calves. J Dairy Sci. (1983) 66:1319–28. doi: 10.3168/jds.S0022-0302(83)81941-9
22. Halleran J, Sylvester HJ, Foster DM. Short communication: apparent efficiency of colostral immunoglobulin G absorption in Holstein heifers. J Dairy Sci. (2017) 100:3282–6. doi: 10.3168/jds.2016-11904
23. Osaka I, Matsui Y, Terada F. Effect of the mass of immunoglobulin (Ig)G intake and age at first colostrum feeding on serum IgG concentration in Holstein calves. J Dairy Sci. (2014) 97:6608–12. doi: 10.3168/jds.2013-7571
24. Kargar S, Roshan M, Ghoreishi SM, Akhlaghi A, Kanani M, Abedi Shams-Abadi AR, et al. Extended colostrum feeding for 2 weeks improves growth performance and reduces the susceptibility to diarrhea and pneumonia in neonatal Holstein dairy calves. J Dairy Sci. (2020) 103:8130–42. doi: 10.3168/jds.2020-18355
Keywords: colostrum, brix refractometry, immunoglobulin G, transfer of passive immunity, radial immunodiffusion
Citation: Sockett D, Breuer RM, Smith LW, Keuler NS and Earleywine T (2023) Investigation of brix refractometry for estimating bovine colostrum immunoglobulin G concentration. Front. Vet. Sci. 10:1240227. doi: 10.3389/fvets.2023.1240227
Received: 10 July 2023; Accepted: 29 August 2023;
Published: 25 September 2023.
Edited by:
Flaviana Gottardo, University of Padua, ItalyReviewed by:
Manuel Chamorro, Auburn University, United StatesDerek Foster, North Carolina State University, United States
Copyright © 2023 Sockett, Breuer, Smith, Keuler and Earleywine. This is an open-access article distributed under the terms of the Creative Commons Attribution License (CC BY). The use, distribution or reproduction in other forums is permitted, provided the original author(s) and the copyright owner(s) are credited and that the original publication in this journal is cited, in accordance with accepted academic practice. No use, distribution or reproduction is permitted which does not comply with these terms.
*Correspondence: Donald Sockett, Donald.Sockett@WVDL.wisc.edu