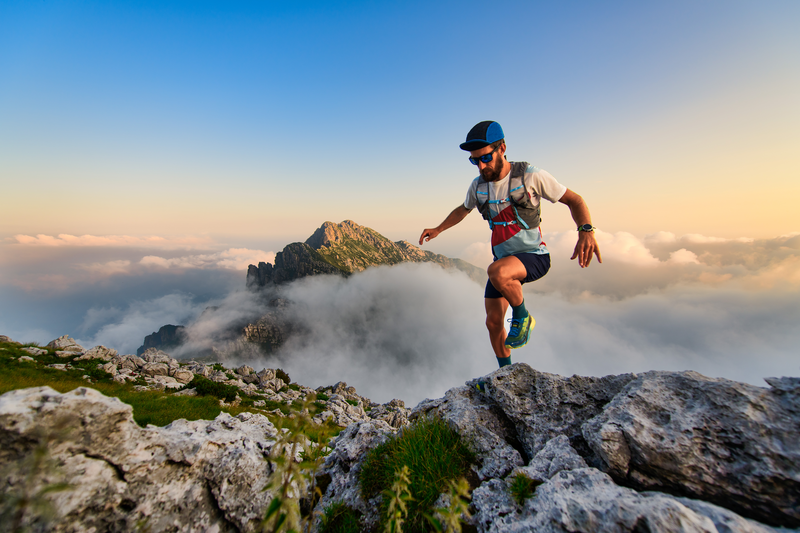
95% of researchers rate our articles as excellent or good
Learn more about the work of our research integrity team to safeguard the quality of each article we publish.
Find out more
BRIEF RESEARCH REPORT article
Front. Vet. Sci. , 22 September 2023
Sec. Animal Behavior and Welfare
Volume 10 - 2023 | https://doi.org/10.3389/fvets.2023.1228155
This article is part of the Research Topic Animal Health and Production: Identifying Challenges and Finding a Way Forward View all 40 articles
In the context of global warming, heat stress has become one of the major stress factors limiting dairy cattle production. Although many methods have been explored to help cows mitigate the negative effects of heat stress during the hot summer months, maintaining the performance of high-yielding cows under heat stress is still a great challenge. The aim of this trial was to investigate the effect of RP-GABA in the diet on milk yield, milk composition and serum biochemical parameters in heat-stressed cows. Twenty Chinese Holstein cows in early lactation (51.00 ± 4.92 kg milk/d, 71 ± 10.94 d in milk and 2.68 ± 0.73 parities) were included in this experiment and randomly divided into four groups (n = 5/group). The four experimental groups consisted of one control group (0 g RP-GABA/d) and three treatment groups, given 5, 7.5 and 10 g RP-GABA/d of dry matter (DM) per cow, respectively. The results showed that supplementing high-yielding cows with 10 g/d of RP-GABA improved milk protein production but had no effect on the improvement of other production performance, the alleviation of heat stress in cows, or the improvement of immune function and antioxidant capacity. Ultimately, we conclude that the supplementation of 10 g/d RP-GABA to heat-stressed, high-yielding dairy cows can provide a degree of performance enhancement. Furthermore, our study provides some reference for nutritional improvement measures for summer heat stress in dairy cows, especially high-yielding cows.
With ambient temperatures rising by 1.0°C since the 19th century and a further 1.5°C expected between 2030 and 2052, heat stress is a major stressor affecting dairy production in the context of global warming (1). Heat stress is defined as the sum of the internal and external forces acting on the animal, resulting in an increase in body temperature and causing a physiological response (2). When heat stress occurs in cows, they are unable to regulate their body temperature effectively and have difficulty producing normally, resulting in increased body temperature and respiratory rate, reduced rumination time and feed intake, ultimately leading to reduced milk production and reproductive performance (2–6). Although some of the negative effects associated with heat stress can be helped by the provision of feeding facilities such as shades, fans and misters, heat stress remains a serious problem in the dairy industry (7–9). Hence, there is a need to explore new nutritional strategies based on physiological and metabolic adaptations, which can help to help cows resist heat stress (10).
The γ-aminobutyric acid (GABA) is an important functional non-protein amino acid and is the predominant inhibitory neurotransmitter in the central nervous tissue of animals (11, 12). GABA not only has various physiological activities such as sedation, anti-inflammation, appetite regulation and hormone secretion, but also has certain physiological functions such as regulation of body temperature and feed intake (12–15). Under hot environment conditions, a single oral dose of GABA can reduce the body’s core temperature by reducing total heat production (16). GABA can improve animal performance and organism health by increasing feed intake, apparent nutrient utilization and immunity (12, 13, 17, 18). In the hot summer months, feeding GABA to cows can reduce rectal temperature, increase DMI and milk yield, and improve milk protein and lactose concentrations, thereby alleviating heat stress without affecting nutrient digestibility (13). Cheng et al. showed that supplementing heat-stressed cows with rumen-protected GABA improved their immune function and antioxidant activity (17). In addition to this, dietary supplementation with GABA improved nutrient digestibility, growth performance and antioxidant status in heat-stressed beef cows (18). Chen et al. study showed that GABA in synergy with Chinese herbs could effectively modulate the heat stress response in beef cows by improving antioxidant capacity, serum parameters and heat shock protein (HSP) expression (19). Previous studies have shown GABA to be beneficial to heat-stressed cows in terms of feed intake, lactation performance and animal health. However, most of the cows selected for these studies were in mid-lactation rather than at the peak of lactation. The nutritional requirements of high-yielding cows under heat stress conditions are more stringent, but the role of RP-GABA in this is poorly understood.
Hence, we hypothesized that supplementing heat-stressed cows in early lactation with rumen-protected GAGB (RP-GABA) during the summer months will improve lactation performance and body health. Therefore, the aim of this trial was to investigate the effect of supplementing the diet with rumen-protective GABA on lactation performance, serum immunity and antioxidant parameters in heat-stressed cows during lactation.
This experiment was conducted in July 2022 at the Jinshan Star II dairy farm in Shanghai, China. All cows used in this study were kept under the permission of the standards of Yangzhou University, the Institutional Animal Care and Use Committee (SYXK (Su) IACUC 2016–0019). Twenty lactating Holstein bovines (51.00 ± 4.92 kg milk/d, 71 ± 10.94 d in milk and 2.68 ± 0.73 parities at the start of the experiment) were included in this experiment and randomly divided into four groups (n = 5/group). The experiment lasted for 48 days as phase 1 (1–12 days), phase 2 (13–24 days), phase 3 (25–36 days) and phase 4 (27–48 days). The four experimental groups consisted of a control group (0 g RP-GABA/d) and three treatment groups, given 5, 7.5 and 10 g RP-GABA/d DM per cow, respectively. The RP-GABA (75% GABA coated by 25% hydrogenated palm oil, 12 h Rumen protection >85%, Jinan Xuze Biotechnology Co., Ltd.) was supplemented to cows using the same method as cheng et al. (20). Specifically, a portion of the TMR was mixed with RP-GABA and fed to the cows first, and then the remaining TMR was supplemented after the cows had finished eating. The TMR was formulated according to the NRC and its composition and nutritional levels are shown in Table 1. All test cows were assigned to the same barn (different segregated areas of the same barn) for management, with a consistent cooling system, free access to fresh water and a 10% refusal diet. Cows were milked 3 times a day at 8-h intervals (6,00, 14:00 and 22:00). The ambient temperature (T) and relative humidity (RT) of the barn were recorded daily (07,00, 14:00 and 21:00) using the thermometer and hygrometer on the barn temperature control and ventilation system. THI was calculated using the following formula: THI = (1.8 × T + 32) - (0.55–0.0055 × RH) × (1.8 × T - 26) (21).
In the last two days of each experimental phase the feed provided and the remaining feed was weighed to determine DMI. All feed collected was mixed in proportion and subsequently dried and stored in an oven at 65°C for 48 h until its chemical composition was analyzed according to the previous method (20).
Milk production was measured on the last day of each experimental phase and milk samples were collected from the morning, mid and evening milkings using a meter (DeLaval, Sweden) and milk samples were synthesized according to 4:3:3. This was then submitted to Dairy One Cooperative Inc. (Shanghai, China) for milk composition analysis within 12 h.
On the same experimental day as the milk yield calculation, a blood sample (15 mL) was collected from the tail vein of the cow prior to morning feeding using a syringe. It was immediately placed in a 15 mL tube, allowed to clot and then centrifuged at 3000 g for 15 min at 4°C to collect the serum. The serum samples were stored in liquid nitrogen for rapid cooling and then stored at - 80°C for later biochemical analysis. The superoxide dismutase (SOD), glutathione peroxidase (GSH-PX), total antioxidant capacity (T-AOC), and malondialdehyde (MDA) concentrations were determined using a colourimetric kit (Nanjing Jiancheng Bioengineering Institute). Alanine aminotransferase (ALT), aspartate aminotransferase (AST), alkaline phosphatase (ALP), total protein (TP), triglyceride (TG) and nonesterified fatty acid (NEFA) were detected using a biochemical analyzer (Mindray; BS-420) and the kits (BioSino Bio-Technology & Science, Bejing, China) mentioned. IgG, tumor necrosis factor alpha (TNF-α), interleukin 6 (IL-6), heat shock protein 70 (HSP-70), neuropeptide Y (NPY), corticotrophin releasing hormone (CRH), adrenocorticotropic hormone (ACTH), thyroid stimulating hormone (TSH) and cortisol (COR) were detected using an Elisa kit (Beijing Sino-Uk Institute of Biological Technology, Bejing, China; intra-assay CV ≤ 5%; interassay CV ≤ 10%).
Data obtained from the trials were subjected to a two-way ANOVA using the General Linear Model (GLM) procedure of SAS 9.4 (SAS Institute Inc., Cary, NC, United States). The model included the amount of supplemented RP-GABA, the experimental phase and the main effects of its interaction. Each cow was used as the experimental unit. Differences between means were tested using the least significant difference (LSD) method and significance was declared at p < 0.05.
The mean THI was 80.66 (73.87 to 80.6) at 07:00 h, 81.84 (75.6 to 83.3) at 14:00 h and 79.48 (74.8 to 80.8) at 21:00 h (Figure 1). As the trial progressed, there was a decreasing trend in the average temperature of THI in the barn at each phase (phase 1: 84.43; phase 2: 84.29; phase 3: 77.79; phase 4: 76.14). The rectal temperature in heat-stressed cows decreased with the test phase (p < 0.001) but was not affected by RP-GAGB supplementation (p > 0.05).
As shown in Table 2, The interaction between the addition of RP-GABA and the phase of trial did not affect the productive performance of the cows (p > 0.05). Supplementation with RP-GABA did not affect (p > 0.05) cow DMI and cows in the phase 1 of trial had the lowest (p < 0.001) DMI. Under RP-GABA treatment conditions, milk protein production was higher in cows supplemented with 10 g/d RP-GABA than in the control and 5 g/d RP-GABA treatment groups (p < 0.05). However, the addition of a low dose (5 g/d) of RP-GABA to the ration reduced milk fat production compared to the control (p < 0.05).
Under the time conditions of the trial, cows had the highest yields of lactation, 4% ECM, FCM, milk fat, milk protein, lactose, and total solids in milk in the phase 1 of the trial (p < 0.05). However, milk fat, milk protein, lactose and total solids in milk were highest in the phase 4 of the trial (p < 0.001). In contrast, MUN levels were lower in the phase 3 of feeding compared to the other phase (p < 0.001) (see Figure 2).
The interaction between the addition of RP-GABA and the duration of the test affected blood MDA (p < 0.05) levels (Table 3), but had no effect on blood TP, ALT and NEFA (p > 0.05).
Under RP-GABA treatment conditions, supplementation with 7.5 g/d RP-GABA up-regulated blood MDA and TG levels (p < 0.05). In contrast, the addition of 10 g/d RP-GABA to the diet increased blood AST and ALP concentrations (p < 0.05).
Under the time conditions of the trial, SOD, GSH-PX, TAOC and TSH concentrations in the blood of cows were highest in the phase 1 of the trial compared to the other phases (p < 0.05). In the phase 2 of the trial, IgG levels in the blood of cows were higher than phase 3 and phase 4 (p < 0.05). However, MDA, NPY, CRH, ACTH, TNF-α, IL-6, HSP-70, COR, AST, and TG concentrations were higher in cow blood in the phase 4 (p < 0.05).
Chinese Holstein cows are very susceptible to heat stress and have a relatively narrow thermal comfort zone due to their cold and heat-tolerant nature (1, 22). In hot conditions, cows are susceptible to heat stress, which can impair their performance and health (1, 23). The Earth’s ambient temperature has increased by 1.0°C since the 19th century and is expected to increase by a further 1.5°C between 2030 and 2052, making managing heat stress more challenging than ever (1, 8). In a previous study, cheng et al. showed that supplementing mid-lactation cows with RP-GABA could alleviate heat stress by reducing the rectal temperature, increasing DMI and milk production and improving milk composition (13, 17). However, it is unclear whether supplementation with RP-GABA has the ability to alleviate heat stress in early lactation cows. Maintaining lactation and body health in heat-stressed cows in early lactation appears to be more challenging than ever due to the stress of the immediate parturition and transition period and the rapid attainment of peak lactation in response to endocrine hormones (24–26). Our hypothesis is that supplementation of RP-GAGB to heat-stressed cows in early lactation during the summer may improve lactation performance and body health by enhancing antioxidant capacity and attenuating the heat shock response. In this study, RP-GAGB supplementation increased milk protein production in cows, although it did not enhance antioxidant capacity or attenuate the heat shock response. These findings may provide a more effective nutritional strategy for maintaining the performance of heat-stressed cows in early lactation.
In summer, cows begin to experience the negative effects of severe heat stress when rectal temperatures exceed 38.9°C (22, 27). In addition, cows begin to experience heat stress when THI > 72 (27, 28). In our study, the maximum THI averaged 81.84 and the minimum THI averaged 79.48, and the majority of the time cows had rectal temperatures above 38.9°C. In addition to this, it was easy to see from the THI and rectal temperatures that cows suffered more severe heat stress in the first two feeding stages due to higher THI. In conclusion, the cows were subjected to heat stress conditions during the experiment and the THI in the barn gradually decreased as the feeding trial progressed.
In general, heat stress reduces DMI and is a major factor that negatively affects the health and production of cows (25, 29). GABA and its agonists may be able to regulate feed intake in some mammals through effects on the central and peripheral nervous systems (13, 17). Cheng et al. showed that DMI tended to increase linearly with increasing levels of GABA supplementation during the hot summer months, possibly because GABA increased feed intake by reducing heat stress in cows (13). GABA can co-express with NPY in the central nervous system and the increased DMI in GABA-supplemented cows may be associated with increased serum levels of GABA and NPY in these cows (12, 30). Differences in DMI may be due to differences in test animal conditions, GABA supplementation levels, ambient temperature and experimental diet (13). Despite being under summer heat stress, the cows used in this experiment were in peak lactation and their lactation and DMI were high enough that increasing their DMI and milk production again through GABA supplementation would be a huge challenge. In addition, in this experiment, cow DMI and serum NPY concentrations were upregulated with increasing time in the experiment. This may be partly due to the down-regulation of THI in cows to promote feeding, and partly due to cows moving out of the transition period into peak feeding, unfortunately, these related hormonal change indicators were not tested. Although we observed that the interaction between the addition of RP-GABA and the duration of the trial did not affect cow performance, excitingly, we found that 10 g/d of RP-GABA treatment up-regulated milk protein production in cows, suggesting that GABA may have the potential to improve milk protein secretion. However, information on the effect of GABA on milk protein synthesis in dairy cows is scarce and further research is needed.
Heat stress activates the hypothalamic–pituitary–adrenal axis in cows, and this neuroendocrine response aims to reduce the negative effects of heat stress. In fact, during HS, the paraventricular nucleus of the hypothalamus produces corticotropin-releasing hormone (CRH) (31, 32). CRH acts on the adrenocorticotropic hormone secretion of the pituitary gland, ACTH, which regulates heat stress in cows by influencing cortisol (31). In addition to the adrenal glands, the thyroid gland is also highly sensitive to temperature and is affected by heat stress imposed by high ambient temperatures (31–33). However, in the present study, GABA did not alter the relevant neuroendocrine hormones (e.g., CRH, ACTH, TSH and COR) in the blood of heat-stressed cows. Surprisingly, the aforementioned endocrine hormone concentrations were affected by the duration of the experiment, which may be due to the complex endocrine changes in cows during early lactation.
Higher DMI and stable lactation in dairy cows require a better antioxidant status, which is reflected by changes in serum metabolites (12, 34). SOD plays an important role in the animal’s antioxidant defense mechanism, while GSH-PX scavenges peroxides and hydroxyl radicals produced during cellular respiratory metabolism (19, 35). T-AOC is the total antioxidant level of various antioxidants and antioxidant enzymes, representing the body’s ability to fight oxidation (12, 34). The MDA is one of the lipid peroxidation products, and changes in MDA reflect the rate and intensity of lipid peroxidation in the body, and also indirectly reflect the degree of peroxidative damage in tissues (36). Wang et al. showed that GABA supplementation enhanced the antioxidant status of cows by reducing MDA accumulation in serum and increasing GSH-PX and SOD concentrations (12, 34). This is consistent with the study by Cheng et al. where cows fed RP-GABA had an enhanced antioxidant status and reduced oxidative stress under heat stress, as evidenced by a reduction in serum MDA and an increase in SOD, GSH-PX and T-AOC (17). However, in our study, GABA supplementation did not affect the serum concentrations of SOD, GSH-PX and T-AOC in cows and RP-GABA supplementation at 7.5 g/d promoted the accumulation of MDA. In contrast, on the time factor, as the experiment and lactation progressed, MDA accumulated in cow serum and SOD, GSH-PX and T-AOC levels decreased. Considering that the experimental herd maintained high lactation during the hot summer months, we suggest that the accumulation of MDA and the reduced levels of SOD, GSH-PX and T-AOC may be related to the lactation stress endured by the cows, but more data are needed to prove this.
Serum antioxidant levels in dairy cows can influence the level of inflammation in the organism, which is also related to the cow’s immunity. In previous studies, heat stress has led to a down-regulation of serum IgG and thus humoral immunity (37, 38). In addition, heat stress may also lead to inflammation, as evidenced by increased serum levels of IL-6 and TNF-α in cows (39). It is known that increased temperature leads to increased synthesis of HSP, and in particular HSP-70 expression is increased in heat-stressed cows, which has a protective effect against cellular stress (40–42). In our study, the addition of RP-GABA had no effect on IgG, IL-6, TNF-α and HSP-70 in cow serum, which is inconsistent with our hypothesis. Interestingly, on the time scale, levels of the inflammatory factors IL-6 and TNF, as well as HSP-70, which has an anti-stress effect, were upregulated in cow serum as the experiment progressed. Taking into account the changes in body antioxidant levels, we speculate that from 70 days to 120 days of lactation, cows with high yields also experience significant oxidative stress, which leads to the accumulation of inflammatory factors in their serum. Therefore, although cows maintain high-yielding performance despite heat stress, it is not a healthy state and we need to find new ways to promote cow health.
In summary, supplementing high-yielding cows with 10 g/d of RP-GABA during the hot summer months improved milk protein production but had no effect on other performance improvements, heat stress relief, immune function or antioxidant capacity. Although the results of this trial did not prove the initial hypothesis, given the excellent high yielding performance of the trial cows and the significant challenge of improving performance under heat stress, we believe that supplementation of 10 g/d RP-GABA to heat-stressed high yielding cows could provide some boost to performance. In conclusion, our study provides some reference for nutritional improvement measures for summer heat stress in dairy cows, especially high yielding cows.
In conclusion, this study shows that supplementing 10 g/d RP-GABA to early lactation cows in heat stress during the hot summer months can improve milk protein production, but has no effect on reducing heat stress, improving immune function or antioxidant capacity, which provides some nutritional options for the management of high yielding cows on summer farms.
The original contributions presented in the study are included in the article/supplementary material, further inquiries can be directed to the corresponding author.
The animal studies were approved by Yangzhou University, the Institutional Animal Care and Use Committee (SYXK (Su) IACUC 2016-0019). The studies were conducted in accordance with the local legislation and institutional requirements. Written informed consent was obtained from the owners for the participation of their animals in this study.
YS, ZC, and ML conceptualized of research. ZC, WL, TW, and WW curated experimental data. WL and TW performed investigation for research. ZC, YS, TW, WL, and ML drafted writing-original manuscript. ZC and WW analyzed formal analysis. YS and ML presented methodology of research and offered resources. ZC and ML accomplished supervision for research. YS offered funding acquisition. All authors contributed to the article and approved the submitted version.
This study was supported by the Sponsored by Shanghai Risingstar Program (NO.21QB1400300) and China Agriculture Research System (CARS-36).
YS, TW, and WW are employed by Bright Farming Co. LTD.
The remaining authors declare that the research was conducted in the absence of any commercial or financial relationships that could be construed as a potential conflict of interest.
All claims expressed in this article are solely those of the authors and do not necessarily represent those of their affiliated organizations, or those of the publisher, the editors and the reviewers. Any product that may be evaluated in this article, or claim that may be made by its manufacturer, is not guaranteed or endorsed by the publisher.
1. Becker, CA, Collier, RJ, and Stone, AE. Invited review: physiological and behavioral effects of heat stress in dairy cows. J Dairy Sci. (2020) 103:6751–70. doi: 10.3168/jds.2019-17929
2. Tao, S, Orellana, RM, Weng, X, Marins, TN, Dahl, GE, and Bernard, JK. Symposium review: the influences of heat stress on bovine mammary gland function. J Dairy Sci. (2018) 101:5642–54. doi: 10.3168/jds.2017-13727
3. Bernabucci, U, Biffani, S, Buggiotti, L, Vitali, A, Lacetera, N, and Nardone, A. The effects of heat stress in Italian Holstein dairy cattle. J Dairy Sci. (2014) 97:471–86. doi: 10.3168/jds.2013-6611
4. Schüller, LK, Burfeind, O, and Heuwieser, W. Impact of heat stress on conception rate of dairy cows in the moderate climate considering different temperature–humidity index thresholds, periods relative to breeding, and heat load indices. Theriogenology. (2014) 81:1050–7. doi: 10.1016/j.theriogenology.2014.01.029
5. Wilson, SJ, Marion, RS, Spain, JN, Spiers, DE, Keisler, DH, and Lucy, MC. Effects of controlled heat stress on ovarian function of dairy cattle. 1. Lactating cows. J Dairy Sci. (1998) 81:2124–31. doi: 10.3168/jds.S0022-0302(98)75788-1
6. Bernabucci, U, Basiricò, L, Morera, P, Dipasquale, D, Vitali, A, Piccioli Cappelli, F, et al. Effect of summer season on milk protein fractions in Holstein cows. J Dairy Sci. (2015) 98:1815–27. doi: 10.3168/jds.2014-8788
7. Grinter, LN, Mazon, G, and Costa, JHC. Voluntary heat stress abatement system for dairy cows: does it mitigate the effects of heat stress on physiology and behavior? J Dairy Sci. (2023) 106:519–33. doi: 10.3168/jds.2022-21802
8. Becker, CA, and Stone, AE. Graduate student literature review: heat abatement strategies used to reduce negative effects of heat stress in dairy cows. J Dairy Sci. (2020) 103:9667–75. doi: 10.3168/jds.2020-18536
9. Nordlund, KV, Strassburg, P, Bennett, TB, Oetzel, GR, and Cook, NB. Thermodynamics of standing and lying behavior in lactating dairy cows in freestall and parlor holding pens during conditions of heat stress. J Dairy Sci. (2019) 102:6495–507. doi: 10.3168/jds.2018-15891
10. Min, L, Li, D, Tong, X, Nan, X, Ding, D, Xu, B, et al. Nutritional strategies for alleviating the detrimental effects of heat stress in dairy cows: a review. Int J Biometeorol. (2019) 63:1283–302. doi: 10.1007/s00484-019-01744-8
11. Kim, K, and Yoon, H. Gamma-aminobutyric acid Signaling in damage response, metabolism, and disease. IJMS. (2023) 24:4584. doi: 10.3390/ijms24054584
12. Wang, DM, Wang, C, Liu, HY, Liu, JX, and Ferguson, JD. Effects of rumen-protected γ-aminobutyric acid on feed intake, lactation performance, and antioxidative status in early lactating dairy cows. J Dairy Sci. (2013) 96:3222–7. doi: 10.3168/jds.2012-6285
13. Cheng, JB, Bu, DP, Wang, JQ, Sun, XZ, Pan, L, Zhou, LY, et al. Effects of rumen-protected γ-aminobutyric acid on performance and nutrient digestibility in heat-stressed dairy cows. J Dairy Sci. (2014) 97:5599–607. doi: 10.3168/jds.2013-6797
14. Zhang, S, Zhao, J, Hu, J, He, H, Wei, Y, Ji, L, et al. Gama-aminobutyric acid (GABA) alleviates hepatic inflammation via GABA receptors/TLR4/NF-κB pathways in growing-finishing pigs generated by super-multiparous sows. Animal Nutrition. (2022) 9:280–90. doi: 10.1016/j.aninu.2022.02.001
15. Rackwitz, R, and Gäbel, G. Gamma-aminobutyric acid (GABA) permeates ovine ruminal and jejunal epithelia, mainly by passive diffusion. J Anim Physiol Anim Nutr. (2017) 101:38–45. doi: 10.1111/jpn.12497
16. Miyazawa, T, Kawabata, T, Okazaki, K, Suzuki, T, Imai, D, Hamamoto, T, et al. Oral administration of γ-aminobutyric acid affects heat production in a hot environment in resting humans. J Physiol Anthropol. (2012) 31:3. doi: 10.1186/1880-6805-31-3
17. Cheng, J, Zheng, N, Sun, X, Li, S, Wang, J, and Zhang, Y. Feeding rumen-protected gamma-aminobutyric acid enhances the immune response and antioxidant status of heat-stressed lactating dairy cows. J Therm Biol. (2016) 60:103–8. doi: 10.1016/j.jtherbio.2016.06.011
18. Guo, K, Cao, H, Zhu, Y, Wang, T, Hu, G, Kornmatitsuk, B, et al. Improving effects of dietary rumen protected γ-aminobutyric acid additive on apparent nutrient digestibility, growth performance and health status in heat-stressed beef cattle. Anim Sci J. (2018) 89:1280–6. doi: 10.1111/asj.13053
19. Chen, J, Mao, Y, Guo, K, Wu, H, Song, X, Qu, M, et al. The synergistic effect of traditional Chinese medicine prescription and rumen-protected γ-aminobutyric acid on beef cattle under heat stress. J Anim Physiol Anim Nutr. (2021) 105:807–15. doi: 10.1111/jpn.13507
20. Cheng, Z, Meng, Z, Tan, D, Datsomor, O, Zhan, K, Lin, M, et al. Effects of supplementation of sodium acetate on rumen fermentation and microbiota in postpartum dairy cows. Front Microbiol. (2022) 13:1053503. doi: 10.3389/fmicb.2022.1053503
21. National Oceanic and Atmospheric Administration. Livestock hot weather stress. Regional Operations Manual Letter. Kansas City: Department of Commerce, NOAA, National Weather Service Central Region (1976) C31–76.
22. Kadzere, CT, Murphy, MR, Silanikove, N, and Maltz, E. Heat stress in lactating dairy cows: a review. Livest Prod Sci. (2002) 77:59–91. doi: 10.1016/S0301-6226(01)00330-X
23. Dado-Senn, B, Ouellet, V, Lantigua, V, Van Os, J, and Laporta, J. Methods for detecting heat stress in hutch-housed dairy calves in a continental climate. J Dairy Sci. (2023) 106:1039–50. doi: 10.3168/jds.2022-22237
24. Koch, F, Thom, U, Albrecht, E, Weikard, R, Nolte, W, Kuhla, B, et al. Heat stress directly impairs gut integrity and recruits distinct immune cell populations into the bovine intestine. Proc Natl Acad Sci U S A. (2019) 116:10333–8. doi: 10.1073/pnas.1820130116
25. Baumgard, LH, and Rhoads, RP. Effects of heat stress on Postabsorptive metabolism and energetics. Annu Rev Anim Biosci. (2013) 1:311–37. doi: 10.1146/annurev-animal-031412-103644
26. Horst, EA, Kvidera, SK, and Baumgard, LH. Invited review: the influence of immune activation on transition cow health and performance—a critical evaluation of traditional dogmas. J Dairy Sci. (2021) 104:8380–410. doi: 10.3168/jds.2021-20330
27. Bohmanova, J, Misztal, I, and Cole, JB. Temperature-humidity indices as indicators of Milk production losses due to heat stress. J Dairy Sci. (2007) 90:1947–56. doi: 10.3168/jds.2006-513
28. Frigeri, KDM, Kachinski, KD, Ghisi, NDC, Deniz, M, Damasceno, FA, Barbari, M, et al. Effects of heat stress in dairy cows raised in the confined system: a Scientometric review. Animals. (2023) 13:350. doi: 10.3390/ani13030350
29. Dash, S, Chakravarty, AK, Singh, A, Upadhyay, A, Singh, M, and Yousuf, S. Effect of heat stress on reproductive performances of dairy cattle and buffaloes: a review. Vet World. (2016) 9:235–44. doi: 10.14202/vetworld.2016.235-244
30. Pu, S, Jain, MR, Horvath, TL, Diano, S, Kalra, PS, and Kalra, SP. Interactions between neuropeptide Y and γ -aminobutyric acid in stimulation of feeding: a morphological and pharmacological analysis*. Endocrinology. (1999) 140:933–40. doi: 10.1210/endo.140.2.6495
31. Mishra, SR. Thermoregulatory responses in riverine buffaloes against heat stress: an updated review. J Therm Biol. (2021a) 96:102844. doi: 10.1016/j.jtherbio.2021.102844
32. Mishra, SR. Behavioural, physiological, neuro-endocrine and molecular responses of cattle against heat stress: an updated review. Trop Anim Health Prod. (2021b) 53:400. doi: 10.1007/s11250-021-02790-4
33. Ahmad, M, Bhatti, JA, Abdullah, M, Javed, K, Ali, M, Rashid, G, et al. Effect of ambient management interventions on the production and physiological performance of lactating Sahiwal cattle during hot dry summer. Trop Anim Health Prod. (2018) 50:1249–54. doi: 10.1007/s11250-018-1551-5
34. Wang, DM, Chacher, B, Liu, HY, Wang, JK, Lin, J, and Liu, JX. Effects of γ-aminobutyric acid on feed intake, growth performance and expression of related genes in growing lambs. Animal. (2015) 9:445–8. doi: 10.1017/S1751731114002651
35. Zhao, H, Wang, X, Liu, X, Zhang, J, Wan, L, and Jia, L. Antioxidant and Hypolipidemic activities of acid-depolymerised exopolysaccharides by Termitomyces albuminosus. Oxidative Med Cell Longev. (2019) 2019:1–13. doi: 10.1155/2019/8915272
36. Xing, J-J, Hou, J-G, Liu, Y, Zhang, R-B, Jiang, S, Ren, S, et al. Supplementation of Saponins from leaves of Panax quinquefolius mitigates cisplatin-evoked cardiotoxicity via inhibiting oxidative stress-associated inflammation and apoptosis in mice. Antioxidants. (2019) 8:347. doi: 10.3390/antiox8090347
37. Kelley, KW, Osborne, CA, Evermann, JF, Parish, SM, and Gaskins, CT. Effects of chronic heat and cold stressors on plasma immunoglobulin and mitogen-induced Blastogenesis in calves. J Dairy Sci. (1982) 65:1514–28. doi: 10.3168/jds.S0022-0302(82)82376-X
38. Sun, Y, Liu, J, Ye, G, Gan, F, Hamid, M, Liao, S, et al. Protective effects of zymosan on heat stress-induced immunosuppression and apoptosis in dairy cows and peripheral blood mononuclear cells. Cell Stress and Chaperones. (2018) 23:1069–78. doi: 10.1007/s12192-018-0916-z
39. Chen, S, Wang, J, Peng, D, Li, G, Chen, J, and Gu, X. Exposure to heat-stress environment affects the physiology, circulation levels of cytokines, and microbiome in dairy cows. Sci Rep. (2018) 8:14606. doi: 10.1038/s41598-018-32886-1
40. Guerriero, V, and Raynes, DA. Synthesis of heat stress proteins in lymphocytes from livestock. J Anim Sci. (1990) 68:2779. doi: 10.2527/1990.6892779x
41. Kim, W-S, Ghassemi Nejad, J, Roh, S-G, and Lee, H-G. Heat-shock proteins gene expression in peripheral blood mononuclear cells as an indicator of heat stress in beef calves. Animals. (2020) 10:895. doi: 10.3390/ani10050895
Keywords: rumen-protective γ-aminobutyric acid, heat stress, lactating cows, serum biochemistry, lactation performance
Citation: Su Y, Cheng Z, Liu W, Wu T, Wang W and Lin M (2023) Effects of rumen-protective γ-aminobutyric acid additive on lactation performance and serum biochemistry in heat-stressed cows. Front. Vet. Sci. 10:1228155. doi: 10.3389/fvets.2023.1228155
Received: 24 May 2023; Accepted: 06 September 2023;
Published: 22 September 2023.
Edited by:
Yangchun Cao, Northwest A&F University, ChinaReviewed by:
Jianbo Cheng, Anhui Agricultural University, ChinaCopyright © 2023 Su, Cheng, Liuweng, Wu, Wang and Lin. This is an open-access article distributed under the terms of the Creative Commons Attribution License (CC BY). The use, distribution or reproduction in other forums is permitted, provided the original author(s) and the copyright owner(s) are credited and that the original publication in this journal is cited, in accordance with accepted academic practice. No use, distribution or reproduction is permitted which does not comply with these terms.
*Correspondence: Miao Lin, bGlubWlhb0B5enUuZWR1LmNu
†These authors have contributed equally to this work
Disclaimer: All claims expressed in this article are solely those of the authors and do not necessarily represent those of their affiliated organizations, or those of the publisher, the editors and the reviewers. Any product that may be evaluated in this article or claim that may be made by its manufacturer is not guaranteed or endorsed by the publisher.
Research integrity at Frontiers
Learn more about the work of our research integrity team to safeguard the quality of each article we publish.