- 1Arrábida Veterinary Hospital, Arrábida Animal Rehabilitation Center, Setubal, Portugal
- 2Superior School of Health, Protection and Animal Welfare, Polytechnic Institute of Lusophony, Lisboa, Portugal
- 3Faculty of Veterinary Medicine, Lusófona University, Lisboa, Portugal
- 4Faculty of Veterinary Medicine, University of Lisbon, Lisboa, Portugal
- 5Departamento de Clínicas Veterinárias, Instituto de Ciências Biomédicas de Abel Salaza, Universidade do Porto, Porto, Portugal
- 6Centro de Estudos de Ciência Animal, Instituto de Ciências, Tecnologias e Agroambiente da Universidade do Porto, Porto, Portugal
- 7Associate Laboratory for Animal and Veterinary Science (AL4AnimalS), Lisboa, Portugal
- 8Instituto Universitário de Ciências da Saúde (IUCS), Cooperativa de Ensino Superior Politécnico e Universitário (CESPU), Gandra, Portugal
- 9CIISA - Centro Interdisciplinar-Investigáo em Saúde Animal, Faculdade de Medicina Veterinária, Av. Universi dade Técnica de Lisboa, Lisboa, Portugal
Introduction: Degenerative myelopathy (DM) is a neurodegenerative spinal cord disease with upper motor neurons, with progressive and chronic clinical signs, similar to amyotrophic lateral sclerosis (ALS). DM has a complex etiology mainly associated with SOD1 gene mutation and its toxic role, with no specific treatment. Daily intensive rehabilitation showed survival time near 8 months but most animals are euthanized 6–12 months after clinical signs onset.
Methods: This prospective controlled blinded cohort clinical study aims to evaluate the neural regeneration response ability of DM dogs subjected to an intensive neurorehabilitation protocol with mesenchymal stem cells (MSCs) transplantation. In total, 13 non-ambulatory (OFS 6 or 8) dogs with homozygous genotype DM/DM and diagnosed by exclusion were included. All were allocated to the intensive neurorehabilitation with MSCs protocol (INSCP) group (n = 8) or to the ambulatory rehabilitation protocol (ARP) group (n = 5), which differ in regard to training intensity, modalities frequency, and MSCs transplantation. The INSCP group was hospitalized for 1 month (T0 to T1), followed by MSCs transplantation (T1) and a second month (T2), whereas the ARP group was under ambulatory treatment for the same 2 months.
Results: Survival mean time of total population was 375 days, with 438 days for the INSCP group and 274 for the ARP group, with a marked difference on the Kaplan–Meier survival analysis. When comparing the literature's results, there was also a clear difference in the one-sample t-test (p = 0.013) with an increase in time of approximately 70%. OFS classifications between groups at each time point were significantly different (p = 0.008) by the one-way ANOVA and the independent sample t-test.
Discussion: This INSCP showed to be safe, feasible, and a possibility for a long progression of DM dogs with quality of life and functional improvement. This study should be continued.
1. Introduction
Degenerative myelopathy (DM) is a neurodegenerative spinal cord disease characterized by the progressive and chronic onset of clinical signs in large-breed dogs (1, 2). These signs are mainly of T3-L3 neuro-localization and include proprioceptive ataxia and spastic paraparesis (3, 4), similar to those presented in human patients with amyotrophic lateral sclerosis (ALS) (5).
Most dogs are between 5 and 14 years of age (6) and clinical signs are presented on average in 9 years old (3, 7), regardless of gender and other diseases (3, 8).
This disease has a prevalence of 0.19% in dogs (1, 7) but a specific prevalence of 2.01% in the German shepherd (1), which is the first described breed with DM (9). However, different authors have already described histological confirmation of the disease in other breeds, such as Siberian Husky (10), Miniature Poodle (11), Boxer (12–14), Pembroke Welsh Corgi (1, 15–17), Rhodesian ridgeback (12), Chesapeake Bay Retriever (12), Bernese Bouvier, Kerry Blue terrier, Golden Retriever, Pug (3) and also mixed breed dogs (9). There are also other described breeds but without histological confirmation (6).
Degenerative myelopathy has a complex and unknown etiology (7), but recent studies have been developed demonstrating the SOD1 gene mutation as one of the main causes (18). This gene presents as a homodimer that converts superoxide radicals into hydrogen and oxygen peroxide (5). Thus, superoxide dismutase (SOD), an enzyme involved in the dismutase of superoxide radicals, has three SOD isoforms: cytoplasmic (SOD1), mitochondrial (SOD2), and secreted outside the cell (SOD3). Also, some research in DM has highlighted two SOD1 mutant proteins (E40K and T18S) as insoluble and capable of inducing a toxic role associated with these isoforms (19–21).
There are several differential diagnoses related to geriatric dogs and T3-L3 spinal cord lesions (e.g., intervertebral disc disease (IVDD) Hansen type II, neoplasia, and meningoencephalomyelitis) (4). Therefore, antemortem diagnosis depends on the clinical history (the onset and progression of neurological signs), upper motor neurons (UMNs) clinical signs compatible with T3-L3 myelopathy, absence of spinal cord compressive lesion, and inflammatory changes on the cerebrospinal fluid (CSF). The gold standard complementary exam is magnetic resonance imaging (MRI) but computed tomography/CT is also performed to exclude disc disease, discospondylitis, and neoplasia (4, 22).
Thus, for the final diagnosis of DM, it is necessary to have compatible history and clinical signs (9), the presence of SOD1 gene mutation (5), and the exclusion of other spinal cord diseases (4, 7). Nevertheless, DM may coexist with other diseases of the nervous system, resulting in the need for an accurate diagnosis in the future, for example, through specific biomarkers (23). According to a study carried out in 2017, a suitable biomarker would be the neurofilament heavy chain (pNFH) that is released into the interstitial fluid during axonal injury and neurodegeneration (24).
The connection between DM and ALS is based on the genetic similarities of both diseases, that is, the mutation in the SOD1 nucleotide (25). In ALS, approximately 20% of cases have the SOD1 mutation (26) transmitted as an autosomal dominant disease, whereas DM is an autosomal recessive one (5). Furthermore, the results of studies performed in dogs with DM may allow a better understanding of the effectiveness of therapeutic interventions in the treatment of ALS (3).
There is no specific treatment for DM (6, 27). The medical pharmacological approach is based on non-steroidal anti-inflammatory drugs or steroids, such as prednisolone, due to its effect on the management of neurological signs, however with low evidence with regard to disease progression (28).
Other therapeutic approaches include an initial protocol based on active physical exercises and supplementation with Vitamin B, Vitamin E, Aminocaproic acid, and N-acetylcysteine (8, 29).
Aminocaproic acid blocks inflammation pathways and reduces fibrin degradation (29). On the other hand, N-acetylcysteine, a glutathione precursor, scavenges free radicals, preventing the activation of enzymes that cause tissue damage (30). Vitamin supplementation can contribute to the improvement of DM dogs, inhibiting the release of prostaglandins and cytokines that act on the inflammation cascade (29).
Degenerative myelopathy dogs that are not on a multimodal approach reach end-stage disease within 6 months of initial diagnosis (29, 31). A study by Kathmann et al. (6) reported that daily physiotherapy increased survival time in these dogs. In 22 DM dogs, the ones that had intensive physiotherapy protocol showed survival of nearly 8 months, when compared to a moderate protocol (~4 months) or without any protocol (~2 months). In another study with dogs under a similar protocol, 15–20% did not show worsening of neurological status, with some dogs surviving for more than 4 years (31). Thus, the prognosis of DM remains to be poor and, in most cases, dogs are euthanized between 6 and 12 months after the first clinical signs (1).
Regenerative medicine is the branch of medicine that promotes tissue regeneration and consequent functional recovery through the regrowth or replacement of injured cells, tissues, or organs. Among the different therapeutic approaches considered in Regenerative Medicine, cell-based therapies, namely those using stem cells, are the most explored. In particular, the transplantation of MSCs, which are multipotent cells isolated from mature tissues with paracrine effects and the ability to differentiate into specific lineages (32), has been studied to potentially regenerate damaged tissues or organs (33, 34). This application has been described in several human diseases with clear pro-regenerative effects, such as diabetes mellitus (35), chronic myeloid leukemia (36), cirrhosis (37), pulmonary fibrosis (38), Crohn's disease s (39), heart failure (40), and diseases of the nervous system, such as multiple sclerosis (41), Parkinson's disease (42), and other neurological diseases (43). More recently, new methodologies have begun to be explored to maximize the effectiveness of MSCs as a therapeutic component, namely the use of their secretion products as a substitute for the use of the cells themselves. The set of soluble factors secreted by cells (secretome) includes growth factors, cytokines, chemokines, and glycoproteins, and the vesicular factors include microvesicles and exosomes. As a whole, the pro-regenerative efficacy of these paracrine factors has been shown to be as effective or more effective than the use of cells, without the disadvantages associated with direct cellular administration in a living organism (44).
In addition, there are several transplantation studies using MSCs applied in the dog as a clinical model, namely in spinal cord injury (45), chronic superficial keratitis (46), dermatitis (47), osteoarthritis, and cartilage regeneration (48–50), as well as other musculoskeletal diseases (51).
Transplantation of stem cells in ALS has been described to decrease clinical symptoms, with reported signs of neuroprotection, nervous tissue healing, and increased survival time (52). Other studies showed that intrathecal administration improved motor function, with less neuronal degenerescence (53), and astrogliosis and microgliosis reduction, mainly due to its anti-inflammatory effects (54).
The set of different modalities associated with active exercises (6) and stem cell administration (54) can potentially increase DM dogs' wellbeing in the long term (55).
In regard to neurorehabilitation modalities, functional electrical stimulation (FES) promotes neuromodulation with a low current intensity allowing muscle contraction (56, 57), with short electric pulses that stimulate motor neurons near the motor point or through peripheral afferent stimulation (57, 58). Electrical parameters are usually from 25 to 50 Hz for 15–20 min each session (59).
Additionally, locomotor training based on task repetition intends to achieve neuroplasticity (57), improving muscle mass and oxidative ability (60). This may result in coordinated, consistent (61), and symmetric ambulation (62). Underwater treadmill training may be a fundamental element in the rehabilitation of these dogs, stimulating the neuromuscular system and increasing the range of motion, even when compared to land treadmill training (63, 64).
Therefore, this prospective controlled blinded cohort clinical study aims to evaluate the neural regeneration response ability of DM dogs subjected to an intensive neurorehabilitation protocol with MSCs transplantation. It is hypothesized that the association of this protocol with stem cell transplantation may be a possibility for a positive and longer progression of these dogs.
2. Materials and methods
This prospective controlled blinded cohort clinical study was conducted at the Arrábida Animal Rehabilitation Center (CRAA and CR2AL, Portugal) between March 2015 and March 2023, after approval from the Lusófona Veterinary Medicine Faculty (Lisbon, Portugal) ethics committee (No. 113-2021) and after signing an informed consent by the owners. Our study has been submitted for publishing on the AVMA Animal Health Studies (AAHSD) website and has been assigned the following number: Study #: AAHSD005642.
2.1. Population presentation
The present study included 13 dogs (n = 13) with non-ambulatory paraparesis, classified with the open field score (OFS) (65) as OFS 6 or OFS 8, without spinal hyperesthesia and that presented UMN clinical signs compatible with T3-L3 neuro-localization at the neurorehabilitation examination, indicating some degree of chronicity and spasticity in the hindlimbs.
In all dogs, the diagnosis of DM was made by exclusion of immune-mediated myelopathies (e.g., babesiosis and ehrlichiosis); parasitic myelopathies (e.g., leishmaniasis, neosporosis, and toxoplasmosis); and viral myelopathy (e.g., distemper). All were performed by serology of blood and CSF. Cytology of the CSF was also performed to analyze the protein content. CT and/or MRI was performed to exclude T3-L3 compressive myelopathies, such as IVDD Hansen type I and type II; spinal arachnoid cysts; traumatic injury; and vascular injury (fibrocartilaginous embolism).
Followed by the negative results of all aforementioned exams, all dogs had to present a positive genetic test for the SOD1 gene mutation exon 2 (homozygous genotype DM/DM) from the same laboratory (Genevet®).
Sample characterization of the population in regard to age, sex, breed, etc., is described in Table 1.
2.2. Study design
After exclusion, 13 dogs remained in the study regardless of age, weight, sex, breed, and clinical occurrences. All underwent a neurorehabilitation consultation on admission and were randomized by stratification according to the owners' treatment decisions. The study group included dogs whose owners accepted intensive neurorehabilitation with stem cells protocol (INSCP) (n = 8) and the control group included dogs subjected to an ambulatory rehabilitation protocol (ARP) (n = 5).
Admission consultation and further evaluations throughout the study were recorded (Canon EOS Rebel T6 1300 D camera) and performed by a certified canine rehabilitation practitioner (CCRP) examiner and instructor (Â.M.), who was blinded to the randomization and protocol implemented. The randomization process was completed by a CCRP student (D.G.) who implemented the protocol and worked with the dogs with the remaining rehabilitation team, one other CCRP (A.O.) and one other CCRP student (C.C.). One-third blinded CCRP (A.C.) was responsible for the evaluation of all movies (in regular and slow motion), considering an interobserver disagreement <20%.
The neurorehabilitation consultation took place in a controlled and calm environment, using a 12-cm Halsted mosquito forceps and an 18-cm Taylor hammer, including gait assessment (6-meter walk) and OFS classification; mental state; posture; palpation of the spine; postural reactions; peripheral spinal reflexes (patellar, withdrawal, cranial-tibial, cross-extensor, Babinsky, and cutaneous trunci reflexes); superficial and deep perception of pain; muscle tonus; and flexion/extension range of motion of all joints to assess muscle rigidity and/or spasticity.
Dogs of both groups presented with OFS 6 or OFS 8, clonic patellar and cranial-tibial reflexes, decreased withdrawal reflex in some cases but in others positive for cross-extensor reflex, positive cutaneous trunci reflex until L5, and positive pain perception. All presented hypertonia of the hindlimbs extensor muscle group and hypotonia of the hamstrings muscles, showing also some degree of muscle weakness and atrophy. The representative study algorithm is described in the Supplementary material.
2.2.1. INSCP
The INSCP group included dogs that were under a hospitalization regimen for 2 months and performed the following training (Table 2).
2.2.1.1. Locomotor training
The locomotor training implemented was based on fast step-cycle repetitions, always applied in a quiet environment, and ideally with musical stimulation (66, 67). Due to the presentation of clonic reflexes, bicycle movements were only necessary to modulate the rhythm of the step cycle, rarely requiring tail and perineal stimulation.
In these cyclic movements, stretching of the hindlimbs should be avoided, but with vigorous cutaneous afferent receptors stimulation on the treadmill surface (68). Early implementation of the quadrupedal training is intended for complete stimulation.
Land treadmill training was initiated with 0.9 km/h aiming to achieve 2.8 km/h for 10 min until a maximum of 60 min, initially with 5 repetitions a day in the first week, until 2 repetitions in the last week (Figure 1A). Underwater treadmill training was initiated at admission or the following day, always with water temperature nearly 26°C, beginning with 5 min until reaching 1 h, 5 days/week, and speeds of 1 km/h until 3.5 km/h (Figure 1B), with care for signs of overtraining (67) (Table 2).
Kinesiotherapy exercises were also included in the protocol, such as walking up/down stairs and ramps; on different floor surfaces; and cavaletti rails. These circuits were done 2–5 times/day, 4–5 days/week for 5–10 min.
2.2.1.2. Electrical stimulation
Functional electrical stimulation consisted of nerve stimulation with one electrode applied on L7-S1 anatomical region and the other electrode on the hamstring's muscle motor point. Parameters were 40–60 Hz, 10–36 mA, pulse duration 1:4, ramp up 4 s, plateau 8 s, and ramp down 2 s. These were done 2–3 times/day, 5 days/week, according to neurological evolution.
2.2.1.3. Mesenchymal stem cells preparation
The preparation of the cell-based therapies used in this clinical study followed an adaptation of the protocol patented by the University of Porto (WO2019175773—Compositions for use in the treatment of musculoskeletal conditions and methods for producing the same leveraging the synergistic activity of two different types of mesenchymal stromal/stem cells (Regenera®), PCT/IB2019/052006) (69). Previously harvested, isolated, and characterized (data not yet published) canine synovial membrane MSCs (cSM-MSCs) were used in this study. All SM-MSCs used in this study were obtained from the synovial membrane of a healthy and young donor dog and collected using arthroscopy. Before the harvest, the owners signed an informed consent, and the animal underwent a complete medical and orthopedic evaluation, as well as a screening for infectious and contagious diseases. After harvesting the synovial membrane, cell isolation was performed as previously described (70). The applied therapeutic combination consisted of the administration of allogeneic cSM-MSCs suspended in autologous serum. Total blood was previously collected from the animals to be treated, in dry blood collection tubes. After coagulation, the tubes were centrifuged at 2,300 rpm for 10 min and their supernatant (serum) was isolated and collected. Then, the serum was inactivated by immersion in a water bath at 56°C for 20 min followed by cooling on ice. Finally, the serum was centrifuged and filtered using a 0.22-μm syringe filter and stored at −20°C until further use. For each administration, ~5 × 106 cryopreserved low passage (P2) allogeneic cSM-MSCs were thawed in a 37°C water bath. After thawing, the cell content was transferred to a sterile tube and diluted in sterile and previously filtered DPBS. The mixture was centrifuged at 1,600 rpm for 10 min. After centrifugation, the supernatant was eliminated with a single movement, and the cell pellet was resuspended in ~2 ml of autologous serum previously thawed in a 37°C water bath. Cell counting and viability were determined through the Trypan Blue exclusion dye assay (InvitrogenTM) using an automatic counter (Countess II FL Automated Cell Counter, Thermo Fisher Scientific®). The cell number was then adjusted to 5 × 106 cells/ml. Then, 2 ml of the solution of cSM-MSCs suspended in autologous serum was transferred to a perforable capped vial and preserved on ice until the time of administration.
2.2.1.4. Mesenchymal stem cells transplantation
The stem cells transplantation was performed on day 30 (T1) by the head of the neurology department of the Veterinary Medicine Faculty—Lisbon University (A.F.) and his team, as follows: vascular access and fluid therapy during the procedure; induction with propofol (2 mg/kg); endotracheal intubation and anesthetic maintenance with isoflurane; trichotomy of the dorsal cervical region and asepsis; intrathecal transplantation based on the landmarks of the occipital prominence and the spinous process of C2, with a 22-gauge pencil-point needle inserted cranially to the atlas wings edge, penetrating the subarachnoid space; extraction of 1 ml of CSF for a sterile tube; administration of 1 ml of stem cells; and vertical positioning of the dog for 10 min.
2.2.2. Ambulatory rehabilitation protocol
The ambulatory rehabilitation protocol group was under an ambulatory regimen, 4–5 times a week. This protocol was based on locomotor training and electrical stimulation with few alterations (Table 2). Moreover, there was no MSCs administration in these dogs.
2.2.2.1. Locomotor training
The main difference in locomotor training was the frequency. Dogs of the ARP group only performed two repetitions for 15–30 min of land treadmill training for the session. Regarding the underwater treadmill, it was performed once for 10–40 min. Both training had speeds of 0.8–1.8 km/h, according to each dog's tolerance, and sessions were done 4–5 days/week.
2.2.2.2. Electrical stimulation
As for the functional electrical stimulation, the protocol was the same for both groups; however, the ARP group only had one repetition each session, 4–5 days/week.
2.3. Outcomes and monitorization
Dogs of both groups were admitted (T0) and evaluated according to their neurological status and classified with the OFS. The INSCP dogs were hospitalized in the rehabilitation center and after the first month of intensive rehabilitation (T1), they were subjected to stem cell transplantation at day 30 (T1), followed by the second month of rehabilitation (T2) and medical release at the end. The ARP dogs were under ambulatory sessions of rehabilitation during the same 2 months.
Dogs of both groups were evaluated at the end of day 30 (T1) and day 60 (T2). Follow-ups were performed and recorded 1 month after medical release (1FU) and the last follow-up was according to each dog, as presented in Figure 2.
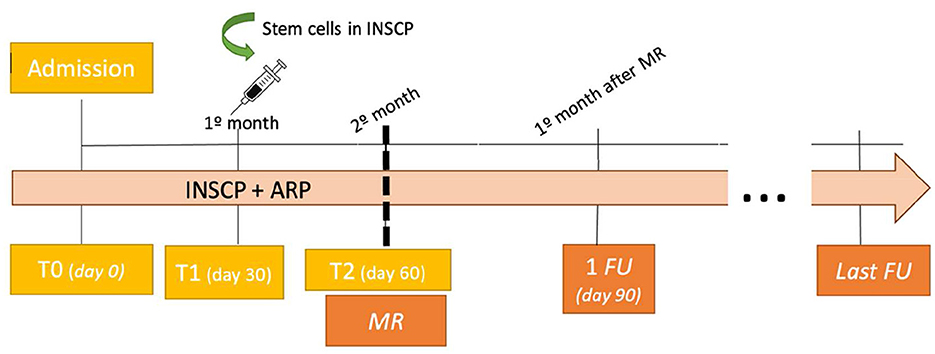
Figure 2. Outcomes and monitorization algorithm (n = 13). INSCP, intensive neurorehabilitation stem cell protocol; ARP, ambulatory rehabilitation protocol; MR, medical release; FU, follow-up.
2.4. Statistical analysis
Records were documented using Microsoft Office Excel 365® (Microsoft Corporation, Redmond, WA, USA) and processed in IBM SPSS Statistics 25® (International Business Machines Corporation, Armonk, NY, USA) software. Shapiro–Wilk normality test, arithmetic means, minimum, maximum, standard deviation (SD), and standard error of the mean (SEM) were recorded for continuous variables age and weight. Descriptive statistics with frequency analysis was performed for all categorical nominal variables. Chi-square tests were also performed to verify relevant analogies proven by a p-value of <0.05. One sample t-test was used for comparison with previously published studies. The estimated marginal means for comparison at each time point regarding the OFS scores and survival time were performed using Analysis of Variance (One-Way ANOVA) for repeated measures and the post-Tukey HSD test. In addition, the Kaplan–Meier survival analysis was performed.
3. Results
In this prospective controlled blinded cohort clinical study, from the 13 homozygotic DM dogs, the binominal qualitative variable sex presented 53.8% (7/13) of female and 46.2% (6/13) of male dogs. For the binominal variable breed, it was observed that 15.4% (2/13) were mixed-breed dogs and 84.6% (11/13) were pure-breed dogs, including German Shepherds (n = 7) as the most prevalent, followed by the Collie (n = 1), Alaskan Malamute (n = 1), Weimaraner (n = 1), and Setter (n = 1).
Descriptive analysis of the continuous quantitative variables for age and weight is reported in the Supplementary material, with a mean of 9.69 years and 30.54 kg, and a verified normal distribution by the Shapiro–Wilk Normality Test (n < 50) for age (p = 0.684) and weight (p = 0.233).
Clinical occurrences were absent in 61.5% (8/13) and present in 38.5% (5/13), of which three belonged to the study group and two to the control group.
The OFS outcomes were documented for both INSCP and ARP groups, at each time point (T0, T1, T2, 1FU, and Last FU), and ambulation was considered when OFS ≥ 11, as shown in Figure 3. Regarding the OFS classification, inter-observer disagreement was 11%.
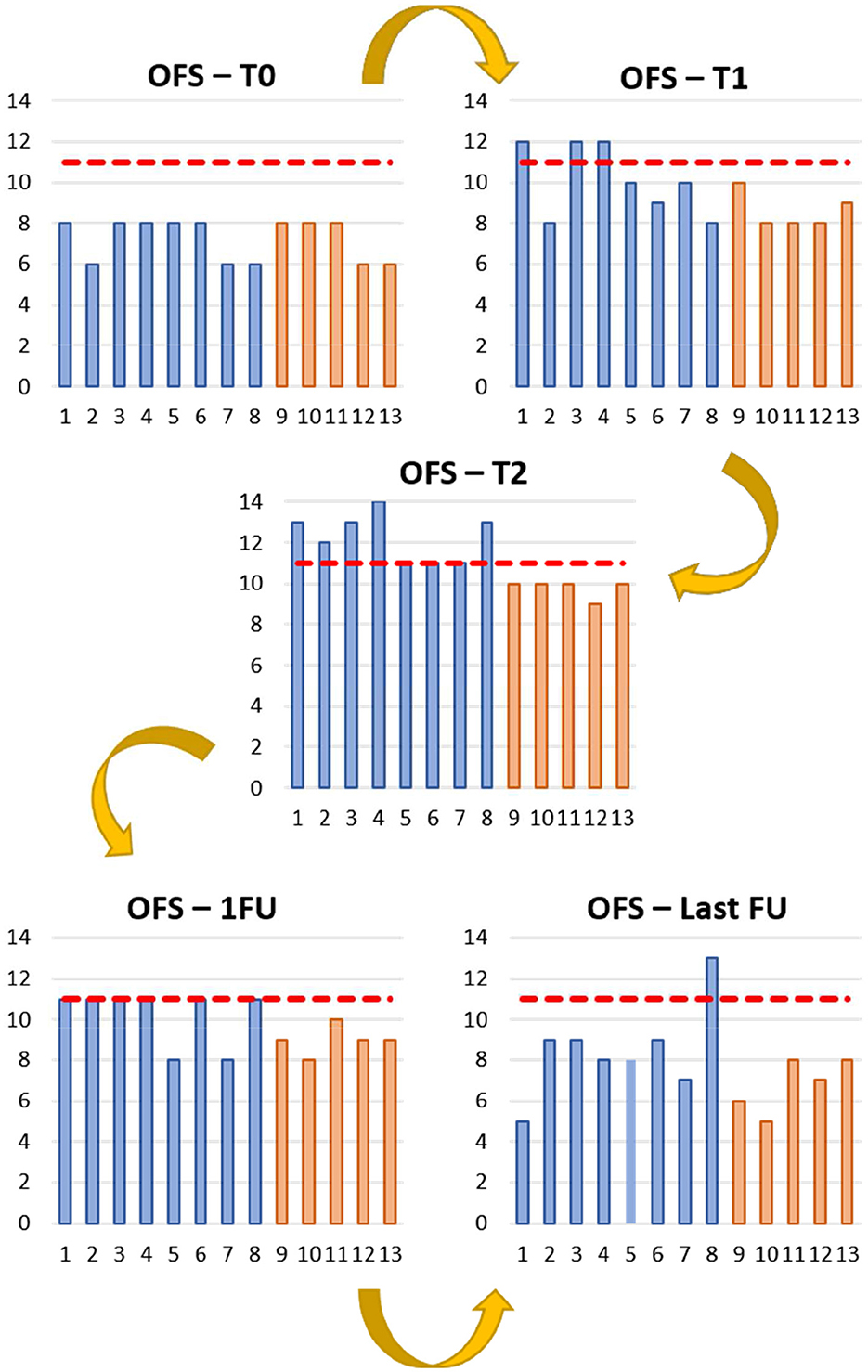
Figure 3. Open field score (OFS) reports from the study (blue) and control (orange) Groups during the different time points. T0 (day 0); T1 (day 30); T2 (day 60); 1FU (day 90); Last FU (last follow-up). Y-axis: OFS; X-axis: Dogs 1 to 13; — OFS 11.
In the present study, a total mean survival time of 375 days was obtained, with the INSCP group presenting a mean survival time of 438 days compared to 274 days for the ARP group, as shown in Table 3 and Figure 4A. The minimum days of survival time for each group was 91 days, although the INSCP group presented a maximum of 791 days compared with 396 days for the ARP group. Comparing both groups according to survival time, differences were also demonstrated by the survival analysis (Kaplan–Meier), as shown in Figure 4B.
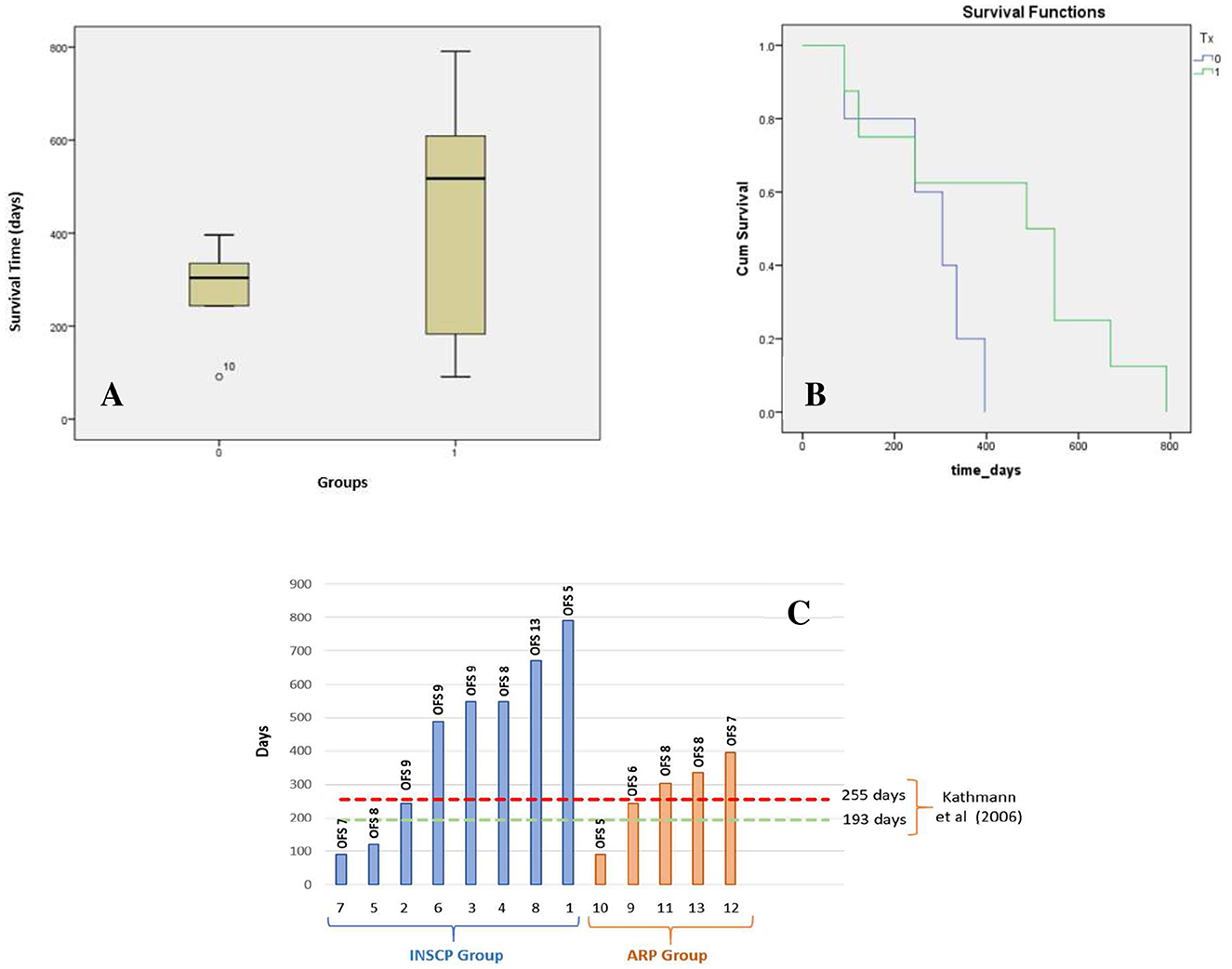
Figure 4. Study's population survival time. (A) Survival time (days) boxplot for INSCP (1) and ARP (0) groups; X-axis: groups (INSCP and ARP); Y-axis: survival time (days). (B) Kaplan–Meier survival analysis; y-axis: cumulative (Cum) survival; X-axis: survival time (days) from INSCP group (green) and ARP group (blue). (C) Survival time of the INSCP and ARP groups with open field score (OFS) for each dog on the last follow-up and comparison with Kathmann et al. (6); Y-axis: days; X-axis: dogs from INSCP group (blue) and ARP group (orange).
By the Chi-square tests, age [ = 0.008, p = 0.928], weight [ = 1.593, p = 0.207], and sex [ = 0.124, p = 0.725] did not show any interference in the survival time.
Kathmann et al. (6) demonstrated a mean survival time of 255 days for nine dogs and 130 days for six dogs, considering intensive and moderate physiotherapy, respectively. The present study reported survival time (days) for each dog of both groups and the OFS results at the last follow-up. This comparison is presented in Figure 4C.
When comparing both intensive and moderate physiotherapy of Kathmann et al. (6) (mean survival time 193 days) with the present study (n = 13), significance in the one sample t-test [t(12) = 2.932, p = 0.013] was shown. Although, when considering the INSCP group (n = 8) with the intensive physiotherapy group (n = 9) from Kathmann et al. (6), with a mean survival time of 255 days, no significance was observed [t(7) = 2.009, p = 0.085].
Considering the OFS of the INSCP group and ARP group, a significance [F(1,64) = 7.490, p = 0.008] between them by the One-Way ANOVA was detected, as well as a significance [t(63) = −2.737, p = 0.008] by the independent sample t-test. That finding could also be verified in the OFS estimated marginal means evolution chart (Figure 5).
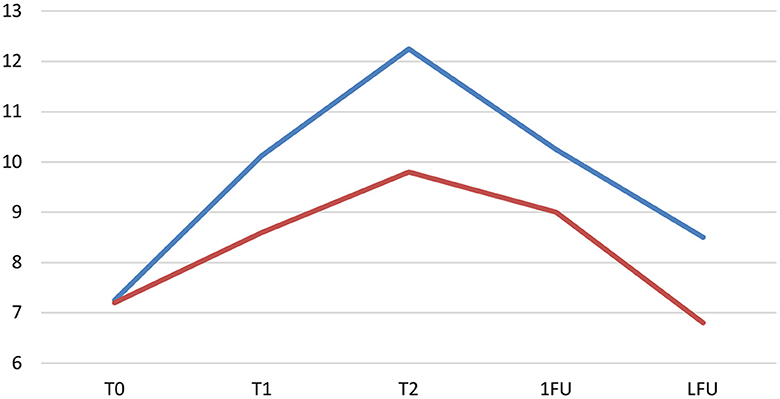
Figure 5. Evolution of the open field score (OFS)—estimated marginal means in both the INSCP group (blue) and ARP group (orange). Y-axis: OFS; X-axis: T0 (day 0); T1 (day30); T2(day 60); 1FU (day 90); LFU, last follow-up.
For the INSCP group, regarding the OFS evolution for each time point, a significant difference [F(4,64) = 14.287, p ≤ 0.001] was reported. The post-Tukey HSD test was performed between time points and showed that from T0 until the first follow-up, significances were always demonstrated (p = 0.003 with T1; p ≤ 0.001 with T2 and p = 0.001 with 1FU). Considering T1 until T2 (1 month after stem cell transplantation), results showed significance (p = 0.039), and, also, from T2 until the last follow-up of each dog (p ≤ 0.001), a consistent descending curve was observed, as shown in Figure 5.
4. Discussion
Distribution in regard to sex was higher for female dogs with 53.8% (7/13), agreeing with Coates et al. (1) who reported a total of 21 dogs with five male and 16 female dogs. Also, a similar prevalence was seen in a study by March et al. (15) with 18 dogs, four male and 14 female dogs. However, many authors referred to the absence of sexual predisposition in DM (3, 8).
As for breed predisposition, which is already stated in the literature (3), the German shepherd had the higher prevalence with 53.8% (7/13), which agrees with most research on DM (9, 12, 71–73). Although the histological diagnosis is described as a mere 0.19%, this percentage increases drastically to 2.01% in the German shepherd (1). In 2018, Donner et al. (74) studied the frequency and distribution of genetic diseases in dogs of mixed and pure breeds, pointing to DM as the number one of the rankings. However, it cannot be ignored the complex etiology of this disease, such as the immunologic (75), metabolic, nutritional (76, 77), oxidative stress (1), and excitotoxic effects (78).
The average age of onset of clinical signs was 9.69 years old, similar to those reported in the literature (3, 7). In contrast, mean weight had high variability between studies according to the most represented breeds. For example, a study in Pembroke Welsh Corgi reported an average of 11 kg (1, 17), much lower than the 30.54 kg of this study. Other studies with larger breeds presented mean weights >25 kg, such as Polizopolou et al. (31) and Johnston et al. (72).
The 13 dogs that were randomized for the INSCP group and ARP group according to the owner's decision of treatment presented a normal distribution by the Shapiro–Wilk Normality Test for age (p = 0.684) and weight (p = 0.233) and were compared in the study. Of the total of these dogs, and in both groups, nearly 60% did not present any type of clinical occurrences, probably due to the inclusion criteria (non-ambulatory paraparesis OFS 6 or 8).
Over the evaluation times (T0, T1, and T2) and first follow-up, an increase of OFS ≥11 was observed in most dogs of the INSCP group, indicating ambulation (79) and reported for each time point with significance (p ≤ 0.001). This was also supported by the post-Hukey test, with significances always present between T0 and 1FU (p = 0.003 with T1; p ≤ 0.001 with T2 and p = 0.001 with 1FU). Although, all dogs in both groups appeared to have an improvement regarding their sensorimotor status up to the first follow-up (1FU).
This increase in neurological status between T0 and T1 was mainly due to the association between locomotor training with electrical stimulation modalities, as expected and already mentioned by Kathmann et al. (80) and Miller et al. (81). These are neurorehabilitation concepts (82), which allow neural control of movement after changing sensory inputs (83) by the work of intrinsic ability to generate rhythmic movements (84).
The locomotor training helps in relearning stepping with a coordinated and modulated ambulation pattern (57, 67) based on a dynamic interaction between afferent inputs for all functional receptors (e.g., proprioceptive and biomechanical) (85, 86). This can be applied through bodyweight-supported treadmill training (BWSTT), which helps reduce spasticity by replacing abnormal hyperexcitable sensory firing with functional signaling, decreasing muscle spasm/contraction (87, 88), and improving coordination and ambulation (89), essential for affected DM dogs with UMN signs, for example, clonic reflexes.
One of the locomotor training strategies resides in the stimulation of descending propriospinal neurons essential to activate neural networks leading to stepping recovery (90, 91).
Furthermore, FES stimulates the unnatural recruitment of muscle fibers by engaging large-diameter motor neurons that have fast conduction velocity fibers, instead of recruiting small-diameter motor neurons, which are slower and fatigue-resistant (92). This modality has been reported to modulate neural circuitry (93, 94) and allows repetitive cycles of inputs/outputs, suppressing excessive afferent synaptogenesis and improving remaining neural circuits (95).
DM dogs present changes in myofibers and connective tissues that can alter the electrical properties, with progressive decrease in myofiber size, and increase in fat and connective tissue that may increase resistance (96). In the early stage of DM, dogs are characterized by disuse atrophy (3, 97), but in late DM, lower motor neuron signs begin, such as neurogenic atrophy. This is always with a caudodistal location associated with atrophy secondary to distal axonopathy (25, 96), with myelin profiles replaced by large areas of astrogliosis (9, 13, 72, 98).
In Figures 3, 5, the higher OFS results were from T1 to T2 mainly in the INSCP group, with a marked significance between groups (p = 0.039). Thus, after intrathecal transplantation (IT) of stem cells, which results in indirect cell distribution through the central nervous system (CNS) by the CSF (99). This technique intends to avoid the “first-pass” pulmonary effect and cell entrapment, limiting their therapeutic effect (100, 101). In addition, IT avoids the blood-brain barrier, allowing to focus the cells' trophic effects directly on the CNS (99, 102, 103).
The MSCs applied were synovial membrane-derived mesenchymal cells. MSCs can promote cell survival and tissue repair by increasing paracrine secretion of neurotrophic and angiogenic factors (104–106), such as brain-derived neurotrophic factor (BDNF), ciliary neurotrophic factor (CNTF) (107, 108), neurotrophin-1 (NT-1), neurotrophin-3 (NT-3), nerve growth factor (NGF), fibroblast growth factor (FGF), and glial cell-derived neurotrophic factor (GDNF) (109–114). All these support neurogenesis, axonal growth, re-myelinization, and cell metabolism (114–119), contributing also to the ability to recruit oligodendrocytes precursors (41). Recently, the therapeutic effects and also the immunomodulatory potential of SM-MSCs were demonstrated after application in dogs in Veterinary Medicine and after a preliminary characterization of its secretome (70).
In this prospective controlled blinded cohort clinical study, the mean survival time was 375 days in the total population, with the INSCP group having 438 days compared to the ARP group with 274 days. In the INSCP group, there was a maximum survival of 791 days (Table 3), which can be observed in the survival time boxplot (Figure 4A) and in the Kaplan–Meier survival analysis (Figure 4B).
Thus, the main difference may be linked to the MSCs transplantation in the INSCP group, in addition to intensive neurorehabilitation. MSCs potentiate aquaporin 1 and CXCR4 expression, two membrane proteins involved in cell migration, which help in the “homing” mechanisms (114), potentiated by the vertical position of the dog for 10 min that was included after this stem cells transplantation technique.
As previously explained and considering the study design, the selection of both groups was according to the owners' treatment decision. Although there was no clear evidence of the sole role of the MSCs given the differences of both protocols and even if the intensive neurorehabilitation demonstrated to have always a slight improvement compared to the ARP, an evident positive progression was observed after transplantation (T1), with all dogs from the INSCP achieving ambulation in this period (Figure 3). It is the authors' opinion that these results were not only due to the same intensive training applied until discharge, suggesting that it was also the combination of the neurorehabilitation intensive protocol with the MSCs transplantation that allowed this evolution.
DM implies Wallerian degeneration that causes fragmentation of damaged axons, generating debris and extracellular deposition of myelin-related molecules with chondroitin sulfate proteoglycans inhibiting neural regeneration and neuroplasticity in the long term. To delay this degenerative progression of the disease, the role of GDNF is reported (120), possibly contributing to the increase of dendrites length, number of dendrites, or a combination of both. Thus, aiming to achieve long-term potentiation due to reactivable remaining tissue (95).
Comparison of the total population (n = 13) with previous literature (6) considering dogs submitted to moderate or intensive physiotherapy (n = 15), by the one-sample t-test, revealed a significant difference (p = 0.013), as can be seen in Figure 4C with only three dogs (two from the INSCP and one from the ARP) achieving a survival rate lower than the 193 days reported by Kathmann et al. (6).
The mean survival time is compared between the mentioned study's intensive physiotherapy group (255 days) and our INSCP group (438 days). Although an increase of nearly 70% was visible, there was no significance obtained. Therefore, the authors believe that intensive rehabilitation is the minimum necessary that these dogs need to increase their survival time as it has already been proven, but stem cell transplantation may delay the progression and increase the survival time. However, there is not enough data, and research must continue.
When comparing the OFS results between the INSCP and ARP groups (one-way ANOVA and independent-samples t-test), there was also a clear significant difference. Figure 5 demonstrates this disparity in relation to the estimated marginal means at each moment of the study. In the same figure, there is a significant decrease from medical release to the last follow-up, justified by the degenerative and progressive nature of the disease, where glial cells play a critical role in the pathogenesis of this type of neurodegenerative diseases, such as ALS (121–123). Few studies also report the importance of increased glial cells and inflammatory molecules, partially responsible for the disease progression (15, 124, 125).
In the near future, it would be of main interest to have a quick and feasible test to assess the phosphorylated neurofilament heavy subunit (pNF-H) protein, a major structural component of large myelinated axons, which could be useful to measure axonal damage (23, 126–128).
The major cause of death was euthanasia due to the progression of DM with 69.2% (9/13). Euthanasia was perfromed after the last follow-up of each dog only if they presented the following clinical signs: thoracolumbar weakness; hindlimbs muscle mass atrophy; decreased hindlimbs spinal reflexes and cutaneous trunci reflex; decreased abdominal muscle tone; and decreased trunci balance.
The remaining 30.8% (4/13) died due to internal medical causes, all belonging to the INSCP group. From these, three dogs had cardiorenal syndrome secondary to dilated cardiomyopathy (with a survival time of 244, 487, and 670 days) and one dog was secondary to splenic neoplasia (with a survival time of 781 days). The one dog that died from dilated cardiomyopathy had a positive histopathologic exam for degenerative myelopathy (Necropsy Report N°40/20).
For neurorehabilitation in DM dogs, it is important to the notion of astrogliosis, which happens mainly in the dorsal horn region, that it gives rise to the dorsal spinocerebellar tracts (72). In this disease, the lesion will focus on the dorsal funiculus, including ascending and descending tracts within the dorsal portion of the lateral funiculus and the ascending pathways within the dorsal funiculus (3, 25). These may explain the constant loss of proprioception, leading to the need for locomotor training and association with kinesiotherapy exercises (e.g., alternating water levels, backward treadmill, and home exercises). In this study after T2, all dogs were only stimulated by home exercises 3 times/day, such as leach walking (15 min), cavaletti rails (2 min), stairs (2 min), ramps (2 min), and proprioceptive unbalance exercises (5 min).
Therefore, these results are in agreement with previous literature and suggest that the combination of AD-MSCs transplantation with intensive neurorehabilitation protocols may provide a functional improvement (129) with no long-term related side effects (130, 131). The same association was already applied in ALS patients (120, 131), resulting in a safe and feasible approach (132, 133).
It is possible to conclude that this INCSP may be an option for a long progression of DM dogs with quality of life and without suffering. In the author's opinion, its implementation should be as early as possible and not in the late stage of DM.
The limitations of this study were the small sample size and the randomization process based on owner consent. In addition, there was only one case with a definitive histological diagnosis, lack of biomarkers, and the absence of intensive neurorehabilitation after medical release.
Data availability statement
The raw data supporting the conclusions of this article will be made available by the authors, without undue reservation.
Ethics statement
The animal study was reviewed and approved by CEBEA-Comissão de Ética e Bem-estar Animal da Universidade Lusófona (Lusófona Veterinary Medicine Faculty, Lisbon, Portugal, No. 113-2021). Written informed consent was obtained from the tutors for the participation of their animals in this study.
Author contributions
All authors listed have made a substantial, direct, and intellectual contribution to the work and approved it for publication.
Funding
RA acknowledges the Centro de Estudos de Ciência Animal (CECA), Instituto de Ciências, Tecnologias e Agroambiente (ICETA), Porto University (UP), and Fundação para a Ciência e Tecnologia (FCT) for the funding and availability of all technical, structural, and human resources necessary for the development of this study. This study was supported through the project UIDB/00211/2020 funded by FCT/MCTES through national funds. The authors acknowledge FCT for funding the project 2022.04501.PTDC (Olfabionerve—Olfactory Mucosa Mesenchymal Stem Cells and Biomaterials Promoting Peripheral Nerve Regeneration) and the PhD Scholarships AS (SFRH/BD/146689/2019) BL (2021.05265.BD). PS acknowledges Instituto Politécnico de Leiria—Center for Rapid and Sustainable Product Development (CDRSP), University of Porto (UP), Centro de Estudos de Ciência Animal (CECA), and Instituto de Ciências, Tecnologias e Agroambiente (ICETA) for the funding (UIDB/04044/2020) and availability of all resources needed for this study. Amo acknowledges the project H2Cure—Desenvolvimento de formulações de géis e pensos de Mel, Goma Gelana e Ácido Hialurónico para tratamento de feridas—POCI-01-0247-FEDER-047032 for financial support. Aco acknowledges the project PRECOGFIL—Filamentos dentados pre-tensionados para correção transvaginal do prolapso do compartimento anterior e posterior—PTDC/EMD-EMD/2229/2020 for financial support.
Conflict of interest
The authors declare that the research was conducted in the absence of any commercial or financial relationships that could be construed as a potential conflict of interest.
Publisher's note
All claims expressed in this article are solely those of the authors and do not necessarily represent those of their affiliated organizations, or those of the publisher, the editors and the reviewers. Any product that may be evaluated in this article, or claim that may be made by its manufacturer, is not guaranteed or endorsed by the publisher.
Supplementary material
The Supplementary Material for this article can be found online at: https://www.frontiersin.org/articles/10.3389/fvets.2023.1192744/full#supplementary-material
References
1. Coates JR, March PA, Oglesbee M, Ruaux CG, Olby NJ, Berghaus RD, et al. Clinical characterization of a familial degenerative myelopathy in Pembroke Welsh Corgi Dogs. J Vet Intern Med. (2007) 21:1323–31. doi: 10.1111/j.1939-1676.2007.tb01955.x
2. Guzzi RF, Formenton MR, Colombia KP, Veras M, Guilherme Joaquim JF, Fantoni DT. Mielopatia Degenerativa Em Cães: Um Desafio Na Medicina Veterinária E Na Reabilitação Animal. Rev Unimar Ciênc. (2014) 23, 11–16.
3. Coates JR, Wininger FA. Canine degenerative myelopathy. Vet Clin N Am Small Anim Pract. (2010) 40:929–50. doi: 10.1016/j.cvsm.2010.05.001
4. Neeves J, Granger N. An update on degenerative myelopathy in dogs. Comp Anim. (2015) 20:408–12. doi: 10.12968/coan.2015.20.7.408
5. Awano T, Johnson GS, Wade CM, Katz ML, Johnson GC, Taylor JF, et al. Genome-wide association analysis reveals a SOD1 mutation in canine degenerative myelopathy that resembles amyotrophic lateral sclerosis. Proc Natl Acad Sci USA. (2009) 106:2794–9. doi: 10.1073/pnas.0812297106
6. Kathmann I, Cizinauskas S, Doherr MG, Steffen F, Jaggy A. Daily controlled physiotherapy increases survival time in dogs with suspected degenerative myelopathy. J Vet Intern Med. (2006) 20:927–32. doi: 10.1111/j.1939-1676.2006.tb01807.x
7. Zeng R, Coates JR, Johnson GC, Hansen L, Awano T, Kolicheski A, et al. Breed distribution of SOD1 alleles previously associated with canine degenerative myelopathy. J Vet Intern Med. (2014) 28:515–21. doi: 10.1111/jvim.12317
8. Dewey CW, da Costa RC. “Myelopathies: disorders of the spinal cord” in Dewey CW, da Costa Rc, editors. Pratical Guide to Canine and Feline Neurology, 3rd ed. Ames IA: Willey Blackwell (2016). p. 353–356.
9. Averill DR. Degenerative myelopathy in the aging German Shepherd dog: clinical and pathologic findings. J Am Vet Med Assoc. (1973) 162:1045–51.
10. Bichsel P, Vandevelde M, Lang J, Kull-Hächler S. Degenerative myelopathy in a family of Siberian husky dogs. J Am Vet Med Assoc. (1983) 183:998–1000.
11. Matthews NS, de Lahunta A. Degenerative myelopathy in an adult miniature poodle. J Am Vet Med Assoc. (1985) 186:1213–5.
12. Awano T, Johnson GS, Wade CM, Katz ML, Johnson GC, Taylor JF, et al. Degenerative myelopathy in Chesapeake Bay retrievers. J Vet Intern Med. (2009) 23:401–2.
13. Miller AD, Barber R, Porter BF, Peters RM, Kent M, Platt SR, et al. Brief communication: degenerative myelopathy in two boxer dogs. Vet Pathol. (2009) 46:684–7. doi: 10.1354/vp.08-VP-0270-M-BC
14. Zeiler GE, van der Zwan H, Oosthuizen MC. Genetic testing of canine degenerative myelopathy in the South African boxer dog population. J S Afr Vet Assoc. (2013) 84:1–5. doi: 10.4102/jsava.v84i1.1005
15. March PA, Coates JR, Abyad RJ, Williams DA, O'Brien DP, Olby NJ, et al. Degenerative myelopathy in 18 Pembroke Welsh Corgi dogs. Vet Pathol. (2009) 46:241–50. doi: 10.1354/vp.46-2-241
16. Ogawa M, Uchida K, Park E-S, Kamishina H, Sasaki J, Chang H-S, et al. Immunohistochemical observation of canine degenerative myelopathy in two Pembroke Welsh Corgi dogs. J Vet Med Sci. (2011) 73:1275–9. doi: 10.1292/jvms.11-0097
17. Ogawa M, Uchida K, Yamato O, Inaba M, Uddin MM, Nakayama H. Neuronal loss and decreased GLT-1 expression observed in the spinal cord of Pembroke Welsh Corgi dogs with canine degenerative myelopathy. Vet Pathol. (2014) 51:591–602. doi: 10.1177/0300985813495899
18. Mandrioli L, Gandini G, Gentilini F, Chiocchetti R, Turba ME, Avallone G, et al. Degenerative myelopathy in hovawart dogs: molecular characterization, pathological features and accumulation of mutant superoxide dismutase 1 protein. J Comp Pathol. (2021) 182:37–42. doi: 10.1016/j.jcpa.2020.11.006
19. Crisp MJ, Beckett J, Coates JR, Miller TM. Canine degenerative myelopathy: biochemical characterization of superoxide dismutase 1 in the first naturally occurring non-human amyotrophic lateral sclerosis model. Exp Neurol. (2013) 248:1–9. doi: 10.1016/j.expneurol.2013.05.009
20. Nagai M, Aoki M, Miyoshi I, Kato M, Pasinelli P, Kasai N, et al. Rats expressing human cytosolic copper-zinc superoxide dismutase transgenes with amyotrophic lateral sclerosis: associated mutations develop motor neuron disease. J Neurosci. (2001) 21:9246–54. doi: 10.1523/JNEUROSCI.21-23-09246.2001
21. Rothstein JD. Current hypotheses for the underlying biology of amyotrophic lateral sclerosis. Ann Neurol. (2009) 65:53–4. doi: 10.1002/ana.21543
22. Cooper JJ, Young BD. 4th JFG, Fosgate GT, and Levine JM. (2014). Comparison between non-contrast computed tomography and magnetic resonance imaging for detection and characterization of thoracolumbar myelopathy caused by intervertebral disk herniation in dogs. Vet Radiol. Ultrasound. 55, 182–189. doi: 10.1111/vru.12114
23. Toedebusch CM, Bachrach MD, Garcia VB, Johnson GC, Katz ML, Shaw G, et al. Cerebrospinal fluid levels of phosphorylated neurofilament heavy as a diagnostic marker of canine degenerative myelopathy. J Vet Intern Med. (2017) 31:513–20. doi: 10.1111/jvim.14659
24. Vu LT, Bowser, R. Fluid-based biomarkers for amyotrophic lateral sclerosis. Neurotherapeutics. (2017) 14:119–34. doi: 10.1007/s13311-016-0503-x
25. Nardone R, Höller Y, Taylor AC, Lochner P, Tezzon F, Golaszewski S, et al. Canine degenerative myelopathy: a model of human amyotrophic lateral sclerosis. Zoology. (2015) 119:64–73. doi: 10.1016/j.zool.2015.09.003
26. Valdmanis PN, Belzil VV, Lee J, Dion PA, St-Onge J, Hince P, et al. A mutation that creates a pseudoexon in SOD1 causes ALS. Ann Hum Genet. (2009) 73:652–7. doi: 10.1111/j.1469-1809.2009.00546.x
27. Kobatake Y, Nakata K, Sakai H, Sasaki J, Yamato O, Takashima S, et al. The long-term clinical course of canine degenerative myelopathy and therapeutic potential of curcumin. Vet Sci. (2021) 8:192. doi: 10.3390/vetsci8090192
28. Corral C. Canine degenerative myelopathy. Vet Nurs. (2020) 11:228–31. doi: 10.12968/vetn.2020.11.5.228
29. Clemmons RM. Degenerative myelopathy. Vet Clin N Am Small Anim Pract. (1992) 22:965–71. doi: 10.1016/S0195-5616(92)50087-0
30. Boothe DM. Drugs acting on coagulation and clotting. In:Boothe DM, , editor. Small Animal Clinical Pharmacology and Therapeutics. Philadelphia, PA: W. B. Saunders (2001). p. 107–24.
31. Polizopoulou ZS, Koutinas AF, Patsikas MN, Soubasis N. Evaluation of proposed therapeutic protocol in 12 dogs with tentative degenerative myelopathy. Acta Vet Hung. (2008) 56:1588–2705. doi: 10.1556/avet.56.2008.3.3
32. Kolios G, Moodley Y. Introduction to stem cells and regenerative medicine. Respiration 85. (2013) 3–10. doi: 10.1159/000345615
33. Ratajczak MZ, Ratajczak J, Kucia M. Very small embryonic-like stem cells (VSELs). Circ Res. (2019) 124:208–10. doi: 10.1161/CIRCRESAHA.118.314287
34. Mocchi M, Dotti S, Bue MD, Villa R, Bari E, Perteghella S, et al. Veterinary regenerative medicine for musculoskeletal disorders: can mesenchymal stem/stromal cells and their secretome be the new frontier? Cells. (2020) 9:1453. doi: 10.3390/cells9061453
35. Trivedi P, Hematti P. Derivation and immunological characterization of mesenchymal stromal cells from human embryonic stem cells. Exp Hematol. (2008) 36:350–9. doi: 10.1016/j.exphem.2007.10.007
36. Hackanson B, Waller CF. Long-term follow-up of patients with chronic myeloid leukemia having received autologous stem cell transplantation. Ann Hematol. (2011) 90:395–9. doi: 10.1007/s00277-010-1094-y
37. Chen C-T, Shih Y-RV, Kuo TK, Lee OK, Wei Y-H. Coordinated changes of mitochondrial biogenesis and antioxidant enzymes during osteogenic differentiation of human mesenchymal. Stem Cells. (2008) 26:960–8. doi: 10.1634/stemcells.2007-0509
38. Tzouvelekis A, Koliakos G, Ntolios P, Baira I, Bouros E, Oikonomou A, et al. Stem cell therapy for idiopathic pulmonary fibrosis: a protocol proposal. J Transl Med. (2011) 9:182. doi: 10.1186/1479-5876-9-182
39. Clerici M, Cassinotti A, Onida F, Trabattoni D, Annaloro C, Volpe AD, et al. Immunomodulatory effects of unselected haematopoietic stem cells autotransplantation in refractory Crohn's disease. Dig Liver Dis. (2011) 43:946–52. doi: 10.1016/j.dld.2011.07.021
40. Pouly J, Bruneval P, Mandet C, Proksch S, Peyrard S, Amrein C, et al. Cardiac Stem cells in the real world. J Thorac Cardiovasc Surg. (2008) 135:673–8. doi: 10.1016/j.jtcvs.2007.10.024
41. Uccelli A, Laroni A, Freedman M. Mesenchymal stem cells for the treatment of multiple sclerosis and other neurological diseases. Review. (2011) 10:649–56. doi: 10.1016/S1474-4422(11)70121-1
42. Filho MD, Ribeiro P, Oliveira LF, Paula D, Capuano V, Assunção T, et al. Therapy with mesenchymal stem cells in Parkinson disease. Neurologist. (2018) 23:141–7. doi: 10.1097/NRL.0000000000000188
43. Gögel S, Gubernator M, Minger S. Progress and prospects: stem cells and neurological diseases. Gene Ther. (2011) 18:1–6. doi: 10.1038/gt.2010.130
44. Muzes G, Sipos F. Mesenchymal stem cell-derived secretome: a potential therapeutic option for autoimmune and immune-mediated inflammatory diseases. Cells. (2022) 11:2300. doi: 10.3390/cells11152300
45. Moore SA, Granger N, Olby NJ. Targeting translational successes through CANSORT-SCI: using pet dogs to identify effective treatments for spinal cord injury. J Neurotrauma. (2017) 34:2007–18. doi: 10.1089/neu.2016.4745
46. Pereira AL, Bittencourt MKW, Barros MA, Malago R, Panattoni JFM, de Morais BP, et al. Subconjunctival use of allogeneic mesenchymal stem cells to treat chronic superficial keratitis in German shepherd dogs: Pilot study. Open Vet J. (2022) 12:744–53. doi: 10.5455/OVJ.2022.v12.i5.20
47. Najera J, Hao J. Recent advance in mesenchymal stem cells therapy for atopic dermatitis. J Cell Biochem124. (2023) 181–7. doi: 10.1002/jcb.30365
48. Olsen A, Johnson V, Webb T, Santangelo KS, Dow S, Duerr FM. Evaluation of intravenously delivered allogeneic mesenchymal stem cells for treatment of elbow osteoarthritis in dogs: a pilot study. Vet Comp Orthop Traumatol. (2019) 32:173–81. doi: 10.1055/s-0039-1678547
49. Brondeel C, Pauwelyn G, de Bakker E, Saunders J, Samoy Y, Spaas JH. Review: mesenchymal stem cell therapy in canine osteoarthritis research: “experientia docet” (experience will teach us). Front Vet Sci. (2021) 8:668881. doi: 10.3389/fvets.2021.668881
50. Olsson DC, Teixeira BL, Jeremias TD, Réus JC, Canto GD, Porporatti AL, et al. Administration of mesenchymal stem cells from adipose tissue at the hip joint of dogs with osteoarthritis: a systematic review. Res Vet Sci. (2021) 135:495–503. doi: 10.1016/j.rvsc.2020.11.014
51. Guercio A, Di Marco P, Casella S, Cannella V, Russotto L, Purpari G, et al. Production of canine mesenchymal stem cells from adipose tissue and their application in dogs with chronic osteoarthritis of the humeroradial joints. Cell Biol Int. (2012) 36:189–94. doi: 10.1042/CBI20110304
52. Fontanilla CV, Gu H, Liu Q, Zhu TZ, Zhou C, Johnstone BH, et al. Corrigendum: adipose-derived stem cell conditioned media extends survival time of a mouse model of amyotrophic lateral sclerosis. Sci Rep. (2016) 6:20747. doi: 10.1038/srep20747
53. Chudickova M, Vackova I, Urdzikova LM, Jancova P, Kekulova K, Rehorova M, et al. The effect of Wharton jelly-derived mesenchymal stromal cells and their conditioned media in the treatment of a rat spinal cord injury. Int J Mol Sci. (2019) 20:4516. doi: 10.3390/ijms20184516
54. Andrzejewska A, Lukomska B, Janowski M. Mesenchymal stem cells: from roots to boost. Stem Cells. (2019) 37:855–64. doi: 10.1002/stem.3016
55. Mcgonagle L, Blythe L, Levine D. History of canine physical rehabilitation. In:Millis DL, Levine D, , editors. D Canine Rehabilitation and Physical Therapy, 2nd ed. Philadelphia, PA: Elsevier Saunders (2014). p. 1–7.
56. Hamid S, Hayek R. Role of electrical stimulation for rehabilitation and regeneration after spinal cord injury: an overview. Eur Spin J. (2008) 17:1256–69. doi: 10.1007/s00586-008-0729-3
57. Martins Â, Gouveia D, Cardoso A, Carvalho C, Coelho T, Silva C, et al. A Controlled clinical study of intensive neurorehabilitation in post- surgical dogs with severe acute intervertebral disc extrusion. Animals. (2021) 11:3034. doi: 10.3390/ani11113034
58. Holsheimer J. Computer modelling of spinal cord stimulation and its contribution to therapeutic efficacy. Spin Cord. (1998) 36:531–40. doi: 10.1038/sj.sc.3100717
59. Bockstahler B, Levine D. Electrical stimulation. In:Millis DL, Levine D, , editors. Canine Rehabilitation and Physical Therapy, 2nd ed. Philadelphia, PA: Elsevier Saunders (2014). p. 342–58.
60. Hicks AL. Locomotor training in people with spinal cord injury: is this exercise? Spinal Cord. (2020) 59:1–8. doi: 10.1038/s41393-020-0502-y
61. Martinez M, Delivet-Mongrain H, Rossignol S. Treadmill training promotes spinal changes leading to locomotor recovery after partial spinal cord injury in cats. J Neurophysiol. (2013) 109:2909–22. doi: 10.1152/jn.01044.2012
62. Rossignol S, Martinez M, Escalona M, Kundu A, Delivet-Mongrain H, Alluin O, et al. The “beneficial” effects of locomotor training after various types of spinal lesions in cats and rats. Prog Brain Res. (2015) 218:173–98. doi: 10.1016/bs.pbr.2014.12.009
63. Marsolais GS, Dvorak G, Conzezmius MG. Effects of postoperative rehabilitation on limb function after cranial cruciate ligament repair in dogs. J Am Vet Med Assoc. (2002) 220:1325–1133. doi: 10.2460/javma.2002.220.1325
64. Monk ML, Preston CA, McGowan CM. Effects of early intensive postoperative physiotherapy on limb function after tibial plateau levelling osteotomy in dogs with deficiency of the cranial cruciate ligament. Am J Vet Res. (2006) 67:529–36. doi: 10.2460/ajvr.67.3.529
65. Olby NJ, De Risio L, Muñana KR, Wosar MA, Skeen TM, Sharp NJ, et al. Development of a functional scoring system in dogs with acute spinal cord injuries. Am J Vet Res. (2001) 62:1624–8. doi: 10.2460/ajvr.2001.62.1624
66. Lewis MJ, Bowditch J, Laflen B, Perry N, Yoquelet R, Thomovsky SA. Pilot study on feasibility of sensory-enhanced rehabilitation in canine spinal cord injury. Front Vet Sci. (2022) 9:921471. doi: 10.3389/fvets.2022.921471
67. Gouveia D, Cardoso A, Carvalho C, Almeida A, Gamboa Ó, Ferreira A, et al. Approach to small animal neurorehabilitation by locomotor training: an update. Animals 12. (2022) 3582. doi: 10.3390/ani12243582
68. Gouveia D, Cardoso A, Carvalho C, Gonçalves AR, Gamboa Ó, Canejo-Teixeira R, et al. Influence of spinal shock on the neurorehabilitation of ANNPE dogs. Animals. (2022) 12:1557. doi: 10.3390/ani12121557
69. Santos AR, Pedrosa S, Branquinho M, Alvites R, Mauricio A, Camões S, et al. WO2019175773 – Compositions for Use in the Treatment of Musculoskeletal Conditions and Methods for Producing the Same Leveraging the Synesgistic Activity of Two Different Types of Mesenchymal Stromal/ Stem Cells (Regenera®). Universidade do Porto (PCT/IB2019/052006) (2019).
70. Leal Reis I, Lopes B, Sousa P, Sousa AC, Branquinho M, Caseiro AR, et al. Allogenic synovia-derived mesenchymal stem cells for treatment of equine tendinopathies and desmopathies—Proof of concept. Animals. (2023) 13:1312. doi: 10.3390/ani13081312
71. Braund KG, Vandevelde M. German Shepherd dog myelopathy: a morphologic and morphometric study. Am J Vet Res. (1978) 39:1309–15.
72. Johnston PE, Barrie JA, McCulloch MC, Anderson TJ, Griffiths IR. Central Nervous system pathology in 25 dogs with chronic degenerative radiculomyelopathy. Vet Rec. (2000) 146:629–33. doi: 10.1136/vr.146.22.629
73. Holder AL, Price JA, Adams JP, Volk HA, Catchpole B. A retrospective study of the prevalence of the canine degenerative myelopathy associated superoxide dismutase 1 mutation (SOD1:c.118G > A) in a referral population of German Shepherd dogs from the UK. Canine Genet Epidemiol. (2014) 1:10. doi: 10.1186/2052-6687-1-10
74. Donner J, Anderson H, Davison S, Hughes AM, Bouirmane J, Lindqvist J, et al. Frequency and distribution of 152 genetic disease variants in over 100,000 mixed breed and purebred dogs. PLoS Genet. (2018) 14:e1007361. doi: 10.1371/journal.pgen.1007361
75. Waxman FJ, Clemmons RM, Hinrichs DJ. Progressive myelopathy in older German shepherd dogs. I Depressed response to thymus-dependent mitogens. J Immunol. (1980) 124:1209–15. doi: 10.4049/jimmunol.124.3.1209
76. Johnston PE, Knox K, Gettinby G, Griffiths IR. Serum a-tocopherol concentrations in German shepherd dogs with chronic degenerative radiculomyelopathy. Vet Rec. (2001) 148:403–7. doi: 10.1136/vr.148.13.403
77. Fechner H, Johnston PE, Sharp NJ, Montague P, Griffiths IR, Wang X, et al. Molecular genetic and expression analysis of alpha-tocopherol transfer protein mRNA in German shepherd dogs with degenerative myelopathy. Berl Munch Tierarztl Wochenschr. (2003) 11:631–6.
78. Olby NJ, Sharp NJ, Muñana KR, Papich MG. Chronic and acute compressive spinal cord lesions in dogs are associated with increased lumbar CSF glutamate levels. J Vet Intern Med. (1999) 13:240. doi: 10.1089/neu.1999.16.1215
79. Lewis MJ, Jeffery ND, Olby NJ, Canrt-S C. I. Ambulation in dogs with absent pain perception after acute thoracolumbar spinal cord injury. Front Vet Sci. (2021) 7:560. doi: 10.3389/fvets.2020.00560
80. Kathmann I, Demierre S, Jaggy A. Rehabilitation methods in small animal neurology. Schweiz Arch Tierheilkd. (2001) 143:495–502.
81. Miller LA, Torraca D, Taboada L. Retrospective observational study and analysis of two different photobiomodulation therapy protocols combined with rehabilitation therapy as therapeutic interventions for canine degenerative myelopathy. Photobiomodul Photomed Laser Surg. (2020) 38:195–205. doi: 10.1089/photob.2019.4723
82. Lima R, Monteiro A, Salgado AJ, Monteiro S, Silva NA. Pathophysiology and therapeutic approaches for spinal cord injury. Int J Mol Sci. (2022) 23:13833. doi: 10.3390/ijms232213833
83. Sherrington CS. Flexion-reflex of the limb, crossed extension-reflex, and reflex stepping and standing. J Physiol. (1910) 40:28–121. doi: 10.1113/jphysiol.1910.sp001362
84. Brown TG, Sherrington CS. The intrinsic factors in the act of progression in the mammal. Proc R Soc Lond Ser B Contain Pap A Biol Character. (1911) 84:308–19. doi: 10.1098/rspb.1911.0077
85. Rossignol S, Frigon A. Recovery of locomotion after spinal cord injury: some facts and mechanisms. Annu Rev Neurosci. (2011) 34:413–40. doi: 10.1146/annurev-neuro-061010-113746
86. Bouyer LJG, Rossignol S. Contribution of cutaneous inputs from the hindpaw to the control of locomotion in intact cats. J Neurophysiol. (2003) 90:3625–39. doi: 10.1152/jn.00496.2003
87. Edgerton VR, Leon RD, Harkema SJ, Hodgson JA, London N, Reinkensmeyer DJ, et al. Retraining the injured spinal cord. J Physiol. (2001) 533:15–22. doi: 10.1111/j.1469-7793.2001.0015b.x
88. Norton JA, Gorassini MA. Changes in cortically related intermuscular coherence accompanying improvements in locomotor skills in incomplete spinal cord injury. J Neurophysiol. (2006) 95:2580–9. doi: 10.1152/jn.01289.2005
89. Smith AC, Knikou M. A review on locomotor training after spinal cord injury: reorganization of spinal neuronal circuits and recovery of motor function. Neural Plast. (2016) 1216258:1–20. doi: 10.1155/2016/1216258
90. Behrman AL, Harkema SJ. Locomotor training after human spinal cord injury: a series of case studies. Phys Ther. (2000) 80:688–700. doi: 10.1093/ptj/80.7.688
91. Shah PK, Garcia-Alias G, Choe J, Gad P, Gerasimenko Y, Tillakaratne N, et al. Use of quadrupedal step training to re-engage spinal interneuronal networks and improve locomotor function after spinal cord injury. Brain. (2013) 136:3362–77. doi: 10.1093/brain/awt265
92. Fouad K, Tetzlaff W. Rehabilitative training and plasticity following spinal cord injury. Exp Neurol. (2012) 235:91–9. doi: 10.1016/j.expneurol.2011.02.009
93. Kralj A, Bajd T, Turk R, Krajnik J, Benko H. Gait restoration in paraplegic patients: a feasibility demonstration using multichannel surface electrode FES. J Rehabil R D. (1983) 20:3–20.
94. Hendricks HT, IJzerman MJ, de Kroon JR, Groen FA, Zilvold G. Functional electrical stimulation by means of the ‘Ness Handmaster Orthosis' in chronic stroke patients: an exploratory study. Clin Rehabil. (2001) 15:217–20. doi: 10.1191/026921501672937235
95. Shinozaki M, Nagoshi N, Nakamura M, Okano H. Mechanisms of stem cell therapy in spinal cord injuries. Cells. (2021) 10:2676. doi: 10.3390/cells10102676
96. Kowal JB, Verga SA, Pandeya SR, Cochran RJ, Sabol JC, Rutkove SB, et al. Electrical impedance myography in dogs with degenerative myelopathy. Front Vet Sci. (2022) 9:874277. doi: 10.3389/fvets.2022.874277
97. Shelton GD, Johnson GC, O'Brien DP, Katz ML, Pesayco JP, Chang BJ, et al. Degenerative myelopathy associated with a missense mutation in the superoxide dismutase 1 (sod1) gene progresses to peripheral neuropathy in pembroke welsh corgis and boxers. J Neurol Sci. (2012) 318:55–64. doi: 10.1016/j.jns.2012.04.003
98. Griffiths IR, Duncan ID. Chronic degenerative radiculomyelopathy in the dog. J Small Anim Pract. (1975) 16:461–71. doi: 10.1111/j.1748-5827.1975.tb05773.x
99. Karussis D, Karageorgiou C, Vaknin-Dembinsky A, Gowda-Kurkalli B, Gomori JM, Kassis I, et al. Safety and immunological effects of mesenchymal stem cell transplantation in patients with multiple sclerosis and amyotrophic lateral sclerosis. Arch Neurol. (2010) 67:1187–94. doi: 10.1001/archneurol.2010.248
100. Fischer UM, Harting MT, Jimenez F, Monzon-Posadas WO, Xue H, Savitz SI, et al. Pulmonary passage is a major obstacle for intravenous stem cell delivery: the pulmonary first-pass effect. Stem Cell Dev. (2009) 18:683–92. doi: 10.1089/scd.2008.0253
101. Takahashi Y, Tsuji O, Kumagai G, Hara CM, Okano HJ, Miyawaki A, et al. Comparative study of methods for administering neural stem/progenitor cells to treat spinal cord injury in mice. Cell Trans. (2011) 20:727–39. doi: 10.3727/096368910X536554
102. Bakshi A, Hunter C, Swanger S, Lepore A, Fischer I. Minimally invasive delivery of stem cells for spinal cord injury: advantages of the lumbar puncture technique. J Neurosurg Spine. (2004) 1:330–7. doi: 10.3171/spi.2004.1.3.0330
103. Benavides FP, Pinto GB, Heckler MC, Hurtado DM, Teixeira LR, Monobe MM, et al. Intrathecal transplantation of autologous and allogeneic bone marrow-derived mesenchymal stem cell in dogs. Cell Transplant. (2021) 30:1–11. doi: 10.1177/09636897211034464
104. Paul G, Anisimov SV. The secretome of mesenchymal stem cells: potential implications for neuroregeneration. Biochimie. (2013) 95:2246–56. doi: 10.1016/j.biochi.2013.07.013
105. Villatoro AJ, Alcoholado C, Martín-Astorga MC, Fernández V, Cifuentes M, Becerra J. Comparative analysis and characterization of soluble factors and exosomes from cultured adipose tissue and bone marrow mesenchymal stem cells in canine species. Vet Immun Immunopathol. (2019) 208:6–15. doi: 10.1016/j.vetimm.2018.12.003
106. Murphy MB, Moncivais K, Caplan AI. Mesenchymal stem cells: environmentally responsive therapeutics for regenerative medicine. Exp Mol Med. (2013) 45:e54–e16. doi: 10.1038/emm.2013.94
107. Aras Y, Sabanci PA, Kabatas S, Duruksu G, Subasi C, Erguven M, et al. The Effects of adipose tissue-derived mesenchymal stem cell transplantation during the acute and subacute phases following spinal cord injury. Turk Neurosurg. (2016) 26:127–39. doi: 10.5137/1019-5149.JTN.15724-15.0
108. Huang L, Fu C, Xong F. Stem cell therapy for spinal cord injury. Cell Transplant. (2021) 30:1–16. doi: 10.1177/0963689721989266
109. Potapova IA, Gaudette GR, Brink PR, Robinson RB, Rosen MR, Cohen IS, et al. Mesenchymal stem cells support migration, extracellular matrix invasion, proliferation, and survival of endothelial cells in vitro. Stem Cells. (2007) 25:1761–8. doi: 10.1634/stemcells.2007-0022
110. Boido M, Piras A, Valsecchi V, Spigolon G, Mareschi K, Ferrero I, et al. Human mesenchymal stromal cell transplantation modulates neuroinflammatory milieu in a mouse model of amyotrophic lateral sclerosis. Cytotherapy. (2014) 16:1059–72. doi: 10.1016/j.jcyt.2014.02.003
111. Teixeira FG, Carvalho MM, Neves-Carvalho A, Panchalingam KM, Behie LA, Pinto L, et al. Secretome of mesenchymal progenitors from the umbilical cord acts as modulator of neural/glial proliferation and differentiation. Stem Cell Rev. (2015) 11:288–97. doi: 10.1007/s12015-014-9576-2
112. Vizoso FJ, Eiro N, Cid S, Schneider J, Perez-Fernandez R. Mesenchymal stem cell secretome: toward cell-free therapeutic strategies in regenerative medicine. Int J Mol Sci. (2017) 25:1852. doi: 10.3390/ijms18091852
113. Kolar MK, Itte VN, Kingham PJ, Novikov LN, Wiberg M, Kelk P. The neurotrophic effects of different human dental mesenchymal stem cells. Sci Rep. (2017) 7:1260. doi: 10.1038/s41598-017-12969-1
114. Cofano F, Boido M, Monticelli M, Zenga F, Ducati A, Vercelli A, et al. Mesenchymal stem cells for spinal cord injury: current options, limitations, and future of cell therapy. Int J Mol Sci. (2009) 20:2698. doi: 10.3390/ijms20112698
115. Lu P, Jones LL, Tuszynski MH. BDNF-expressing marrow stromal cells support extensive axonal growth at sites of spinal cord injury. Exp Neurol. (2005) 191:344–60. doi: 10.1016/j.expneurol.2004.09.018
116. Crigler L, Robey RC, Asawachaicharn A, Gaupp D, Phinney DG. Human mesenchymal stem cell subpopulations express a variety of neuro-regulatory molecules and promote neuronal cell survival and neuritogenesis. Exp. Neurol. (2006) 198:54–64. doi: 10.1016/j.expneurol.2005.10.029
117. Wright KT, Masri WE, Osman A, Roberts S, Chamberlain G, Ashton BA, et al. Bone marrow stromal cells stimulate neurite outgrowth over neural proteoglycans (CSPG), myelin associated glycoprotein and Nogo-A. Biochem Biophys Res Commun. (2007) 354:559–66. doi: 10.1016/j.bbrc.2007.01.013
118. Teixeira FG, Carvalho MM, Sousa N, Salgado AJ. Mesenchymal stem cells secretome: a new paradigm for central nervous system regeneration? Cell Mol Life Sci. (2013) 70:3871–82. doi: 10.1007/s00018-013-1290-8
119. Hofer HR, Tuan RS. Secreted trophic factors of mesenchymal stem cells support neurovascular and musculoskeletal therapies. Stem Cell Res Ther. (2016) 7:131. doi: 10.1186/s13287-016-0394-0
120. Deng L, Ruan Y, Chen C, Frye CC, Xiong W, Jin X, et al. Characterization of dendritic morphology and neurotransmitter phenotype of thoracic descending propriospinal neurons after complete spinal cord transection and GDNF treatment. Exp Neurol. (2016) 277:103–14. doi: 10.1016/j.expneurol.2015.12.018
121. Boillée S, Velde CV, Cleveland DW. ALS: a disease of motor neurons and their nonneuronal neighbors. Neuron. (2006) 52:39–59. doi: 10.1016/j.neuron.2006.09.018
122. Yamanaka K, Chun SJ, Boillee S, Fujimori-Tonou N, Yamashita H, Gutmann DH, et al. Astrocytes as determinants of disease progression in inherited amyotrophic lateral sclerosis. Nat Neurosci. (2008) 11:251–3. doi: 10.1038/nn2047
123. Naji A, Favier B, Deschaseaux F, Rouas-Freiss N, Eitoku M, Suganuma N. Mesenchymal stem/stromal cell function in modulating cell death. Stem Cell Res Ther. (2019) 10:56. doi: 10.1186/s13287-019-1158-4
124. Lovett MC, Coates JR, Shu Y, Oglesbee MJ, Fenner W, Moore SA. Quantitative assessment of hsp70, IL-1β and TNF-α in the spinal cord of dogs with E40K SOD1-associated degenerative myelopathy. Vet J. (2014) 2:312–7. doi: 10.1016/j.tvjl.2014.03.003
125. Hashimoto K, Kobatake Y, Asahina R, Yamato O, Islam MS, Sakai H, et al. Up-regulated inflammatory signatures of the spinal cord in canine degenerative myelopathy. Res Vet Sci. (2020) 135:442–9. doi: 10.1016/j.rvsc.2020.11.001
126. Shaw G, Yang C, Ellis R, et al. Hyperphosphorylated neurofilament NF-H is a serum biomarker of axonal injury. Biochem Biophys Res Commun. (2005) 336:1268–77. doi: 10.1016/j.bbrc.2005.08.252
127. Petzold A, Shaw G. Comparison of two ELISA methods for measuring levels of the phosphorylated neurofilament heavy chain. J Immunol Methods. (2007) 319:34–40. doi: 10.1016/j.jim.2006.09.021
128. Anderson KJ, Scheff SW, Miller KM, Roberts KN, Gilmer LK, Yang C, et al. The phosphorylated axonal form of the neurofilament subunit NF-H (pNF-H) as a blood biomarker of traumatic brain injury. J Neurotrauma. (2008) 25:1079–85. doi: 10.1089/neu.2007.0488
129. Ma Y-H, Liang Q-Y, Ding Y, Han I, Zeng X. Multimodal repair of spinal cord injury with mesenchymal stem cells. Neurospine. (2022) 19:616–29. doi: 10.14245/ns.2244272.136
130. El-Kheir WA, Gabr H, Awad MR, Ghannam O, Barakat Y, Farghali HAMA, et al. Autologous bone marrow-derived cell therapy combined with physical therapy induces functional improvement in chronic spinal cord injury patients. Cell Transplant. (2014) 23:729–45. doi: 10.3727/096368913X664540
131. Li M, Chen H, Zhu M. Mesenchymal stem cells for regenerative medicine in central nervous system. Front Neurosci. (2022) 16:1068114. doi: 10.3389/fnins.2022.1068114
132. Syková E, Rychmach P, Drahorádová I, Konrádová Š, RuŽičková K, Voríšek I, et al. Transplantation of mesenchymal stromal cells in patients with amyotrophic lateral sclerosis: results of phase I/IIa clinical trial. Cell Transplant. (2017) 26:647–58. doi: 10.3727/096368916X693716
Keywords: mesenchymal stem cells, intensive neurorehabilitation, dogs, degenerative myelopathy, locomotor training, electrical stimulation
Citation: Gouveia D, Correia J, Cardoso A, Carvalho C, Oliveira AC, Almeida A, Gamboa Ó, Ribeiro L, Branquinho M, Sousa A, Lopes B, Sousa P, Moreira A, Coelho A, Rêma A, Alvites R, Ferreira A, Maurício AC and Martins  (2023) Intensive neurorehabilitation and allogeneic stem cells transplantation in canine degenerative myelopathy. Front. Vet. Sci. 10:1192744. doi: 10.3389/fvets.2023.1192744
Received: 23 March 2023; Accepted: 12 June 2023;
Published: 13 July 2023.
Edited by:
Jennifer Genevieve Barrett, Virginia Tech, United StatesReviewed by:
Zbigniew Adamiak, Vet Clinic Białystok, PolandHaithem Ali Mohamed Ahmed Farghali, Cairo University, Egypt
Rogerio Martins Amorim, São Paulo State University, Brazil
Copyright © 2023 Gouveia, Correia, Cardoso, Carvalho, Oliveira, Almeida, Gamboa, Ribeiro, Branquinho, Sousa, Lopes, Sousa, Moreira, Coelho, Rêma, Alvites, Ferreira, Maurício and Martins. This is an open-access article distributed under the terms of the Creative Commons Attribution License (CC BY). The use, distribution or reproduction in other forums is permitted, provided the original author(s) and the copyright owner(s) are credited and that the original publication in this journal is cited, in accordance with accepted academic practice. No use, distribution or reproduction is permitted which does not comply with these terms.
*Correspondence: Ana Colette Maurício, acmauricio@icbas.up.pt; ana.colette@hotmail.com