- Department of Veterinary Medicine, College of Agriculture and Veterinary Medicine, Qassim University, Buraydah, Saudi Arabia
The rise of multidrug resistant (MDR) microorganisms is a great hazard worldwide and has made it difficult to treat many infectious diseases adequately. One of the most prevalent causes of outbreaks of foodborne illness worldwide is Salmonella. The ability of this and other harmful bacteria to withstand antibiotics has recently proven crucial to their effective control. Since the beginning of time, herbal medicines and phytochemicals have been employed for their potent antibacterial action and there is a growing trend toward the production of plant based natural products for the prevention and treatment of pathogenic infections. Numerous phytochemicals have been proven effective against the molecular determinants responsible for attaining drug resistance in pathogens like efflux pumps, membrane proteins, bacterial cell communications and biofilms. The medicinal plants having antibacterial activity and antibiotics combination with phytochemicals have shown synergetic activity against Salmonella enterica serovar Typhimurium. The inhibitory effects of tannins on rumen proteolytic bacteria can be exploited in ruminant nutrition. Improved control of the rumen ecology and practical use of this feed additive technology in livestock production will be made possible by a better knowledge of the modulatory effects of phytochemicals on the rumen microbial populations in combination with fermentation. This review focuses on the development of antibacterial resistance in Salmonella, the mechanism of action of phytochemicals and the use of phytochemicals against S. enterica serovar Typhimurium. The advances and potential future applications of phytochemicals in the fight against resistant are also discussed.
Introduction
Foodborne diseases are brought on by consuming food, herbs, and beverages that have been contaminated by microorganisms as well as hazardous compounds including heavy metals (1), mycotoxins and bacterial toxins, as well as fermentation byproducts including biogenic amines and ethyl carbamate (2). Most of these foodborne illnesses are a problem for worldwide public health because they are brought on by pathogenic bacteria, viruses and parasites (3, 4). One of the main causes of foodborne diseases is Salmonellosis infection, which is brought on by a species of Salmonella (5, 6). Salmonella has long been recognized as an important zoonotic pathogen of economic importance in animals and humans. Salmonella enterica serovar Typhimurium can infect a wide range of animal species, e.g., cattle, sheep, goats, pigs, horses and poultry (7). There are pathovariants within serovar Typhimurium that are host-adapted, including sequence type (ST) 313 (8, 9) linked to invasive NTS (iNTS) in humans in sub-Saharan Africa and definitive phage types (DT) 2 and DT99 in pigeons (10), DT40 and DT56 in passerine birds (11) and U288 in pigs (12).
Salmonella Typhimurium belongs to the Enterobacteriaceae family. These Gram negative, flagellated rods are facultative anaerobic and do not produce spores (13). S. Typhi, which causes typhoid fever and gastrointertitis, a multi systemic disease, is a public health concern in developing countries. Salmonella species can be found throughout nature, although their primary sources include the GIT of mammals, reptiles, birds, and insects, as well as the environment that has been contaminated by human or animal waste (14). The most common clinical manifestation of salmonellosis in animals is an enteric disease, but numerous other conditions may be observed including acute septicemia, abortion, arthritis and respiratory diseases (15, 16).
Antibiotics are used in food animal production to promote growth and to prevent, treat and control infectious diseases. The antibiotics chloramphenicol, trimethoprim-sulfamethoxazole, ampicillin, fluoroquinolones and cephalosporin are the treatment options for S. Typhimurium (17). In emerging and particularly underdeveloped nations, the rise in antibiotic resistance in this disease has been a major concern. Resistance to antimicrobial agents may be defined as is the inability of bacteria to respond to medications that were once thought to be useful in treating infections brought on by that particular pathogen (18). By absorbing foreign DNA or by mutating its own DNA, S. Typhimurium can develop antibiotic resistance (19). Resistance to these antibiotics in S. Typhimurium strains is known as multi drug resistance (MDR) (20, 21). The rapid emergence of MDR among bacteria is caused by ongoing selective pressure and the evolution of new bacterial survival mechanisms in response to commonly used or recently produced antibiotics (22). Like all bacteria and depending on the strain and external factors, Salmonella attach to a variety of biotic and abiotic surfaces and form biofilms, posing a concern in food sectors and healthcare settings (23, 24). Biofilms are linked to about 80% of all bacterial illnesses in humans (25). Thus, Salmonella species discovered in their planktonic phase are typically susceptible to being eliminated by disinfectants or antibiotics, are significantly more resistant to these actions in biofilms (26). However, it costs a lot of money and time to find new antibiotics, and it takes around 10 years to get a new antibiotic on the market (27).
Therefore, there is a great effort to tackle antibacterial resistance and create effective, ecofriendly, and safe anti-biofilm techniques as well as therapeutic methods (28). Natural compounds, especially those derived from plants, have been an essential source of therapeutic medications over the past years with distinct features that make them suitable for use as alternative treatments for MDR infections that pose medical challenges (29). In order to protect themselves from microbial, herbivore, and insect predators, plants have an almost infinite capacity to mix aromatic molecules, primarily phenolic compounds, polyphenols, alkaloids, flavonoid, terpenoids, ketones, and essential oils (30). Many bioactive substance derived from substances, known as phytochemicals have been studied and found to be relatively safer than synthetic counterparts (31). These compounds also exert various therapeutic effects due to their high potency (32). Phytochemicals also known as phytobiotics or phytogenics that are added to animal feed to increase production. Phytochemicals are also proposed for use as antioxidants in animal feed, which will protect animals from oxidative damage caused by free radicals (33, 34). These phytochemicals have a variety of mechanisms of action, including the inhibition of efflux pumps and target altering and drug degrading enzymes (35). When used alone or in combination with other antibiotic compounds, phytochemicals have been found to have antimicrobial activities against clinically significant pathogens like Salmonella species, lowering the risk of developing a variety of diseases (36, 37). A successful method for modifying resistance is to use antimicrobial agents and phytochemicals in combination that will eliminate the resistance mechanism and still allow the medicine to be effective against resistant microorganisms (38). Plant extracts can be used to make natural additives with antibacterial properties that can be added to animal feed in an effort to reduce the use of antibiotics and switch to a more natural diet for animals (39). The main challenges that prevent plant based bioactive chemicals from being used commercially include a lack of raw materials, poor stability, high production costs, an unclear mode of action, and a lack of efficient regulatory systems (40). The aim of this review is to comprehensively present antibacterial resistance in Salmonella, the mechanism of action of phytochemicals and the use of plant-derived medicinal plants against S. Typhimurium.
Antibacterial resistance in Salmonella
Salmonella, that is multi drug-resistant, has emerged as one of the major foodborne pathogens, threatening global public health safety (41). Antibiotics are used as feed supplements at sub therapeutic doses to the economic effectiveness of animal production, to enhance growth and feed conversion efficiency and to avoid diseases (42). However, using in feed antibiotics (IFAs) could result in the emergence of antimicrobial resistance as animal farming intensifies, posing a potential risk to human health (43).
Salmonella resistance has been reported to a wide variety of antibiotics including sulfamethoxazole, tetracycline, cefotaxime chloramphenicol, compound trimethoprim, ampicillin, cephalosporins and nalidixic acid (44, 45). It is well known that the development of biofilms results in a high level of resistance in the bacteria as well as the horizontal transmission of resistance between bacterial cells through transformation and conjugation (46, 47). The activity of efflux pumps, target adaptation, enzymes expressions and mutation are the antimicrobial resistance mechanisms that occur in planktonic cells (48).
Mechanism of action of phytochemicals
Phytochemicals have possible biological effects, including antibacterial, antiviral, antioxidant, and anti-inflammatory, and used for animal nutrition and health improvement (43, 49, 50). Phytochemicals inhibit the growth of S. Typhimurium by several mechanisms (51). These might include preventing the bacterial attachment to host cells (52), reduction in the bacterial ability to produce proteins, cell wall, and nucleic acids (53), loss of the transmembrane electrochemical gradient and reduction of the osmoregulation of bacteria and increased nitric oxide (NO) synthesis, which has a deadly effect (54). Additionally, phytochemicals influence the immune system through immunomodulatory effects such as enhanced immune cell proliferation, modification of cytokines as well as higher antibody titers (55, 56).
Inhibition of cell wall synthesis
N-acetylglucosamine (NAcGlc) and N-acetylmuramic acid (NAcMur) residues are repeated units that make up peptidoglycan and these repeating units are joined by short amino acid chains. The arrangement of amino acid residues is essential for giving bacteria strength and consequently protection (57). In order to better control the formation of the bacterial cell wall, phytochemicals have been found to be helpful in therapeutic approaches. Due to their impact on the bacterial cell wall, flavonoids have a marked antibacterial effect against a variety of bacterial and infectious diseases. The presence of more lipophilic flavonoids may also disrupt bacterial membranes (58). The lysis of cell walls has also been notice in bacteria exposed to phenolic mixtures. By targeting bacterial cell wall, tannins have qualities that inhibit the growth and protease activity of ruminal bacteria and if they are highly lipophilic, they also disrupt cell layers (59). The tannin of Sorghum has antibacterial activity against S. Typhi (60). Alkaloids often exert their antibacterial effects by intercalating themselves into the DNA and cell wall of bacteria (61). Through the upregulation of immunoglobulin A and mucin 2, tannins are helpful in maintaining chicken mucosal immune system components. Through paracellular and transcellular pathways, Salmonella spp. can enter the bloodstream and use immune cells to enter enterocytes, which are then dispersed throughout the muscles and organs of chickens. Tannins change the functions and expression of immune cells, mucus and tight junction proteins of chickens (62–64) as shown in Figure 1. Tannins inhibit the growth of Salmonella spp. in the intestine and decrease the quorum sensing of bacteria.
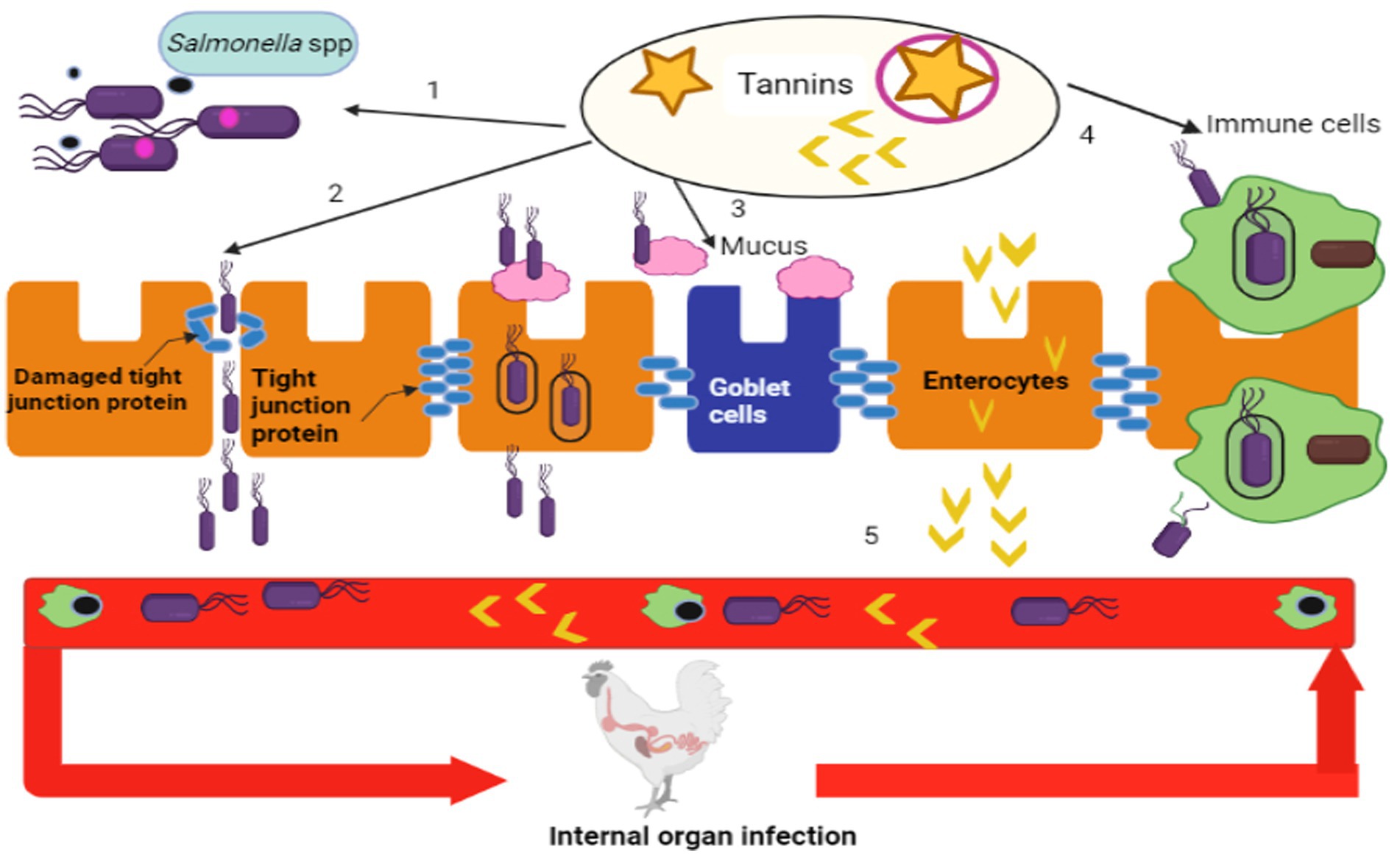
Figure 1. Systemic infection routes of Salmonella spp. and potential mechanisms of antibacterial actions of tannins (Retrieved from bio render).
Tannins that are used against S. Typimurium is Condensed tannins from Quebaracho and Calliandra calothyrsus, Gallotannins from Tara and Sumach (Gall nuts), Flavanol gallates from Tea and Acacia nilotica, Tannic acid and Gallic acid. All of the tannins inhibited the growth of the S. Typimurium (65).
Inhibition of bacterial physiology
When phytochemicals are added to the medium, the ensuing changes in membrane potential, inhibition of the function of membrane bound ATPase alter the physiological condition of the bacteria and metal ion chelation ultimately leading to bacterial death (66). The disruption of the membranes integrity by carvacrol, eugenol thymol and catechins has been observed to result in the release of cellular components and the ATP levels of cells (67). Additionally, terpinen-4-ol, 1,8-cineol, terpenes, alpha-terpineol and sesquiterpenes found in tea tree oil have the ability to alter membrane permeability, disrupt cell membranes, and inhibit cell development, leading to cell death in resistant organisms like S. Typhimurium (68).
Inhibition of biofilms
Biofilm is a collection of microbial populations with surface integration that is enclosed in an exopolysaccharide matrix (69). Phytochemicals are employed to prevent and inhibit biofilm growth as well as to combat the development of antibacterial resistance, by taking advantage of their disruption of some of the key elements involved in the formation of biofilms, such as motility, attachment, intercellular accumulation and interaction (70, 71) shown in Figure 2.
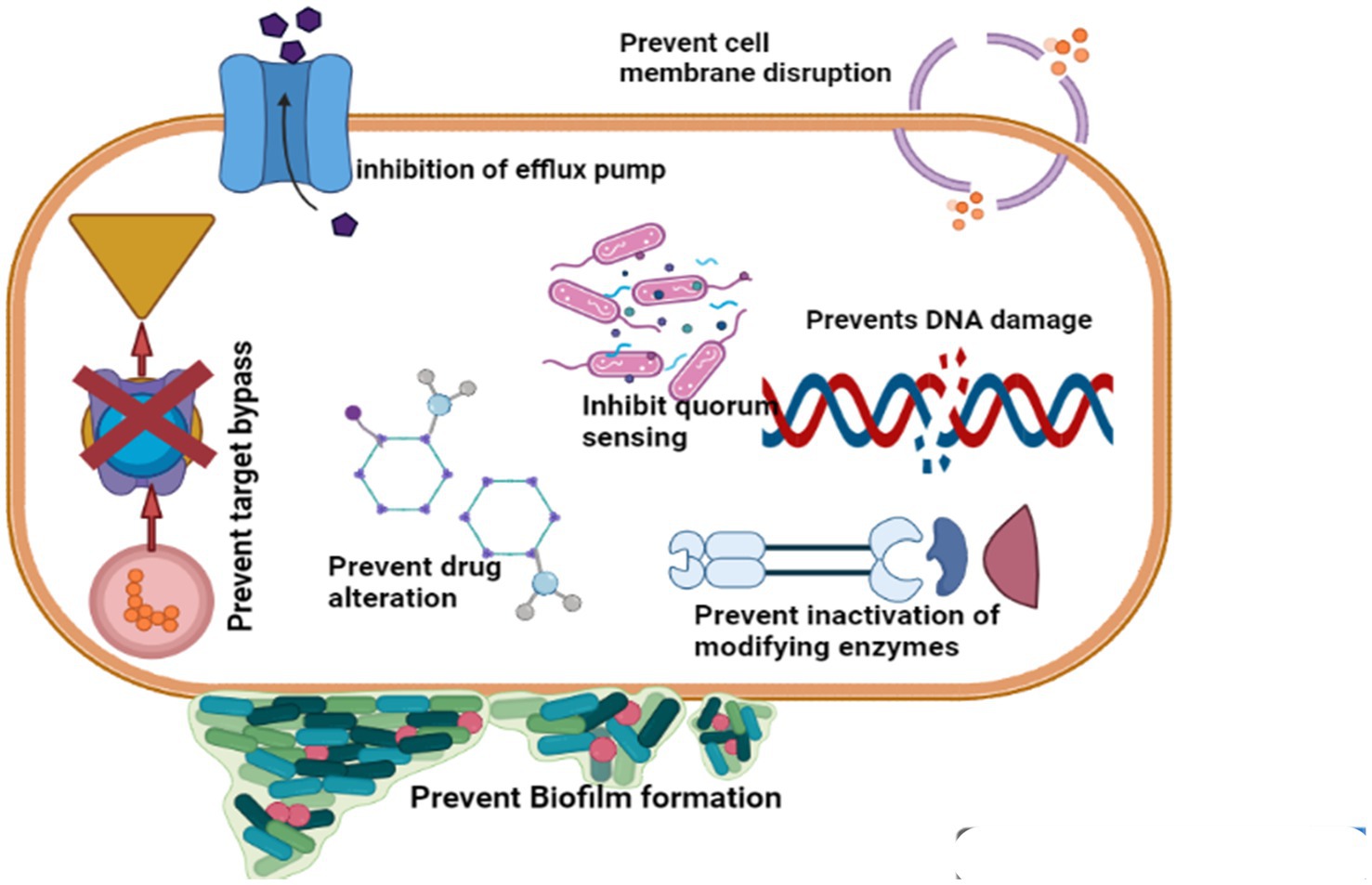
Figure 2. Phytochemicals are antibiotic alternatives and their mode of action (Retrieved from bio render).
Essential oils (EOs) components, lectins, alkaloids, polyacetylenes and polypeptides and terpenoids, phenolics, inhibit Salmonella growth and biofilm formation (72–74). The major ingredients in thyme oil and oregano, thymol and carvacrol, have antibiofilm properties against S. Enteritidis and S. Typhimurium on polypropylene (75). However, it has been demonstrated that Salmonella adapts to EOs and their constituents after being exposed to them at sub lethal concentrations by changing the expression of some important stress response genes. As a result, gains tolerance to both heterologous stressors and homologous (76). The anti-biofilm efficacy of two nutraceuticals of plant sources, Andrographis paniculata (Ap) and Holarrhena antidysentrica (Ha) are shown against S. Typhi biofilm development, whereby both exhibited and both showed antibiofilm and antimicrobial action by rupturing the membrane permeability of this pathogen (77).
Synergistic phytochemicals as active site modification inhibitors
To mitigate the harmful effects of enteric infections, a number of phytochemicals are combined to create synergistic effects. Different resistance mechanisms such as increased activation of efflux pumps (EPs), expression of drug inactivating and target site modifying enzymes and modification of permeability barriers, can be neutralized by phytochemicals in combination with currently available antibiotics in a synergistic manner (78). Antibiotics and phytochemical substances have been given together to stop the emergence of resistance and it is effective tool for the management of MDR (79). For instance, ubiquitous phytochemicals from the barberry plant berberine and 5′-methoxyhydnocarpin exhibit synergism by accumulating inside bacteria and blocking the MDR pump (80). It has been discovered that streptomycin in combination with eugenol or cinnamaldehyde work synergistically to destroy the S. Typhimurium biofilm (81). Geraniol, bioactive compound that can be found in the essential oil of Helichrysum italicum, can restore the effectiveness of quinolones, chloramphenicol and beta lactam antibiotics against MDR bacteria (82). Studies on β-resorcylic acid, thymol, eugenol, carvacrol and trans-cinnamaldehyde revealed that they boosted S. Typhi DT104’s susceptibility to 5 antibiotics due to an inhibitory activity on EPs (83). The synergistic activity of phytochemicals with antibiotics shown in Table 1.
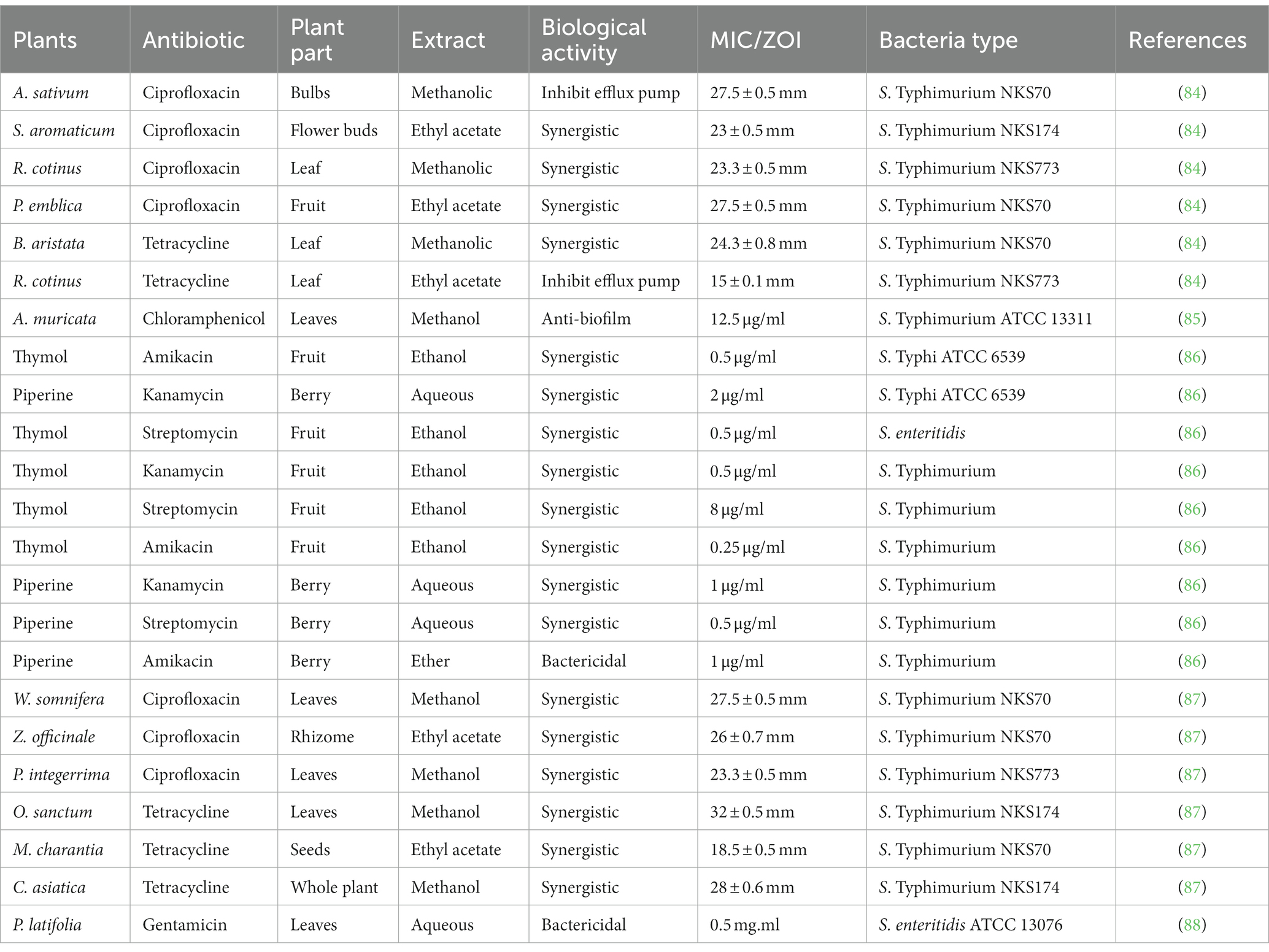
Table 1. Synergistic activity of phytochemicals with antibiotics and their minimum inhibitory concentration (MIC) or zone of inhibition (ZOI) values.
Plant- derived phytochemicals against Salmonella
Antibacterial resistance can be prevented, mitigated and reversed in a number of methods, whereby employing medicinal plant extracts with intrinsic antibacterial characteristics has been shown to be one of the most successful approaches (79, 89). When compared to synthetic chemicals, plant-derived antimicrobials have been found to be one of the most advantageous sources that are harmless due to their natural origin (90). For many years, bacterial infections have been treated by means of traditional healing systems using medicinal herbs (91). Around 80% of the developing nations uses traditional medicine made from phytochemicals as their primary health care modality (92, 93). Compared to their synthetic counterparts, medicinal plants are frequently less expensive, safer to use in terms of side effects and more accessible and also the probability for resistance development is most likely lessened due to the synergism of different bioactive compounds that can be present in plant-based formulation (any of them may belong to a different chemical group and be with a different mechanisms of action) (94). Gram-negative and Gram-positive bacteria are all affected by the bacteriostatic properties of resveratrol, which is a compound found in grapes and Itadori plants (95). Blackberry (Rubus fruticosus) and blueberry (Vaccinium corymbosum) pomace extracts were tested against S. Typhimurium at lethal and sub-lethal concentrations for their antibacterial, anti-motility, and antibiofilm activity. As growth promoters and to alter the gut microbiota, tannins and EOs are commercial food to a variety of domestic animal species (96). A commercial blend of phytonutrients that boosts innate immunity and lessens the harmful effects of enteric bacteria was approved in the Europe as the first botanical feed additive for enhancing the performance of broilers and livestock. This blend contains capsicum oleoresin, carvacrol and cinnamaldehyde (97). However, the best way to deal with antibacterial resistance is probably through a combinational strategy that allows for a synergistic interaction between plant extracts and conventional antibiotics. Streptomycin with either cinnamaldehyde or eugenol has been shown to work synergistically to destroy the S. Typhimurium biofilm (81). A detailed list of antibacterial activity of important medicinal plant extract and phytochemicals against Salmonella strains is provided in Tables 1, 2.
Conclusion and future prospective
Salmonella species have been labeled environmental persisters, mostly because of their powerful biofilm forming capacity. Because of this, a long lasting and persistent colonization of people, animals and plants is typically occurring. It is essential to develop antibiotics alternatives as soon as possible due to growing concerns about the spread of superbugs and the slow development of new medications for both livestock and humans. However, it has been found that numerous plant extract and their isolated phytochemicals exhibit strong efficacy against organisms that cause foodborne diseases. Numerous phytochemicals have showed promise as bactericidal or antimicrobial agents that can enhance the effects of already available antibiotics. These phytochemicals have demonstrated the ability to block key mechanisms for the development of resistance, including cell permeability, replication machinery, efflux pumps, and other processes necessary for the pathogen’s survival and resistance. These phytochemicals have displayed great effectiveness against bacteria that are resistant to antibiotics when used in combination. The possibility of a synergistic interaction between phytochemicals and established or newly developed antimicrobial agents is an opportunity, while the development of novel plant based antibacterial products through combinatorial chemistry and computational design continues to be an exciting challenge. Future research should also concentrate on the toxicological and pharmacokinetic properties of plant extracts and phytochemicals.
Author contributions
The author confirms being the sole contributor of this work and has approved it for publication.
Acknowledgments
The researcher would like to thank the Deanship of Scientific Research, Qassim University, for funding the publication of this project.
Conflict of interest
The author declares that the research was conducted in the absence of any commercial or financial relationships that could be construed as a potential conflict of interest.
Publisher’s note
All claims expressed in this article are solely those of the authors and do not necessarily represent those of their affiliated organizations, or those of the publisher, the editors and the reviewers. Any product that may be evaluated in this article, or claim that may be made by its manufacturer, is not guaranteed or endorsed by the publisher.
References
1. Ejaz, H, Junaid, K, Yasmeen, H, Naseer, A, Alam, H, Younas, S, et al. Multiple antimicrobial resistance and heavy metal tolerance of biofilm-producing bacteria isolated from dairy and non-dairy food products. Foods. (2022) 11:2728. doi: 10.3390/foods11182728
2. Gao, D, Ma, Z, and Jiang, Y. Recent advances in microfluidic devices for foodborne pathogens detection. TrAC Trends Anal Chem. (2022) 157:116788. doi: 10.1016/j.trac.2022.116788
3. Pires, SM, Desta, BN, Mughini-Gras, L, Mmbaga, BT, Fayemi, OE, Salvador, EM, et al. Burden of foodborne diseases: think global, act local. Curr Opin Food Sci. (2021) 39:152–9. doi: 10.1016/j.cofs.2021.01.006
4. Jamil, T, Kalim, F, Aleem, MT, Mohsin, M, Hadi, F, Ali, K, et al. Rodents as reservoirs and carriers of different zoonotic diseases. Continent Vet J. (2022) 2:1–14.
5. Wibisono, FM, Wibisono, FJ, Effendi, MH, Plumeriastuti, H, Hidayatullah, AR, Hartadi, EB, et al. A review of salmonellosis on poultry farms: public health importance. Syst Rev Pharm. (2020) 11:481–6.
6. Rabie, NS, Fedawy, HS, Sedeek, DM, Bosila, MA, Ghetas, AM, Elbayoumi, KM, et al. Trial for preparation and evaluation of autogenous killed vaccine against some locally isolated strains of Salmonella enterica from chickens in Egypt. Int J Vet Sci. (2023) 12:230–5. doi: 10.47278/journal.ijvs/2022.209
7. Demirbilek, SK. Salmonella: a re-emerging pathogen. Salmonellosis Anim. (2017) 2:19–31. doi: 10.5772/intechopen.72192
8. Kingsley, RA, Msefula, CL, Thomson, NR, Kariuki, S, Holt, KE, Gordon, MA, et al. Epidemic multiple drug resistant Salmonella typhimurium causing invasive disease in sub-Saharan Africa have a distinct genotype. Genome Res. (2009) 19:2279–87. doi: 10.1101/gr.091017.109
9. Okoro, CK, Barquist, L, Connor, TR, Harris, SR, Clare, S, Stevens, MP, et al. Signatures of adaptation in human invasive Salmonella typhimurium ST313 populations from sub-Saharan Africa. PLoS Negl Trop Dis. (2015) 9:e0003611. doi: 10.1371/journal.pntd.0003611
10. Cohen, E, Azriel, S, Auster, O, Gal, A, Zitronblat, C, Mikhlin, S, et al. Pathoadaptation of the passerine-associated Salmonella enterica serovar Typhimurium lineage to the avian host. PLoS Pathog. (2021) 17:e1009451. doi: 10.1371/journal.ppat.1009451
11. Kingsley, RA, Kay, S, Connor, T, Barquist, L, Sait, L, Holt, KE, et al. Genome and transcriptome adaptation accompanying emergence of the definitive type 2 host-restricted Salmonella enterica serovar Typhimurium pathovar. mBio. (2013) 4:e00565-13. doi: 10.1128/mBio.00565-13
12. Kirkwood, M, Vohra, P, Bawn, M, Thilliez, G, Pye, H, Tanner, J, et al. Ecological niche adaptation of Salmonella typhimurium U288 is associated with altered pathogenicity and reduced zoonotic potential. Commun Biol. (2021) 4:498. doi: 10.1038/s42003-021-02013-4
13. Jahan, F, Chinni, SV, Samuggam, S, Reddy, LV, Solayappan, M, and Su, YL. The complex mechanism of the Salmonella typhi biofilm formation that facilitates pathogenicity: a review. Int J Mol Sci. (2022) 23:6462. doi: 10.3390/ijms23126462
14. Jajere, SM. A review of Salmonella enterica with particular focus on the pathogenicity and virulence factors, host specificity and antimicrobial resistance including multidrug resistance. Vet World J. (2019) 12:504–21. doi: 10.14202/vetworld.2019.504-521
15. Uzal, FA, Navarro, MA, Li, J, Freedman, JC, Shrestha, A, and McClane, BA. Comparative pathogenesis of enteric clostridial infections in humans and animals. Anaerobe. (2018) 53:11–20. doi: 10.1016/j.anaerobe.2018.06.002
16. Kang, J, Hossain, MA, Park, HC, Kim, Y, Lee, KJ, and Park, SW. Pharmacokinetic and pharmacodynamic integration of enrofloxacin against Salmonella enteritidis after administering to broiler chicken by per-oral and intravenous routes. J Vet Sci. (2019) 20:e15. doi: 10.4142/jvs.2019.20.e15
17. Radha, S, Murugesan, M, and Rupali, P. Drug resistance in Salmonella typhi: implications for South Asia and travel. Curr Opin Infect Dis. (2020) 33:347–54. doi: 10.1097/QCO.0000000000000672
18. Sayyar, HT, Afroz, S, and Assad, T. Evaluation of phytochemical screening, antimicrobial and antioxidant activities of ethanol extracts of Cucumis flexouses and Cucumis reticulatus seeds. Pak Vet J. (2021) 41:142–6. doi: 10.29261/pakvetj/2020.089
19. Li, G, Huang, Y, Duan, M, Xing, K, You, X, Zhou, H, et al. Biosensing multiplexer based on immunochromatographic assay for rapid and high-throughput classification of Salmonella serogroups. Sensors Actuators B Chem. (2019) 282:317–21. doi: 10.1016/j.snb.2018.11.081
20. Klemm, EJ, Shakoor, S, Page, AJ, Qamar, FN, Judge, K, Saeed, DK, et al. Emergence of an extensively drug-resistant Salmonella enterica serovar Typhi clone harboring a promiscuous plasmid encoding resistance to fluoroquinolones and third-generation cephalosporins. mBio. (2018) 9:e00105-18. doi: 10.1128/mBio.00105-18
21. Morshdy, AE, Nahla, BM, Shafik, S, and Hussein, MA. Antimicrobial effect of essential oils on multidrug-resistant Salmonella typhimurium in chicken fillets. Pak Vet J. (2021) 41:545–51. doi: 10.29261/pakvetj/2021.055
22. Bukowski, K, Kciuk, M, and Kontek, R. Mechanisms of multidrug resistance in cancer chemotherapy. Int J Mol Sci. (2020) 21:3233. doi: 10.3390/ijms21093233
23. Moraes, JO, Cruz, EA, Souza, EG, Oliveira, TC, Alvarenga, VO, Peña, WE, et al. Predicting adhesion and biofilm formation boundaries on stainless steel surfaces by five Salmonella enterica strains belonging to different serovars as a function of pH, temperature and NaCl concentration. Int J Food Microbiol. (2018) 281:90–100. doi: 10.1016/j.ijfoodmicro.2018.05.011
24. Merino, L, Procura, F, Trejo, FM, Bueno, DJ, and Golowczyc, MA. Biofilm formation by Salmonella sp. in the poultry industry: detection, control and eradication strategies. Food Res Int. (2019) 119:530–40. doi: 10.1016/j.foodres.2017.11.024
25. Rumbaugh, KP, and Sauer, K. Biofilm dispersion. Nat Rev Microbiol. (2020) 18:571–86. doi: 10.1038/s41579-020-0385-0
26. Giaouris, E, and Nesse, LL. Attachment of Salmonella spp. to food contact and product surfaces and biofilm formation on them as stress adaptation and survival strategies In: CB Hackett, editor. Salmonella: prevalence, risk factors and treatment options. New York: Nova Science Publishers, Inc (2015). 111–36.
27. Miethke, M, Pieroni, M, Weber, T, Brönstrup, M, Hammann, P, Halby, L, et al. Towards the sustainable discovery and development of new antibiotics. Nat Rev Chem. (2021) 5:726–49. doi: 10.1038/s41570-021-00313-1
28. Giaouris, EE, and Simões, MV. Pathogenic biofilm formation in the food industry and alternative control strategies In: AM Holban and AM Grumezescu, editors. Foodborne diseases. Cambridge, MA: Academic Press (2018). 309–77.
29. Subramani, R, Narayanasamy, M, and Feussner, KD. Plant-derived antimicrobials to fight against multi-drug-resistant human pathogens. 3 Biotech. (2017) 7:1–5. doi: 10.1007/s13205-017-0848-9
30. Ancheeva, E, Daletos, G, and Proksch, P. Bioactive secondary metabolites from endophytic fungi. Curr Med Chem. (2020) 27:1836–54. doi: 10.2174/0929867326666190916144709
31. Yasmin, S, Nawaz, M, Anjum, AA, Ashraf, K, Basra, MA, Mehmood, A, et al. Phytochemical analysis and in vitro activity of essential oils of selected plants against Salmonella enteritidis and Salmonella gallinarum of poultry origin. Pak Vet J. (2020) 40:139–44. doi: 10.29261/pakvetj/2019.110
32. Baranowska, M, and Bartoszek, A. Antyoksydacyjne iprzeciwdrobnoustrojowe właściwości bioaktywnych tozwiązków żurawiny. Postepy Hig Med Dosw. (2016) 70:1460–8. doi: 10.5604/17322693.1227896
33. Gülçin, Ì, Şat, İG, Beydemir, Ş, Elmastaş, M, and Küfrevioğlu, Öİ. Comparison of antioxidant activity of clove (Eugenia caryophylata Thunb) buds and lavender (Lavandula stoechas L.). Food Chem. (2004) 87:393–400. doi: 10.1016/j.foodchem.2003.12.008
34. Raheel, I, Orabi, A, and Tag, N. Down regulation of biofilm and quorum sensing genes of Pseudomonas aeruginosa and Pasteurella multocida isolated from broiler chicken pericarditis lesions by the action of some essential oils. Int J Vet Sci. (2021) 10:301–6. doi: 10.47278/journal.ijvs/2021.058
35. Omojate Godstime, C, Enwa Felix, O, Jewo Augustina, O, and Eze, CO. Mechanisms of antimicrobial actions of phytochemicals against enteric pathogens–a review. J Pharm Chem Biol Sci. (2014) 2:77–85.
36. Fadli, M, Chevalier, J, Saad, A, Mezrioui, NE, Hassani, L, and Pages, JM. Essential oils from Moroccan plants as potential chemosensitisers restoring antibiotic activity in resistant gram-negative bacteria. Int J Antimicrob Agents. (2011) 38:325–30. doi: 10.1016/j.ijantimicag.2011.05.005
37. Kon, KV, and Rai, MK. Plant essential oils and their constituents in coping with multidrug-resistant bacteria. Expert Rev Anti-Infect Ther. (2012) 10:775–90. doi: 10.1586/eri.12.57
38. Ayaz, M, Ullah, F, Sadiq, A, Ullah, F, Ovais, M, Ahmed, J, et al. Synergistic interactions of phytochemicals with antimicrobial agents: potential strategy to counteract drug resistance. Chem Biol Interact. (2019) 308:294–303. doi: 10.1016/j.cbi.2019.05.050
39. Zanini, SF, Rodrigo Aliaga, D, Pina Pérez, MC, Sanz Puig, M, and Martínez, LA. Use of antimicrobials from plants in feed as a control measure for pathogenic microorganisms. J Microb Biochem Technol. (2015) 7:248–52. doi: 10.4172/1948-5948.1000218
40. Prakash, B, Kumar, A, Singh, PP, and Songachan, LS. Antimicrobial and antioxidant properties of phytochemicals: current status and future perspective In: Functional and preservative properties of phytochemicals. ed. R. C. Read (Cambridge, MA: Academic Press) (2020).
41. Hassan, M, Ali, A, Ahmad, A, Saleemi, MK, Wajid, M, Sarwar, Y, et al. Purification and antigenic detection of lipopolysaccharides of Salmonella enterica Serovar Typhimurium isolate from Faisalabad, Pakistan. Pak Vet J. (2021) 41:434–8. doi: 10.29261/pakvetj/2021.046
42. Ayalew, H, Zhang, H, Wang, J, Wu, S, Qiu, K, Qi, G, et al. Potential feed additives as antibiotic alternatives in broiler production. Front Vet Sci. (2022) 9:916473. doi: 10.3389/fvets.2022.916473
43. Gadde, U, Kim, WH, Oh, ST, and Lillehoj, HS. Alternatives to antibiotics for maximizing growth performance and feed efficiency in poultry: a review. Anim Health Res Rev. (2017) 18:26–45. doi: 10.1017/S1466252316000207
44. Karkey, A, Thwaites, GE, and Baker, S. The evolution of antimicrobial resistance in Salmonella typhi. Curr Opin Gastroenterol. (2018) 34:25–30. doi: 10.1097/MOG.0000000000000406
45. Borah, P, Dutta, R, Das, L, Hazarika, G, Choudhury, M, Deka, NK, et al. Prevalence, antimicrobial resistance and virulence genes of Salmonella serovars isolated from humans and animals. Vet Res Commun. (2022) 46:799–810. doi: 10.1007/s11259-022-09900-z
46. Virolle, C, Goldlust, K, Djermoun, S, Bigot, S, and Lesterlin, C. Plasmid transfer by conjugation in gram-negative bacteria: from the cellular to the community level. Genes. (2020) 11:1239. doi: 10.3390/genes11111239
47. Etayash, H, Alford, M, Akhoundsadegh, N, Drayton, M, Straus, SK, and Hancock, RE. Multifunctional antibiotic–host defense peptide conjugate kills bacteria, eradicates biofilms, and modulates the innate immune response. J Med Chem. (2021) 64:16854–63. doi: 10.1021/acs.jmedchem.1c01712
48. Cadena, M, Kelman, T, Marco, ML, and Pitesky, M. Understanding antimicrobial resistance (AMR) profiles of Salmonella biofilm and planktonic bacteria challenged with disinfectants commonly used during poultry processing. Foods. (2019) 8:275. doi: 10.3390/foods8070275
49. Liu, Y, Song, M, Che, TM, Bravo, D, and Pettigrew, JE. Anti-inflammatory effects of several plant extracts on porcine alveolar macrophages in vitro. J Anim Sci. (2012) 90:2774–83. doi: 10.2527/jas.2011-4304
50. Karzan, K, Shnawa, B, and Gorony, S. Antimicrobial activity of Cyperus rotundus Linn. extracts and phytochemical screening. Eurasian J Sci Eng. (2017) 312:82. doi: 10.23918/eajse.v3i2p82
51. Ullah, F, Ayaz, M, Sadiq, A, Ullah, F, Hussain, I, Shahid, M, et al. Potential role of plant extracts and phytochemicals against foodborne pathogens. Appl Sci. (2020) 10:4597. doi: 10.3390/app10134597
52. Klančnik, A, Šimunović, K, Sterniša, M, Ramić, D, Smole Možina, S, and Bucar, F. Anti-adhesion activity of phytochemicals to prevent Campylobacter jejuni biofilm formation on abiotic surfaces. Phytochem Rev. (2021) 20:55–84. doi: 10.1007/s11101-020-09669-6
53. Youmbi, LM, Atontsa, BC, Tankeo, SB, Wamba, BE, Nayim, P, Nganou, BK, et al. Antibacterial potential and mechanism of action of botanicals and phytochemicals from Stachytarpheta cayennensis (Verbenaceae) against gram-negative multidrug-resistant phenotypes expressing efflux pumps. Investig Med Chem Pharm. (2020) 3:1–9. doi: 10.31183/imcp.2020.00035
54. Jubair, N, Rajagopal, M, Chinnappan, S, Abdullah, NB, and Fatima, A. Review on the antibacterial mechanism of plant-derived compounds against multidrug-resistant bacteria (MDR). Evid Based Complement Alternat Med. (2021) 2021:1–30. doi: 10.1155/2021/3663315
55. Kim, DK, Lillehoj, HS, Lee, SH, Jang, SI, Lillehoj, EP, and Bravo, D. Dietary Curcuma longa enhances resistance against Eimeria maxima and Eimeria tenella infections in chickens. Poult Sci. (2013) 92:2635–43. doi: 10.3382/ps.2013-03095
56. Lee, Y, Lee, SH, Gadde, UD, Oh, ST, Lee, SJ, and Lillehoj, HS. Dietary Allium hookeri reduces inflammatory response and increases expression of intestinal tight junction proteins in LPS-induced young broiler chicken. Res Vet Sci. (2017) 112:149–55. doi: 10.1016/j.rvsc.2017.03.019
57. Do, T, Page, JE, and Walker, S. Uncovering the activities, biological roles, and regulation of bacterial cell wall hydrolases and tailoring enzymes. J Biol Chem. (2020) 295:3347–61. doi: 10.1074/jbc.REV119.010155
58. Upadhyay, A, Upadhyaya, I, Kollanoor-Johny, A, and Venkitanarayanan, K. Combating pathogenic microorganisms using plant-derived antimicrobials: a minireview of the mechanistic basis. Biomed Res Int. (2014) 2014:1–18. doi: 10.1155/2014/761741
59. Ekambaram, SP, Perumal, SS, and Balakrishnan, A. Scope of hydrolysable tannins as possible antimicrobial agent. Phytother Res. (2016) 30:1035–45. doi: 10.1002/ptr.5616
60. Chandra, H, Bishnoi, P, Yadav, A, Patni, B, Mishra, AP, and Nautiyal, AR. Antimicrobial resistance and the alternative resources with special emphasis on plant-based antimicrobials—a review. Plan Theory. (2017) 6:16. doi: 10.3390/plants6020016
61. Liu, Y, Cui, Y, Lu, L, Gong, Y, Han, W, and Piao, G. Natural indole-containing alkaloids and their antibacterial activities. Arch Pharm. (2020) 353:2000120. doi: 10.1002/ardp.202000120
62. Urdaneta, V, and Casadesús, J. Interactions between bacteria and bile salts in the gastrointestinal and hepatobiliary tracts. Front Med. (2017) 4:163. doi: 10.3389/fmed.2017.00163
63. Liu, HW, Li, K, Zhao, JS, and Deng, W. Effects of chestnut tannins on intestinal morphology, barrier function, pro-inflammatory cytokine expression, microflora and antioxidant capacity in heat-stressed broilers. J Anim Physiol Anim Nutr. (2018) 102:717–26. doi: 10.1111/jpn.12839
64. Li, X, Bleumink-Pluym, NM, Luijkx, YM, Wubbolts, RW, van Putten, JP, and Strijbis, K. MUC1 is a receptor for the Salmonella SiiE adhesin that enables apical invasion into enterocytes. PLoS Pathog. (2019) 15:e1007566. doi: 10.1371/journal.ppat.1007566
65. Reyes, AW, Hong, TG, Hop, HT, Arayan, LT, Huy, TX, Min, W, et al. The in vitro and in vivo protective effects of tannin derivatives against Salmonella enterica serovar Typhimurium infection. Microb Pathog. (2017) 109:86–93. doi: 10.1016/j.micpath.2017.05.034
66. Ahmad, Z, Hassan, SS, and Azim, S. A therapeutic connection between dietary phytochemicals and ATP synthase. Curr Med Chem. (2017) 24:3894–906. doi: 10.2174/0929867324666170823125330
67. Negi, PS. Plant extracts for the control of bacterial growth: efficacy, stability and safety issues for food application. Int J Food Microbiol. (2012) 156:7–17. doi: 10.1016/j.ijfoodmicro.2012.03.006
68. Çalişkan, U, and Özfenerci, M. Tea tree oil and its use in aromatherapy. Curr Pers Med Aromat Plants. (2018) 1:90–102.
69. Muhammad, MH, Idris, AL, Fan, X, Guo, Y, Yu, Y, Jin, X, et al. Beyond risk: bacterial biofilms and their regulating approaches. Front Microbiol. (2020) 11:928. doi: 10.3389/fmicb.2020.00928
70. Borges, A, Saavedra, MJ, and Simões, M. Insights on antimicrobial resistance, biofilms and the use of phytochemicals as new antimicrobial agents. Curr Med Chem. (2015) 22:2590–614. doi: 10.2174/0929867322666150530210522
71. Sadekuzzaman, M, Yang, S, Mizan, MF, and Ha, SD. Current and recent advanced strategies for combating biofilms. Compr Rev Food Sci Food Saf. (2015) 14:491–509. doi: 10.1111/1541-4337.12144
72. Salanță, LC, and Cropotova, J. An update on effectiveness and practicability of plant essential oils in the food industry. Plan Theory. (2022) 11:2488. doi: 10.3390/plants11192488
73. Raza, QS, Saleemi, MK, Gul, S, Irshad, H, Fayyaz, A, Zaheer, I, et al. Role of essential oils/volatile oils in poultry production—a review on present, past and future contemplations. Agrobiol Rec. (2022) 7:40–56. doi: 10.47278/journal.abr/2021.013
74. Merdana, IM, Watiniasih, NL, Sudira, IW, Arjana, AA, Nico, IW, Gunawan, F, et al. The effect of ethanolic extract of Myrmecodia pendans on gentamicin induced nephrotoxicity in wistar rats. Int J Vet Sci. (2021) 10:96–101. doi: 10.47278/journal.ijvs/2020.025
75. Amaral, VC, Santos, PR, da Silva, AF, dos Santos, AR, Machinski, M Jr, and Mikcha, JM. Effect of carvacrol and thymol on Salmonella spp. biofilms on polypropylene. Int J Food Sci Technol. (2015) 50:2639–43. doi: 10.1111/ijfs.12934
76. Cariri, ML, de Melo, AN, Mizzi, L, Ritter, AC, Tondo, E, de Souza, EL, et al. Quantitative assessment of tolerance response to stress after exposure to oregano and rosemary essential oils, carvacrol and 1, 8-cineole in Salmonella enteritidis 86 and its isogenic deletion mutants∆ dps,∆ rpoS and∆ ompR. Food Res Int. (2019) 122:679–87. doi: 10.1016/j.foodres.2019.01.046
77. Tanwar, A, Chawla, R, Chakotiya, AS, Thakur, P, Goel, R, Basu, M, et al. Effect of Holarrhena antidysentrica (Ha) and Andrographis paniculata (Ap) on the biofilm formation and cell membrane integrity of opportunistic pathogen Salmonella typhimurium. Microb Pathog. (2016) 101:76–82. doi: 10.1016/j.micpath.2016.11.001
78. Callaway, TR, Lillehoj, H, Chuanchuen, R, and Gay, CG. Alternatives to antibiotics: a symposium on the challenges and solutions for animal health and production. Antibiotics. (2021) 10:471. doi: 10.3390/antibiotics10050471
79. Cheesman, MJ, Ilanko, A, Blonk, B, and Cock, IE. Developing new antimicrobial therapies: are synergistic combinations of plant extracts/compounds with conventional antibiotics the solution? Pharmacogn Rev. (2017) 11:57–72. doi: 10.4103/phrev.phrev_21_17
80. Stermitz, FR, Lorenz, P, Tawara, JN, Zenewicz, LA, and Lewis, K. Synergy in a medicinal plant: antimicrobial action of berberine potentiated by 5′-methoxyhydnocarpin, a multidrug pump inhibitor. Proc Natl Acad Sci. (2000) 97:1433–7. doi: 10.1073/pnas.030540597
81. Liu, Q, Niu, H, Zhang, W, Mu, H, Sun, C, and Duan, J. Synergy among thymol, eugenol, berberine, cinnamaldehyde and streptomycin against planktonic and biofilm-associated food-borne pathogens. Lett Appl Microbiol. (2015) 60:421–30. doi: 10.1111/lam.12401
82. Lorenzi, V, Muselli, A, Bernardini, AF, Berti, L, Pagès, JM, Amaral, L, et al. Geraniol restores antibiotic activities against multidrug-resistant isolates from gram-negative species. Antimicrob Agents Chemother. (2009) 53:2209–11. doi: 10.1128/AAC.00919-08
83. Johny, AK, Hoagland, T, and Venkitanarayanan, K. Effect of subinhibitory concentrations of plant-derived molecules in increasing the sensitivity of multidrug-resistant Salmonella enterica serovar Typhimurium DT104 to antibiotics. Foodborne Pathog Dis. (2010) 7:1165–70. doi: 10.1089/fpd.2009.0527
84. Mehta, JY, Jandaik, SU, and Urmila, S. Evaluation of phytochemicals and synergistic interaction between plant extracts and antibiotics for efflux pump inhibitory activity against Salmonella enterica serovar typhimurium strains. Int J Pharm Pharm Sci. (2016) 8:217–23. doi: 10.22159/ijpps.2016v8i10.14062
85. Pinto, N d CC, Campos, LM, Evangelista, ACS, Lemos, ASO, Silva, TP, Melo, RCN, et al. Antimicrobial Annona muricata L. (soursop) extract targets the cell membranes of gram-positive and gram-negative bacteria. Ind Crop Prod. (2017) 107:332–40. doi: 10.1016/j.indcrop.2017.05.054
86. Tokam Kuaté, CR, Bisso Ndezo, B, and Dzoyem, JP. Synergistic antibiofilm effect of thymol and piperine in combination with aminoglycosides antibiotics against four Salmonella enterica serovars. Evid Based Complement Alternat Med. (2021) 2021:1–9. doi: 10.1155/2021/1567017
87. Mehta, J, Rolta, R, and Dev, K. Role of medicinal plants from North Western Himalayas as an efflux pump inhibitor against MDR AcrAB-TolC Salmonella enterica serovar typhimurium: in vitro and in silico studies. J Ethnopharmacol. (2022) 282:114589. doi: 10.1016/j.jep.2021.114589
88. da Silva, LD, Pallaoro, RB, de Freitas, EM, Hoehne, L, Heidrich, D, and Ethur, EM. Antibacterial activity of Lithraea molleoides Hook et Arn. and Poiretia latifolia Vogel essential oils combined with gentamicin on foodborne disease-causing bacteria. Biocatalysis Agric Biotechnol. (2023) 48:102620. doi: 10.1016/j.bcab.2023.102620
89. Elghobashy, KA, Eldanasoury, MM, Elhadary, AA, and Farid, M. Phytochemical constituent, HPLC profiling and antioxidant activity of Passiflora incarnata and Arctium lappa leaves extracts. Int J Vet Sci. (2020) 9:42–9.
90. Casciaro, B, Calcaterra, A, Cappiello, F, Mori, M, Loffredo, MR, Ghirga, F, et al. Nigritanine as a new potential antimicrobial alkaloid for the treatment of Staphylococcus aureus-induced infections. Toxins. (2019) 11:511. doi: 10.3390/toxins11090511
91. Tuasha, N, Petros, B, and Asfaw, Z. Medicinal plants used by traditional healers to treat malignancies and other human ailments in Dalle District, Sidama Zone, Ethiopia. J Ethnobiol Ethnomed. (2018) 14:1–21. doi: 10.1186/s13002-018-0213-z
92. Kasole, R, Martin, HD, and Kimiywe, J. Traditional medicine and its role in the management of diabetes mellitus:“patients’ and herbalists’ perspectives”. Evid Based Complement Alternat Med. (2019) 2019:1–12. doi: 10.1155/2019/2835691
93. Rafay, M, Ghaffar, MU, Abid, M, Malik, Z, and Madnee, M. Phytochemicals analysis and antimicrobial activities of Echinops echinatus from Cholistan Desert, Pakistan. Agrobiol Rec. (2021) 5:21–7. doi: 10.47278/journal.abr/2021.001
94. Rahman, MM, Dhar, PS, Anika, F, Ahmed, L, Islam, MR, Sultana, NA, et al. Exploring the plant-derived bioactive substances as antidiabetic agent: an extensive review. Biomed Pharmacother. (2022) 152:113217. doi: 10.1016/j.biopha.2022.113217
95. Taylor, EJ, Yu, Y, Champer, J, and Kim, J. Resveratrol demonstrates antimicrobial effects against Propionibacterium acnes in vitro. Dermatol Ther. (2014) 4:249–57. doi: 10.1007/s13555-014-0063-0
96. Lillehoj, H, Liu, Y, Calsamiglia, S, Fernandez-Miyakawa, ME, Chi, F, Cravens, RL, et al. Phytochemicals as antibiotic alternatives to promote growth and enhance host health. Vet Res. (2018) 49:1–8. doi: 10.1186/s13567-018-0562-6
97. Bravo, D, Pirgozliev, V, and Rose, SP. A mixture of carvacrol, cinnamaldehyde, and capsicum oleoresin improves energy utilization and growth performance of broiler chickens fed maize-based diet. J Anim Sci. (2014) 92:1531–6. doi: 10.2527/jas.2013-6244
98. Oussalah, M, Caillet, S, Saucier, L, and Lacroix, M. Inhibitory effects of selected plant essential oils on the growth of four pathogenic bacteria: E. coli O157: H7, Salmonella typhimurium, Staphylococcus aureus and Listeria monocytogenes. Food Control. (2007) 18:414–20. doi: 10.1016/j.foodcont.2005.11.009
99. Aliyu, MS, Hanwa, UA, Tijjani, MB, Aliyu, AB, and Ya’u, B. Phytochemical and antibacterial properties of leaf extract of Stereospermum kunthianum (Bignoniaceae). Nigerian J Basic Appl Sci. (2009) 17:235–9. doi: 10.4314/njbas.v17i2.49912
100. Talib, WH, and Mahasneh, AM. Antimicrobial, cytotoxicity and phytochemical screening of Jordanian plants used in traditional medicine. Molecules. (2010) 15:1811–24. doi: 10.3390/molecules15031811
101. Das, D, Ghosh, R, and Mandal, P. Biogenic synthesis of silver nanoparticles using S1 genotype of Morus alba leaf extract: characterization, antimicrobial and antioxidant potential assessment. SN Appl Sci. (2019) 1:1–6. doi: 10.1007/s42452-019-0527-z
102. Kumar, V, Shriram, V, and Mulla, J. Antibiotic resistance reversal of multiple drug resistant bacteria using Piper longum fruit extract. J Appl Pharm Sci. (2013) 3:112–6. doi: 10.7324/JAPS.2013.30322
103. Panda, SK. Ethno-medicinal uses and screening of plants for antibacterial activity from similipal biosphere reserve, Odisha. India J Ethnopharmacol. (2014) 151:158–75. doi: 10.1016/j.jep.2013.10.004
104. Al-Snafi, AE. Therapeutic properties of medicinal plants: a review of their antibacterial activity (part 1). Int J Pharmacol Toxicol. (2015) 6:137–58.
105. Bisi-Johnson, MA, Obi, CL, Samuel, BB, Eloff, JN, and Okoh, AI. Antibacterial activity of crude extracts of some South African medicinal plants against multidrug resistant etiological agents of diarrhoea. BMC Complement Altern Med. (2017) 17:1–9. doi: 10.1186/s12906-017-1802-4
106. Elisha, IL, Botha, FS, McGaw, LJ, and Eloff, JN. The antibacterial activity of extracts of nine plant species with good activity against Escherichia coli against five other bacteria and cytotoxicity of extracts. BMC Complement Altern Med. (2017) 17:133. doi: 10.1186/s12906-017-1645-z
107. Olivas-Quintero, S, López-Angulo, G, Montes-Avila, J, Díaz-Camacho, SP, Vega-Aviña, R, López-Valenzuela, JÁ, et al. Chemical composition and biological activities of Helicteres vegae and Heliopsis sinaloensis. Pharm Biol. (2017) 55:1473–82. doi: 10.1080/13880209.2017.1306712
108. Ebani, VV, Nardoni, S, Bertelloni, F, Tosi, G, Massi, P, Pistelli, L, et al. In vitro antimicrobial activity of essential oils against Salmonella enterica serotypes Enteritidis and Typhimurium strains isolated from poultry. Molecules. (2019) 24:900. doi: 10.3390/molecules24050900
109. Mahmood, N, Nazir, R, Khan, M, Khaliq, A, Adnan, M, Ullah, M, et al. Antibacterial activities, phytochemical screening and metal analysis of medicinal plants: traditional recipes used against diarrhea. Antibiotics. (2019) 8:194. doi: 10.3390/antibiotics8040194
110. AlSheikh, HM, Sultan, I, Kumar, V, Rather, IA, Al-Sheikh, H, Tasleem Jan, A, et al. Plant-based phytochemicals as possible alternative to antibiotics in combating bacterial drug resistance. Antibiotics. (2020) 9:480. doi: 10.3390/antibiotics9080480
111. Chingwaru, C, Bagar, T, and Chingwaru, W. Aqueous extracts of Flacourtia indica, Swartzia madagascariensis and Ximenia caffra are strong antibacterial agents against Shigella spp., Salmonella typhi and Escherichia coli O157. S Afr J Bot. (2020) 128:119–27. doi: 10.1016/j.sajb.2019.10.022
112. Dougnon, V, Hounsa, E, Agbodjento, E, Keilah, LP, Legba, BB, Sintondji, K, et al. Percentage destabilization effect of some West African medicinal plants on the outer membrane of various bacteria involved in infectious diarrhea. Biomed Res Int. (2021) 2021:1–12. doi: 10.1155/2021/4134713
113. Gado, DA, Abdalla, MA, Ahmed, AS, Madikizela, B, Nkadimeng, SM, Ehlers, MM, et al. In vitro antibacterial activity of Loxostylis alata extracts and isolated compounds against Salmonella species. BMC Complement Med Ther. (2021) 21:1–6. doi: 10.1186/s12906-021-03292-4
114. Iyevhobu, KO, Edo, EO, Airefetalor, AI, Jabbo, AA, Dare, DI, Ken-Iyevhobu, BA, et al. In-vitro evaluation of the anti-bacterial effect of Gossypium barbadense extracts on isolates of Salmonella typhi. J Microbes Res. (2022):1. doi: 10.0810/JMR.2022/0003
115. Akinyemi, KO, Mendie, UE, Smith, ST, Oyefolu, AO, and Coker, AO. Screening of some medicinal plants used in south-west Nigerian traditional medicine for anti-Salmonella typhi activity. J Herb Pharmacother. (2005) 5:45–60. doi: 10.1080/J157v05n01_06
116. Usman, H, and Osuji, JC. Phytochemical and in vitro antimicrobial assay of the leaf extract of Newbouldia laevis. Afr J Tradit Complement Altern Med. (2007) 4:476–80. doi: 10.4314/ajtcam.v4i4.31240
117. Oluwafemi, F, and Debiri, F. Antimicrobial effect of Phyllanthus amarus and Parquetina nigrescens on Salmonella typhi. Afr J Biomed Res. (2008) 11:2015–19. doi: 10.4314/ajbr.v11i2.50712
118. Negi, BS, and Dave, BP. In vitro antimicrobial activity of Acacia catechu and its phytochemical analysis. Indian J Microbiol. (2010) 50:369–74. doi: 10.1007/s12088-011-0061-1
119. Kothari, S, Mishra, V, Bharat, S, and Tonpay, SD. Antimicrobial activity and phytochemical screening of serial extracts from leaves of Aegle marmelos (Linn.). Acta Pol Pharm. (2011) 68:687–92.
120. Mursyid, AM. uji aktivitas antimikroba ekstrak dietil eter akar anting-anting (Acalypha australis L.) secara klt-bioautografi. As Syifaa J Farmasi. (2012) 4:209–18. doi: 10.33096/ja.v4i2.86
121. Pripdeevech, P, and Saansoomchai, J. Antibacterial activity and chemical composition of essential oil and various extracts of Fagraea fragrans Roxb. flowers. Chiang Mai J Sci. (2013) 40:214–23.
122. Deshmukh, SR, Dhas, YK, and Patil, BA. Comparative account on medicinal importance of Momordica charantia and its endophytes. World J Pharm Res. (2014) 3:632–40.
123. Osuagwu, GG, and Nwoko, N. The phytochemical screening and antibacterial activity of the leaves of CombretumPaniculatum (Vent) SolaniumMacrocarpon (L) and CatharanthusRoseus (L) G. Don. IOSR J Pharm Biol Sci. (2014) 9:58–65. doi: 10.9790/3008-091258
124. Kumar, RS, Balasubramanian, P, Govindaraj, P, and Krishnaveni, T. Preliminary studies on phytochemicals and antimicrobial activity of solvent extracts of Coriandrum sativum L. roots (Coriander). J Pharm Phytochem. (2014) 2:74–8.
125. Sadiq, MB, Hanpithakpong, W, Tarning, J, and Anal, AK. Screening of phytochemicals and in vitro evaluation of antibacterial and antioxidant activities of leaves, pods and bark extracts of Acacia nilotica (L.). Del Indus Crops Prod. (2015) 77:873–82. doi: 10.1016/j.indcrop.2015.09.067
126. Ajayi, O, Awala, S, Ogunleye, A, Okogbue, F, and Olaleye, BF. Antimicrobial screening and phytochemical analysis of Elaeis guineensis (ewe igi ope) against salmonella strains. Br J Pharm Res. (2016) 10:1–9. doi: 10.9734/BJPR/2016/23659
127. Subba, B, Srivastav, C, and Kandel, RC. Scientific validation of medicinal plants used by Yakkha community of Chanuwa VDC, Dhankuta, Nepal. Springerplus. (2016) 5:155. doi: 10.1186/s40064-016-1821-5
128. Musa, FM, Ameh, JB, Ado, SA, and Olonitola, OS. Evaluation of phytochemical and antibacterial properties of Terminalia avicennioides crude extract against selected bacteria from diarrhoeic patients. Bayero J Pure Appl Sci. (2016) 9:129–37. doi: 10.4314/bajopas.v9i1.20
129. Kuncha, J, Thirugnanasambantham, P, Shanmugam, K, and Narayanan, N. In vitro antibacterial and antifungal activity of hydro-alcoholic extract of Polyherbal formulation. J Pharm Sci Res. (2019) 11:721–5.
130. Santos, CA, Almeida, FA, Quecán, BX, Pereira, PA, Gandra, KM, Cunha, LR, et al. Bioactive properties of Syzygium cumini (L.) skeels pulp and seed phenolic extracts. Front Microbiol. (2020) 11:990. doi: 10.3389/fmicb.2020.00990
131. Lamponi, S, and Baratto, MC. Bioactivity of hydro-alcoholic extract of Petroselinum crispum. Longhua Chinese Med. (2020) 3:2616–806. doi: 10.21037/lcm-20-47
132. Ramos, S, De Grandis, RA, Dokkedal, AL, Bauab, TM, Pavan, FR, and Resende, FA. Antimicrobial, cytotoxic and mutagenic activities of Bauhinia holophylla hydroalcoholic extract. Am J Essen Oils Nat Prod. (2021) 9:1–6.
133. El-Shiekh, RA, Al-Mahdy, DA, Hifnawy, MS, and Abdel-Sattar, E. Biological and chemical assessment of Ochrosia elliptica Labill leaves. Arab J Sci Eng. (2021) 46:5247–55. doi: 10.1007/s13369-020-04986-6
134. Naz, S, Alam, S, Ahmed, W, Khan, SM, Qayyum, A, Sabir, M, et al. Therapeutic potential of selected medicinal plant extracts against multi-drug resistant Salmonella enterica serovar typhi. Saudi J Biol Sci. (2022) 29:941–54. doi: 10.1016/j.sjbs.2021.10.008
Keywords: Salmonella , antimicrobial resistance, plant extract, immune response, livestock
Citation: Almuzaini AM (2023) Phytochemicals: potential alternative strategy to fight Salmonella enterica serovar Typhimurium. Front. Vet. Sci. 10:1188752. doi: 10.3389/fvets.2023.1188752
Edited by:
Rao Zahid Abbas, University of Agriculture, Faisalabad, PakistanReviewed by:
Bushra Shnawa, Soran University, IraqRiaz Hussain, Islamia University of Bahawalpur, Pakistan
Filip Štrbac, University of Belgrade, Serbia
Copyright © 2023 Almuzaini. This is an open-access article distributed under the terms of the Creative Commons Attribution License (CC BY). The use, distribution or reproduction in other forums is permitted, provided the original author(s) and the copyright owner(s) are credited and that the original publication in this journal is cited, in accordance with accepted academic practice. No use, distribution or reproduction is permitted which does not comply with these terms.
*Correspondence: Abdulaziz M. Almuzaini, YW1temllbnlAcXUuZWR1LnNh