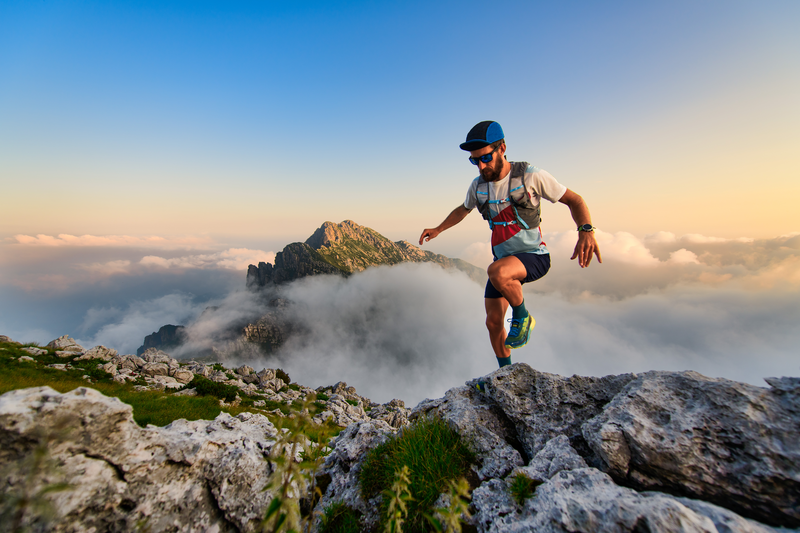
95% of researchers rate our articles as excellent or good
Learn more about the work of our research integrity team to safeguard the quality of each article we publish.
Find out more
ORIGINAL RESEARCH article
Front. Vet. Sci. , 07 August 2023
Sec. Veterinary Infectious Diseases
Volume 10 - 2023 | https://doi.org/10.3389/fvets.2023.1162402
Introduction: The introduction of invasive species into an ecosystem could result in biodiversity loss and the spread of infectious agents that could cause re-emergent or emergent zoonotic diseases. Monk parakeets (Myiopsitta monachus) and rose-ringed parakeets (Psittacula krameri) are considered widespread invasive exotic species in urban habitats from the Iberian Peninsula. The aim of this study was to assess the presence of relevant infectious agents in wild parakeets captured in urban parks in Madrid and Seville (Spain).
Methods: A total of 81 cloacal samples were collected and analysed using molecular techniques.
Results: The prevalence of infectious agents varied between parakeet species: 9.5% of monk parakeets and 15% of rose-ringed parakeets were positive for enteropathogenic E. coli (EPEC), 13.3% of rose-ringed parakeets for avian influenza virus (AIV), 3.3% of rose-ringed parakeets for Newcastle disease virus (NDV), and a 23.8% of monk parakeets for Chlamydia psittaci.
Discussion: All C. psittaci-identified isolates were classified as B, E, or E/B genotypes, indicating transmission from wild urban pigeons to parakeets. These results highlight the need for monitoring parakeet populations due to the implications for human and animal health.
The introduction of invasive species into a new environment where they have never been before may have the same negative effects on the ecosystem as the introduction of exotic diseases, which can lead to biodiversity loss or even the extinction of local species (1). In this sense, numerous issues have been reported around the world, such as the loss of amphibian populations caused by Batrachochytrium dendrobatidis, a fungus carried by the African clawed frog (Xenopus laevis) (2), or the decline of the European red squirrel (Sciurus vulgaris) in the United Kingdom due to a poxvirus transmitted by the American grey squirrel (Sciurus carolinensis) (3). Moreover, invasive species can also lead to the emergence, or re-emergence, of zoonotic diseases (4). An example is the role of coypus (Myocastor coypus) in the spread of zoonotic Leptospira (5), or raccoons (Procyon lotor) as hosts for Baylisascaris procyonis, which causes neurological and ocular disease in humans (6).
Regarding the Aves Class, up to 971 introduced bird species have been reported in 230 countries (7). However, only a few studies confirmed the introduction of diseases by alien bird species, mostly restricted to sporadic cases (8). The most relevant was the historical exportation of feral pigeons (Columba livia) from the Mediterranean countries. This synanthropic species has been proven to carry more than 110 zoonotic pathogens (9); some of them in high proportions, such as Campylobacter jejuni and Chlamydia psittaci (10). Other alien species carrying zoonotic pathogens described in the scientific literature are the house sparrow (Passer domesticus), the common starling (Sturnus vulgaris), and the song thrush (Turdus philomelos) (8). Therefore, health status variables should be included when conducting foreign species risk assessments to evaluate the introduction of new agents and the changes in the epidemiology of existing ones (11).
In Spain, the estimated monk parakeet (Myiopsitta monachus) population was between 18,980 and 21,455 in 2016 (13), while the rose-ringed parakeet (Psittacula krameri) population ranged between 3,005 and 3,115 in 2015 (14). Since then, the populations of both species have largely increased. For example, in the capital, Madrid, the population of monk parakeets grew from 7,248–8,193 in 2015 to 11,154–12,975 in 2019 (15). Although both species are worldwide invasive gregarious Psittacidae, monk parakeets build communal nests that can be used by other species (13), which favours the diffusion, amplification, and spread of numerous pathogens, while rose-ringed parakeets do not. Instead, after the mating season, rose-ringed parakeets gather to roost reaching high-density flocks that pose the same hazard as communal nests. However, there is a lack of knowledge about the infectious agents that both invasive species can harbour.
In this context, the aim of this study was to assess the presence of zoonotic and loss biodiversity-related infectious agents in both species, namely monk parakeets and rose-ringed parakeets, from two densely populated Spanish cities.
Due to the invasive ability of monk parakeets and rose-ringed parakeets, Spanish legislation includes an invasive species control program in which the removal of nests and euthanasia of trapped birds from these species are considered key to reducing populations (12).
First, the population size of each species in the different capture areas was established based on previous studies (16–19). Then, captures of monk parakeets in Madrid were performed between 2016 and 2017, while captures of rose-ringed parakeets in Sevilla took place between 2019 and 2020. Birds were trapped with both floor decoys with clap nets and nest traps. The sampling size (monk parakeets = 21 and rose-ringed parakeets = 60) was sufficient to detect a minimum expected prevalence of 13.2% and 4.8% in monk parakeets and rose-ringed parakeets, respectively for each analysed pathogen (Table 1) (www.winepi.net).
Table 1. Captured birds (N), number of analysed animals (N analysed), and percentage of animals analysed (%N) from the city council of Sevilla and Madrid, respectively.
All the captured birds were checked by a veterinarian and sampled before euthanasia to obtain the cloacal content by enema, as described by Vázquez (10). In brief, 1 mL of sterile PBS was introduced into the cloaca using a sterile Pasteur pipette and immediately aspirated. The cloacal sample was transferred into a 2-ml microtube and diluted to a total volume of 2 ml to perform further analysis.
Handling procedures complied with European (Directive 2010/63/EU) and Spanish legislation (Royal Decree 53/2013). For sample collection, ethics approval was not necessary as samples were collected within the framework of a veterinary disease control intervention, and sampling was performed following standard procedural guidelines.
For each sample, RNA and DNA extraction were simultaneously performed from the cloacal enema using a pressure filtration method (QuickGene DNA Tissue Kit S, Fujifilm Life Science, Tokyo, Japan) and adding an RNA carrier (20).
Real-time PCRs (RT-PCRs) based on TaqManTM probes were performed for the partial amplification of the incA gene of Chlamydia psittaci (21), the mapA gene of Campylobacter jejuni (23), and the Prot6e gene of Salmonella spp. (26). Samples positive for C. psittaci were typed by RT-PCR based on Eva Green, with high-resolution melting (HRM) analysis (22), which amplifies a partial fragment (274 bp) of the ompA gene. Positive confirmation was sought by Sanger sequencing of the amplicons. In addition, real-time reverse transcriptase (RT-rtPCR) based on TaqManTM probes was performed to detect the avian influenza virus (AIV) matrix gene (24) and the Newcastle disease virus (NDV) matrix gene (25). Finally, zoonotic E. coli was detected following the protocol described previously for the detection of the intimin gene (eaeA) (27, 28). Positive samples for the eaeA gene were analysed to assess the presence of stx-1 (29) and stx-2 (28). If the sample was positive for at least one of them, it was considered enterotoxigenic (STEC) strain, but if the sample was negative for both genes, it was considered enteropathogenic (EPEC) (20, 27). Primers, probes, and methodology are summarised in Table 2.
A non-parametric test (Mann–Whitney U-test) was applied to establish differences between monk parakeets and rose-ringed parakeets in the presence of each agent. Statistics were carried out using a commercially available software application (SPSS 29.0 software package; SPSS Inc., Chicago, IL, USA, 2002).
In total, 81 birds were included in the study: 21 monk parakeets and 60 rose-ringed parakeets. Among monk parakeets, 23.8% were positive for C. psittaci (5/21; CI 95% 5.6–42.0%). The results of partial ompA gene sequencing revealed that positive samples could be classified as B, E, or E/B genotypes. No positive rose-ringed parakeets were detected for C. psittaci (p < 0.001). AIVs and NDVs were detected only in rose-ringed parakeets: 13.3% were positive for AIV (8/60: CI 95% 4.7–21.9%) and 3.3% for NDV (2/60; CI 95% 0.0–7.9%). Regarding E. coli presence, 9.5% of the monk parakeets were positive for the intimin (eaeA) gene (2/21; CI 95% 0.0–22.1%), while the prevalence of this gene in rose-ringed parakeets was slightly higher (15%; 9/60; CI 95% 6.0–24%). All positive samples to eaeA were analysed for stx-1 and stx-2 genes, all of which were negative and therefore enteropathogenic strains (EPEC). All samples were negative for Campylobacter jejuni and Salmonella spp. (Table 3).
Table 3. Results after analysis in search of the different infectious agents in both invasive species (Myiopsitta monachus and Psittacula krameri): number of positive birds, percentage from the analysed population for each agent, and interval confidence at 95% level.
None of the birds showed clinical signs compatible with those pathogens, so positive birds were considered asymptomatic carriers.
To the best of our knowledge, this is the first study of potentially zoonotic bacterial and viral agents present in invasive Psittacidae living in urban areas. The results demonstrate that invasive species, namely monk parakeets and rose-ringed parakeets, can host zoonotic pathogens such as Chlamydia psittaci, AIV, NDV, or EPEC.
The prevalence of C. psittaci detected in monk parakeets was in concordance with previous studies with captive individuals of the same species (30). However, although the results are similar, it is important to consider that the habitat conditions are not the same. In contrast to our results, C. psittaci was also described in rose-ringed parakeets in previous studies with different prevalence trends, but none of those studies employed specific PCR for C. psittaci detection (31, 32). Among the Chlamydia genotypes described for birds, all authors agree that genotype A is the most prevalent in Psittacidae (33, 34), while genotypes B, E, and E/B are more common in pigeons (35–37). To the best of our knowledge, this is the first C. psittaci genotyping in monk parakeets worldwide. The presence of genotypes B, E, or E/B in monk parakeets suggests that bacteria have been transmitted from pigeons to parakeets in the opposite direction than expected. The different prevalence trends between monk and rose-ringed parakeets could be due to the feeding behaviour of each species and, thus, their interactions with other species. While monk parakeets feed mostly on the ground (38), rose-ringed parakeets do so in trees more frequently (39). Feeding on the ground gives monk parakeets the chance to interact with feral pigeons and then share pathogens with them, mainly respiratory ones. C. psittaci is highly prevalent in pigeons from Madrid (10), magnifying the transmission between pigeons and monk parakeets. To confirm this hypothesis, several approaches could be attempted. One approach could be to demonstrate the presence of C. psittaci in pigeons from Seville. The other approach could be to genotype positive samples from pigeons. In this sense, previously unpublished results obtained by our research group confirmed the presence of genotypes B, E, and B/E in pigeons from Madrid, which are those found in the present study, supporting this hypothesis. However, a strong phylogenetic analysis such as sequencing the full ompA gene is required to demonstrate this potential transmission.
Information about avian influenza in monk and rose-ringed parakeets is scarce. To the best of our knowledge, the present study is the first one conducted on urban free-living birds. In monk parakeets, only one study assessed the presence of AIV by hemagglutination assay in a monk parakeet imported to Austria with a negative result (47). In rose-ringed parakeets, two positive captive birds for H9N2 strains were reported during routine virologic diagnosis of the birds imported to Japan (48). Unfortunately, in our study, assays for the identification of highly pathogenic AIV (i.e., H5 or H7 variants) could not be attempted due to the limited amount of sample.
Similarly, the literature on NDV in monk and rose-ringed parakeets is old, and studies were performed using serology assays; therefore, positive samples only confirmed the contact of animals with the virus (49). Other studies focused on Psittacidae showed negative results for NDV detection (50, 51), with the exception of the study of NDV prevalence in India (52), where they found two positive samples out of four Psittaciformes analysed. Unfortunately, no data on which species were analysed were available. The present study confirms the presence of NDV in two rose-ringed parakeets. Unfortunately, the identification of velogenic strains could not be attempted due to the lack of a sample. Both AIV and NDV are notifiable in aviculture due to their serious economic and health repercussions, and wild birds, such as feral pigeons or hybrid ducks (Anas spp.), are considered reservoirs of both viruses (10).
Diarrheagenic E. coli (DEC) is one of the main causes of human diarrhoea, and wild birds have been suggested as potential reservoirs for these pathogens (45). Only few articles about DEC detection in monk and rose-ringed parakeets have been published. In 1978, Graham and Graham could not find E. coli in the faeces of seven captive monk parakeets by bacteriological culture (46). Our results showed that 9.5% of monk parakeets and 15% of rose-ringed parakeets were positive for EPEC, but none of the strains was classified as STEC. Although there is a disparity, it is hardly comparable because of the publication date, the technique used, and the bird habitat. This represents the first description of EPEC in those Psittacine species.
Finally, only few studies about Campylobacter jejuni and Salmonella spp. presence have been carried out in monk or rose-ringed parakeets (40–44). Our negative results agree with those published before for both species. However, a study performed on ring-rose parakeets confirmed 67% positivity for Campylobacter spp. with PCR detection (41).
It is important to highlight that the gregarious behaviour of each species contributes to the spread of pathogens through their ecosystem. Moreover, co-infection has been observed in two rose-ringed parakeets, one of which is positive for AIV and EPEC and the other for AIV and NDV. In conclusion, the present study focuses on pathogens with potential zoonotic effects present in two invasive Psittacidae species and provides an approach to assess their health risk in the ecosystem. The increase of their populations in urban green zones could represent a hazard to both humans and biodiversity due to their role as reservoirs of zoonotic pathogens. In this context, our results highlight the need for surveillance and monitoring programs for these species.
The original contributions presented in the study are included in the article/supplementary material, further inquiries can be directed to the corresponding author.
The animal study was reviewed and approved by Universidad Alfonso X El Sabio. Institutional Ethics Committee approval Reference Number: 2023_3/181.
JL: experimental design and text writing. MM: style and text revision. CB: sampling and text revision. BM-M and IS: sample lab analysis, results, and statistics. RG: sampling. JO: general revision. FE: experimental design, sample lab analysis, results, and statistics. All authors contributed to the article and approved the submitted version.
This study has been funded by the City Councils of Madrid and Sevilla.
JL, CB, BM-M, and RG are employed by Servicio de Consultoría para la Recuperación y Ordenación de la Fauna y su Ambiente S.L.
The remaining authors declare that the research was conducted in the absence of any commercial or financial relationships that could be construed as a potential conflict of interest.
All claims expressed in this article are solely those of the authors and do not necessarily represent those of their affiliated organizations, or those of the publisher, the editors and the reviewers. Any product that may be evaluated in this article, or claim that may be made by its manufacturer, is not guaranteed or endorsed by the publisher.
1. Bellard C, Bernery C, Leclerc C. Looming extinctions due to invasive species: Irreversible loss of ecological strategy and evolutionary history Running title: Functional and phylogenetic extinctions due to biological invasions. Glob Chang Biol. (2021) 27:4967–79. doi: 10.1111/gcb.15771
2. Stuart SN, Chanson JS, Cox NA, Young BE, Rodrigues ASL, Fischman DL, et al. Status and trends of amphibian declines and extinctions worldwide. Science. (2004) 306:1783–6. doi: 10.1126/science.1103538
3. Sainsbury AW, Deaville R, Lawson B, Cooley WA, Farelly SSJ, Stack MJ, et al. Poxviral disease in red squirrels Sciurus vulgaris in the UK: spatial and temporal trends of an emerging threat. Ecohealth. (2008) 5:305–16. doi: 10.1007/s10393-008-0191-z
4. Hulme PE. One Biosecurity: A unified concept to integrate human, animal, plant, and environmental health. Emerg Top Life Sci Portland Press Ltd. (2020) 4:539–49. doi: 10.1042/ETLS20200067
5. Michel V, Ruvoen-Clouet N, Menard A, Sonrier C, Fillonneau C, Rakotovao F, et al. Role of the coypu (Myocastor coypus) in the epidemiology of leptospirosis in domestic animals and humans in source. Eur J Epidemiol. (2001) 17:111–21. doi: 10.1023/A:1017931607318
6. Popiołek M, Szczęsna-Staśkiewicz J, Bartoszewicz M, Okarma H, Smalec B, Zalewski A. Helminth parasites of an introduced invasive carnivore species, the raccoon (Procyon lotor L), from the warta mouth national park (Poland). J Parasitol. (2011) 97:357–60. doi: 10.1645/GE-2525.1
7. Dyer EE, Redding DW, Blackburn TM. The global avian invasions atlas, a database of alien bird distributions worldwide. Sci Data. (2017) 4:170041. doi: 10.1038/sdata.2017.41
8. Roy HE, Tricarico E, Hassall R, Johns CA, Roy KA, Scalera R, et al. The role of invasive alien species in the emergence and spread of zoonoses. Biol Invasions. (2022) 25:1249–64. doi: 10.1007/s10530-022-02978-1
9. Mia MM, Hasan M, Hasnath MR. Global prevalence of zoonotic pathogens from pigeon birds: a systematic review and meta-analysis. Heliyon. (2022) 8:e09732. doi: 10.1016/j.heliyon.2022.e09732
10. Vázquez B, Esperón F, Neves E, López J, Ballesteros C, Muñoz MJ. Screening for several potential pathogens in feral pigeons (Columba livia) in Madrid. Acta Vet Scand. (2010) 52:45. doi: 10.1186/1751-0147-52-45
11. Andersen MC, Adams H, Hope B, Powell M. Risk assessment for invasive species. Risk Anal. (2004) 24:787–93. doi: 10.1111/j.0272-4332.2004.00478.x
13. Molina B, Postigo JL, Muñoz AR, del Moral JC. La Cotorra Argentina en España, población reproductora en 2015 y método de censo. Dissertation. Madrid: ED. SEO/BirdLife (2016).
14. del Moral JC, Somoza A, Muñoz AR, Molina B. La cotorra de Kramer en España, población reproductora en 2015 y método de censo. Dissertation. Madrid: ED. SEO/BirdLife (2017).
15. Nebreda A, Escudero E, del Moral JC. Censo de Cotorra Argentina en el municipio de Madrid. Dissertation. Madrid: ED. SEO/BirdLife (2019).
16. López R, Ballesteros C, López J. Memoria final sobre el inventariado y evaluación de nidos de Cotorra Argentina (Myopsitta monachus) en el Parque de las Cruces. Dissertation. Madrid: ED. SCROFA (2016).
17. López R, Ballesteros C, López J. Memoria final sobre el inventariado y evaluación de nidos de Cotorra Gris Argentina (Myopsitta monachus) en el Parque del Oeste. Dissertation. Madrid: ED. SCROFA (2016).
18. López R, Ballesteros C, López J. Memoria final sobre el inventariado y evaluación de nidos de Cotorra Argentina (Myopsitta monachus) en los jardines del Campo del Moro. Dissertation. Madrid: ED. SCROFA (2016).
19. López R, Ballesteros C, López J. Informe sobre la estima poblacional de la Cotorra de Kramer (Psittacula krameri) en el Parque de María Luisa (Sevilla)-Dormidero Avenida de Borbolla. Dissertation. Sevilla: ED. SCROFA (2019).
20. Sacristán C, Esperón F, Herrera-León S, Iglesias I, Neves E, Nogal V, et al. Virulence genes, antibiotic resistance and integrons in Escherichia coli strains isolated from synanthropic birds from Spain. Avian Pathol. (2014) 43:172–5. doi: 10.1080/03079457.2014.897683
21. Ménard A, Clerc M, Subtil A, Mégraud F, Bébéar C, De Barbeyrac B. Development of a real-time PCR for the detection of Chlamydia psittaci [2]. J Med Microbiol. (2006) 55:471–3. doi: 10.1099/jmm.0.46335-0
22. Mitchell SL, Wolff BJ, Thacker WL, Ciembor PG, Gregory CR, Everett KDE, et al. Genotyping of Chlamydophila psittaci by real-time PCR and high-resolution melt analysis. J Clin Microbiol. (2009) 47:175–81. doi: 10.1128/JCM.01851-08
23. Best EL, Powell EJ, Swift C, Grant KA, Frost JA. Applicability of a rapid duplex real-time PCR assay for speciation of Campylobacter jejuni and Campylobacter coli directly from culture plates. FEMS Microbiol Lett. (2003) 229:237–41. doi: 10.1016/S0378-1097(03)00845-0
24. Spackman E, Senne DA, Myers TJ, Bulaga LL, Garber LP, Perdue ML, et al. Development of a real-time reverse transcriptase PCR assay for type A influenza virus and the avian H5 and H7 hemagglutinin subtypes. J Clin Microbiol. (2002) 40:3256–60. doi: 10.1128/JCM.40.9.3256-3260.2002
25. Wise MG, Suarez DL, Seal BS, Pedersen JC, Senne DA, King DJ, et al. Development of a real-time reverse-transcription pcr for detection of newcastle disease virus RNA in clinical samples. J Clin Microbiol. (2004) 42:329–38. doi: 10.1128/JCM.42.1.329-338.2004
26. Malorny B, Bunge C, Helmuth R, A. real-time PCR for the detection of Salmonella Enteritidis in poultry meat and consumption eggs. J Microbiol Methods. (2007) 70:245–51. doi: 10.1016/j.mimet.2007.04.013
27. Torres-Mejía AM, Blanco-Peña, K, Rodríguez C, Duarte F, Jiménez-Soto M, Esperón F. Zoonotic agents in Feral pigeons (Columba livia) from Costa Rica: possible improvements to dominish contagion risks. Vector Borne Zoonotic Dis. (2018) 18:49–54. doi: 10.1089/vbz.2017.2131
28. Vidal R, Vidal M, Lagos R, Levine M, Prado V. Multiplex PCR for diagnosis of enteric infections associated with diarrheagenic Escherichia coli. J Clin Microbiol. (2004) 42:1787–9. doi: 10.1128/JCM.42.4.1787-1789.2004
29. Chui L, Couturier MR, Chiu T, Wang G, Olson AB, McDonald RR, et al. Comparison of Shiga toxin-producing Escherichia coli detection methods using clinical stool samples. J Mol Diagn. (2010) 12:469–75. doi: 10.2353/jmoldx.2010.090221
30. Origlia JA, Cadario ME, Frutos, MC, López NF, Corva S, et al. Detection and molecular characterization of Chlamydia psittaci and Chlamydia abortus in psittacine pet birds in Buenos aires province, Argentina. Rev Argent Microbiol. (2019) 51:130–5. doi: 10.1016/j.ram.2018.04.003
31. Pisanu B, Laroucau K, Aaziz R, Vorimore F, Gros A, le Chapuis JL, et al. Chlamydia avium detection from a ring-necked parakeet (Psittacula krameri) in France Chlamydia avium detection from a ring-necked parakeet (Psittacula krameri). J Exot Pet Med. (2018) 27:68–74. doi: 10.1053/j.jepm.2018.02.035
32. Chahota R, Katoch RC, Batta MK. Prevalence of Chlamydia psittaci among feral birds in himachal Pradesh, India. J Appl Anim Res. (1997) 12:89–94. doi: 10.1080/09712119.1997.9706190
33. Sutherland M, Sarker S, Vaz PK, Legione AR, Devlin JM, Macwhirter PL, et al. Disease surveillance in wild Victorian cacatuids reveals co-infection with multiple agents and detection of novel avian viruses. Vet Microbiol. (2019) 235:257–64. doi: 10.1016/j.vetmic.2019.07.012
34. Vanrompay D, Butaye P, Sayada C, Ducatelle R, Haesebrouck F. Characterization of avian Chlamydia psittaci strains using omp1 restriction mapping and serovar-specific monoclonal antibodies. Res Microbiol. (1997) 148:327–33. doi: 10.1016/S0923-2508(97)81588-4
35. Andersen AA. Serotyping of Chlamydia psittaci isolates using serovar-specific monoclonal antibodies with the microimmunofluorescence test. J Clin Microbiol. (1991) 29:707–11. doi: 10.1128/jcm.29.4.707-711.1991
36. Sachse K, Laroucau K, Vanrompay D. Avian chlamydiosis. Curr Clin Microbiol Rep. (2015) 2:10–21. doi: 10.1007/s40588-014-0010-y
37. Stokes HS, Berg ML, Bennett ATD, A. Review of chlamydial infections in wild birds. Pathogens. (2021) 10:948. doi: 10.3390/pathogens10080948
38. Postigo JL, Carrillo-Ortiz J, Domènech J, Tomàs X, Arroyo L, Senar JC. Dietary plasticity in an invasive species and implications for management: the case of the monk parakeet in a Mediterranean city. Anim Biodivers Conserv. (2021) 44:185–94. doi: 10.32800/abc.2021.44.0185
39. Fraticelli F. The rose-ringed parakeet Psittacula krameri in a urban park: demographic trend, interspecific relationships and feeding preferences (Rome, central Italy). Avocetta. (2014) 38:23–8.
40. De Luca C, Niero G, Cattarossi D, Bedin M, Piccirillo A. Pet and captive birds as potential reservoirs of zoonotic bacteria. J Exot Pet Med. (2018) 27:17–20. doi: 10.1053/j.jepm.2017.10.017
41. Seifi S, Khoshbakht R, Azizpour A, Seifi S. Occurrence of Campylobacter, Salmonella, and Arcobacter in pet birds of northern Iran. J Hellenic Vet Med Soc. (2019) 17:70. doi: 10.12681/jhvms.22248
42. Lopes ES, Cardoso WM, Albuquerque ÁH, Teixeira RSC, Salles RPR, Bezerra WGA, et al. Isolation of Salmonella spp. in captive Psittaciformes from zoos and a commercial establishment of Fortaleza, Brazil. Arq Bras Med Vet Zootec. (2014) 66:965–8. doi: 10.1590/1678-41626643
43. Gonzalez GA. Estudio serológico de Chlamidia psittaci y Salmonella sp., Virus Pox Aviar, Adenovirus y virus polioma en aves del orden psittaciforme en cautiverio en Dissertation. Chile: Universidad de Chile (2006).
44. Allgayer MC, Lima-Rosa CA, Weimer TA, Rodenbusch CR, Pereira RA, Streck AF, et al. Molecular diagnosis of Salmonella species in captive psittacine birds. Vet Rec. (2008) 162:816–9. doi: 10.1136/vr.162.25.816
45. Lopes ES, Maciel WC, Medeiros PHQS, Bona MD, Bindá AH, Lima SVG, et al. Molecular diagnosis of diarrheagenic Escherichia coli isolated from Psittaciformes of illegal wildlife trade. Pesqui Vet Bras. (2018) 38:762–6. doi: 10.1590/1678-5150-pvb-5083
46. Graham CL, Graham DL. Occurrence of Escherichia coli in feces of psittacine birds. Avian Dis. (1978) 22:717–20. doi: 10.2307/1589649
47. Stunzner D, Thiel W, Potsch F, Sixl W. Isolation of influenza viruses from exotic and central european birds. Zentralbl Bakteriol Mikrobiol Hyg. (1980) 247:8–17. doi: 10.1016/S0172-5599(80)80015-0
48. Mase M, Imada T, Sanada Y, Etoh M, Sanada N, Tsukamoto K, et al. Imported parakeets harbor H9N2 influenza A viruses that are genetically closely related to those transmitted to humans in Hong Kong. J Virol. (2001) 75:3490–4. doi: 10.1128/JVI.75.7.3490-3494.2001
49. Vijayan V. Role of Parrots in the Epizootiology of Newcastle Disease. Thesis. Mannuthy: College of Veterinary and Animal Sciences, Mannuthy (1981).
50. Gilardi KVK, Lowenstine LJ, Gilardi JD, Munn4 CA, A. Survey for selected viral, Chlamydial, and parasitic diseases in wild dusky-headed parakeets (Aratinga weddelii) and tui parakeets (Brotogeris Sanctithomae) in Peru. J Wildl Dis. (1995) 31:523–8. doi: 10.7589/0090-3558-31.4.523
51. Johnson DC, Couvillion CE, Pearson JE. Failure to demonstrate viscerotropic velogenic newcastle disease in psittacine birds in the Republic of the Philippines. Avian Dis. (1986) 30:813–5. doi: 10.2307/1590590
Keywords: Myiopsitta monachus, one health, Psittacula krameri, real time PCR, urban parks, zoonotic pathogens, invasive species
Citation: López J, Mogedas M, Ballesteros C, Martín-Maldonado B, Sacristán I, García R, Ortiz JC and Esperón F (2023) Infectious agents present in monk parakeet (Myiopsitta monachus) and rose-ringed parakeet (Psittacula krameri) invasive species in the parks of Madrid and Seville, Spain. Front. Vet. Sci. 10:1162402. doi: 10.3389/fvets.2023.1162402
Received: 09 February 2023; Accepted: 17 July 2023;
Published: 07 August 2023.
Edited by:
Marta Canuti, University of Milan, ItalyReviewed by:
Jesús Cardells, Universidad CEU Cardenal Herrera, SpainCopyright © 2023 López, Mogedas, Ballesteros, Martín-Maldonado, Sacristán, García, Ortiz and Esperón. This is an open-access article distributed under the terms of the Creative Commons Attribution License (CC BY). The use, distribution or reproduction in other forums is permitted, provided the original author(s) and the copyright owner(s) are credited and that the original publication in this journal is cited, in accordance with accepted academic practice. No use, distribution or reproduction is permitted which does not comply with these terms.
*Correspondence: Juan López, anVhbmxvcm9AdWF4LmVz
†These authors share first authorship
Disclaimer: All claims expressed in this article are solely those of the authors and do not necessarily represent those of their affiliated organizations, or those of the publisher, the editors and the reviewers. Any product that may be evaluated in this article or claim that may be made by its manufacturer is not guaranteed or endorsed by the publisher.
Research integrity at Frontiers
Learn more about the work of our research integrity team to safeguard the quality of each article we publish.