- 1Department of Animal Breeding and Animal Productions, University of Agricultural Sciences and Veterinary Medicine, Cluj-Napoca, Romania
- 2Department of Pathology, University of Agricultural Sciences and Veterinary Medicine, Cluj-Napoca, Romania
- 3Synevovet, Bucharest, Romania
- 4Molecular Diagnosis Laboratory, Clinical Hospital of Infectious Diseases of Cluj-Napoca, Cluj-Napoca, Romania
- 5Department of Parasitology and Parasitic Diseases, University of Agricultural Sciences and Veterinary Medicine, Cluj-Napoca, Romania
- 6Parasitology Consultancy Group, Coruşu, Romania
The American bison (Bison bison) is the largest terrestrial mammal of North America, with around 350,000 individuals in the wild and in private herds but the knowledge regarding the presence of different vector-borne pathigens in these mammals is very poor. Babesia and Theileria spp. are tick-borne apicomplexan parasites which are considered to be among the most commonly found blood parasites of large ruminants, often with a high economic importance. However, the knowledge on piroplasms of bisons is extremely scarce. The aim of our study was to evaluate the presence of apicomplexan parasites in blood and tissues of farmed American bison from Romania. Overall, we tested 222 blood samples and 11 tissues samples (heart, liver, and spleen) from farmed B. bison raised for meat in Romania. All the samples were analyzed by nPCR targeting the 18SrRNA gene for piroplasmids. All positive samples were sequenced and analyzed phylogenetically. The overall prevalence of infection with piroplasmids in American bison was 1.65%, with Babesia divergens and Theileria sp. identified following sequencing. To our knowledge, this is the first report of piroplasms detected in blood and tissues of farmed B. bison from Europe. Further studies are necessary in order to obtain a better overview on the epidemiological status and clinical relevance of piroplasms in farmed American bisons.
1. Introduction
Bisons are large ungulates which became almost extinct by the beginning of the 20th century due to extreme hunting, habitat reduction and diseases transmitted from domestic livestock, especially cattle (1). They are the largest terrestrial mammals in North America and Europe. Currently there are two extant species: the American bison, Bison bison and the European bison or wisent, Bison bonasus, and six extinct species (Bison palaeosinensis, Bison priscus, Bison schoetensacki, Bison antiquus, Bison latifrons, and Bison occidentalis) which were present in south and north Asia, Europe, and North America (2–5). The number of bisons (Bison bison) from Romania is estimated to be around 1,500, all being raised for the meat industry.
The American bison is raised for meat production in a wide range of environments, using different management systems. The heard size ranges between 10 and several hundred individuals. These bisons are considered semi-domesticated, and their meat is highly appreciated due to the lack of antibiotics and hormones, low fat (<3%) and low cholesterol content (6).
Most studies regarding the diseases of American bisons were focused on viral and bacterial diseases, such as malignant catarrhal fever, brucellosis (particularly in the Yellowstone Park), anthrax, clostridiosis and vibriosis (7–9). In farmed bisons, the studies are even more scarce (10, 11). Surprisingly, there is only one field study reporting the presence of piroplasms in American bisons (12), followed by an experimental study (13).
Generally speaking, the most common piroplasms infecting large domestic ruminants (cattle, water buffalo) in North America are Babesia bovis, Babesia bigemina (14), and Theileria orientalis (15). Conversely, in Europe, the piroplasms more commonly reported in large ruminants are Babesia divergens, Babesia major, Babesia occultans, and T. orientalis (16). To the current date, there are no reports of piroplasmid infections in European bisons, nor in farmed American bisons from Europe. Hence, the aim of the present study was to evaluate the presence of piroplasmids in samples collected from Bison bison from Romania and to evaluate their phylogenetic relations with other piroplasms isolated from different large ungulates.
2. Material and methods
2.1. Sampling and DNA isolation
2.1.1. Dead bisons
Between 2010 and 2015, a total of 36 dead adult bisons were brought to the Department of Pathology (Faculty of Veterinary Medicine Cluj-Napoca, Romania) for post-mortem examination. All individuals originated from a single location (Taga, Cluj County), where they were raised for meat. From the examined dead bisons, 13 cases were diagnosed (by PCR and microbiological tests) with various infectious diseases, including bovine viral diarrhea (BVD; n = 8), pulmonary pasteurelllosis (n = 3), and intestinal clostridiosis (n = 2). Cachexia related to severe infestation with hepatic flukes (Fasciola hepatica) and intestinal helminths (Trichuris spp.) was identified in seven cases. Three bisons died because of traumatic reticulopericarditis and peritonitis with intralesional metallic foreign bodies (Supplementary Figure 1). Fatal fight-related injuries were identified in other three individuals. In four bisons, because of advanced postmortem autolytic changes, the cause of death could not be established. The examination of the body surface of dead animals revealed the presence of hard ticks (Ixodidae) in three cases, which were collected and further morphologically identified using Olympus Bx51 microscope following the keys by Estrada-Pena et al. (17). Tissue samples were collected from six individuals with a suspicion of Babesia spp. infection, based on pathological findings. The collected tissue samples were represented by heart (n = 4), spleen (n = 3) and liver (n = 4). Unfortunately, in some animals, certain organs presented advanced stages of postmortem autolysis. Tissue samples were collected from individuals with a suspicion of Babesia spp. infection, based on gross pathological findings (e.g., splenomegaly, pale liver). Kidneys and lungs were collected for histological examination. On the other hand, in some animals, certain organs presented advanced stages of postmortem autolysis (especially the brain). Samples were first taken from dead bison and later, when lesions and signs of babesiosis were detected, blood samples were also taken from live animals to test for the presence of piroplasms. The clinical signs cannot be assessed because the animals roam freely in a very large area and cannot be cached. Samples were taken from dead bisons brought to necropsy by the owners and from live animals during blood collection for the national survey on diseases. DNA was extracted and tested for the presence of piroplasmids from each tissue sample. For cytology, multiple imprint smears from the spleen (n = 3) were stained with Dia-Quick Panoptic (Reagent Ltd., Budapest, Hungary) and subsequently examined using a high magnification objective (40 ×) and oil immersion (100 ×) to evaluate the morphological changes and to determine the presence of parasitic organisms. For histology, the samples were fixed in 10% phosphate buffered formalin for 24 h, embedded in paraffin wax, cut into 3 μm sections, and stained with hematoxylin and eosin (HE). Samples were examined using an Olympus Bx51 microscope and the photomicrographs were taken using an Olympus SP 350 digital camera and Stream basic imaging software (Olympus Corporation, Tokyo, Japan).
2.1.2. Live bisons
Additionally, in order to evaluate the occurrence of piroplasmids in live bisons, a total of 222 blood samples were collected from American bisons between 2016 and 2017, originating from three farms, closely located to the farm from which dead individuals were obtained, where animals were also raised for meat (Figure 1). The blood was collected as part of the national strategic program for monitoring brucellosis and leucosis. The number of samples collected from each location was as follows: from Panticeu 66 samples, Recea Cristur 97 samples, and Sărata 59 samples. These numbers of samples collected represent the total number of animals that were on the farm at the time of collection. The bison are free-ranging and contact with ectoparasites, especially ticks, is very common. Collecting ticks from live animals is not possible because their size and temperament. For blood collection from live animals, they were held for a short period and not anesthetized.
2.2. DNA extraction
All blood samples were stored at −20°C until DNA extraction and the tissues in formalin for histopathological examination. Genomic DNA was extracted as soon as possible from 25 mg of heart or liver tissue, 10 mg of spleen tissue or 200 μl of blood, using Isolate II Genomic DNA Kit (Meridian Bioscience, London, United Kingdom) according to the manufacturer's instructions and stored at −20°C until further testing. The sample quantity for DNA extraction was established following the manufacturer's instructions and literature overview.
2.3. PCR amplification and electrophoresis
A nested PCR (nPCR) targeting a 561 bp fragment of the 18S rDNA nuclear gene of piroplasmids was employed. The primers used for the nPCR were the following: BTH-1 F (5′-CCT GAG AAA CGG CTA CCA CAT CT-3′), and BTH-1R (5′-TTG CGA CCA TAC TCC CCC CA-3′) for the first round and GF2 (5′-GTC TTG TAA TTG GAA TGA TGG-3′), GR2 (5′-CCA AAG ACT TTG ATT TCT CTC-3′) for the second round. For PCR amplification the T1000™ Thermal Cycler (Bio-Rad, London, UK) was used with the following conditions: initial denaturation at 95°C for 3 min, then 40 cycles of denaturation at 95°C for 30 s, annealing at 60°C for 30 s (for the first round), 50°C for 30 s (for the second round) and extension at 72°C for 1 min (for the first round), 72°C for 40 s (for the second round) and a final extension at 72°C for 7 min (18). During the protocol, two negative controls (PCR water) were used for checking the possible contamination and one positive control consisting in DNA isolated from the blood of a naturally infected dog with Babesia canis (confirmed by sequencing) were included in each amplification set.
Amplification products were visualized by electrophoresis on 1.5% agarose gel stained with RedSafe™ 20,000 × Nucleic Acid Staining Solution (Chembio, UK), and their molecular weight was assessed by comparison to a molecular marker (HyperLadderTM 100 bp, Bioline, London, United Kingdom).
2.4. DNA sequencing and phylogenetics analysis
Positive samples were purified using Gel/PCR DNA fragments extraction kit (Geneaid, Taipei, Taiwan) and sent for sequencing (performed by Macrogen Europe, Amsterdam, Netherlands). The sequences were compared with those available in GenBank™ by means of Basic Local Alignments Tool (BLASTn). All sequences were analyzed and edited using MEGA X software (19). The phylogenetic analysis was performed using the same software, using the Maximum Likelihood method: Tamura 3-parameter model (20), with a discrete Gamma distribution was used to model evolutionary rate differences among sites [five categories (+G, parameter = 0.2843)] for Babesia spp., and Tamura-Nei model (20), with a discrete Gamma distribution was used to model evolutionary rate differences among sites [five categories (+G, parameter = 0.2777)] for Theileria spp. The rate variation model allowed for some sites to be evolutionarily invariable [(+I), 39.05% sites].
For the phylogenetic analysis, all the sequences were trimmed to a length of 485 and 548 base pairs for Babesia and Theileria, respectively. In total, in the analysis we included 25 unique Babesia and 23 Theileria sequences from domestic and wild ungulates, including our sequences. Hepatozoon sp. was chosen as an outgroup.
3. Results
3.1. Pathological findings
Out of 36 examined bison carcasses, in six cases, the macroscopic findings raised a suspicion of babesiosis: the post-mortem examination of these individuals revealed pale mucosae, mild to moderate jaundice, multifocal skin lesions associated with ticks (Ixodes ricinus) on the inner side of the thigh (Figure 2a), mammary gland, abdomen, ears and neck, petechial hemorrhages and echymosses on the digestive tract serosa (Figure 2b), cerebellum (Figure 2c), epicardium (Figure 2d) and pleura, splenic congestion, and severe dilation of the gallblader. Pulmonary congestion and edema, hydropericardium and reddish-brown urine were identified in only one case. Aditionally, the histological examination revealed extensive renal tubular epithelium necrosis (Figure 2e) and necrosis of periacinar hepatocytes (Figure 2f). Cytological evaluation of the spleen imprints was negative for the presence of intraerythrocytic piroplasms.
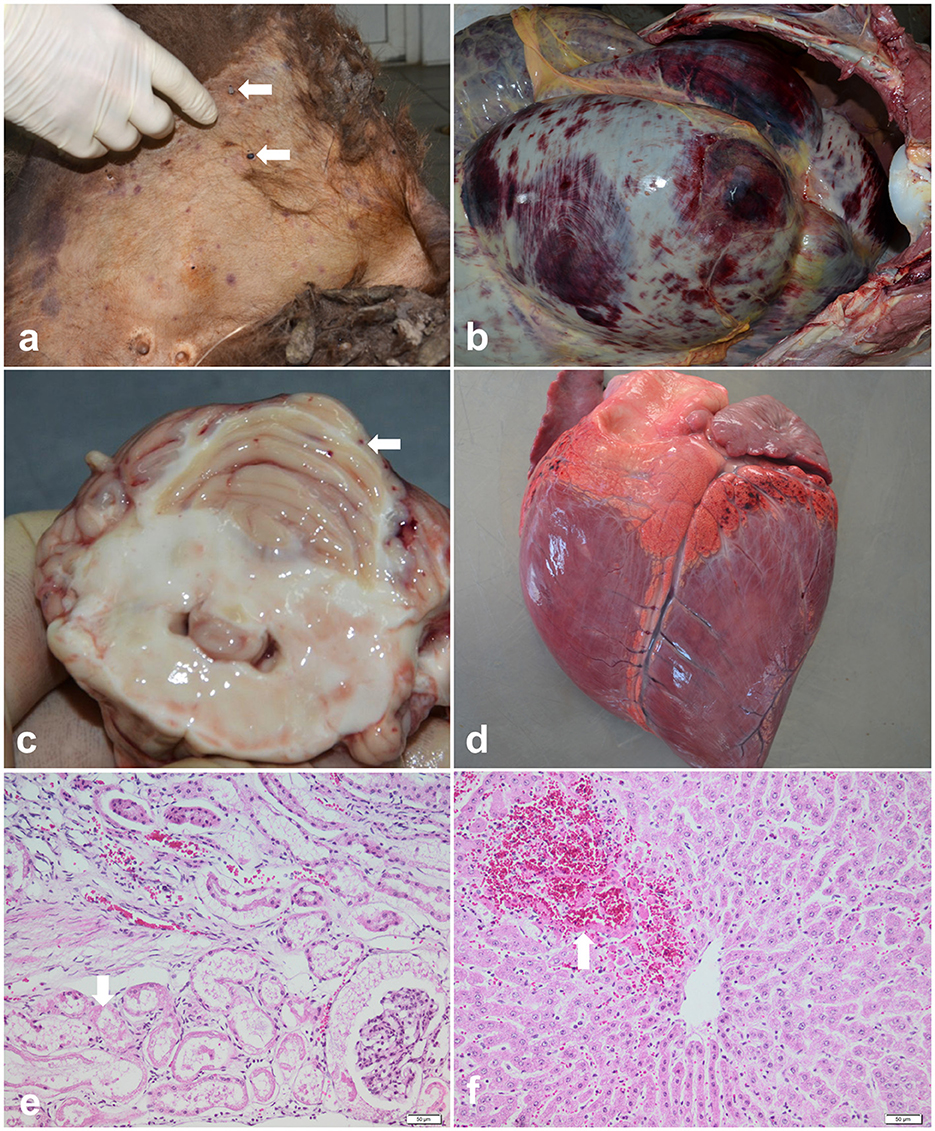
Figure 2. Pathological findings of babesiosis in American bisons (Bison bison). (a) External examination of the body showing skin jaundice and presence of ticks (Ixodes spp.) on the inner side of the thigh (arrows), case no. 1; (b) Postmortem exam of abdominal cavity revealing petechial hemorrhages and echymosses on the digestive tract serosa, case no.1; (c) Cross section through the cerebellum showing multiple petechial hemorrhages (arrow), case no.2; (d) Multifocal petechial hemorrhages on the epicardium (arrow), case no. 2; (e, f) Representative photomicrographs of the histopathological changes observed in bisons infected with Babesia spp. (case no.3): (e) extensive renal tubular epithelium necrosis and ocasionally intratubular acidophilic proteinaceous material (arrow), and (f) coagulative necrosis of periacinar hepatocytes and hemorhages (arrow), HE stain, scale bar = 50 μm.
3.2. Molecular results
One out of the six dead bisons (16.66%; spleen) was PCR-positive for the 18S rDNA gene (Taga, Cluj County). The sequence was 100% similar with sequences of Theileria isolated from cattle: T. orientalis (e.g., MH208641), Theileria annulata (e.g., MF287924), Theileria sergenti (e.g., KX375823), and Theileria buffeli (e.g., KX965722) and clustered within the same clade (Figure 3). The sequence was submitted to the GenBank database under the accession numbers: OM753106.
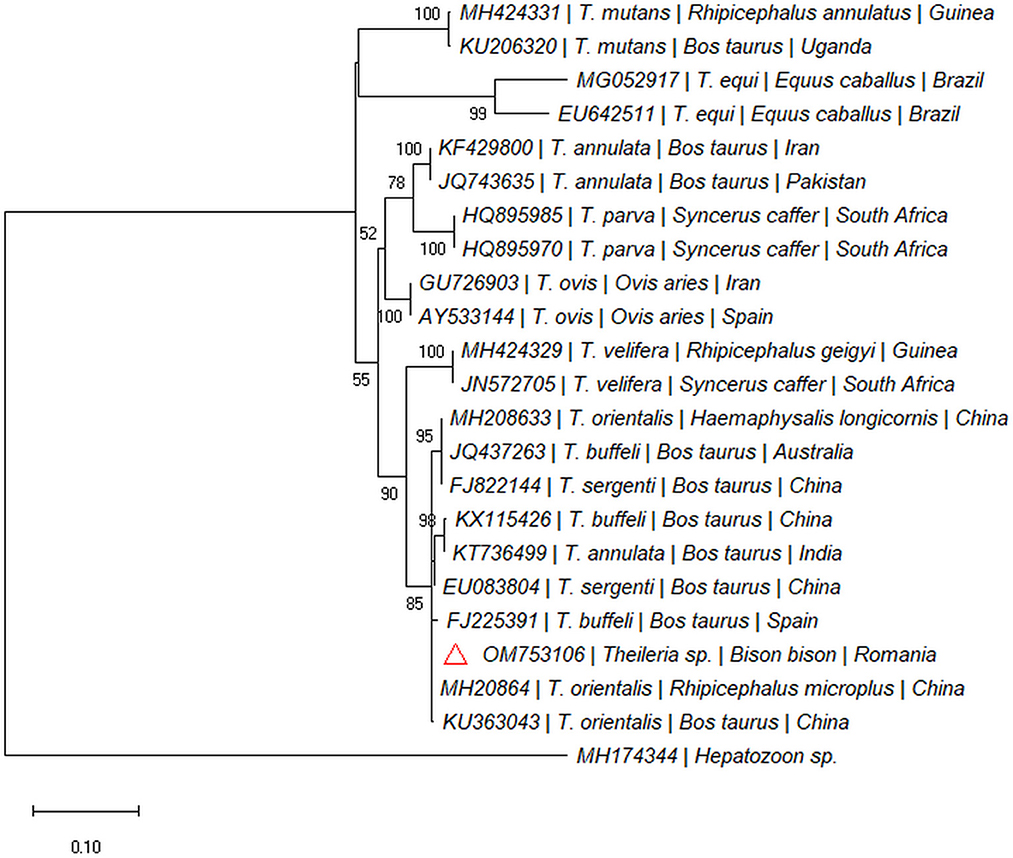
Figure 3. Pylogenetic tree constructed by maximum likelihood method on nucleotide sequences of 18S rRNA gene of Theileria sp. Hepatozoon sp. was used as the outgroup.
Two out of 97 blood samples (2.06%) collected from live animals from Recea Cristur were positive for the 18S rDNA gene of piroplasmids. We didn't detect piroplasmids in other two farms (Păticeu, Sărata). The BLAST analysis of the 18S rDNA sequences for these positive samples showed a 99.79% to 100% similarity with several B. divergens isolates (e.g., MG344772, LC477141, and KY242398). The two sequences were highly similar to each other (99%), and formed a separate clade with B. divergens isolated from cattle in Europe (Figure 4). Both sequences were submitted to the GenBank database under the accession numbers: OM753104 and OM753105.
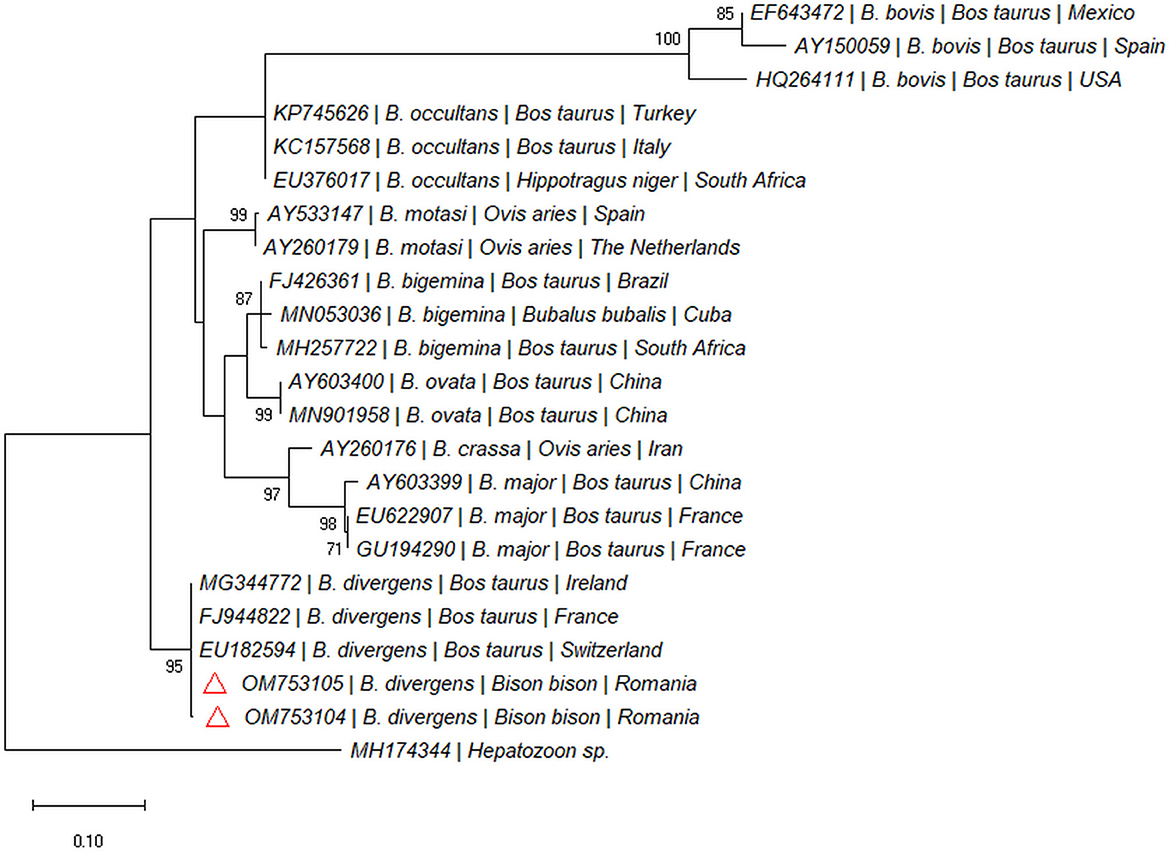
Figure 4. Pylogenetic tree constructed by maximum likelihood method on nucleotide sequences of 18S rRNA gene of Babesia spp. Hepatozoon sp. was used as the outgroup.
4. Discussion
As far as we are aware, in Europe there is no study focusing on the detection of vector-borne pathogens in B. bison and this is the first report of naturally occurring piroplasm infection in farmed American bisons from Europe. Babesiosis in animals and humans is caused by infection with tick-borne apicomplexan parasites of the genus Babesia (21). The present study reports the presence of B. divergens DNA in samples collected from live and dead bisons. The presence of Babesia was established by the association of the epidemiological, cytological and molecular findings. The animals were raised in farms for meat production and had access to pastures from which they could get the vector tick, I. ricinus. This tick species is known to be able to transmit, among others, several species of Babesia such as B. divergens, Babesia venatorum, and Babesia microti (both in humans and in animals) (22). The live animals from which the blood was collected were not searched for the presence of ticks. The ticks collected from dead animals were identified as I. ricinus in the Department of Parasitology and Parasitic Diseases, but no photo was taken then.
In Europe, different Babesia species can infect large ruminants: B. divergens (the main agent of babesiosis in cattle) (23), Babesia capreoli (free-ranging asymptomatic roe deer) (24), B. venatorum or Babesia sp. EU1 (25), B. bigemina, and B. bovis (in cattle) (26). Babesia divergens is also thought to be responsible for most European cases of human babesiosis (27). As this pathogen is transmitted by the ubiquitously distributed vector, I. ricinus, it can pose a threat of being transmitted to other animals and to humans. However, it is unknown if bisons can serve as reservoir hosts for this zoonotic haemoparasite.
Babesia in large ruminants can affect the health and economic productivity and management practices play an important role in limiting the spread of this parasite (28, 29). The overall impact of this disease on the productivity in the meat industry of bisons is still unknown, as the pathogenicity of piroplasms for bisons has been very poorly investigated. In USA, an experimental infection of B. bigemina was conducted in two splenectomized and one non-splenectomized bison calves. After the inoculation, all animals developed acute babesiosis with moderate to severe hemolysis, hemoglobinuria and hematuria (13). It was thus demonstrated that the American bison is susceptible to the infection with B. bigemina, showing once again that prevention and limiting the contact between wildlife and domestic animals is very important in order to avoid infections. Our study is the first report of B. divergens in American bisons.
Another protozooan parasite which can infect animals is Theileria sp. (30). The species that are known to infect large ruminants, especially cattle, are represented by T. annulata (tropical theileriosis) (31), Theileria parva (east coast fever) (32) and the T. orientalis group (T. sergenti, T. buffeli, T. orientalis) (33). Theileria orientalis group species are transmitted by ticks from the genus Haemaphysalis (34, 35). Different prevalences of infections with Theileria sp. were detected in cattle from Europe, ranging between 3.70 and 79.1% (36, 37), and the most common species identified was the group T. sergenti/T. buffeli/T. orientalis. The phylogenetic analysis of the Theileria sp. detected in our study showed that it clusters together with species from the T. sergenti/T. buffeli/T. orientalis group.
Our study showed the presence of piroplasmids in samples collected from B. bison, broadening the knowledge on the pathogens that can infect these animals. Further studies on prevalence and impact of babesiosis on farmed bison are recommended.
Data availability statement
The datasets presented in this study can be found in online repositories. The names of the repository/repositories and accession number(s) can be found in the article/Supplementary material.
Ethics statement
Ethical review and approval was not required for the animal study because all samples were collected during a necropsy (approved by the owner) or during sample collection for a national survey for disease. Written informed consent was obtained from the owners for the participation of their animals in this study.
Author contributions
Conceived and designed the experiment and wrote and revised the paper: AC, MT, and AM. Performed the experiment: AC, TU, and MT. Laboratory work: AC and TU. Phylogenetic analysis: AI. All authors have read and approved the final manuscript.
Funding
This study has been financed by the Department of Veterinary Pathology and the Department of Parasitology and Parasitic Diseases and was supported by the CNCS-UEFISCDI Grant Agency Romania, grant numbers PD39/2020.
Conflict of interest
MT was employed by Synevovet.
The remaining authors declare that the research was conducted in the absence of any commercial or financial relationships that could be construed as a potential conflict of interest.
Publisher's note
All claims expressed in this article are solely those of the authors and do not necessarily represent those of their affiliated organizations, or those of the publisher, the editors and the reviewers. Any product that may be evaluated in this article, or claim that may be made by its manufacturer, is not guaranteed or endorsed by the publisher.
Supplementary material
The Supplementary Material for this article can be found online at: https://www.frontiersin.org/articles/10.3389/fvets.2023.1158072/full#supplementary-material
References
1. Isenberg AC. The Destruction of the Bison: An Environmental History, 1750–1920. Cambridge: Cambridge University Press (2020). doi: 10.1017/9781108848879
2. Marsolier-Kergoat M-C, Palacio P, Berthonaud V, Maksud F, Stafford T, Bégouën R, et al. Hunting the extinct steppe bison (Bison priscus) mitochondrial genome in the Trois-Freres Paleolithic Painted Cave. PLoS ONE. (2015) 10:e0128267. doi: 10.1371/journal.pone.0128267
3. Massilani D, Guimaraes S, Brugal J-P, Bennett EA, Tokarska M, Arbogast R-M, et al. Past climate changes, population dynamics and the origin of Bison in Europe. BMC Biol. (2016) 14:1–17. doi: 10.1186/s12915-016-0317-7
4. Metcalf JL, Prost S, Nogués-Bravo D, DeChaine EG, Anderson C, Batra P, et al. Integrating multiple lines of evidence into historical biogeography hypothesis testing: a Bison bison case study. Proc R Soc B Biol Sci. (2014) 281:20132782. doi: 10.1098/rspb.2013.2782
5. Palacio P, Berthonaud V, Guérin C, Lambourdière J, Maksud F, Philippe M, et al. Genome data on the extinct Bison schoetensacki establish it as a sister species of the extant European bison (Bison bonasus). BMC Evol Biol. (2017) 17:1–11. doi: 10.1186/s12862-017-0894-2
6. Galbraith J, Rodas-González A, López-Campos Ó, Juárez M, Aalhus J. Bison meat: characteristics, challenges, and opportunities. Anim. Front. (2014) 4:68–73. doi: 10.2527/af.2014-0036
7. Clarke PR, Frey RK, Rhyan JC, McCollum MP, Nol P, Aune K. Feasibility of quarantine procedures for bison (Bison bison) calves from Yellowstone National Park for conservation of brucellosis-free bison. J Am Vet Med Assoc. (2014) 244:588–91. doi: 10.2460/javma.244.5.588
8. Epp T, Waldner C, Woodbury M. An observational study of mortality on bison farms in Saskatchewan with special emphasis on malignant catarrhal fever. Can Vet J. (2016) 57:37.
9. Salb A, Stephen C, Ribble C, Elkin B. Descriptive epidemiology of detected anthrax outbreaks in wild wood bison (Bison bison athabascae) in northern Canada, 1962–2008. J Wildl Dis. (2014) 50:459–68. doi: 10.7589/2013-04-095
10. Bras AL, Barkema HW, Woodbury MR, Ribble CS, Perez-Casal J, Windeyer MC. Clinical presentation, prevalence, and risk factors associated with Mycoplasma bovis-associated disease in farmed bison (Bison bison) herds in western Canada. J Am Vet Med Assoc. (2017) 250:1167–75. doi: 10.2460/javma.250.10.1167
11. Bras AL, Barkema HW, Woodbury M, Ribble C, Perez-Casal J, Windeyer MC. Risk factors for Mycoplasma bovis-associated disease in farmed bison (Bison bison) herds in western Canada: a case-control study. Prev Vet Med. (2016) 129:67–73. doi: 10.1016/j.prevetmed.2016.05.011
12. Findlay C, Begg T. Redwater in American bison caused by Babesia major. Vet Rec. (1977) 100:406. doi: 10.1136/vr.100.19.406-a
13. Zaugg JL, Kuttler KL. Experimental infections of Babesia bigemina in American bison. J Wildl Dis. (1987) 23:99–102. doi: 10.7589/0090-3558-23.1.99
14. Bock R, Jackson L, De Vos A, Jorgensen W. Babesiosis of cattle. Parasitology. (2004) 129:S247–69. doi: 10.1017/S0031182004005190
15. Oakes VJ, Yabsley MJ, Schwartz D, LeRoith T, Bissett C, Broaddus C, et al. Theileria orientalis Ikeda genotype in cattle, Virginia, USA. Emerg Infect Dis. (2019) 25:1653. doi: 10.3201/eid2509.190088
16. Ganzinelli S, Rodriguez A, Schnittger L, Florin-Christensen M. Babesia in domestic ruminants. In:Florin-Christensen M, Schnittger L, , editors. Parasitic Protozoa of Farm Animals and Pets. New York, NY: Springer (2018), p. 215–39 doi: 10.1007/978-3-319-70132-5_9
17. Estrada-Pena A, Mihalca AD, Petney TN. Ticks of Europe and North Africa - A guide to species identification. Cham: Springer (2018).
18. Corduneanu A, Hrazdilová K, Sándor AD, Matei IA, Ionică AM, Barti L, et al. Babesia vesperuginis, a neglected piroplasmid: new host and geographical records, and phylogenetic relations. Parasites Vectors. (2017) 10:1–8. doi: 10.1186/s13071-017-2536-3
19. Kumar S, Stecher G, Li M, Knyaz C, Tamura K. MEGA X: molecular evolutionary genetics analysis across computing platforms. Mol Biol Evol. (2018) 35:1547. doi: 10.1093/molbev/msy096
20. Tamura K, Nei M. Estimation of the number of nucleotide substitutions in the control region of mitochondrial DNA in humans and chimpanzees. Mol Biol Evol. (1993) 10:512–26.
21. Zhou X, Xia S, Huang J-L, Tambo E, Zhuge H-X, Zhou X-N. Human babesiosis, an emerging tick-borne disease in the People's Republic of China. Parasit Vectors. (2014) 7:1–10. doi: 10.1186/s13071-014-0509-3
22. Uilenberg G. Babesia—a historical overview. Vet Parasitol. (2006) 138:3–10. doi: 10.1016/j.vetpar.2006.01.035
23. L'hostis M, Chauvin A. Babesia divergens in France: descriptive and analytical epidemiology. Parassitologia. (1999) 41:59–62.
24. Malandrin L, Jouglin M, Sun Y, Brisseau N, Chauvin A. Redescription of Babesia capreoli (Enigk and Friedhoff, 1962) from roe deer (Capreolus capreolus): isolation, cultivation, host specificity, molecular characterisation and differentiation from Babesia divergens. Int J Parasitol. (2010) 40:277–84. doi: 10.1016/j.ijpara.2009.08.008
25. Fanelli A. A historical review of Babesia spp. associated with deer in Europe: Babesia divergens/Babesia divergens-like, Babesia capreoli, Babesia venetorum, Babesia cf. odocoilei. Vet. Parasitol. (2021) 294:109433. doi: 10.1016/j.vetpar.2021.109433
26. Silva MG, Henriques G, Sanchez C, Marques PX, Suarez CE, Oliva A. First survey for Babesia bovis and Babesia bigemina infection in cattle from Central and Southern regions of Portugal using serological and DNA detection methods. Vet Parasitol. (2009) 166:66–72. doi: 10.1016/j.vetpar.2009.07.031
27. Hunfeld K-P, Hildebrandt A, Gray J. Babesiosis: recent insights into an ancient disease. Int J Parasitol. (2008) 38:1219–37. doi: 10.1016/j.ijpara.2008.03.001
28. He L, Bastos RG, Sun Y, Hua G, Guan G, Zhao J, et al. Babesiosis as a potential threat for bovine production in China. Parasit Vectors. (2021) 14:1–12. doi: 10.1186/s13071-021-04948-3
29. Ozubek S, Bastos RG, Alzan HF, Inci A, Aktas M, Suarez CE. Bovine babesiosis in Turkey: impact, current gaps, and opportunities for intervention. Pathogens. (2020) 9:1041. doi: 10.3390/pathogens9121041
30. Bishop R, Musoke A, Morzaria S, Gardner M, Nene V. Theileria: intracellular protozoan parasites of wild and domestic ruminants transmitted by ixodid ticks. Parasitology. (2004) 129:S271–83. doi: 10.1017/S0031182003004748
31. Brown C. Control of tropical theileriosis (Theileria annulata infection) of cattle. Parassitologia. (1990) 32:23–31.
32. Nene V, Kiara H, Lacasta A, Pelle R, Svitek N, Steinaa L. The biology of Theileria parva and control of East Coast fever–current status and future trends. Ticks Tick Borne Dis. (2016) 7:549–64. doi: 10.1016/j.ttbdis.2016.02.001
33. Watts J, Playford M, Hickey K. Theileria orientalis: a review. N Z Vet J. (2016) 64:3–9. doi: 10.1080/00480169.2015.1064792
34. Gebrekidan H, Perera PK, Ghafar A, Abbas T, Gasser RB, Jabbar A. An appraisal of oriental theileriosis and the Theileria orientalis complex, with an emphasis on diagnosis and genetic characterisation. Parasitol Res. (2020) 119:11–22. doi: 10.1007/s00436-019-06557-7
35. Yokoyama N, Sivakumar T, Ota N, Igarashi I, Nakamura Y, Yamashina H, et al. Genetic diversity of Theileria orientalis in tick vectors detected in Hokkaido and Okinawa, Japan. Infect Genet Evol. (2012) 12:1669–75. doi: 10.1016/j.meegid.2012.07.007
36. Vasić A, Nieder M, Zdravković N, Bojkovski J, Bugarski D, Pavlović I, et al. Tick infestation and occurrence of Anaplasma phagocytophilum and piroplasms in cattle in the Republic of Serbia. Parasitol Res. (2018) 117:1813–8. doi: 10.1007/s00436-018-5867-x
Keywords: bison (North American bison), Babesia, Theileria, piroplasm, farmed
Citation: Corduneanu A, Taulescu M, Ursache TD, Ionică AM and Mihalca AD (2023) Piroplasms in farmed American bison, Bison bison from Romania. Front. Vet. Sci. 10:1158072. doi: 10.3389/fvets.2023.1158072
Received: 03 February 2023; Accepted: 13 March 2023;
Published: 29 March 2023.
Edited by:
Nicola Pugliese, University of Bari Aldo Moro, ItalyReviewed by:
Mohamed Abdo Rizk, Mansoura University, EgyptDean Konjević, University of Zagreb, Croatia
Shimaa El-Sayed, Mansoura University, Egypt
Copyright © 2023 Corduneanu, Taulescu, Ursache, Ionică and Mihalca. This is an open-access article distributed under the terms of the Creative Commons Attribution License (CC BY). The use, distribution or reproduction in other forums is permitted, provided the original author(s) and the copyright owner(s) are credited and that the original publication in this journal is cited, in accordance with accepted academic practice. No use, distribution or reproduction is permitted which does not comply with these terms.
*Correspondence: Alexandra Corduneanu, alexandra.corduneanu@usamvcluj.ro