- 1Leshan Academy of Agriculture Science, Leshan, Sichuan, China
- 2College of Life Science and Engineering, Southwest University of Science and Technology, Mianyang, Sichuan, China
Introduction: The purpose of this research was to investigate how dietary supplementation with fermented herbal residues (FCMR) affected birds' development capacity, quality of meat, gut barrier, and cecum microbiota.
Methods: 540 cyan-shank partridge birds aged 47 days were chosen and divided into two groups of six replicates each and 45 birds for each replicate. The control group (CON) received a basal diet, while the trial group decreased a basic diet containing 5% FCMR.
Results and discussion: The findings revealed that the addition of FCMR decreased FCR and increased ADG in broilers (P < 0.05). Adding FCMR increased steaming loss in broiler chicken breasts (p < 0.05). Supplementation with FCMR significantly enhanced VH/CD and VH in the bird's intestine (jejunum, duodenum, and ileum) (p < 0.05). In addition, the addition of FCMR significantly down-regulated mRNA expression of INF-γ, IL-6, IL-1β, and TNF-α and up-regulated mRNA expression of ZO-1, Occludin, and Claudin (P < 0.05). Microbial 16S rDNA high-throughput sequencing study revealed that supplements with FCMR modified the cecum microbiota, and α-diversity analysis showed that supplementation with FCMR reduced the cecum bacterial abundance in broilers (P < 0.05). At the phylum level, the relative abundance of Spirochaetota increased considerably following FCMR supplementation (P < 0.05). The broiler cecum's close lot of Prevotellaceae_UCG-001 (P < 0.05), Desulfovibrio, Muribaculaceae, and Fusobacterium (p < 0.05) reduced when FCMR was supplemented. Supplementation with FCMR can promote growth capacity and maintain intestinal health in birds by enhancing gut barrier function and modulating the inflammatory response and microbial composition.
1. Introduction
In recent years, antibiotic additives have played a significant role in preventing animal diseases and improving production performance (1). However, the massive application of antibiotics can lead to harmful strains of bacteria developing resistance, reduce the quality of livestock products, and even affect the ecological environment and threaten human health (2, 3). Therefore, the use of green and efficient methods to replace antibiotics has become popular research in the field of nutrition. Chinese medicine extract production process will produce many by-products; these by-products contain polysaccharides, alkaloids, amino acids, vitamins, and other active ingredients, which can improve animal performance by enhancing the body's immunity, and anti-inflammatory functions, as well as anti-bacterial, appetite, anti-stress, and other functions (4–7). If these herbal residues are discarded, it will result in a waste of resources and pollute the environment (8). However, due to the bitter taste of the herbal residues, feeding them directly to animals will affect their palatability and thus reduce their intake (9).
Biological fermentation technology combines fermentation engineering and enzyme engineering, which effectively reduces anti-nutritional factors in plant-based raw materials, increases active substances and bioactive ingredients in raw materials, improves feed palatability and processing performance, and makes it easy for animals to eat (10, 11). The microbial fermentation process in the cellulose cell wall, lignin and other substances is degraded, its active ingredients can be released, and the functional elements in the Chinese medicine residues are enzymatically dissolved into small molecules, which can enhance the effectiveness of the medicine (12–15). It was found that the inhibition of the growth of Staphylococcus aureus, Salmonella cholerae, and Escherichia coli may be increased by fermented herbs (9). Research has shown that fermented Chinese medicines can improve growth capacity, meat quality and immune performance in pigs (16), cattle (17), and ducks (18). Fermented Chinese medicines can regulate birds' antioxidant capacity, gut barriers, and microflora (19–21).
Gut health is strongly linked with the integrity of the gut barrier and the composition of gut microbiota (22, 23). An optimal intestinal wall prevents the invasion of harmful substances of exogenous origin and helps preserve gut balance and safeguard health (24). Therefore, this research was carried out to understand better how FCMR affects the growth, quality of meat, and intestinal health of chicks. To provide data support for the rational use of FCMR on broiler chickens and provide a scientific basis for chickens' healthy breeding.
2. Materials and methods
2.1. Animals
All broilers are sourced from a local commercial farm (Leshan, Sichuan, China) and broiler breeding trials are conducted at this commercial farm (Lihui Family Farm). At the beginning of the trial, we weighed all chickens after 12 h of starvation and selected 540 47-day-old male cyan-shank partridge chickens of similar body weight randomly divided into two groups, with six parallel pens (45 birds per pen, 120 cm−60 cm−50 cm). The rearing period continued for 42 days. The control group (CON) received a basal diet, while the trial group decreased an essential diet containing 5% FCMR. Chickens were allowed to feed and drink freely every day, and the daily amount of remaining feed was collected to calculate the daily feed intake. The chicken coop has a temperature of 18–25°C, a humidity of 45%−55%, and receives natural light every day. All chickens in each replicate were weighed after 12 h of starvation on day 42, and average daily feed intake (ADFI), average daily gain (ADG), and feed conversion ratio (FCR) were calculated based on feed consumption.
2.2. Diets and feeding
The basal feed formulas and nutrients for this experiment are shown in Table 1, and the FCMR formulas and nutrients are shown in Table 2. The Chinese medicine residues in FCMR are the by-product of the extraction or decoction of Chinese medicines with the ingredients isatis root, spatholobus stem, radix bupleuri, Poria Cocos, and Sedum sarmentosum Bunge. The FCMR is made using a synergistic fermentation method of probiotics and enzymes. Briefly, Chinese medicine residues and other ingredients (Table 2) are crushed and mixed thoroughly, then put into fermentation bags with a one-way breathing valve, fermented at 36°C for 3 days and ready for use. The probiotics used for fermentation contain Lactobacillus plantarum, Bacillus subtilis, and Saccharomyces cerevisiae. The enzymes contained: cellulase, xylanase, β-glucanase, and β-mannanase.
2.3. Sample collection
At the end of the experiment, broilers were fasted for 12 h before weighing, and one was randomly selected from each replicate, for a total of 12 birds. The broilers were euthanized by cervical dislocation and necropsied immediately. The pectoralis major muscle from the same side was then taken for meat quality analysis, and a portion was stored in a refrigerator at 4°C for meat quality trait analysis and another portion was stored at −80°C for amino acid composition analysis of the pectoralis muscle. After the empty intestine was gently washed off the contents with saline, about 2 cm of the intestine was taken and placed in 4% paraformaldehyde solution for paraffin sectioning, and then the remaining portion of the empty intestine was placed in a −80 refrigerator for gene expression measurement. Cecal contents were also collected for 16S rDNA gene sequencing.
2.4. Meat quality analysis
2.4.1. Meat quality traits
Using a piercing electrode coupled to a mobile pH-meter (Testo 205, Germany) into the quadriceps forms and pectoralis major muscle, the pH of the pectoral muscle was determined at around 45 min and 24 h postoperatively. Before use, the pH meter was validated using standard phosphate buffer at pH 4 and 7.
Using a portable color scanner, the color evaluations of the chicken meat (a* = redness, b* = yellowness, and L* = brightness) were taken (3NH Technologies, China). The measurements are collected from the sample's inner side, representing the flesh's entire surface. A white tile (a* 16.22, b* 6.23, and L* 3.76) was chosen as a reference.
Approximately 2 × 2 × 2 cm of chicken breasts were used to measure the muscle's drip loss, steaming loss, and freezing loss (25). Drip loss: the samples were measured and recorded as W1, then the powers were tied with thin string, put in an air-filled poly pouch, and hung at 4°C for 24 h, then weighed and recorded as W2. Steaming loss: the samples were measured and recorded as W3, then put in a polyethylene film, heated in a water shower at 70°C for 30 min, and weighed as W4. Freezing loss: the muscle samples were weighed and recorded as W5, placed in a plastic bag, and placed in a −20°C refrigerator for 24 h, thawed and weighed as W6.
• Drip loss = (W1 – W2)/W1 × 100 %
• Steaming loss = (W3 – W4)/W3 × 100 %
• Freezing loss = (W5 – W6)/W5 × 100 %.
2.4.2. Determination of amino acids
The preserved muscle samples were taken, and the amino acid content was determined using an amino acid auto-analyzer. Briefly, a suitable amount of well-mixed piece (about 1 g) was weighed, and the sample was hydrolyzed in a vacuum-sealed glass tube with 6 mol/L HCl for 22 h at 110 ± 1°C. The solution was filtered using a 0.45 μm membrane filter into an autosampler vial, and then analyzed by L-8900 amino acid analyzer (Hitachi LA8080).
2.5. Jejunal histomorphology
According to our previous method (26), ~2 cm of duodenum, jejunum, and ileum were removed and secured with 4% paraformaldehyde. The intestinal tissues were dehydrated using ethanol, then cleaned with xylene, followed by wax immersion, embedding, sectioning (μm), and hematoxylin-eosin (HE) staining, and observed under a microscope for photographs. The VH and CD of each intestinal segment were measured by Image-Pro Plus, software and the VH/CD values were calculated.
2.6. Jejunal gene expression
Intestinal tissues stored at −80°C were placed in RNAase-free centrifuge tubes and ground using a microtissue homogenizer. Total RNA was extracted from the intestinal tissues by the Trizol method (TaKaRa, Japan). The integrity and concentration of RNA were examined by 1% agarose polyacrylamide gel electrophoresis and micro ultraviolet spectrophotometer. The first-strand cDNA was synthesized using the kit (TaKaRa, Japan) according to the instructions. Protocol for reverse transcription: 37°C for 15 min; 85°C for 5 s. The PCR amplification reactions were carried out with the CFX96 real-time quantitative fluorescence PCR assay system (Bio-Rad, USA) according to the kit's instructions (TaKaRa). The total amount of the real-time qPCR reaction system was 20 μl. Thermocycling amplified procedures included denaturing for 15 s at 95°C, heating for 15 s at 56°C, extending for 30 s at 72°C, and continuing for 40 cycles. Using GADPH as the inner reference gene, the mRNA expression level of targeted genes was obtained by the 2−ΔΔCt approach (27). The Table 3 showed primers sequences used in this study.
2.7. 16S rDNA-based sequencing analysis
The MagPure Soil DNA LQ kit was used to extract microbial DNA from 200 mg cecal content samples obtained from all groups. A Thermo Fisher Scientific NanoDrop 2000 spectrometer and electrophoresis on agarose gels were used to assess the DNA's concentration and integrity. TksGflflex DNA polymerase (Takara, R060B) and the universal primer pairs 343F (5′-TACGGRAGGCAGCAG-3′) and 798R (5′-AGGGTATCTAATCCT-3′) were used. Afterward, PCR amplification of the high variant region V3–V4 in the 16S rDNA gene of bacteria was performed in 30 ml of the reaction mixture. Two paired-end read cycles of 250 bases each were used for reading an Illumina NovaSeq6000. The sequencing and profiling of 16S rDNA amplicons were performed by OE (Shanghai, China).
2.8. Statistical analysis
The experimental results were expressed as mean ± SD, and independent samples t-test in SPSS 23.0 software was applied to analyze the significance of differences in growth performance, meat quality, intestinal morphology, and relative levels of gene expression, with p < 0.05 indicating significant differences.
The Quantitative Insights Into Microbial Ecology (QIIME, version 1.17) was used to demultiplex and quality-filter raw pair-end sequences for microbiota characterization. For rarefaction curves and α-diversity analysis were calculated using QIIME. β-diversity was estimated using principal coordinate analysis (PCoA). R software's “vegan” and “ggplot2” packages were used to plot the findings (Version 3.4.4). The significance of microbial community differences among groups was assessed using ANOSIM with R package “vegan” Before Linear discriminant analysis (LDA) combined effect size (LEfSe) estimate the impact of the abundance of bacteria on the difference effect of bacteria among groups (LDA > 3, p < 0.05), non-parametric factorial Kruskal–Wallis sum-rank test was employed to explore the differences in the relative abundances of bacteria among groups. Correlations were analyzed using spearman correlation with the pheatmap package (p < 0.05).
3. Results
3.1. Growth performance
The effects of FCHM on the growth performance of broilers are presented in Table 4. Supplementation with FCHM significantly enhanced FW and ADG in broilers (p < 0.05). Supplementation with FCHM significantly reduced FCR in broilers (p < 0.05). Supplementation with FCHM reduced ADFI and mortality in broilers, the differences were not statistically significant (p > 0.05).
3.2. Meat quality traits
Table 5 summarizes the effect of FCHM supplementation on meat quality traits of broilers. Supplementation with FCHM did not have a significant effect on the meat color and pH of broiler breast meat (p > 0.05). However, FCHM supplementation significantly reduced drip loss (p > 0.05) and steaming loss (p < 0.05) in broiler chicken breasts.
3.3. Amino acids
The effect of the dietary addition of FCHM on the amino acid profile were presented in Table 6. The addition of FCHM decreased the level of serine (p < 0.05), which is a non-essential amino acid. Supplementation with FCHM had no significant effects on broiler muscle TAA, EAA, NEAA and DAA (p > 0.05).
3.4. Intestinal histomorphology
Light microscopic photos of the gut morphology are presented in Figure 1. It can see that supplementation with FCHM improved the height of the villi in the small broiler intestine. The effects of FCHM on broiler intestinal villi are shown in Table 7. Supplementation with RCHM significantly enhanced VH/CD and VH in the broiler intestine (duodenum, jejunum, and ileum; p < 0.05). The addition of FCHM significantly decreased CD in the duodenum and jejunum of broiler chickens (p > 0.05).
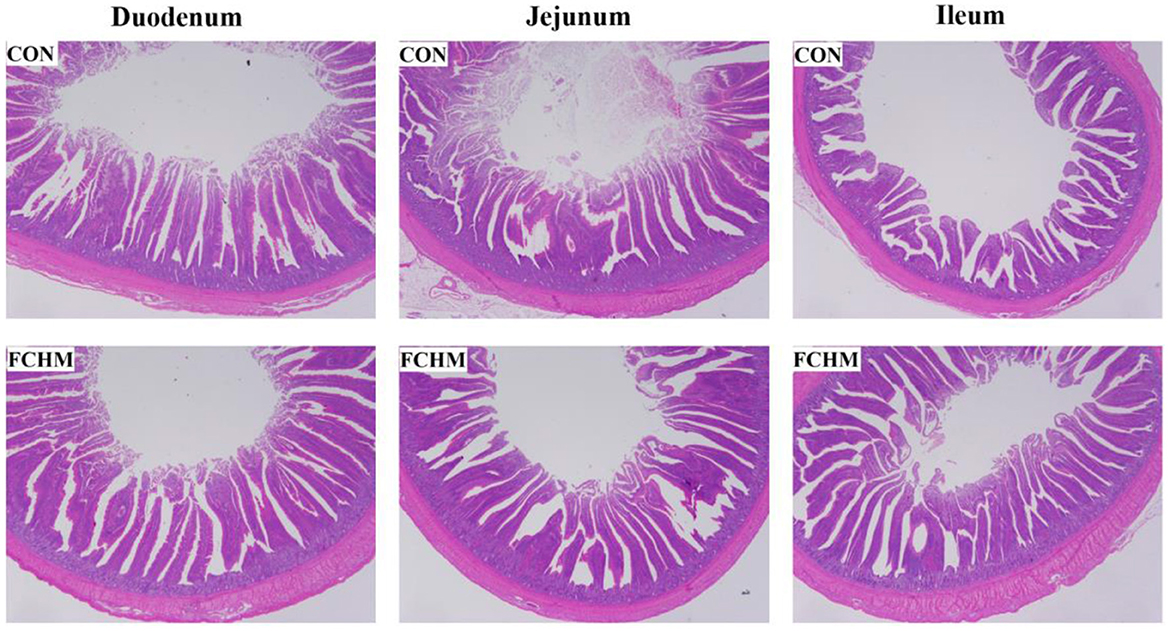
Figure 1. The morphologic structures of the gut (duodenum, jejunum, and ileum) of broiler chickens on day 42. The photos were viewed under 20× magnification. CON, control group, fed essential diet; FCHM, 5% FCHM replacement group.
3.5. The mRNA expression levels of tight junction protein and immunomodulatory genes
The expression levels of mRNA for tight junction proteins and immunoregulatory genes in broiler jejunum are shown in Figure 2. Supplementation with FCHM enhanced the expression of relative mRNA in broiler jejunum for tight junction proteins (ZO-1, Occludin, and Claudin; p < 0.05). Supplementation of FCHM significantly decreased the relative mRNA expression of immune genes (1L-1β, 1L-6, TNF-α, and INF-γ) in broiler jejunum (p < 0.05).
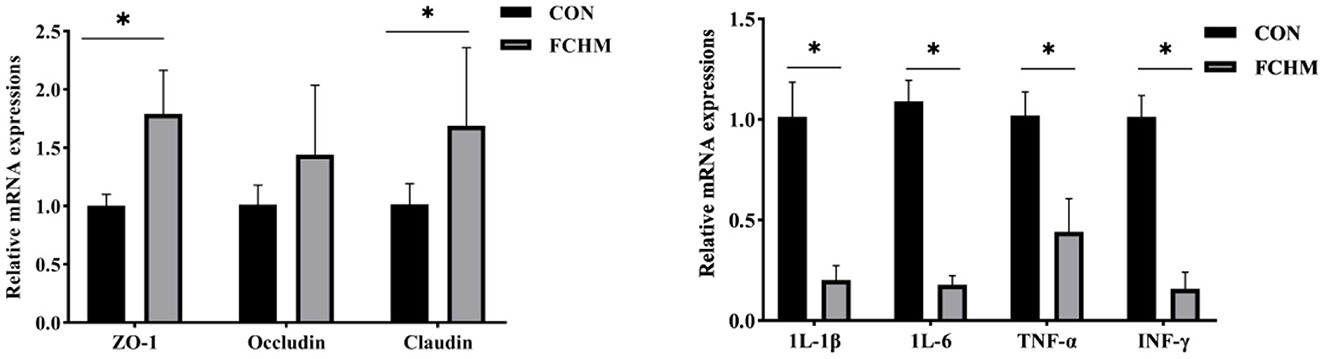
Figure 2. The mRNA expression level of tight junction protein and immunomodulatory genes in broilers jejunum. *Represents a remarkable difference among the two groups (n = 6, p < 0.05); ZO-1, zonula occluden-1; IL-1β, interleukin-1β; IL-6, interleukin-6; TNF-α, tumor necrosis factor-α; INF-γ, interferon-γ.
3.6. Cecal microbiota analysis by 16S rDNA
There were 1,962 ASVs detected in broiler cecum feces, and 768 ASVs were shared between the two groups, with the CON and FCMR groups specific for ASV numbers 683 and 511 (Figure 3). Supplementation with FCHM significantly reduced the indices of cecum Chao1, Simpson (p < 0.05), and ACE, Shannon indices (p < 0.01). Significant differences between two groups of cecum microbiota based on PCoA (p < 0.05).
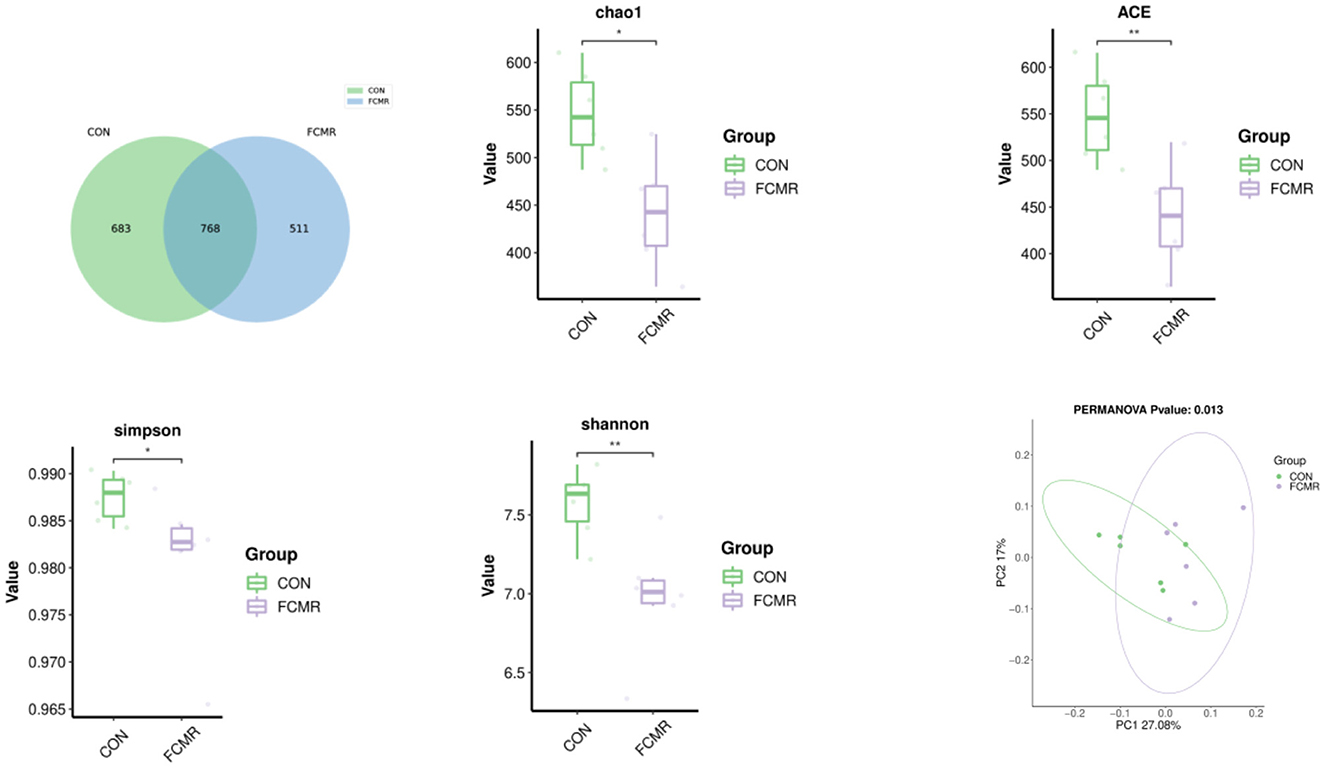
Figure 3. The effects of FCMR addition to the diet on the Venn diagram, α-diversity, and β-diversity of broiler cecum microorganisms. Principal coordinate analysis (PCoA) calculated based on weighted UniFrac distances from the ASV abundance matrix; *indicates that there is a considerable difference between the two groups (*p < 0.05, **p < 0.01).
At the phylum level, Bacteroidota and Firmicutes dominated both groupings (Figure 4A). Adding FCMR decreased the relative abundance of Proteobacteria at the gate level (6.05 vs. 5.67%). After supplementation with FCMR compared to the control group, the close lot of Spirochaetota increased considerably (0.36 vs. 4.36%, p < 0.05). At the genus level, we identified the top 15 wealthiest genera (Figure 4B). The dominant species between the two groups included Bacteroides, Rikenellaceae_RC9_gut_group, Clostridia_vadinBB60_group, Prevotellaceae_UCG-001, Prevotellaceae_Ga6A1_group. Parabacteroides, Clostridia_UCG-014, Faecalibacterium. Adding FCMR significantly enhanced the relative abundance of Clostridia_vadinBB60_group in broiler cecum (2.45 vs. 6.40%, p < 0.05). Adding FCMR significantly reduced the close lot of Prevotellaceae_UCG-001 (5.47 vs. 2.63%, p < 0.05). Feeding FCMR reduced the relative abundance of Desulfovibrio, Muribaculaceae, and Fusobacterium in the broiler cecum (p > 0.05).
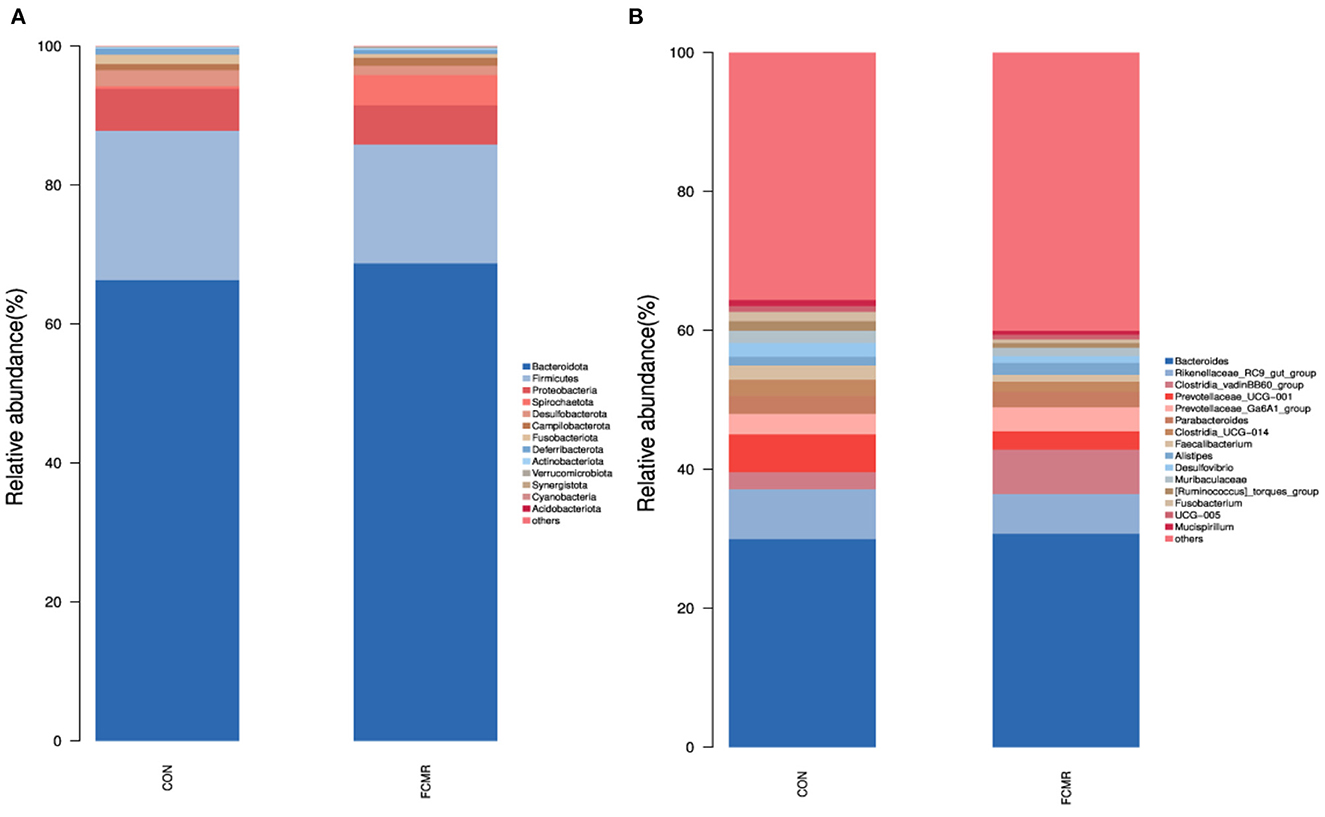
Figure 4. The microbiota composition and identification of differential species in the cecum of birds. (A, B) The phylum and genus level compositions of the bacterial communities, respectively.
Moreover, we explored species with marked differences using the LEFSE approach and indicated them with cladograms and LDA scores (Figure 5). At the phylum level, we found that Spirochaetota is a potential biomarker in the FCMR group. At the Class level, Bacilli was enriched in the CON group, and Brevinematia was increased in the FCMR group. Lachnospirales, Erysipelotrichales, and Oscillospirales were enriched in the CON group, Clostridia_vadinBB60_group, at the Order level Brevinematales, and Vibrionales were improved in the FCMR group. At the Family level, Lachnospiraceae, Ruminococcaceae, Faecalibacterium, and Erysipelatoclostridiaceae are potential biomarkers in the CON group, Clostridia_vadinBB60_group Brevinemataceae and Vibrionaceae were potential biomarkers in the FCMR group. Prevotellaceae_UCG_001, Faecalibacterium, Colidextribacter, Faecalibaculum, Ralstonia and Oscillospira we, re potential at the Genus level biomarkers in the CON group, Clostridia _vadinBB60_group, Treponema, Brevinema and Vibrio were potential biomarkers in the FCMR group.
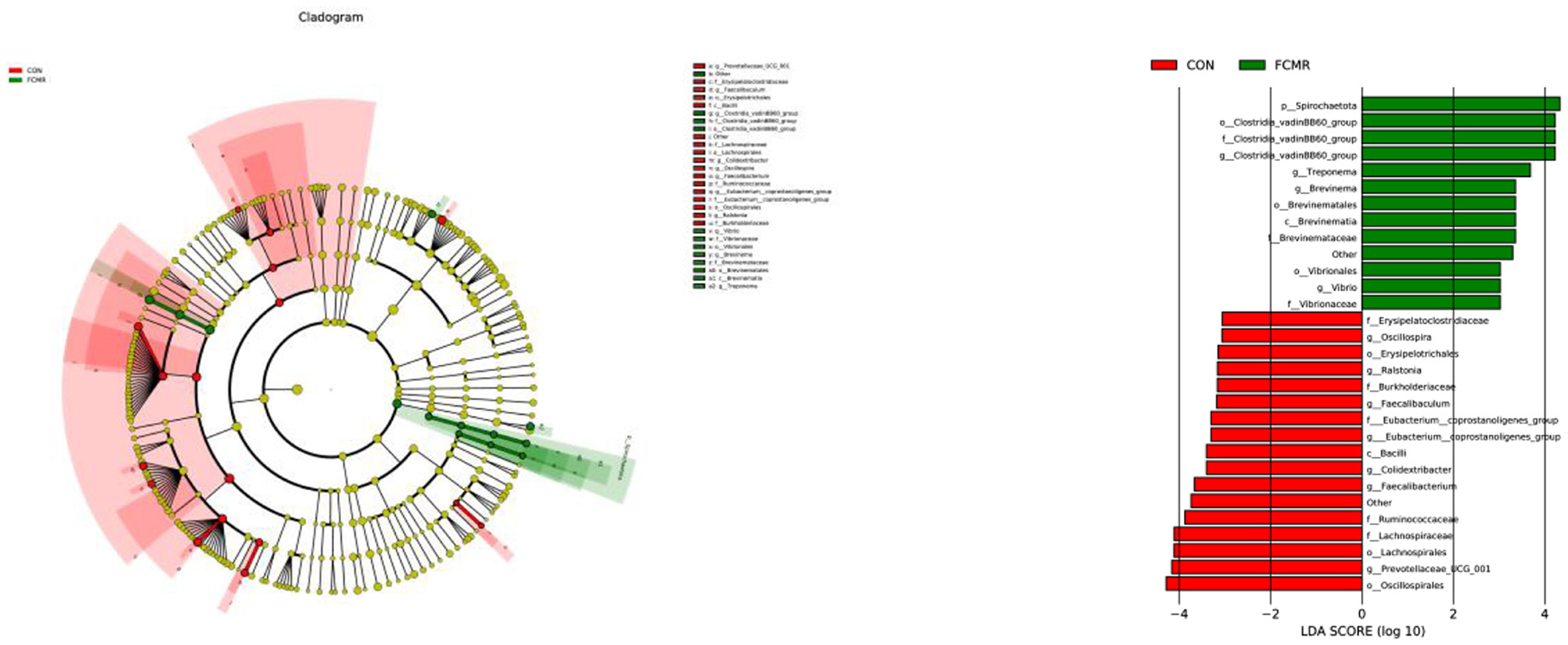
Figure 5. The microbiomes of the two groups were described using LEfSe and LDA analyses based on ASVs disparities in the (log LDA >3.0; n = 6).
4. Discussion
Microbial fermentation can boost a feed's nutritional content and make it more palatable, increase beneficial metabolites, and increase the utilization efficiency of feed as well as the growth performance of livestock and poultry (28, 29). We found that supplementation with FCMR increased ADG and decreased FCR in broilers, this may be due to the fact that supplementation with FCMR can better facilitate the digestion and absorption of nutrients in the broiler intestine, thus promoting ADG, and in agreement with this study, feeding fermented feeds can promote growth performance in poultry (30–32).
Meat quality is a critical factor in livestock production and is usually reflected by pH, meat color, freezing loss, drip loss, steaming loss, and amino acids (33). The pH level will directly affect the quality of the muscle, as the animal is slaughtered and its circulating nutrient supply is interrupted, causing a glycolytic reaction, which produces lactic acid, thus lowering the pH in the muscle (34). Consumers often base their decisions on the color of the meat, and the indications L*, a*, and b* represent the color of the flesh. Higher L* values indicate high muscle water exudation and easier formation of PSE meat. In general, the higher the a* value and the lower the b* value, the better the sensory quality of the meat (35). In the present research, we identified that FCMR supplementation had no significant effect on PH, meat color, drip loss, freezing loss, and amino acids of broilers and significantly reduced steaming loss, but data on PH and meat color of broilers showed better advantages, indicating that feeding FCMR could improve the sensory index of muscles.
The intestinal mucosa not only digests and absorbs nutrients but also serves as an essential defense barrier for the body (36). Better healthy digestion and absorption by the organism are indicated by longer gut villi and a shallower crypt depth (37, 38). This study found that FCMR supplementation increased VH and reduced CD in the small intestine of birds, possibly due to the intestinal benefits of the probiotics and bioactives in FCMR, which promote healthy intestinal growth. This suggests that FCMR can promote gut nutrient absorption and digestion in broiler chickens, thus promoting growth performance. Tight junctions are cellular gaps between adjacent epithelial cells, where claudin, occludin and ZO-1 comprise essential protein molecules in tight junctions (39). The tight junction proteins not only close the gaps among subepithelial tissues, creating a physical shield to prevent the passage of pro-inflammatory molecules, but also tight junction proteins are the main barrier to the paracellular pathway, preventing the passage of large molecules through the intestinal epithelium at the paracellular level while allowing the diffusion of ions and small molecules (40, 41). The IL family is a critical element in regulating inflammation, among which 1L-1β and 1L-6 plays a vital function in sustaining intestinal mucosal integrity and regulating intestinal immunity and are involved in many responses related to apoptosis or inflammation (42, 43). TNF-α is a classic indicator of inflammation and has been established as a critical modulator of the pro-inflammatory response (44). IFN-γ is associated with many infections, and elevated levels of IFN-γ can lead to automotive immune disease (45, 46). Chinese medicine residues contain many bioactive substances, such as polysaccharides, volatile oils, flavonoids, alkaloids and glycosides, which can promote the immunity of the body, and the bioactive substances in Chinese medicine can be improved by fermentation (47, 48). In our study, adding FCMR enhanced the relative mRNA expression of claudin, occluding, and ZO-1 and decreased the relative mRNA expression of inflammatory markers TNF-α, IFN-γ, 1L-1β, 1L-6 in broiler intestinal mucosa. Like our study, it was found that feeding herbs or fermented feeds can increase the integrity of the barrier in the intestine of poultry (49–52). Therefore, we believe that FCMR modulates gut mucosal barrier function and exhibits a strong anti-inflammatory effect, capable of reducing the number of inflammatory factors in animals.
As we all know, the intestinal microbiota plays a vital function in absorption and immune function, which helps to increase the growth performance of birds (53, 54). The intestinal microbiota regulates multiple host metabolic pathways, molding the immune response, preserving the gut mucosa integrity, and secreting certain enzymes and other metabolites (55, 56). Research has shown that adding fermented feeds to a diet can help regulate the makeup of intestinal microbes and prevent the gut from overreacting in an inflammatory way to infections, preserving intestinal health (57). Interestingly, our study found that supplementation with FCMR reduced ACE, Chao1, Shannon and Simpson indices of microorganisms in the broiler cecum, indicating a reduction in microbial diversity and abundance in the broiler cecum. This may be due to competition between dominant flora, which inhibits the value added of certain bacteria. Firmicutes and Bacteroides are the two major phyla in the poultry's intestines (58). Our study found that the most prevalent phylum in the cecum was Bacteroides. (66.32 vs. 68.70%) and Firmicutes was the second most abundant phylum. It indicates that feeding FCMR does not change the main bacterial species of the broiler cecum. Supplementation of FCMR significantly improved the abundance of Spirochaetota in the cecum of broiler chickens, which is an acid-producing bacterium that converts carbohydrates into simple volatile fatty acids(VFAS), thus providing energy to the body (59, 60). Supplementation of FCMR reduced the abundance of Prevotellaceae_UCG-001, Desulfovibrio, and Fusobacterium in broiler cecum, Prevotellaceae_UCG-001 is more sensitive to inflammatory responses (61), and Desulfurized Vibrio can cause the host to reduce sulfites and sulfates received from the diet, as well as sulfated mucopolysaccharides, resulting in the production of the cellular toxic agent hydrogen sulfide (62). Studies have shown that patients suffering from fulminant colitis showed an elevated prevalence of Desulfovibrio and a significant inflammatory response, suggesting that FCMR reduced the frequency of sulfate-reducing Desulfovibrio and exerted its anti-inflammatory effects (63). Meanwhile, a total of 12 and 16 potential biomarkers in the CON and FCMR groups were significantly different, and the role of these biomarkers in broiler gut development needs to be further investigated. In conclusion, cecum microbiome mapping suggests that supplementation with FCMR may have the potential to increase colonization of beneficial microbes in the gut, minimize the number of bacteria that are responsible for dysfunction, which will lessen oxidative damage, maintain immunological control, and eventually preserve the integrity of the intestinal barrier.
Although our study found many benefits of FCMR supplementation for broilers, this was only at 5% supplementation and in the future, we will need to study the effects of different supplementation levels on broilers, which may help allow us to identify more advantageous supplementation levels than 5%. Combining the intestinal flora metabolome with the intestinal microbiota may allow us to better understand the mechanisms by which FCMR promotes intestinal health in broiler chickens. In addition, the applicability of FCMR needs to be verified by long-term feeding.
5. Conclusion
The present study showed that supplementation of FCMR in the diet can improve ADG and reduce FCR in broilers. Moreover, supplementation of FCMR can improve intestinal health by improving intestinal barrier function, intestinal inflammatory response and modifying microbial composition. In conclusion, we conclude that supplementation with 5% FCMR is beneficial to broiler health. The understanding of the regulatory role of FCMR on intestinal health and growth performance in broiler production warrants further study.
Data availability statement
The datasets presented in this study can be found in online repositories. The name of the repository and accession numbers can be found at: NCBI; SRR23681981–SRR23681992.
Ethics statement
The animal study was reviewed and approved by Leshan Academy of Agricultural Sciences.
Author contributions
XHZ: conceptualization, methodology, formal analysis, investigation, and writing—original draft. SYL: conceptualization, methodology, and formal analysis. YLJ: formal analysis, data curation, and investigation. JCD and CPY: data curation and investigation. LJK: investigation. HDZ: investigation, supervision, and funding acquisition. XXC: investigation and supervision. All authors contributed to the article and approved the submitted version.
Funding
This work was supported by the Project of Sichuan Science and Technology Program (2021JDRC0142) and Leshan Science and Technology Plan Program (22ZDYJ0122).
Conflict of interest
The authors declare that the research was conducted in the absence of any commercial or financial relationships that could be construed as a potential conflict of interest.
Publisher's note
All claims expressed in this article are solely those of the authors and do not necessarily represent those of their affiliated organizations, or those of the publisher, the editors and the reviewers. Any product that may be evaluated in this article, or claim that may be made by its manufacturer, is not guaranteed or endorsed by the publisher.
References
1. Tian M, He X, Feng Y, Wang W, Chen H, Gong M, et al. Pollution by antibiotics and antimicrobial resistance in LiveStock and Poultry Manure in China, and countermeasures. Antibiotics. (2021) 10:539. doi: 10.3390/antibiotics10050539
2. Serwecińska L. Antimicrobials and antibiotic-resistant bacteria: a risk to the environment and to public health. Water. (2020) 12:3313. doi: 10.3390/w12123313
3. Manyi-Loh C, Mamphweli S, Meyer E, Okoh A. Antibiotic use in agriculture and its consequential resistance in environmental sources: potential public health implications. Molecules. (2018)2 3:795. doi: 10.3390/molecules23040795
4. Abdallah A, Zhang P, Zhong Q, Sun Z. Application of traditional chinese herbal medicine by-products as dietary feed supplements and antibiotic replacements in animal production. Curr Drug Metab. (2019) 20:54–64. doi: 10.2174/1389200219666180523102920
5. Kuralkar P, Kuralkar SV. Role of herbal products in animal production – AN updated review. J Ethnopharmacol. (2021) 278:114246. doi: 10.1016/j.jep.2021.114246
6. Zhu F. A review on the application of herbal medicines in the disease control of aquatic animals. Aquaculture. (2020) 526:735422. doi: 10.1016/j.aquaculture.2020.735422
7. Li L, Sun X, Luo J, Chen T, Xi Q, Zhang Y, et al. Effects of herbal tea residue on growth performance, meat quality, muscle metabolome, and rumen microbiota characteristics in finishing steers. Front Microbiol. (2022) 12:821293. doi: 10.3389/fmicb.2021.821293
8. Guo Q, Li J, Chen G, Guo X, Cheng Z, Yan B, et al. A comprehensive comparison study: the impacts of gasifying agents and parameters on Chinese Herb medicine residue gasification. Waste Biomass Valorization. (2021) 12:3059–73. doi: 10.1007/s12649-020-01037-x
9. Zou W, Huang H, Wu H, Cao Y, Lu W, He Y, et al. Preparation, antibacterial potential, and antibacterial components of fermented compound chinese medicine feed additives. Front Vet Sci. (2022) 9:808846. doi: 10.3389/fvets.2022.808846
10. Niba AT, Beal JD, Kudi AC, Brooks PH. Bacterial fermentation in the gastrointestinal tract of non-ruminants: influence of fermented feeds and fermentable carbohydrates. Trop Anim Health Prod. (2009) 41:1393. doi: 10.1007/s11250-009-9327-6
11. Olukomaiya O, Fernando C, Mereddy R, Li X, Sultanbawa Y. Solid-state fermented plant protein sources in the diets of broiler chickens: a review. Anim Nutr. (2019) 5:319–30. doi: 10.1016/j.aninu.2019.05.005
12. Chang J, Cheng W, Yin Q, Zuo R, Song A, Zheng Q, et al. Effect of steam explosion and microbial fermentation on cellulose and lignin degradation of corn stover. Bioresour Technol. (2012) 104:587–92. doi: 10.1016/j.biortech.2011.10.070
13. Hussain A, Bose S, Wang J-H, Yadav MK, Mahajan GB, Kim H, et al. Fermentation, a feasible strategy for enhancing bioactivity of herbal medicines. Food Res Int. (2016) 81:1–16. doi: 10.1016/j.foodres.2015.12.026
14. Mandebvu P, West J, Froetschel M, Hatfield R, Gates R, Hill G, et al. Effect of enzyme or microbial treatment of bermudagrass forages before ensiling on cell wall composition, end products of silage fermentation and in situ digestion kinetics. Anim Feed Sci Technol. (1999) 77:317–29. doi: 10.1016/S0377-8401(98)00247-8
15. Wang G-H, Chen C-Y, Lin C-P, Huang C-L, Lin C-H, Cheng C-Y, et al. Tyrosinase inhibitory and antioxidant activities of three Bifidobacterium bifidum-fermented herb extracts. Ind Crops Prod. (2016) 89:376–82. doi: 10.1016/j.indcrop.2016.05.037
16. Zhou H, Wang C, Ye J, Chen H, Tao R. Effects of dietary supplementation of fermented Ginkgo biloba L. residues on growth performance, nutrient digestibility, serum biochemical parameters and immune function in weaned piglets. Anim Sci J. (2015) 86:790–9. doi: 10.1111/asj.12361
17. Wang X, Xie H, Liu F, Wang Y. Production performance, immunity, and heat stress resistance in Jersey cattle fed a concentrate fermented with probiotics in the presence of a Chinese herbal combination. Anim Feed Sci Technol. (2017) 228:59–65. doi: 10.1016/j.anifeedsci.2017.03.015
18. Liu X, Cao G, Zhou J, Yao X, Fang B. The effects of Bacillus coagulans-fermented and non-fermented Ginkgo biloba on abdominal fat deposition and meat quality of Peking duck. Poult Sci. (2017) 96:2264–73. doi: 10.3382/ps/pex017
19. Liu S, Yan W, Ma C, Liu Y, Gong L, Levesque C, et al. Effects of supplemented culture media from solid-state fermented Isaria cicadae on performance, serum biochemical parameters, serum immune indexes, antioxidant capacity and meat quality of broiler chickens. Asian-Australas J Anim Sci. (2020) 33:568–78. doi: 10.5713/ajas.19.0179
20. Qiao H, Song Y, Shi H, Bian C. Fermented Astragalus in diet altered the composition of fecal microbiota in broiler chickens. AMB Express. (2018) 8:151. doi: 10.1186/s13568-018-0682-4
21. Zhang XH, Sun ZY, Cao FL, Ahmad H, Yang XH, Zhao LG, et al. Effects of dietary supplementation with fermented ginkgo leaves on antioxidant capacity, intestinal morphology and microbial ecology in broiler chicks. Br Poult Sci. (2015) 56:370–80. doi: 10.1080/00071668.2015.1030590
22. Chelakkot C, Ghim J, Ryu SH. Mechanisms regulating intestinal barrier integrity and its pathological implications. Exp Mol Med. (2018) 50:1–9. doi: 10.1038/s12276-018-0126-x
23. Natividad JMM, Verdu EF. Modulation of intestinal barrier by intestinal microbiota: pathological and therapeutic implications. Pharmacol Res. (2013) 69:42–51. doi: 10.1016/j.phrs.2012.10.007
24. Yang L, Liu G, Lian K, Qiao Y, Zhang B, Zhu X, et al. Dietary leonurine hydrochloride supplementation attenuates lipopolysaccharide challenge-induced intestinal inflammation and barrier dysfunction by inhibiting the NF-κB/MAPK signaling pathway in broilers. J Anim Sci. (2019) 97:1679–92. doi: 10.1093/jas/skz078
25. Zhou X, Wang Y, Yu J, Li J, Wu Q, Bao S, et al. Effects of dietary fermented Chinese herbal medicines on growth performance, digestive enzyme activity, liver antioxidant capacity, and intestinal inflammatory gene expression of juvenile largemouth bass (Micropterus salmoides). Aquac Rep. (2022) 25:101269. doi: 10.1016/j.aqrep.2022.101269
26. Jiang L, Zhou X, Yu J, Bao S, Li J, Wu Q, et al. Fermented tea residue improved growth performance, liver antioxidant capacity, intestinal morphology and resistance to Aeromonas hydrophila infection in juvenile largemouth bass (Micropterus salmoides). Front Mar Sci. (2022) 9. doi: 10.3389/fmars.2022.999947
27. Livak KJ, Schmittgen TD. Analysis of relative gene expression data using real-time quantitative PCR and the 2–ΔΔCT method. Methods. (2001) 25:402–8. doi: 10.1006/meth.2001.1262
28. Dawood MAO, Koshio S. Application of fermentation strategy in aquafeed for sustainable aquaculture. Rev Aquacult. (2020) 12:987–1002. doi: 10.1111/raq.12368
29. Al-Khalaifah HS, Shahin SE, Omar AE, Mohammed HA, Mahmoud HI, Ibrahim D, et al. Effects of graded levels of microbial fermented or enzymatically treated dried brewer's grains on growth, digestive and nutrient transporter genes expression and cost effectiveness in broiler chickens. BMC Vet Res. (2020) 16:424. doi: 10.1186/s12917-020-02603-0
30. Yan J, Zhou B, Xi Y, Huan H, Li M, Yu J, et al. Fermented feed regulates growth performance and the cecal microbiota community in geese. Poult Sci. (2019) 98:4673–84. doi: 10.3382/ps/pez169
31. Xie PJ, Huang LX, Zhang CH, Zhang YL. Nutrient assessment of olive leaf residues processed by solid-state fermentation as an innovative feedstuff additive. J Appl Microbiol. (2016) 121:28–40. doi: 10.1111/jam.13131
32. Devendran P, Jayachandran, S, Visha P, Nanjappan K, Panneerselvam S. Panneerselvam. Hematology and blood profile of Coimbatore sheep. Indian J Small Rumin. (2009) 15:98–101.
33. Scheier R, Bauer A, Schmidt H. Early postmortem prediction of meat quality traits of porcine semimembranosus muscles using a portable raman system. Food Bioprocess Technol. (2014) 7:2732–41. doi: 10.1007/s11947-013-1240-3
34. Matarneh SK, Scheffler TL, Gerrard DE. Chapter 5 - The conversion of muscle to meat. In: Toldrá F, editor. Lawrie's Meat Science, 9th ed. Cambridge: Woodhead Publishing (2023). doi: 10.1016/B978-0-323-85408-5.00010-8
35. Li J, Yang C, Peng H, Yin H, Wang Y, Hu Y, et al. effects of slaughter age on muscle characteristics and meat quality traits of Da-Heng meat type birds. Animals. (2020) 10:69. doi: 10.3390/ani10010069
36. Du Z, Zhu L, Dong M, Wang J, Wang J, Xie H, et al. Effects of the ionic liquid [Omim]PF6 on antioxidant enzyme systems, ROS and DNA damage in zebrafish (Danio rerio). Aquat Toxicol. (2012) 124–125:91–93. doi: 10.1016/j.aquatox.2012.08.002
37. Pham VH, Kan L, Huang J, Geng Y, Zhen W, Guo Y, et al. Dietary encapsulated essential oils and organic acids mixture improves gut health in broiler chickens challenged with necrotic enteritis. J Anim Sci Biotechnol. (2020) 11:18. doi: 10.1186/s40104-019-0421-y
38. Kiela PR, Ghishan FK. Physiology of intestinal absorption and secretion. Best Pract Res Clin Gastroenterol. (2016) 30:145–59. doi: 10.1016/j.bpg.2016.02.007
39. Pearce SC, Al-Jawadi A, Kishida K, Yu S, Hu M, Fritzky LF, et al. Marked differences in tight junction composition and macromolecular permeability among different intestinal cell types. BMC Biol. (2018) 16:19. doi: 10.1186/s12915-018-0481-z
40. Ulluwishewa D, Anderson RC, McNabb WC, Moughan PJ, Wells JM, Roy NC, et al. Regulation of tight junction permeability by intestinal bacteria and dietary components. J Nutr. (2011) 141:769–76. doi: 10.3945/jn.110.135657
41. Awad WA, Hess C, Hess M. Enteric pathogens and their toxin-induced disruption of the intestinal barrier through alteration of tight junctions in chickens. Toxins. (2017) 9:60. doi: 10.3390/toxins9020060
42. Li C, Wen R, Liu D, Yan L, Gong Q, Yu H, et al. Assessment of the Potential of Sarcandra glabra (Thunb.) Nakai. in treating ethanol-induced gastric ulcer in rats based on metabolomics and network analysis. Front Pharmacol. (2022) 13:810344. doi: 10.3389/fphar.2022.810344
43. Sims JE, Smith DE. The IL-1 family: regulators of immunity. Nat Rev Immunol. (2010) 10:89–102. doi: 10.1038/nri2691
44. Bradley JR. TNF-mediated inflammatory disease. J Pathol. (2008) 214:149–60. doi: 10.1002/path.2287
45. Zelová H, Hošek J. TNF-α signalling and inflammation: interactions between old acquaintances. Inflamm Res. (2013) 62:641–51. doi: 10.1007/s00011-013-0633-0
46. Karki R, Sharma BR, Tuladhar S, Williams EP, Zalduondo L, Samir P, et al. Synergism of TNF-α and IFN-γ triggers inflammatory cell death, tissue damage, and mortality in SARS-CoV-2 infection and cytokine shock syndromes. Cell. (2021) 184:149–68.e17. doi: 10.1016/j.cell.2020.11.025
47. Zhang X, Miao Q, Pan C, Yin J, Wang L, Qu L, et al. Research advances in probiotic fermentation of Chinese herbal medicines. iMeta. (2023) 2:1–19.e93. doi: 10.1002/imt2.93
48. Wei C, Qiu J, Wu Y, Chen Z, Yu Z, Huang Z, et al. Promising traditional Chinese medicine for the treatment of cholestatic liver disease process (cholestasis, hepatitis, liver fibrosis, liver cirrhosis). J Ethnopharmacol. (2022) 297:115550. doi: 10.1016/j.jep.2022.115550
49. Gao J, Wang R, Liu J, Wang W, Chen Y, Cai W, et al. Effects of novel microecologics combined with traditional Chinese medicine and probiotics on growth performance and health of broilers. Poult Sci. (2022) 101:101412. doi: 10.1016/j.psj.2021.101412
50. Chen Z, Dai G, Wu X, Li L, Tian Y, Tan L, et al. Protective effects of Fagopyrum dibotrys on oxidized oil-induced oxidative stress, intestinal barrier impairment, and altered cecal microbiota in broiler chickens. Poult Sci. (2023) 102:102472. doi: 10.1016/j.psj.2022.102472
51. Tsai CF, Lin LJ, Wang CH, Tsai CS, Chang SC, Lee TT, et al. Assessment of intestinal immunity and permeability of broilers on partial replacement diets of two-stage fermented soybean meal by Bacillus velezensis and Lactobacillus brevis ATCC 367. Animals. (2021) 11:2336. doi: 10.3390/ani11082336
52. Wang Y, He B, Liu K, Shi J, Li A, Cheng J, et al. Effects of long-term dietary supplementation of fermented wheat bran on immune performance and inflammatory response in laying hens. Food Agric Immunol. (2022) 33:150–66. doi: 10.1080/09540105.2021.2025346
53. Yadav S, Jha R. Strategies to modulate the intestinal microbiota and their effects on nutrient utilization, performance, and health of poultry. J Anim Sci Biotechnol. (2019) 10:2. doi: 10.1186/s40104-018-0310-9
54. Zhou X, Zhang B, Zhao X, Lin Y, Wang J, Wang X, et al. Chlorogenic acid supplementation ameliorates hyperuricemia, relieves renal inflammation, and modulates intestinal homeostasis. Food Funct. (2021) 12:5637–49. doi: 10.1039/D0FO03199B
55. Shang Y, Kumar S, Oakley B, Kim WK. Chicken gut microbiota: importance and detection technology. Front Vet Sci. (2018) 5:254. doi: 10.3389/fvets.2018.00254
56. Jandhyala SM, Talukdar R, Subramanyam C, Vuyyuru H, Sasikala M, Nageshwar Reddy D, et al. Role of the normal gut microbiota. World J Gastroenterol. (2015) 21:8787–803. doi: 10.3748/wjg.v21.i29.8787
57. Missotten JA, Michiels J, Dierick N, Ovyn A, Akbarian A, De Smet S, et al. Effect of fermented moist feed on performance, gut bacteria and gut histo-morphology in broilers. Br Poult Sci. (2013) 54:627–34. doi: 10.1080/00071668.2013.811718
58. Kohl KD. Diversity and function of the avian gut microbiota. J Comp Physiol B. (2012) 182:591–602. doi: 10.1007/s00360-012-0645-z
59. Zhu R, Wang D-H, Zheng Y, Zou H, Fu S-F. Understanding the mechanisms behind micro-aeration to enhance anaerobic digestion of corn straw. Fuel. (2022) 318:123604. doi: 10.1016/j.fuel.2022.123604
60. Yang B, Xu H, Liu Y, Li F, Song X, Wang Z, et al. Role of GAC-MnO2 catalyst for triggering the extracellular electron transfer and boosting CH4 production in syntrophic methanogenesis. Chem Eng J. (2020) 383:123211. doi: 10.1016/j.cej.2019.123211
61. Cai B, Pan J, Chen H, Chen X, Ye Z, Yuan H, et al. Oyster polysaccharides ameliorate intestinal mucositis and improve metabolism in 5-fluorouracil-treated S180 tumour-bearing mice. Carbohydr Polym. (2021) 256:117545. doi: 10.1016/j.carbpol.2020.117545
62. Kau AL, Ahern PP, Griffin NW, Goodman AL, Gordon JI. Human nutrition, the gut microbiome and the immune system. Nature. (2011) 474:327–36. doi: 10.1038/nature10213
Keywords: broilers, fermented Chinese medicine residues, growth capacity, intestinal, meat quality
Citation: Zhou XH, Li SY, Jiang YL, Deng JC, Yang CP, Kang LJ, Zhang HD and Chen XX (2023) Use of fermented Chinese medicine residues as a feed additive and effects on growth performance, meat quality, and intestinal health of broilers. Front. Vet. Sci. 10:1157935. doi: 10.3389/fvets.2023.1157935
Received: 03 February 2023; Accepted: 08 March 2023;
Published: 28 March 2023.
Edited by:
Fazul Nabi, Lasbela University of Agriculture, Water and Marine Sciences, PakistanReviewed by:
Gerson Scheuermann, Brazilian Agricultural Research Corporation (EMBRAPA), BrazilSihem Dabbou, University of Trento, Italy
Copyright © 2023 Zhou, Li, Jiang, Deng, Yang, Kang, Zhang and Chen. This is an open-access article distributed under the terms of the Creative Commons Attribution License (CC BY). The use, distribution or reproduction in other forums is permitted, provided the original author(s) and the copyright owner(s) are credited and that the original publication in this journal is cited, in accordance with accepted academic practice. No use, distribution or reproduction is permitted which does not comply with these terms.
*Correspondence: Huaidan Zhang, emhkNTIwODg4QDE2My5jb20=; Xianxin Chen, Y2hlbnhpYW54aW4yMDExQDE2My5jb20=